Abstract
Immunotoxicity is the critical endpoint used by some regulatory agencies to establish toxicity values for perfluorooctanoic acid (PFOA) and perfluorooctane sulfonate (PFOS). However, the hypothesis that exposure to certain per- and polyfluoroalkyl substances (PFAS) causes immune dysregulation is subject to much debate. An independent, international expert panel was engaged utilizing methods to reduce bias and “groupthink”. The panel concluded there is moderate evidence that PFOS and PFOA are immunotoxic, based primarily on evidence from animal data. However, species concordance and human relevance cannot be well established due to data limitations. The panel recommended additional testing that includes longer-term exposures, evaluates both genders, includes other species of animals, tests lower dose levels, assesses more complete measures of immune responses, and elucidates the mechanism of action. Panel members agreed that the Faroe Islands cohort data should not be used as the primary basis for deriving PFAS risk assessment values. The panel agreed that vaccine antibody titer is not useful as a stand-alone metric for risk assessment. Instead, PFOA and PFOS toxicity values should rely on multiple high-quality studies, which are currently not available for immune suppression. The panel concluded that the available PFAS immune epidemiology studies suffer from weaknesses in study design that preclude their use, whereas available animal toxicity studies provide comprehensive dataset to derive points of departure (PODs) for non-immune endpoints. The panel recommends accounting for potential PFAS immunotoxicity by applying a database uncertainty factor to POD values derived from animal studies for other more robustly supported critical effects.
1. Introduction
The hypothesis that chronic exposure to environmentally relevant concentrations (e.g. parts per trillion) of per- and polyfluoroalkyl substances (PFAS) may increase the risk (both frequency and severity) of immune-related adverse health responses in humans is of increasing interest to regulators, public health officials, and the general public. The immune endpoint is the critical effect endpoint used by the U.S. Environmental Protection Agency (USEPA) and European Food Safety Authority (EFSA) to establish the most sensitive toxicity value for perfluorooctanoic acid (PFOA) and perfluorooctane sulfonate (PFOS) derived to date. Associations between exposure to PFAS, in particular perfluorooctanoic acid (PFOA) and perfluorooctane sulfonate (PFOS), and immune responses have been reported in both animal bioassays (see for example DeWitt, Williams, et al. Citation2016; NTP Citation2016, Citation2019, Citation2020; Zhong et al. Citation2016) and human epidemiological studies (Grandjean et al. Citation2012; Stein et al. Citation2016; Grandjean et al. Citation2017; Budtz-Jørgensen and Grandjean Citation2018; Timmermann et al. Citation2022). However, the clinical relevance of observations at the low end of the dose/exposure range have been questioned. Among the factors that led to significant debates and/or inconclusive or controversial outcomes are the lack of systematic studies on time-course kinetics of immune triggers (stimuli, antigens) such as PFOS- (or PFOA)-induced altered developmental phases of immune response (acute, intermediate, or chronic inflammation) that often leads to multistep immune dysfunction. The overall specific hypothesis is that exposure to certain PFAS introduces stressors to immune system function, thereby increasing the susceptibility to specific diseases and weakened immune surveillance of tumors; however, this has not been clearly demonstrated by available data to date. To address the current state of science on immune effects from exposure to PFOA and PFOS, an independent expert peer review was conducted The independent expert panel was engaged using the SciPinion methods described in Kirman et al. (Citation2019) and utilizing multiple design elements to help reduce bias and “groupthink” in peer-review panels (see Methods below). As described in detail in Kirman et al. (Citation2019), independent scientific peer review via the use of blinded expert panels can advance complex scientific challenges to support decision-making. The expert panel described in this manuscript was convened to provide insight and guidance on key scientific questions related to the hypothesis that low-level exposure to PFAS causes stress to the immune system sufficient to increase susceptibility to specific diseases. The expert panel critically evaluated some of the key epidemiology and animal toxicity study data for PFOA and PFOS that may inform this hypothesis. Specifically, given the current state of the science and regulations, the panelists reviewed the weight of evidence for establishing quantitative estimates of risk associated with exposure to PFAS when considering immune effects to establish the point of departure (POD) for dose-response assessment for PFOA and PFOS as well as the potential for these data to be extrapolated to other PFAS. They grappled with common challenges for this hypothesis, including:
Do the study designs follow standard practice for scientific research on factors that may contribute to immune system dysfunction/deficits?
Are the approaches to study design and data analysis sufficient to meet the study objectives? Do the methods provide reliable means to distinguish between results that are due to chance, versus real differences in antigen-specific immune responses?
Are associations between biomarkers of immune function and exposure to PFOA and PFOS interpreted correctly, given potential covariates/confounders, complexities in describing low level chemical exposure-response relationships, and the uncertainty in their use in predicting immune system dysregulation?
Is there consistency across studies that examined similar endpoints?
2. Background
Immunotoxicity is among the adverse effect endpoints considered when developing toxicity values for PFAS to be used in regulatory criteria, e.g. in establishing drinking water benchmarks and remediation goals (see below). Review articles and technical support documents indicate that there is some evidence that PFOA and PFOS exposure may have immune modulating effects, (defined as any change to the immune system, such as changes in serum immunoglobulin levels, changes in antibody levels and antibody response, NK cell activity, delayed-type hypersensitivity response, or monocyte phagocytosis, that either suggests the chemical agent activates or suppresses the function of the immune system), although the data are viewed by some public health agencies as inconsistent, weak, and of questionable relevance to environmentally relevant human exposures (WHO and IPCS Citation2012; ATSDR Citation2021; FSANZ Citation2021; Antoniou et al. Citation2022).
In their draft technical support documents for deriving drinking water criteria for PFOA and PFOS, USEPA relied upon multiple lines of evidence, including epidemiological studies, animal studies, and mechanistic studies to select the key critical effect (USEPA Citation2021a, Citation2021b). Based on their evaluation, USEPA proposed chronic reference doses (RfDs) based on the epidemiology studies of Faroe Islands populations (Grandjean et al. Citation2012; Grandjean et al. Citation2017; Grandjean, Heilmann, Weihe, Nielsen, Mogensen Ulla, et al. Citation2017; Budtz-Jørgensen and Grandjean Citation2018). The study authors reported associations between serum PFAS levels and reductions in VAT counts for serum anti-diphtheria and anti-tetanus antibodies in infants and/or young children at some of the time-points evaluated. Although some human and animal studies with other endpoints (e.g. liver, endocrine effect, birth weight) were rated with higher confidence,1 USEPA selected the immune effect endpoints from this cohort as the most protective (i.e. lowest) critical effect for deriving PODs for the proposed RfDs for PFOA and PFOS (USEPA Citation2022a, Citation2022b). Other governmental bodies chose other endpoints and/or datasets, identifying a potential association for PFAS and immunomodulating effects, but concluding the Faroe Island studies were not robust enough for criteria development (Health Canada Citation2018; EFSA Citation2020; EFSA CONTAM Panel et al. Citation2020; OEHHA Citation2021).
The USEPA concluded that there was “generally consistent” evidence of an association between PFOA and PFOS exposure and immunosuppression, specifically focusing on the observed associations between anti-tetanus and anti-diphtheria antibody levels. This was despite wide confidence intervals and some null and negative findings (Antoniou et al. Citation2022; Zhang et al. Citation2022). USEPA acknowledges some inconsistencies in results and the lack of a “biological rationale” and suggests that although it is not possible to completely rule out other factors, the available evidence suggests that confounders/covariates were unlikely to explain the observations. USEPA also determined that there is increased incidence of some infectious diseases as a function of PFOA or PFOS exposure, although results are not consistent across studies, particularly for PFOS.
shows some of the key epidemiology studies for PFOA and PFOS that provide evidence of immunotoxicity. A meta-analysis by Zhang et al. (Citation2022) of the available epidemiology studies for PFOA and PFOS and immune effects found only PFOA and one six-carbon chain length PFAS, perfluorohexane sulfonic acid (PFHxS), to have a consistent (without heterogeneity across studies) negative association between serum levels and antibody levels. Moreover, Food Standards Australia New Zealand (FSANZ) also recently reviewed the available epidemiology literature pertaining to PFAS and immunotoxicity and concluded that
Table 1. Key epidemiological studies for human immune response to PFAS exposures (as PFAS in serum).
…the data are insufficient to establish causal relationships and it cannot be ruled out with reasonable confidence that the observed statistical associations may have been due to confounding, bias or chance. On the basis of the uncertainties and limitations in the evidence base, immunomodulation is not currently considered suitable as a critical endpoint for quantitative risk assessment of PFAS. (FSANZ Citation2021)
Controlled laboratory experimental data (in vivo and in vitro cell assays) have been widely used as supporting lines-of-evidence for the hypothesized human immunotoxicity for PFOA and PFOS. For experimental animals models, it has been generally accepted that a combination of two or three “Tier 1”2 immunotoxicity assays is sufficient to accurately predict an immunotoxic response in mice (Luster et al. 1992, Citation1993; DeWitt, Germolec, et al. Citation2016). The highest correlations between an immune test result and predicting immunotoxicity in laboratory animals were for the tests for antibody plaque forming cell (PFC) response (78% concordance) and lymphocyte counts by cell surface markers (83%) with no other test being considered a good predictor of immunotoxicity when evaluated individually (Luster et al. Citation1992). However, the extrapolation of these predictive results to humans introduces considerable uncertainty, due to qualitative and quantitative differences in immune parameters and disease progression between species (DeWitt, Germolec, et al. Citation2016).
Numerous animal toxicity studies with PFOA and PFOS provide evidence of their potential immunotoxicity under experimental conditions, such as high (parts per million) dose levels (). Most study reviews report adverse outcomes on immune system in rodents exposed to PFOA and PFOS, with lowest observed adverse effect levels (LOAELs) ranging from ∼3 to 100 mg/kg-day for PFOA and ∼1 to 50 for PFOS and no observed adverse effect levels (NOAELs) around 1 to 5 mg/kg-day for both compounds (as defined by OEHHA Citation2021 and EFSA Citation2020). However, there are also reports from several rodent studies of null or non-adverse findings for similar immune endpoints, e.g. no effects or conflicting observations on white blood cell populations and serum IgM levels and increases in serum globulin levels (e.g. Keil et al. Citation2008; Loveless et al. Citation2008; Qazi et al. Citation2010; Butenhoff, Chang, et al. Citation2012; Butenhoff, Kennedy, et al. Citation2012; Dong et al. Citation2012; Torres et al. Citation2021). Systematic reviews of the literature have been performed multiple times in the past 5 to 10 years (NTP Citation2016; ATSDR Citation2021; OEHHA Citation2021; USEPA Citation2021a, Citation2021b). The WoE indicates that PFOA and PFOS can cause immune suppression in laboratory animals although results vary.
Table 2. Key animal toxicity studies to evaluate immune response to PFOA and/or PFOS since 2016.
Currently, there are few examples of use of immune endpoints as critical effects for deriving toxicity values (e.g. RfDs). Some regulatory agencies have used other critical effect endpoints (e.g. developmental) to derive toxicity values for PFOA and PFOS, often accounting for the immune endpoint in the database uncertainty factor (see review in (Post Citation2021)). Two recent examples where immunotoxicity identified from epidemiological studies was selected to derive a POD for PFOA and PFOS are from the EFSA (EFSA CONTAM Panel et al. Citation2020) and the USEPA draft technical support documents for deriving drinking water criteria as maximum contaminant level goals (MCLGs) (USEPA Citation2021a, Citation2021b). These agencies used the animal immunotoxicity data as supporting evidence to supplement the epidemiological data.
In summary, there has been substantial debate on the selection of immune dysregulation as the critical effect for PFOA and/or PFOS, on the use of vaccine antibody titer studies as the marker for immune suppression, and on the relevance of rodent and other data to potential human health risk at low level exposure levels. The work described herein used an expert panel of toxicologists, immunologists, and risk assessors to review the available literature, provide expert opinions, participate in iterative scientific discourse, and summarize any consensus opinions and remaining disagreements for the hypothesis that PFOA and/or PFOS are immunotoxicants to humans.
3. Methods
An expert panel was engaged utilizing the methods described in (Kirman et al. Citation2019). Because risk assessment issues associated with PFAS are complex and controversial, multiple design elements were included in this review to minimize potential sources of bias and groupthink. These elements include the following: (1) a double-blinded process was adopted for panel recruitment and engagement to minimize potential participation bias; (2) objective expertise metrics were used to guide panel selection decisions to minimize potential selection bias; (3) experts were assembled into subpanels (animal data, epidemiology data, dose-response, and oversight/weight of evidence) to ensure sufficient coverage of the topic and minimize potential expertise gaps; (4) the identity of experts were masked (e.g. labeled as Expert 1, Expert 2, and so on) during all online deliberations, (5) a multi-round, modified Delphi format was adopted to collect both independent and deliberative input from the topic experts in an effort to minimize potential groupthink; (6) panelists were permitted to ask their own charge questions in an effort to minimize scope bias; (7) individual responses and comments from the panelists are provided in their entirety (Supplementary Appendix A) to ensure transparency and minimize potential reporting bias; and (8) although individual responses are provided, they are attributed anonymously (e.g. to Expert 1, Expert 2, etc.) rather than to specific panelists in an effort to provide psychological safety (i.e. scientists should not be subject to attack for expressing their scientific opinions on controversial topics).
3.1. Panel selection and engagement
Scientists with expertise in PFAS, risk assessment, and/or immunotoxicology were identified from a variety of sources including these: (1) SciPinion’s internal database of users; (2) searches for authors of recent publications on the topic of interest in online databases (e.g. Pubmed; Google Scholar); (3) searches of profiles on social media databases (i.e. LinkedIn); (4) general internet searches; and (5) referrals from other scientists. Candidates were invited to apply to this expert panel opportunity via a web app (https://app.scipinion.com).
Fourteen (14) experts were selected from the available applicants based upon a consideration of objective expertise metrics (e.g. number of publications, years of experience, key word counts in CV). The experts selected for this panel originate from seven different countries (Australia, Canada, India, Italy, Mexico, Sweden, and the United States). The combined expertise of the panel includes 16 advanced degrees (13 PhDs; 2 MDs; 1 DVM), more than 500 years of post-degree experience, and more than 2600 publications (). In bringing a diverse group of experts together for this review, expertise coverage by the panel includes those anticipated to be required for this review, including PFAS, immune system in animals (immunotoxicity, infectious diseases), immune system in humans (epidemiology, immunotoxicity, clinical), weight of evidence assessment, dose-response assessment, and risk assessment. The experts were divided into four subpanel groups: (1) animal data review; (2) dose-response analysis; (3) epidemiology data review; and (4) overall “oversight”/WOE (or rather, how the data might be best compiled by regulatory agencies for decision making). In addition to these expert panelists, a team of three toxicologists with expertise in PFAS risk assessment provided independent technical support to SciPinion and served as moderators and technical topic leads (authors GJG, JKA, PEG) for each round of questions and responses.
Table 3. Expert panel participants.
To minimize potential participation and selection bias, as well as to minimize potential groupthink, the panelists were blinded to the review sponsor, and to each other during the review. All participation in this review was performed online via a web app (https://app.scipinion.com). The review was structured using a modified Delphi format that consisted of five rounds of participation.
Round 1—During Round 1 the panelists worked independently. All panelists were tasked with reviewing a summary document and answering initial charge questions, which were prepared by the review topic leads (authors JKA, PEG) and review managers (authors SMH, CRK). To minimize potential scope bias, the panelists were also asked to submit a charge question of their own for their fellow panel members to answer in Round 3.
Round 2—During Round 2 the panelists were permitted to interact anonymously (e.g. as “Expert 1,” “Expert 2,” …; with numbers assigned randomly to each panelist). The panel was tasked with reviewing each other’s answers to Round 1 charge questions (provided as a downloadable pdf report and via online access). They were given the opportunity to interact with one another by submitting comments on each other’s answers, and rating (thumbs up or down) each other’s comments during the round.
Round 3—During Round 3 the panel was tasked with working independently in answering new charge questions, which were prepared by the review topic leads and review managers based on the discussions in Rounds 1 and 2 and also includes questions as provided by fellow panel members.
Round 4—As in Round 2, the panelists were permitted to interact anonymously. The panel was tasked with reviewing each other’s answers to Round 3 charge questions (provided as a downloadable pdf report and also via online access). They were given the opportunity to interact with one another by submitting comments on each other’s answers, and rating (thumbs up or down) each other’s comments during the round. One member of the dose-response subpanel was unable to participate in Rounds 4 and 5 due to a schedule conflict.
Round 5—During Round 5 the panel was tasked with working independently in revising all previous answers as needed and answering new charge questions prepared by the review topic leads and review managers.
The subpanels were asked different sets of questions, depending on the subpanel’s focus area. For example, the animal subpanel was asked a round of questions about the availability, quality, and applicability of immunotoxicity tests conducted in laboratory animal models, both with respect to study designs, and with respect to their extrapolation to human health in general and to PFOA/PFOS immunotoxicity. Follow-up rounds for these discussions were then open to both the animal immunotoxicity subpanel and the entire expert panel, which also included an evaluation of the animal data using the modified Bradford-Hill considerations often used to assess evidence of causality (Meek et al. Citation2014; Fedak et al. Citation2015). A true and accurate transcript of all panelists comments and discussions in included in Supplemental Materials.
3.2. Supplemental document review
The expert panel was asked to consider USEPA technical support documents as well as selected comments on the USEPA documents provided by interested parties during the external peer review process (see below). These documents provide other scientific approaches to toxicity value derivation and independent conclusions presented by other governmental agencies and the scientific community at large. For example, EFSA and USEPA concluded that the WOE is sufficient to use vaccine response to define the POD and corresponding chronic RfDs for PFOA and PFOS, while other agencies (ATSDR, OEHHA, FSANZ, WHO) did not find the evidence compelling enough to use vaccine response as the POD for criteria development.
The panelists were asked to consider the following documents:
USEPA (Citation2021a) External Peer Review Draft: Proposed Approaches to the Derivation of a Draft Maximum Contaminant Level Goal for Perfluorooctane Sulfonic Acid (PFOS) (CASRN 1763-23-1) in Drinking Water (p. 149–167, 334–337; Supplementary Appendix B)
USEPA (Citation2021b) External Peer Review Draft: Proposed Approaches to the Derivation of a Draft Maximum Contaminant Level Goal for Perfluorooctanoic Acid (PFOA) (CASRN 335-67-1) in Drinking Water (p. 134–158, 305–307; Supplementary Appendix B)
“Comments on Meeting Material for Public Meetings of the Science Advisory Board Per and Polyfluoroalkyl Substances (PFAS) Review Panel,” letter dated 30 December 2021 (3M 2021)
Perfluorooctanoic acid and Perfluorooctane Sulfonic Acid in Drinking Water. First Public Draft. California Environmental Protection Agency. (OEHHA Citation2021, p. 62–76, 176–178, 191–192)
A critical review of perfluorooctanoate and perfluorooctanesulfonate exposure and immunological health conditions in humans (Chang et al. Citation2016)
Application of benchmark analysis for mixed contaminant exposures: Mutual adjustment of perfluoroalkylate substances associated with immunotoxicity (Budtz-Jørgensen and Grandjean Citation2018)
The dose-response subpanel was also provided the following publications/reports as supplemental materials: (Weihe et al. Citation2008; Grandjean et al. Citation2012; Grandjean and Budtz-Jørgensen Citation2013; Grandjean et al. Citation2017; Grandjean, Heilmann, Weihe, Nielsen, Mogensen Ulla, et al. Citation2017).
Each subsection of panelists also had access to relevant literature and the primary studies of key importance. Panelists also had opportunities to request additional publications/reports and to identify any additional literature for fellow experts to review. The Supplemental Materials provides the transcript of all panel questions, comments and discussions.
4. Results
The expert panel engaged in iterative written discussions of a wide range of topics related to PFAS and immunotoxicity over multiple rounds, as described above. Conclusions on the animal data as supporting lines of evidence for human immunotoxicants are summarized first, followed by detailed observations regarding the human data, the strengths and weaknesses of the key human studies (Grandjean et al. Citation2012; Grandjean et al. Citation2017; Grandjean, Heilmann, Weihe, Nielsen, Mogensen Ulla, et al. Citation2017; Budtz-Jørgensen and Grandjean Citation2018) and the use thereof by USEPA. Finally, the overall conclusions reached by the panelists as well as data gaps and recommendations for further study are presented.
4.1. Animal studies
For PFOA/PFOS, a number of different animal studies and study designs have addressed immunotoxicity, however study designs and results differ significantly. The purpose of the animal effects sub-panel was to have an independent assessment whether studies of PFOA/PFOS effect on the immune system in animals are sufficient to serve as a point of departure for toxicity values or for supporting evidence for the epidemiology findings. The subpanel identified to review and comment on the animal immunotoxicity data initially responded to a series of questions and answers amongst their group.
4.1.1. Standardized immunotoxicity guidelines only provide screening-level analysis
A battery of standard immunotoxicity assays is recommended by authoritative agencies worldwide. Overall, the expert panel agreed that the current available experimental animal models for evaluating immunotoxicity could be updated and improved in many aspects, in particular when considering the available data for immunotoxicity of PFOA and PFOS. The panel was first asked to consider the strengths and limitations of the U.S. federal test guidelines for immunotoxicity (OPPTS 870.7800 Immunotoxicity—Health Effects Test Guidelines; USEPA Citation1998). The guideline describes tests that complement data from routine toxicity testing such as hematology, histopathology, and lymphoid organ weights. The following specific components are included in the guidelines (USEPA Citation1998):
Functional responsiveness to a T-cell dependent antigen, sheep red blood cells (SRBC); and
PFC assay or an enzyme linked immunosorbent assay (ELISA) as models for vaccination to a T-cell-dependent antigen in humans.
One of the requirements under the USEPA guideline is the T-cell-dependent antibody response (TDAR) assay. The TDAR is a measure of immune function that is dependent upon the effectiveness of multiple immune processes, including antigen uptake and innate immune or antigen-presenting cells (e.g. mast cells, macrophages, dendritic cell) sensitization and activation, B/plasma cell activation, T-helper cell, and antibody production using SRBC (Lebrec et al. Citation2014).
Antigen-specific IgM to a T-cell dependent antigen such as SRBC is considered one of the most predictive measures of overall immune function because proper response requires cooperation between T-cells, B-cells, and antigen-presenting cells to develop an antibody response (Luster et al. Citation1992). If the selected chemical produces a significant suppression during the above-mentioned tests, the effects on lymphocyte populations and T-cell subpopulations are determined using a flow cytometry test. When a chemical does not have a significant suppression effect in the first round of tests, a functional test for Natural Killer (NK) cells is conducted.
One key issue identified by the expert panel is that this suite of animal assays includes basic guidelines for short term testing in rodents only. When considering these guidelines for evaluating immunotoxicity due to exposure to PFOA and PFOS, the expert panel agreed that (a) these models are useful for basic screening via measurement of immune biomarkers; but (b) they do not elucidate a MoA for immunotoxicity of PFOA or PFOS. In short, association, but not causation, may be determined. The expert panel identified several key areas wherein improvements in the scientific methods being used may be warranted. summarizes the key issues recommended by the expert panel as areas of improvement for the current animal models for immunotoxicity.
Table 4. Key areas of improvement with current animal models for immunotoxicity.
The World Health Organization (WHO) also provides guidance for immunotoxicity testing and steps for a WoE approach to risk assessment (WHO Citation2012). To develop conclusions for immunosuppression hazard identification, the WHO recommends taking into account the following considerations:
Well-controlled clinical and epidemiological studies
Host resistance data (reduced resistance or tolerance to infections and/or tumors)
Immune function data (antibody production, NK cell function, mixed leukocyte reaction, cytotoxic T lymphocyte, etc.)
Observational immune assays (lymphocyte activation-deactivation [M1/M2] phenotyping, cytokines, complement lymphocyte proliferation, etc.)
Hematological data (altered white blood cells count)
Histopathological data (thymus, spleen, lymph nodes, etc.)
Organ weight data (thymus, spleen, lymph nodes, etc.)
The panel discussed the differences in endpoints selected in animal studies from those expected to be relevant for humans. Likewise, possible adverse effects on the developing immune system in humans may be difficult or impossible to assess based on rodent bioassay data due to missing comparative pharmacokinetics and pharmacodynamics data, high doses irrelevant in rodents that do not bear on realistic human exposure, and unknown predictive power of tests (Neubert et al. Citation2002).
The experts agreed that the standard rodent immunotoxicity assays provide a standardized test method to screen for potential immunotoxicants in short-term (and often high dose) exposure conditions. The subpanel identified important data gaps and recommended animal studies they considered relevant to hypothesis testing for PFOA and PFOS. To supplement the short-term studies, the subpanel recommended additional long-term studies in at least two different rodent species followed by confirmative testing in other non-rodent species. These measures would provide stronger support for establishing immunotoxicity than the short-term studies using a single species, sex, and strain of rodents. Information on MoA, especially for compounds such as PFOA and PFOS that might disrupt multiple aspects of the complex immune system, is desirable to inform human relevance.
4.1.2. Animal studies related to PFOA and/or PFOS immunotoxicity lack genetic diversity and relevant exposure levels and durations to inform human health risks
The experts reviewed and discussed the specific animal data available for PFOA and PFOS. The animal data subpanel was asked about specific biomarkers and/or immunoassays that would be considered predictive of immunotoxicity in humans. The subpanel did not identify any single animal model or study that would be considered clearly predictive of immunotoxicity in humans. The subpanel agreed that while animal models can be problematic when used to predict human toxicity, these data are preferable to human epidemiology data due to the ability to control for confounders and covariates that are more difficult, if not even possible, to control in human studies.
A limitation identified by the subpanel is the lack of species/strain diversity in the available rodent database. Immunotoxic responses in mice are highly strain-dependent and few other rodent species have been subject to the same assays (i.e. studies using rats largely only evaluated endpoints such as immune organ weights, WBC counts, and did not look specifically at immune responses). The current mouse strain (C57BL/6) used in most of the animal studies reviewed by the subpanel has a different innate immune response compared to other mouse strains, such as Balb/c mice, which may show a stronger antibody/humoral response. The panel also indicated it would be informative to use a mouse-human chimera (humanized mouse model) in evaluating immunotoxicity.
4.1.3. Animal data conclusions - modified Bradford-Hill considerations
The animal subpanel was asked to consider the extent to which available animal data support that PFOA and/or PFOS is an immunotoxicant to humans using the modified Bradford-Hill considerations (Meek et al. Citation2014). The experts were in general agreement for medium strength and specificity (3–4 rating), but were split (i.e. responses span at least two number difference in ratings) for the other considerations (consistency, coherence, analogy, biological plausibility, experimental evidence, and temporal relationship). “Coherence” of the animal data compared with the other lines of evidence (e.g. epidemiology and mechanistic) was judged to be low (responses range from 1 to 3). Two of the panel members noted the lack of a consistency in the observed patterns of effects, i.e. other studies showing heterogeneous responses (see Zhang et al. Citation2022) as a main concern for the available data. Biological plausibility was ranked in the medium category by all experts. Only one “5” rating, indicating highest confidence, was recorded for experimental evidence. Another panel member rated this category a “1,” indicating the lowest confidence. The individual panel member ratings for each Bradford-Hill criterion or consideration are provided in the Supplemental Material.
Overall, the panelists found that, while the available animal data generally support the hypothesis that PFOA and/or PFOS exposure might disrupt the immune system function, the concordance between animal data and human relevance cannot be well established due to limitations of available data and testing methods. The lack of tests in multiple strains and species was identified as a limiting factor of the current data. The experts also recommended evaluations with both sexes at lower dose levels, and a more complete measurement of immune responses, including IgG levels (rather than IgM).
4.2. Human studies
In their 2021 review in support of draft toxicity criteria, OEHHA determined that approximately one third (4 out of 13) of the studies that evaluated associations between PFOA and serum anti-tetanus toxoid antibodies report a statistically significant inverse association, and about one third (5 out of 13) of the studies report a statistically significant inverse association between PFOA and anti-diphtheria toxoid antibodies. A smaller percentage of studies with PFOS found inverse associations. Specifically, studies reporting statistically significant inverse associations with serums concentrations of PFOS and anti-tetanus and anti-diphtheria toxoid antibodies are 8% (1 out of 13) and 31% (4 out of 13), respectively. OEHHA (Citation2021) reports a statistically significant positive association between PFOS exposure and anti-tetanus toxoid antibody levels (the specific study was not identified by OEHHA).
The panel of experts were asked to consider the OEHHA (Citation2021) evaluation of the Faroe Island data in concert with additional data from a similar study by Kielsen et al. (Citation2016). In their draft document, OEHAA provides a summary table () of epidemiology findings by age group for pre- and post-vaccination VAT responses, as percent changes in IgG levels for every two-fold increase in serum levels of PFOA and PFOS. This table, recreated with slight modification for readability (), shows the inconsistent changes in VAT levels in these populations over time. At some stages, a statistically significant decrease is observed, however, at other stages the decreases are not statistically significant or positive associations were observed. For example, the seven year old PFOS measurements were positivity associated with a 45.4 percent increase in tetanus vaccine antibody titers at age 13. Results were also inconsistent between PFOA to PFOS.
Table 5. Key covariates to consider for establishing fluctuations in vaccine antibody titer (VAT).
In many of the available studies, other common measurements of reduced immune function were not included. Key questions remain on the suitability of the human data to support inference of causal effects of PFAS exposure on immune system function and what the dysregulation mechanism(s) of PFAS-mediated immunotoxicity may be. The expert subpanel on the human data were asked a series of questions about immune measurements in human populations in general, the specific Faroe Island cohort studies used by the USEPA in their recent draft RfD derivations for PFOA and PFOS, and potential covariates relevant to the use and interpretation of these data.
4.2.1. Recommendations for covariates and other metrics for immunotoxicity and the uncertainty of clinically relevant VAT threshold levels
As a starting point, the expert panel was asked if a relative increase or decrease in VAT as a function of chemical exposure is an informative metric of immunotoxicity and if there are key co-variates that are more relevant than VAT to examine in a multivariate analysis of titer levels. The expert panel was asked to consider the relevance of decreases in VAT among populations and at what level of deficiency, if known, potential impacts on human health are anticipated or predicted. As part of the discussions, the panel considered this metric as a component of immune function testing as well as the use of this metric by USEPA for establishing toxicity criteria for risk assessment.
The experts generally agreed that evaluating alterations in VAT is an informative metric with which to evaluate immunomodulation and may be a useful screening tool as it provides preliminary information on potential immunotoxicity. However, the extent to which a reduction in VAT indicates immunotoxicity should be evaluated only with an analysis of covariates including (but not limited to): type of vaccine, site of administration, age, gender, nutrient status, co-morbidities, concurrent medications, previous infections, and time post-inoculation for serum collection. The primary limitation in utilizing VAT as a marker of immune effects is the natural fluctuations and variability observed in populations based on a variety of factors, including:
Overall health
Recent/previous infections
Other diseases
Socioeconomics
Vaccine type3
Number of doses
Other exposures/medications
Based on these natural limitations, single data points are inferior for establishing changes in VAT compared to repeated measurements over time. The expert panel identified a range of covariates to consider that would improve the utility of these types of studies for toxicity assessments, shown in .
Table 6. Recommended biomarkers and metrics for establishing potential immunotoxicity in human studies and animal bioassays.
The expert panel was asked to consider the relevance of a threshold reduction in VAT to an expected disease occurrence, i.e. a clinically-relevant threshold. A few experts cited a range of 1–200 UI/mL for general vaccine threshold levels below which are considered insufficient for antibody response. However, the panel could not identify existing thresholds for most individual vaccines, with special consideration of reductions in anti-tetanus and anti-diphtheria VAT, based on individual fluctuations and immune responses. Some of the panel members cited a threshold value of 0.01 IU/mL that has been cited by the United States Food and Drug Administration (FDA) as the seroprotective level for anti-tetanus and anti-diphtheria vaccines in the 1985 Federal Register. These levels, however, were not included in the 2005 update to the document Biological Products; Bacterial Vaccines and Toxoids; Implementation of Efficacy Review for Tetanus and Diphtheria Vaccines (FDA Citation2005). Therefore, the scientific justification for the continued reference to this value is not clear. WHO also reviewed the literature supporting the threshold level of 0.01 IU/mL for tetanus antibodies but found the evidence for this value “limited” (WHO Citation2018). WHO ultimately considered this threshold a “minimum putatively protective level” for anti-tetanus antibodies, but also cited reports of disease incidence with measured serum antibody levels above the 0.01 UI/mL threshold level. Thus they concluded that this threshold “may not be considered a guarantee of immunity under all circumstances” with an aim to “sustain high antibody concentrations throughout life” (WHO Citation2018).
The expert panel was not aware of additional risk-based levels that could serve as a threshold for defining a clinically significant reduction in VAT counts for either anti-tetanus or anti-diphtheria antibodies. The majority of the panel noted that the variability in individual immune responses would likely make determining such a threshold impossible for most vaccines. Only one panel member was confident that a limit could be established but cautioned that any threshold or level of reduction in antibody response would need to be vaccine-specific. The panel discussed the potential difficulties in designing studies to establishing vaccine-specific thresholds for humans. For example, in the United States where childhood vaccines are available to the general public, the disease prevalence for diphtheria and tetanus is low. In cases such as these, small but significant decreases in VAT are unlikely to have an observable effect on the overall disease incidence for that virus in a population when compared to viruses with much higher disease prevalence, such COVID or influenza, which are more likely to have an observable effect in a smaller sample size.
Overall, the panelists highlighted many questions and limitations associated with the use of VAT alone as a critical effect endpoint. Better analyses of covariates, better justification for a VAT threshold (i.e. if response is considered adverse), and better meta-analyses were suggested as advances needed to provide more conclusive support for this endpoint.
As the panel supported VAT changes as only one informative metric of immunotoxicity, they were asked to describe other metrics or biomarkers that would be in addition to or as a replacement for VAT changes for evaluating the complexities of chemical injuries to the immune system. The panel provided a wide range of metrics (e.g. biomarkers, immunoassays) currently in use, but they could not identify a single parameter as the most indicative of immunotoxicity (). The most recommended biomarkers, however, were measurements of immunoglobulins (e.g. IgE, IgG, IgM), interleukins (e.g. IL-1, IL-6), and natural killer (NK) cells in serum or blood with a suite of measurements receiving the strongest support from the expert panel. Overall, owing to the complexity of the immune system, immune suppression is best evaluated in human cohorts by evaluating viral or bacterial disease incidence rates as a function of PFAS blood levels. Thus far, there is mixed and inconclusive evidence on the association between PFAS exposure and incidences of infectious diseases (see (Antoniou et al. Citation2022) for a review of those data).
4.2.2. Adequacy of the Faroe Island cohort studies
The expert panel was asked to consider USEPA’s technical support documents for the derivation of draft MCLGs and, in particular, use of the epidemiological studies of the Faroese populations to derive RfDs for criteria development (Grandjean et al. Citation2012; Grandjean et al. Citation2017; Grandjean, Heilmann, Weihe, Nielsen, Mogensen Ulla, et al. Citation2017; Budtz-Jørgensen and Grandjean Citation2018). The entire panel was asked to agree/disagree with the following statements:
The studies should serve as the primary basis for the derivation of the RfD and MCLG.
0 of 13 panel members4 agreed with this statement.
The study should be considered as a candidate for RfD development but should not be selected as the primary basis.
4 of 13 (31%) panel members agreed with this statement.
The study should be considered only qualitatively and a different study/endpoint should be used for quantitative risk assessment.
8 of 13 (62%) panel members agreed with this statement.
This is illustrated in . For the epidemiology subpanel group, three of the four epidemiologists answered “yes” when asked if the study design and statistical analysis for the Faroe Islands cohort were appropriate to control for potential confounding factors that may contribute to variability in serum antibody titer levels. After reviewing these data and the supplemental documents, the entire panel of experts was asked what degree of confidence they had in the finding of a causal association between exposure to PFOA and PFOS and immunosuppression based on the Faroe Island cohorts (1 being the lowest confidence and 10 being the highest confidence). A majority of the panelists (8 of 13, or 62%) rated the key study as having moderate to low confidence (score of 5 or less). Three panel members (23%) scored the data with a 6 or 8 rating and two panelists (15%) scored their confidence in the Faroe Island cohorts as high (9 or 10).
Figure 1. Panelist responses to how the Budtz-Jørgensen and Grandjean (Citation2018) study should be used in a risk assessment for PFAS/PFOA.
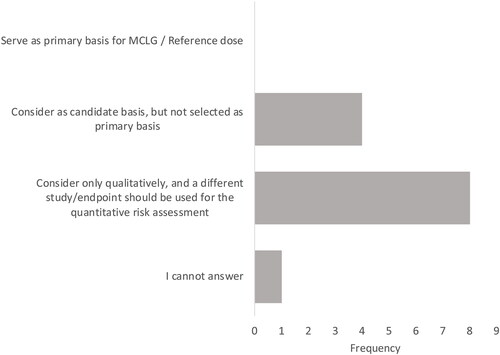
None of the panelists indicated that the Faroe Island study should be used as a primary basis for a toxicity value or MCLG, while eight (62%) said it should be considered only qualitatively and that a different study/endpoint should be used for the quantitative risk assessment (see ). Several panelists indicated that the reduced VAT from the Faroe Island cohort could be used as part of the WOE, but these data do not provide sufficient evidence for quantitative risk assessment.
Several weaknesses and uncertainties were identified in the key studies and discussed over multiple rounds by the expert panelists. For example, a key uncertainty is that the lack of an unexposed/unvaccinated control group in the cohort studies only allows for identifying a statistical difference in some of the cohorts as a function of serum PFOA/PFOS levels pre- and post-vaccinations and can tell little about the general health of the population (Grandjean et al. Citation2012; Grandjean et al. Citation2017; Grandjean, Heilmann, Weihe, Nielsen, Mogensen Ulla, et al. Citation2017; Budtz-Jørgensen and Grandjean Citation2018). The experts were in agreement that it is not clear in the Faroe Island cohort studies that the observed decreases in serum anti-diphtheria and anti-tetanus antibodies are indicators of actual increased risk of adverse public health outcomes (e.g. disease outbreaks) or an individual’s potential immune response from exposure to a particular virus or in the case of tetanus and diphtheria, bacterial toxoids (i.e. disease occurrence). Instead, these reductions in VAT may only be one measure of the overall status of the individual’s immune system at that point in time.
The expert panel’s overall conclusion was that the Faroe Island studies used by USEPA (Grandjean et al. Citation2012; Grandjean, Heilmann, Weihe, Nielsen, Mogensen Ulla, Timmermann, et al. Citation2017; Grandjean, Heilmann, Weihe, Nielsen, Mogensen Ulla, et al. Citation2017; Budtz-Jørgensen and Grandjean Citation2018) are not convincing, on their own, that immune effects observed in the Faroe Island populations were the result of chemical injury by PFOA or PFOS and, therefore, these studies should not be used to derive a POD for toxicity criteria. The panel members who said they would consider these data for candidate selection (but not as the primary basis for criteria development), stated that while these epidemiological studies do provide some evidence of a possible observable effect in these populations, criteria development should rely on multiple high-quality studies. As such, the current group of studies relied on by USEPA suffer from weaknesses in study design (e.g. lack of matched controls, unvaccinated control population, covariate analysis) that should preclude these studies from being used as the primary basis for criteria development.
4.2.3. Assessment of covariates—PCB and Hg co-exposure was not seen as a significant limitation of the Faroe Island cohort studies; however, source of infant milk should have been included as a covariate
During the USEPA Science Advisory Board review of the Agency’s technical support documents, numerous public stakeholders provided technical comments on potential covariates not adequately addressed by Grandjean and colleagues in their analysis of the Faroese cohorts. Two specific examples of potential covariates were raised to the expert panelists for discussion: (1) covariates related to co-exposure to other immunotoxicants and (2) covariates related to source of breast milk.
Co-exposure to PCBs and mercury in the Faroe Island cohorts was mentioned by some public commentors and panel members as a potential concern, though others felt that the co-exposure was adequately accounted for in the evaluation. Because the Faroe Islands study population was also exposed to contaminants linked to immunotoxicity, including methyl mercury and PCBs, there is a potential for results to be incorrectly attributed to PFAS. Biological samples (i.e. serum, breast milk) were collected at multiple time points and ages in the Faroese cohort. Grandjean et al. (Citation2012) state, “Results adjusted for PCBs in milk and five-year serum as predictors of PCB immunotoxicity were not materially different,” however, it was the 18-month serum PCB result that demonstrated the strongest association with antibody levels at five and seven years (not the maternal or five-year serum PCBs), based on work by these same authors reported by Heilmann et al. (Citation2010). Additionally, EPA used the serum PFOA at age 5 and tetanus antibody response at age 5, and serum PFOS at age 5 and diphtheria antibody response at age 7 as the statistically significant dose metric and time periods for their toxicity values. These specific relationships were not explored with regard to PCBs. Overall, the experts did not feel that this was an inadequate evaluation and did not raise significant concern regarding potential covariates with methyl mercury. One expert said that the co-exposure to mercury in the Faroe Island study would not likely impact immunotoxicity endpoints regardless, as the evidence linking low level exposure to either of these compounds with immunotoxicity is weak (Karagas et al. Citation2012).
Additional population descriptor variables that have been examined in multivariate analyses of PFAS and immunotoxicity endpoints include source of milk during infancy (breastfed vs formula fed), and duration of breastfeeding period. Some studies have attempted to control for co-exposure to contaminants (examples include other PFAS, PCBs) measured in maternal serum and/or serum at different time points following birth. Three panel members responded to a question related to the extent to which the source of milk (maternal breast milk vs formula) could serve as a potential explanatory variable for differences in antibody levels when evaluating immune system biomarkers in infants and young children. The three panel members agreed that the source of milk could partially explain the differences in antibody levels and should be considered a potential covariate to the Faroe Island cohorts. Breast milk may simultaneously increase dietary exposure to PFAS in breastfed infants and enhance antibody production associated with certain vaccines. Therefore, the type (i.e. breast milk, formula, enhanced formula), quantity, and duration of consumption of a milk source may each be a relevant covariate in studies that examine correlations between serum PFAS and antibody titer levels.
Breast milk contains numerous protective factors against infectious disease (e.g. antibodies and anti-idiotypic antibodies [“anti-antibodies”], cytokines, anti-inflammatory factors, growth factors, macrophages, granulocytes, T and B lymphocytes) and may influence both passive and long-lasting active immunity, including vaccine response in infants (Hanson Citation1998; Hanson et al. Citation1996; Jackson and Nazar Citation2006). Some, but not all studies have shown stronger serum antibody responses, e.g. higher serum IgG to diphtheria toxoid (Hahn-Zoric et al. Citation2008), to both tetanus and diphtheria toxoid vaccines among breastfed infants when compared to non-breastfed infants (Hanson Citation1998). Hanson (Citation1998) also noted that studies that did not show a stronger antibody response for breast-fed children were often focused on live attenuated vaccines5 such as against poliovirus (OPV), influenza, and rotavirus in areas where these diseases are endemic. One hypothesis for these observations is that effective immunization with live oral virus vaccines depends on viral replication, which could be inhibited by secretory immunoglobulin A (sIgA) antibodies that are present in large quantities in breast milk (Hanson Citation1998; Jackson and Nazar Citation2006; Anderson Citation2019). However, several studies with Haemophilus influenzae type b (Hib) and tetanus toxoid conjugate vaccine also did not show higher responses among breastfed infants. It is unclear if these findings may be partly attributable to the definition of breastfeeding used, duration of breastfeeding, and/or immunologic status of the mother and grandmother (Hanson Citation1998). This is an issue that has not been adequately explored in the evaluation of the epidemiological data on PFOA and PFOS, or any potential immunotoxicant, and immune dysregulation.
4.2.4. Human data conclusions—modified Bradford-Hill consideration results
The four epidemiology subpanel members were asked to rate their confidence (1 being low and 5 being high) in the causal relationship between PFOA/PFOS exposure and immunotoxicity using the epidemiology summarized in the review material and modified Bradford-Hill considerations (USEPA Citation2005). The panelists provided split responses to the questions regarding most of the Bradford-Hill considerations (). The most common response ratings for the eight Bradford-Hill considerations questions ranged between 1 and 3, indicating an overall low confidence in the human data as a basis for concluding that there is a causal association between PFOA/PFAS exposures and immunotoxicity. For three considerations - biological plausibility, specificity, and temporal relationships - the panel were split between very low confidence ratings of “1” and high ratings of confidence (ratings of “4” or “5”). The most agreement was associated with coherence, which the panel voted between ratings of “2” and “3,” which considers cause and effect from other lines of evidence. The remaining considerations (strength, consistency, analogy, and experimental evidence) ranged from moderately to low-rated (between 1 and 3) with “2” and “3” ratings. The only “5” responses, indicating high confidence, were from the same panel member.
Figure 2. Mean confidence ratings by subpanel for each of the modified Bradford Hill criteria for causality.
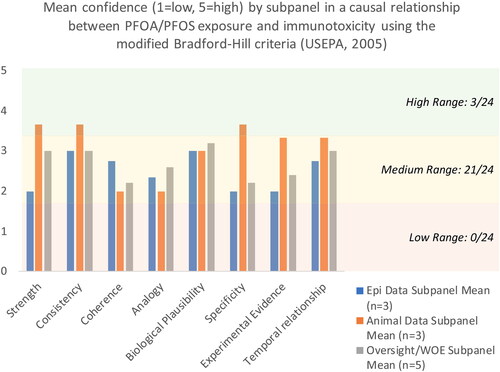
Overall, the experts largely provided “low” confidence scores for the eight considerations, indicating that the evidence provided by the analysis of the Faroe Island cohorts demonstrating a causal association between PFOA/PFAS exposure and immunotoxicity is considered weak by most of the expert panel.
4.3. Extrapolation to other perfluoroalkyl acids (PFAAs)
PFOA and PFOS are typically not the only PFAS to which humans are exposed. Human biomonitoring data consistently demonstrate the detection of other PFAS in serum (see, for example, CDC Citation2022). The consideration of potential risks associated with the cumulative exposure to various PFAS, and the extrapolation of data from well-studied PFAS, such as PFOA and PFOS, to PFAS with fewer data, present substantial challenges for risk assessors. A recent SciPinion independent expert panel evaluated the available data and proposed best practices for grouping PFAS for the purposes of human health risk assessment. That panel concluded that extrapolations across PFAS should only be done as a conservative screening assessment, and that extrapolation should be better informed by chemical-specific toxicological information and information on MoA (Anderson et al. Citation2022). In this “grouping” study, all expert panelists were asked to rate on a scale of 1 to 5 (1 = extrapolation not supported; 5 = extrapolation strongly supported) how well the evidence regarding PFOA and PFOS and immunotoxicity supports extrapolation to other PFAAs. As shown in , the majority of panelists responded with rating scores of 1, extrapolation is not supported (N = 4), or with the low score of 2 (N = 3). Reasons given included these: data limitations for other PFAAs; differences in half-lives; weak evidence for PFOA and PFOS themselves with no well-performed systematic review of meta-analysis that supports the endpoint overall; and lack of MoA information. The prevailing opinion was that each PFAS needs to be evaluated individually and that extrapolation of data from PFOA and PFOS to other PFAAs is not supported at this time.
5. Conclusion—PFOA/PFOS POD based on immunotoxicity is not supported by the available data
Overall, 12 of the 13 (92%) panelists agreed with the following summary of their review of evidence supporting immunotoxicity as the critical effect endpoint to define a POD for PFOA and PFOS (see Supplemental file, Appendix A, p. A-106, Question 5.7):
Overall, the panel members generally agree that while there is some evidence that PFOA and PFOS may be immunotoxicants, the current human data are not sufficient to support the use of human data in the derivation of reference dose values, and that the animal data suffer from limitations including the predominant use of high dose, short-term exposures in a single strain of mouse with uncertain human relevance/concordance.
Furthermore, all of the panel members agreed that the data from the Faroe Islands cohorts should not be used as the primary basis for deriving PFOA/PFOS toxicity values for human health risk assessments. The mean responses to the Bradford Hill considerations were low to moderate across all subpanel expert groups (see )—indicating low to moderate confidence in a causal association between PFOA and/or PFOS exposure and immunotoxicity.
The experts agreed that there is at least some evidence that PFOA and PFOS exposure may have immune modulating effects. However, consistent with most agency approaches, the panelists support accounting for the potential PFOA and PFOS immunotoxicity by applying a database uncertainty factor (UFD) to the POD values derived for other more robust, critical effect endpoints. Although the selection of 3 or 10 for the UFD would be chemical- and assessment-specific, taking into account the totality of other potential RfDs, the quantitative span of those values, and other potential database uncertainties, the experts agreed that the UFD would be protective for both immune effects and other sensitive endpoints not evaluated or included. Animal toxicity studies provide a comprehensive dataset from which to derive a POD for other health effect endpoints; the immune endpoints should not be used.
Notes
The Faroe Island epidemiology data were rated with medium confidence.
As described in Luster et al. (Citation1992), Tier 1 assays include those for cell-mediated immunity, humoral-mediated immunity, as well as immunopathology, and are designed to demonstrate potential immunotoxic doses without inducing overt toxicity.
During peer-review for publication, one reviewer suggested that the addition of pertussis to the booster vaccine be evaluated as a covariate. While this was not discussed by our panel, the authors agree that this is another potential covariate that should have been considered.
One panel member abstained from rating the studies.
Examples of live attenuated vaccines include these: measles, mumps, and rubella (MMR); varicella; polio. Examples of inactivated vaccines include these: tetanus, diphtheria, acellular pertussis (Tdap); human papilloma virus; meningococcus, haemophilus, pneumococcus, hepatitis A. Standard influenza vaccines vary depending on route of administration: inhaled vaccine is live; injected vaccine is inactivated (Anderson Citation2019).
Abbreviations | ||
Hib | = | Haemophilus influenzae type b |
IgG | = | immunoglobulin G |
LOAEL | = | lowest-observed-adverse-effect-level |
MCLG | = | maximum contaminant level goal |
NOAEL | = | no-observed-adverse-effect-level |
OPV | = | polio virus |
PFAS | = | poly/perfluoroalkyl substances |
PFC | = | plaque forming cell |
PFHxS | = | perfluorohexane sulfonic acid |
PFOA | = | perfluorooctanoic acid |
PFOS | = | perfluorooctane sulfonate |
POD | = | point of departure |
RfD | = | reference dose |
SRBC | = | sheep red blood cells |
sIgA | = | secretory immunoglobulin A |
VAT | = | vaccine antibody titer |
WHO | = | World Health Organization |
WOE | = | weight of evidence |
Supplemental Material
Download MS Word (929.6 KB)Acknowledgments
The authors extend an additional thank you to Drs. Yang Cao (Örebro University Hospital & Örebro University), Wendy Oddy (University of Tasmania), Pierluigi Cocco (University of Cagliari), Douglas Weed (DLW Consulting Services, LLC), Peter Vogel (St Jude Children’s Research Hospital), Paul Villeneuve (Carleton University), and Kenny Crump (Independent consultant) for their participation and valued contributions as members of the expert panel (but who declined to review the manuscript). We would like to thank Dr. Michael Luster (Luster Assoc. LLC) for his expert panel participation and for his very thoughtful comments on the draft manuscript. Lastly, we would like to thank the journal peer reviewers for their insightful comments on the draft manuscript. The manuscript is improved as a result of their input.
Declaration of interest
Funding for this project was provided by the American Chemistry Council (ACC). The funding source was not disclosed to the experts during their deliberations and ACC had no input into the selection of the experts, the charge questions, or results from the panel review. The conclusions of this review are those of the authors. None of the opinions expressed by any expert involved in this project represents an official position of the affiliated agency or employer. Member company representatives of ACC were given the opportunity to review the draft manuscript for completeness and clarity purposes, but authors retained full control over the review content. Authors CRK and SMH are owners of SciPinion, and therefore have a financial interest in this work.
Correction Statement
This article has been corrected with minor changes. These changes do not impact the academic content of the article.
References
- Abraham K, Mielke H, Fromme H, Völkel W, Menzel J, Peiser M, Zepp F, Willich SN, Weikert C. 2020. Internal exposure to perfluoroalkyl substances (PFASs) and biological markers in 101 healthy 1-year-old children: associations between levels of perfluorooctanoic acid (PFOA) and vaccine response. Arch Toxicol. 94(6):2131–2147.
- Anderson JK, Brecher RW, Cousins IT, DeWitt J, Fiedler H, Kannan K, Kirman CR, Lipscomb J, Priestly B, Schoeny R, et al. 2022. Grouping of PFAS for human health risk assessment: findings from an independent panel of experts. Regul Toxicol Pharm. 134:105226.
- Anderson PO. 2019. Maternal vaccination and breastfeeding. Breastfeed Med. 14(4):215–217.
- Antoniou E, Colnot T, Zeegers M, Dekant W. 2022. Immunomodulation and exposure to per- and polyfluoroalkyl substances: an overview of the current evidence from animal and human studies. Arch Toxicol. 96(8):2261–2285.
- [ATSDR] Agency for Toxic Substances and Disease Registry. 2021. Toxicological profile for perfluoroalkyls. [updated 2020 March]. Atlanta (GA): U.S. Department of Health and Human Services. https://www.atsdr.cdc.gov/toxprofiles/tp200.pdf
- Beck IH, Timmermann CAG, Nielsen F, Schoeters G, Jøhnk C, Kyhl HB, Høst A, Jensen TK. 2019. Association between prenatal exposure to perfluoroalkyl substances and asthma in 5-year-old children in the Odense Child Cohort. Environ Health. 18(1):97.
- Budtz-Jørgensen E, Grandjean P. 2018. Application of benchmark analysis for mixed contaminant exposures: mutual adjustment of perfluoroalkylate substances associated with immunotoxicity. PLoS One. 13(10):e0205388.
- Butenhoff JL, Chang S-C, Olsen GW, Thomford PJ. 2012. Chronic dietary toxicity and carcinogenicity study with potassium perfluorooctanesulfonate in Sprague Dawley rats. Toxicology. 293(1-3):1–15.
- Butenhoff JL, Kennedy GL, Chang S-C, Olsen GW. 2012. Chronic dietary toxicity and carcinogenicity study with ammonium perfluorooctanoate in Sprague–Dawley rats. Toxicology. 298(1-3):1–13.
- [CDC] Center for Disease Control and Prevention. 2022. Per- and polyfluorinated substances (PFAS) factsheet | National Biomonitoring Program | CDC [Internet]. [accessed 2022 Jul 15]. https://www.cdc.gov/biomonitoring/PFAS_FactSheet.html.
- Chang ET, Adami H-O, Boffetta P, Wedner HJ, Mandel JS. 2016. A critical review of perfluorooctanoate and perfluorooctanesulfonate exposure and immunological health conditions in humans. Crit Rev Toxicol. 46(4):279–331.
- DeWitt JC, Germolec DR, Luebke RW, Johnson VJ. 2016. Associating changes in the immune system with clinical diseases for interpretation in risk assessment. Curr Protoc Toxicol. 67(1):1811–18122.
- DeWitt JC, Williams WC, Creech NJ, Luebke RW. 2016. Suppression of antigen-specific antibody responses in mice exposed to perfluorooctanoic acid: role of PPARα and T- and B-cell targeting. Journal of Immunotoxicology. 13(1):38–45.
- Dong G-H, Wang J, Zhang Y-H, Liu M-M, Wang D, Zheng L, Jin Y-H. 2012. Induction of p53-mediated apoptosis in splenocytes and thymocytes of C57BL/6 mice exposed to perfluorooctane sulfonate (PFOS). Toxicol Appl Pharmacol. 264(2):292–299.
- EFSA CONTAM Panel, Schrenk D, Bignami M, Bodin L, Chipman JK, del Mazo J, Grasl-Kraupp B, Hogstrand C, Hoogenboom L, Leblanc J-C, et al. 2020. Risk to human health related to the presence of perfluoroalkyl substances in food. EFSA J. 18(9):e06223.
- [EFSA] European Food Safety Authority. 2020. Outcome of a public consultation on the draft risk assessment of perfluoroalkyl substances in food. EFSA J. 17(9):1–202.
- [FDA] Food and Drug Administration. 2005. Biological products; bacterial vaccines and toxoids; implementation of efficacy review. Fed Regist. 70(242):75018–75028.
- Fedak KM, Bernal A, Capshaw ZA, Gross S. 2015. Applying the Bradford Hill criteria in the 21st century: how data integration has changed causal inference in molecular epidemiology. Emerg Themes Epidemiol. 12(1):14.
- Fei C, McLaughlin JK, Lipworth L, Olsen J. 2010. Prenatal exposure to PFOA and PFOS and risk of hospitalization for infectious diseases in early childhood. Environ Res. 110(8):773–777.
- [FSANZ] Food Standards Australia New Zealand. 2021. PFAS and immunomodulation: review and update in support of the 27th Australian total diet study [Internet]; [accessed 2022 Mar 24]. https://www.foodstandards.gov.au/publications/Pages/27th-Australian-Total-Diet-Study.aspx.
- Grandjean P, Andersen EW, Budtz-Jørgensen E, Nielsen F, Mølbak K, Weihe P, Heilmann C. 2012. Serum vaccine antibody concentrations in children exposed to perfluorinated compounds. J Am Med Assoc. 307(4):391–397.
- Grandjean P, Budtz-Jørgensen E. 2013. Immunotoxicity of perfluorinated alkylates: calculation of benchmark doses based on serum concentrations in children. Environ Health. 12(1):35.
- Grandjean P, Heilmann C, Weihe P, Nielsen F, Mogensen Ulla B, Budtz-Jørgensen E. 2017. Serum vaccine antibody concentrations in adolescents exposed to perfluorinated compounds. Environ Health Perspect. 125(7):077018.
- Grandjean P, Heilmann C, Weihe P, Nielsen F, Mogensen Ulla B, Timmermann A, Budtz-Jørgensen E. 2017. Estimated exposures to perfluorinated compounds in infancy predict attenuated vaccine antibody concentrations at age 5-years. J Immunotoxicol. 14(1):188–195.
- Grandjean P, Timmermann CAG, Kruse M, Nielsen F, Vinholt PJ, Boding L, Heilmann C, Mølbak K. 2020. Dec 31 Severity of COVID-19 at elevated exposure to perfluorinated alkylates. PLOS One. 15(12):e0244815.
- Granum B, Haug LS, Namork E, Stølevik SB, Thomsen C, Aaberge IS, van Loveren H, Løvik M, Nygaard UC. 2013. Pre-natal exposure to perfluoroalkyl substances may be associated with altered vaccine antibody levels and immune-related health outcomes in early childhood. J Immunotoxicol. 10(4):373–379.
- Hahn-Zoric M, Fulconis F, Minoli I, Moro G, Carlsson B, Böttiger M, Rähä N, Hanson LÅ. 2008. Antibody responses to parenteral and oral vaccines are impaired by conventional and low protein formulas as compared to breast-feeding. Acta Paediatr. 79(12):1137–1142.
- Hanson LA. 1998. Breastfeeding provides passive and likely long-lasting active immunity. Ann Allergy. Asthma Immunol. 81(6):523–537.
- Hanson LA, Wiedermann U, Ashraf R, Zaman S, Adlerberth I, Dahlgren U, Wold A, Jalil F. 1996. Effects of breastfeeding on the baby and on its immune system. Food Nutr Bull. 17(4):1–5.
- Han R, Zhang F, Wan C, Liu L, Zhong Q, Ding W. 2018. Effect of perfluorooctane sulphonate-induced Kupffer cell activation on hepatocyte proliferation through the NF-κB/TNF-α/IL-6-dependent pathway. Chemosphere. 200:283–294.
- Health Canada. 2018. Guidelines for Canadian drinking water quality: guideline technical document: perfluorooctanoic acid (PFOA) [Internet]. [place unknown]: Water and Air Quality Bureau; [accessed 2020 Apr 15]. https://epe.lac-bac.gc.ca/100/201/301/weekly_acquisitions_list-ef/2018/18-51/publications.gc.ca/collections/collection_2018/sc-hc/H144-13-8-2018-eng.pdf.
- Heilmann C, Budtz-Jørgensen E, Nielsen F, Heinzow B, Weihe P, Grandjean P. 2010. Serum concentrations of antibodies against vaccine toxoids in children exposed perinatally to immunotoxicants. Environ Health Perspect. 118(10):1434–1438.
- Jackson-Browne MS, Eliot M, Patti M, Spanier AJ, Braun JM. 2020. PFAS (per- and polyfluoroalkyl substances) and asthma in young children: NHANES 2013–2014. Int J Hyg Environ Health. 229:113565.
- Jackson K, Nazar A. 2006. Breastfeeding, the immune response, and long-term health. J Am Osteopath Assoc. 106(4):203–207.
- Karagas MR, Choi AL, Oken E, Horvat M, Schoeny R, Kamai E, Cowell W, Grandjean P, Korrick S. 2012. Evidence on the human health effects of low-level methylmercury exposure. Environ Health Perspect. 120(6):799–806.
- Keil DE, Mehlmann T, Butterworth L, Peden-Adams MM. 2008. Gestational exposure to perfluorooctane sulfonate suppresses immune function in B6C3F1 mice. Toxicol Sci. 103(1):77–85.
- Kielsen K, Shamim Z, Ryder LP, Nielsen F, Grandjean P, Budtz-Jørgensen E, Heilmann C. 2016. Antibody response to booster vaccination with tetanus and diphtheria in adults exposed to perfluorinated alkylates. J Immunotoxicol. 13(2):270–273.
- Kirman CR, Simon TW, Hays SM. 2019. Science peer review for the 21st century: assessing scientific consensus for decision-making while managing conflict of interests, reviewer and process bias. Regul Toxicol Pharm. 103:73–85.
- Lee JK, Lee S, Baek MC, Lee BH, Lee HS, Kwon TK, Park PH, Shin TY, Khang D, Kim SH. 2017. Association between perfluorooctanoic acid exposure and degranulation of mast cells in allergic inflammation. J Appl Toxicol. 37(5):554–562.
- Lebrec H, Molinier B, Boverhof D, Collinge M, Freebern W, Henson K, Mytych DT, Ochs HD, Wange R, Yang Y, et al. 2014. The T-cell-dependent antibody response assay in nonclinical studies of pharmaceuticals and chemicals: study design, data analysis, interpretation. Regul Toxicol Pharm. 69(1):7–21.
- Looker C, Luster MI, Calafat AM, Johnson VJ, Burleson GR, Burleson FG, Fletcher T. 2014. Influenza vaccine response in adults exposed to perfluorooctanoate and perfluorooctanesulfonate. Toxicol Sci. 138(1):76–88.
- Loveless SE, Hoban D, Sykes G, Frame SR, Everds NE. 2008. Evaluation of the immune system in rats and mice administered linear ammonium perfluorooctanoate. Toxicol Sci. 105(1):86–96.
- Luster MI, Portier C, Pait DG, Rosenthal GJ, Germolec DR, Corsini E, Blaylock BL, Pollock P, Kouchi Y, Craig W, et al. 1993. Risk assessment in immunotoxicology: II. Relationships between immune and host resistance tests. Toxicol Sci. 21(1):71–82.
- Luster MI, Portier C, Pait DG, White KL Jr, Gennings C, Munson AE, Rosenthal GJ. 1992. Risk Assessment in immunotoxicology: I. Sensitivity and predictability of immune tests. Toxicol Sci. 18(2):200–210.
- Manzano-Salgado CB, Granum B, Lopez-Espinosa MJ, Ballester F, Iñiguez C, Gascón M, Martínez D, Guxens M, Basterretxea M, Zabaleta C, et al. 2019. Prenatal exposure to perfluoroalkyl substances, immune-related outcomes, and lung function in children from a Spanish birth cohort study. Int J Hyg Environ Health. 222(6):945–954.
- Meek MEB, Palermo CM, Bachman AN, North CM, Jeffrey Lewis R. 2014. Mode of action human relevance (species concordance) framework: evolution of the Bradford Hill considerations and comparative analysis of weight of evidence. J Appl Toxicol. 34(6):595–606.
- Neubert RT, Webb JR, Neubert D. 2002. Feasibility of human trials to assess developmental immunotoxicity, and some comparison with data on New World monkeys. Hum Exp Toxicol. 21(9-10):543–567.
- [NTP] National Toxicology Program. 2016. Systematic review of immunotoxicity associated with exposure to perfluorooctanoic acid (PFOA) or perfluorooctane sulfonate (PFOS). [place unknown]: National Institute of Environmental Health Sciences, National Institutes of Health, U.S. Department of Health and Human Services.
- [NTP] National Toxicology Program. 2019. NTP technical report on the toxicity studies of perfluoroalkyl carboxylates (perfluorohexanoic acid, perfluorooctanoic acid, perfluorononanoic acid, and perfluorodecanoic acid) administered by gavage to Sprague Dawley (Hsd: Sprague Dawley SD) rats [Internet]. [place unknown]: National Institute of Environmental Health Sciences, National Institutes of Health, U.S. Department of Health and Human Services.
- [NTP] National Toxicology Program. 2020. NTP technical report on the toxicology and carcinogenesis studies of perfluorooctanoic acid (CASRN 335-67-1) administered in feed to Sprague Dawley (Hsd: Sprague Dawley® SD®) rats [Internet]. [place unknown]: National Institute of Environmental Health Sciences, National Institutes of Health, U.S. Department of Health and Human Services.
- [OEHHA] Office of Environmental Health Hazard Assessment. 2021. Public health goals. First public reviewed draft. Perfluorooctanoic acid and perfluorooctane sulfonic acid in drinking water [Internet]. [place unknown]: California Environmental Protection Agency. https://oehha.ca.gov/media/downloads/crnr/pfoapfosphgdraft061021.pdf.
- Post GB. 2021. Recent US state and federal drinking water guidelines for per‐ and polyfluoroalkyl substances. Environ Toxicol Chem. 40(3):550–563.
- Pilkerton CS, Hobbs GR, Lilly C, Knox SS. 2018. Rubella immunity and serum perfluoroalkyl substances: sex and analytic strategy. PLOS One. 13(9):e0203330.
- Qazi MR, Nelson BD, DePierre JW, Abedi-Valugerdi M. 2010. 28-Day dietary exposure of mice to a low total dose (7mg/kg) of perfluorooctanesulfonate (PFOS) alters neither the cellular compositions of the thymus and spleen nor humoral immune responses: does the route of administration play a pivotal role in PFOS-induced immunotoxicity? Toxicology. 267(1–3):132–139.
- Smit LA, Lenters V, Høyer BB, Lindh CH, Pedersen HS, Liermontova I, Jönsson BA, Piersma AH, Bonde JP, Toft G, et al. 2015. Prenatal exposure to environmental chemical contaminants and asthma and eczema in school-age children. Allergy. 70(6):653–660.
- Stein CR, McGovern KJ, Pajak AM, Maglione PJ, Wolff MS. 2016. Perfluoroalkyl and polyfluoroalkyl substances and indicators of immune function in children aged 12-19 y: National Health and Nutrition Examination Survey. Pediatr Res. 79(2):348–357.
- Suo C, Fan Z, Zhou L, Qiu J. 2017. Jul 12 Perfluorooctane sulfonate affects intestinal immunity against bacterial infection. Sci Rep. 7(1):5166.
- Timmermann CA, Budtz-Jørgensen E, Jensen TK, Osuna CE, Petersen MS, Steuerwald U, Nielsen F, Poulsen LK, Weihe P, Grandjean P. 2017. Association between perfluoroalkyl substance exposure and asthma and allergic disease in children as modified by MMR vaccination. J Immunotoxicol. 14(1):39–49.
- Timmermann CAG, Jensen KJ, Nielsen F, Budtz-Jørgensen E, van der Klis F, Benn CS, Grandjean P, Fisker AB. 2020. Serum perfluoroalkyl substances, vaccine responses, and morbidity in a cohort of Guinea-Bissau children. Environ Health Perspect. 128(8):87002.
- Timmermann CAG, Pedersen HS, Weihe P, Bjerregaard P, Nielsen F, Heilmann C, Grandjean P. 2022. Concentrations of tetanus and diphtheria antibodies in vaccinated Greenlandic children aged 7–12 years exposed to marine pollutants, a cross sectional study. Environ Res. 203:111712.
- Torres L, Redko A, Limper C, Imbiakha B, Chang S, August A. 2021. Effect of perfluorooctanesulfonic acid (PFOS) on immune cell development and function in mice. Immunol Lett. 233:31–41.
- [USEPA] U.S. Environmental Protection Agency. 1998. Health effects test guidelines OPPTS 870.7800 immunotoxicity. [place unknown]: Prevention, Pesticides and Toxic Substances, EPA 712–C–98–351.
- [USEPA] U.S. Environmental Protection Agency. 2005. Guidelines for carcinogen risk assessment. Washington (DC): Risk Assessment Forum. http://www.epa.gov/cancerguidelines/
- [USEPA] U.S. Environmental Protection Agency. 2021a. External peer review draft: proposed approaches to the derivation of a draft maximum contaminant level goal for perfluorooctane sulfonic acid (PFOS) (CASRN 1763-23-1) in drinking water. Washington (DC): Office of Water, Health and Ecological Criteria Division.
- [USEPA] U.S. Environmental Protection Agency. 2021b. External peer review draft: proposed approaches to the derivation of a draft maximum contaminant level goal for perfluorooctanoic acid (PFOA) (CASRN 335-67-1) in drinking water. Washington (DC): Office of Water, Health and Ecological Criteria Division.
- [USEPA] U.S. Environmental Protection Agency. 2022a. Interim drinking water health advisory: perfluorooctane sulfonic acid (PFOS) CASRN 1763-23-1. Washington (DC): Office of Water (4304T), Office of Science and Technology Health and Ecological Criteria Division.
- [USEPA] U.S. Environmental Protection Agency. 2022b. Interim drinking water health advisory: perfluorooctanoic Acid (PFOA) CASRN 335-67-1. Washington (DC): Office of Water (4304T), Office of Science and Technology Health and Ecological Criteria Division.
- Weihe P, Kato K, Calafat AM, Nielsen F, Wanigatunga AA, Needham LL, Grandjean P. 2008. Serum concentrations of polyfluoroalkyl compounds in Faroese whale meat consumers. Environ Sci Technol. 42(16):6291–6295.
- [WHO] World Health Organization. 2012. Guidance for immunotoxicity risk assessment for chemicals [Internet]. Geneva: World Health Organization; [accessed 2022 Mar 14]. https://apps.who.int/iris/handle/10665/330098.
- [WHO] World Health Organization. 2018. Immunological basis for immunization series. Module 3: tetanus update; [updated 2018]. [place unknown]: World Health Organization.
- [WHO and IPCS] World Health Organization and the International Programme on Chemical Safety. 2012. Guidance for immunotoxicity risk assessment for chemicals [Internet]. Geneva: World Health Organization and the International Programme on Chemical Safety; [accessed 2022 Mar 14]. https://apps.who.int/iris/handle/10665/330098.
- Xing J, Wang G, Zhao J, Wang E, Yin B, Fang D, Zhao J, Zhang H, Chen YQ, Chen W. 2016. Toxicity assessment of perfluorooctane sulfonate using acute and subchronic male C57BL/6J mouse models. Environ Pollut. 210:388–396.
- Zeng XW, Bloom MS, Dharmage SC, Lodge CJ, Chen D, Li S, Guo Y, Roponen M, Jalava P, Hirvonen MR, et al. 2019. Prenatal exposure to perfluoroalkyl substances is associated with lower hand, foot and mouth disease viruses antibody response in infancy: findings from the Guangzhou Birth Cohort Study. Sci Total Environ. 663:60–67.
- Zeng XW, Li QQ, Chu C, Ye WL, Yu S, Ma H, Zeng XY, Zhou Y, Yu HY, Hu LW, et al. 2020. Apr Alternatives of perfluoroalkyl acids and hepatitis B virus surface antibody in adults: isomers of C8 health project in China. Environ Pollut. 259:113857.
- Zhang X, Xue L, Deji Z, Wang X, Liu P, Lu J, Zhou R, Huang Z. 2022. Effects of exposure to per- and polyfluoroalkyl substances on vaccine antibodies: a systematic review and meta-analysis based on epidemiological studies. Environ Pollut. 306(119442):119442.
- Zhong S-Q, Chen Z-X, Kong M-L, Xie Y-Q, Zhou Y, Qin X-D, Paul G, Zeng X-W, Dong G-H. 2016. Testosterone-mediated endocrine function and TH1/TH2 cytokine balance after prenatal exposure to perfluorooctane sulfonate: by sex status. Int J Mol Sci. 17(9):1509.