Abstract
The mechanisms of particle-induced pathogenesis in the lung remain poorly understood. Neutrophilic inflammation and oxidative stress in the lung are hallmarks of toxicity. Some investigators have postulated that oxidative stress from particle surface reactive oxygen species (psROS) on the dust produces the toxicopathology in the lungs of dust-exposed animals. This postulate was tested concurrently with the studies to elucidate the toxicity of lunar dust (LD), which is believed to contain psROS due to high-speed micrometeoroid bombardment that fractured and pulverized lunar surface regolith. Results from studies of rats intratracheally instilled (ITI) with three LDs (prepared from an Apollo-14 lunar regolith), which differed 14-fold in levels of psROS, and two toxicity reference dusts (TiO2 and quartz) indicated that psROS had no significant contribution to the dusts’ toxicity in the lung. Reported here are results of further investigations by the LD toxicity study team on the toxicological role of oxidants in alveolar neutrophils that were harvested from rats in the 5-dust ITI study and from rats that were exposed to airborne LD for 4 weeks. The oxidants per neutrophils and all neutrophils increased with dose, exposure time and dust’s cytotoxicity. The results suggest that alveolar neutrophils play a critical role in particle-induced injury and toxicity in the lung of dust-exposed animals. Based on these results, we propose an adverse outcome pathway (AOP) for particle-associated lung disease that centers on the crucial role of alveolar neutrophil-derived oxidant species. A critical review of the toxicology literature on particle exposure and lung disease further supports a neutrophil-centric mechanism in the pathogenesis of lung disease and may explain previously reported animal species differences in responses to poorly soluble particles. Key findings from the toxicology literature indicate that (1) after exposures to the same dust at the same amount, rats have more alveolar neutrophils than hamsters; hamsters clear more particles from their lungs, consequently contributing to fewer neutrophils and less severe lung lesions; (2) rats exposed to nano-sized TiO2 have more neutrophils and more severe lesions in their lungs than rats exposed to the same mass-concentration of micron-sized TiO2; nano-sized dust has a greater number of particles and a larger total particle–cell contact surface area than the same mass of micron-sized dust, which triggers more alveolar epithelial cells (AECs) to synthesize and release more cytokines that recruit a greater number of neutrophils leading to more severe lesions. Thus, we postulate that, during chronic dust exposure, particle-inflicted AECs persistently release cytokines, which recruit neutrophils and activate them to produce oxidants resulting in a prolonged continuous source of endogenous oxidative stress that leads to lung toxicity. This neutrophil-driven lung pathogenesis explains why dust exposure induces more severe lesions in rats than hamsters; why, on a mass-dose basis, nano-sized dusts are more toxic than the micron-sized dusts; why lung lesions progress with time; and why dose–response curves of particle toxicity exhibit a hockey stick like shape with a threshold. The neutrophil centric AOP for particle-induced lung disease has implications for risk assessment of human exposures to dust particles and environmental particulate matter.
1. Introduction
1.1. Prolonged exposures to environmental particulate matter (PM) can lead to pulmonary diseases including cancer
1.1.1. Prolonged exposure to urban PM can cause pulmonary diseases
Urban air pollutants contain PM, which is the main contributor to pollution-induced pulmonary diseases including lung cancer (Dockery et al. Citation1993; Pope et al. Citation2002; Krewski et al. Citation2003; Laden Citation2019). PM and diesel particulate matter (DPM) are classified as human carcinogens (IARC Citation1989, Citation2013; NTP Citation2000). The composition of PM is complicated and mechanisms of PM-induced pathogenesis in the lung remain poorly understood. According to California Air Resources Board (CARB Citation2022), “… about 70% of total known cancer risk related to air toxics in California is attributable to DPM and… DPM comprises about 8% of PM2.5 in outdoor air.” DPM or diesel exhaust (DE) particles have a carbon core (carbon black (CB) or black carbon (BC)) that contains adsorbed organic and inorganic compounds. The World Health Organization (WHO Citation2012) noted that, “effect estimates (from both short- and long-term studies) are much higher for BC than PM10 and PM2.5 [measured in µg/m3].” Although DPM constitutes <10% of urban PM2.5, the CARB and WHO findings reveal it could be a major contributing factor to PM2.5-associated pulmonary diseases. Increasing evidence from the epidemiological literature indicates that mortality and morbidity of respiratory diseases are strongly related to exposures to components of DE, such as carbon and CB (Selley et al. Citation2019). The chemical components or factors of PM that lead to adverse outcome pathway (AOP) are unclear. Neutrophilic infiltrate appears to be the most common and first noticeable toxic response to PM exposure (Valderrama et al. Citation2022). Human volunteers exposed to DE particulate or concentrated ambient PM in chamber studies had higher levels of neutrophils in their bronchoalveolar lavage fluids (BALFs) or sputum samples than air-exposed controls (Ghio et al. Citation2000; Nightingale et al. Citation2000; Nordenhäll et al. Citation2000; Salvi et al. Citation2000).
DPM contains adsorbed polycyclic aromatic hydrocarbons, some of which are mutagenic and carcinogenic. Mauderly et al. (Citation1994) exposed rats (1370 total) to 2.5 or 6.5 mg/m3 of diesel soot or CB (organic-free soot surrogate) for 24 months (16 h/d; 5 d/week) and observed nearly identical incidences of cancer and noncancerous effects in all exposure groups. The authors concluded that organic compounds associated with DE soot played no significant role in the observed tumorigenic effect in rats. It is noteworthy that the extent of neutrophil inflammation and incidence of lung lesions, including progressive fibrosis, increased in a dose-dependent manner in this study.
1.1.2. Non-urban natural dusts can produce pulmonary diseases
Exposures to high concentrations of fine natural desert sand are also associated with increased risk of pulmonary diseases (Domínguez-Rodríguez et al. Citation2021). Giannadaki et al. (Citation2014) conducted large epidemiological cohort studies based on WHO mortality rates of adult populations (age >30), who were exposed to airborne respirable desert dust. The authors reported that “Our model results indicate a large number of premature deaths by cardiopulmonary disease and a significant number of deaths by lung cancer, mostly in the dust belt region… The countries with the highest dust-related mortality are Egypt, Pakistan, Nigeria, China, Sudan, and other countries in and around the dust belt.” Yanagisawa et al. (Citation2007) intratracheally instilled (ITI) heat-treated desert sand (from China) to mice and observed “prominently enhanced pulmonary neutrophilic inflammation.”
Amorphous silica, the major component of desert sand, is relatively low in toxicity. When Kolling et al. (Citation2011) instilled 53 rats with nano-size (ns) amorphous silica (SiO2,15 mg/rat), five of the rats developed tumors, whereas none of the 55 rats instilled with saline developed tumors. The tumor incidence in rats instilled with coal dust (10 mg/rat), ns-CB (5 mg/rat), and crystalline silica (3 mg/rat) were 0/51, 9/59, and 12/56, respectively. An increase in the number of lung inflammatory cells and fibrosis measured in this study paralleled the tumor incidence. Johnston et al. (Citation2000) reported neutrophil counts of 17 × 107 and 14 × 107 harvested from the lung of rats 13 weeks after exposure to 50 mg/m3 of ns-SiO2 or 3 mg/m3 of crystalline silica, respectively, whereas air-exposed rats had 0.26 × 107 neutrophils in their BALF samples. These results revealed exposure to a very high concentration of amorphous silica caused severe pulmonary neutrophilia. We use the term neutrophilia to indicate an increased neutrophilic infiltrate in pulmonary tissues. These studies show exposure to high concentrations of organic-free mineral particles can produce pulmonary diseases in rats and in humans. However, humans would not typically be chronically exposed to mineral dusts or other PM at the high concentrations used in animal studies.
1.2. Poorly water-soluble particles (PSPs) can produce pulmonary diseases including cancer in chronically and heavily exposed rats
CB, DE, TiO2, and silica are considered to be PSPs. Compared to urban PM, CB, and TiO2 are chemically and structurally simple dusts, these relatively low toxicity dusts can cause persistent alveolar neutrophilia and lung disease including cancer in chronically and heavily exposed rats (Mauderly et al. Citation1994; Heinrich et al. Citation1995). Driscoll (Citation1996) noted that significant active and chronic inflammation, epithelial hyperplasia and metaplasia, and pulmonary fibrosis precede tumor formation in the lungs of rats chronically exposed to high concentrations of PSPs. Morrow et al. (Citation1996) similarly pointed out that in studies of PSP exposure, tumors were observed only at exposure levels that resulted in pronounced inflammatory and fibrotic responses in the lung.
1.3. A neutrophil-centric toxicity mechanism induced by simple and poorly soluble mineral dusts in the lung could shed some light on the complicated pathogenesis of PM
PM is a class of chemically and toxicologically diverse dusts. In a review of 40 years (1980–2020) of literature on the toxicological role of neutrophils in diseases induced by air pollutants, Valderrama et al. (Citation2022) concluded “environmental pollutants induced neutrophil recruitment as the first sign of inflammation. The effect of neutrophils depended on the type, concentration, and size of the PM particles. These cells release pro-inflammatory cytokines and ROS that cause damage to lung tissue and contributes to the systemic inflammatory response related to cardiovascular diseases. The importance of future research to clearly establish the relationship between neutrophilic activation after exposure to air pollutants with the development of cancer is clear.” It has been shown that neutrophil-generated ROS produce significant DNA damage in hyperoxia-exposed newborn rat lungs (Auten et al. Citation2002). Driscoll, Deyo, et al. (Citation1997) reported that neutrophilic oxidants induced mutations in the lungs of rats exposed to crystalline silica, CB, or TiO2; mutations can lead to carcinogenesis (Driscoll Citation1996). The results of these studies suggest that neutrophil-generated ROS are genotoxic. A large portion of PM is non-genotoxic, establishing how these simple and non-genotoxic mineral dusts induce lung pathogenesis could help elucidate how the non-genotoxic component of the PM contributes to the PM-induced pulmonary diseases including lung cancer. Regardless of the type of PM, if the exposure is substantial, pulmonary neutrophilic inflammation is a toxicological hallmark of PM exposure. Toxicities of PSPs have been well studied; persistent alveolar neutrophilia and fibrosis precede tumor formation in the lungs of rats chronically exposed to high concentrations of PSPs (Driscoll Citation1996; Morrow et al. Citation1996). The crucial role of neutrophils in particle-induced pathogenesis in the lung is the subject of our review and is supported by experimental data assessing the toxicological role of oxidants generated by alveolar neutrophils from rats exposed to LDs and other PSPs presented in our report.
1.4. Review and comments on current mechanisms for particle-induced pathogenesis of lung disease
It is well known that oxidative stress or reactive oxidative species (ROS) is an important contributing factor in development of dust-induced diseases in the lung (Mossman et al. Citation2007). One school of thought is that ROS comes from the particles themselves, whereas others believe that ROS is derived chiefly from alveolar macrophages (AMs) or other inflammatory cells in the lung after exposure to PM. The general AOP for particle-induced pulmonary diseases, based on these two premises, were summarized in the 1990s by Lapp and Castranova (Citation1993). These mechanisms have been accepted in the particle toxicology field even today.
1.4.1. Surface ROS on particles
One proposed mechanism of particle-induced pulmonary toxicity is that the direct cytotoxicity of a dust produces injury to lung cells (Lapp and Castranova Citation1993; Castranova Citation1998). If cells are severely damaged and exposure continues, this can lead to scarring (fibrosis) or uncontrolled hyperplasia and metaplasia that can progress to cancer. Cytotoxicity from particle exposure can arise in several ways. Particles may generate particle surface reactive oxygen species (psROS) under certain conditions (such as being fractured during grinding or sandblasting; Fubini et al. Citation1989). Donaldson et al. (Citation1996) postulated that the ability of particles to generate free radicals at or near their surface, and thereby impose oxidative stress in key target cells, could be central to their pathogenicity. Fubini et al. (Citation1995) proposed that surface radicals and iron-derived ROS are implicated in oxidative stress, which is considered to be the key event in the development of fibrosis and lung cancer.
1.4.2. Oxidants and other harmful cellular contents released by macrophages
An alternative mechanism of particle-induced pathogenesis involves ROS and other deleterious products that are produced by particle-stimulated AMs (Lapp and Castranova Citation1993; Castranova Citation1998). It has been hypothesized that particle exposures induce AMs to produce ROS, pro-inflammatory cytokines including chemokines, fibrogenic factors, and proliferative factors, which can contribute to inflammation, fibrosis, and cancer. Many have similarly proposed a mechanism centered on the role of AMs (Vallyathan and Shi Citation1997; Hamilton et al. Citation2008; Laskin et al. Citation2011). For example, Brain (Citation1980) postulated that the phagocytized particles could damage the AMs triggering these cells to release lysosomal enzymes, toxic proteolytic enzymes, and oxidant radicals, which harm cells and eventually cause lung diseases including fibrosis. Fubini et al. (Citation1989) and Fubini and Hubbard (Citation2003) proposed that free radicals on silica reacted with O2 and H2O, subsequently triggering macrophage-mediated reactions that lead to fibrosis. In a review, Laskin et al. (Citation2019) postulated that host defense agents (including oxidants, mediators, and proteases) that are typically released by AMs against invading pathogens can exacerbate acute lung injury and/or induce chronic disease, such as fibrosis, chronic obstructive pulmonary diseases, and asthma, when they are released in excess.
1.5. Speculation that psROS on lunar dust contributes to the dust’s toxicity
A recent article (Pohlen et al. Citation2022) titled Overview of Lunar Dust Toxicity Risk by a group of researchers outside NASA stated that, “…exposure to galactic and solar radiation likely increase the surface reactivity of the [LD] particles by creating large numbers of dangling chemical bonds and unsatisfied electron valences …the particles may contribute to the formation of reactive oxygen species…when in contact with human tissue, though the likelihood and severity of this possibility are unknown… the extended presence of the particle, even after macrophage phagocytosis with subsequent apoptosis or lymph node migration, can prompt the release of oxidants, cytokines, and growth factors and sets off an inflammatory cascade with the recruitment of additional immune cells (to include neutrophils and new alveolar macrophages). The uncleared particles can be absorbed repeatedly by successive generations of macrophages, leading to chronic inflammation.”
It is believed that lunar dust (LD) is reactive because the surface of the Moon has been constantly bombarded by high-speed micrometeoroids that fractured and pulverized surface regolith into fine dust containing an abundant amount of psROS; solar and cosmic particles could impart free radical on LD. Because the toxicological role of psROS or hydroxyl free radicals (●OH) of mineral dusts had not yet been investigated in animal studies, the NASA lunar geology team meticulously prepared (under ultra-pure nitrogen) three respirable sized LDs that differed 14-fold in particle-generated ●OH from the same parent Apollo-14 lunar regolith for toxicity investigations (McKay et al. Citation2015). The toxicity of these three LDs, along with two terrestrial toxicity reference dust (TiO2 and quartz), was assessed at three different dose levels and two different time points in rats instilled with these five dusts (Lam et al. Citation2022). Toxicological biomarkers measured in BALF samples, and the pulmonary histopathology of rats exposed to these five types of particles did not correlate with the particles’ levels of particle-generated psROS. Quartz (aged) had the lowest level of psROS but was the most toxic. The toxic potency of these dusts was quartz > LD > TiO2. Ball-milled LD had 14-fold higher levels of psROS than unground LD (); however, these two LDs were equally toxic (Lam et al. Citation2022).
Figure 1. Hydroxyl free radicals •OH in lunar dusts and reference dusts. Specific chemical reactivity was assessed by measuring the amount of •OH/10 mg of dust generated in the presence of terephthalate. The content of •OH in the dust was estimated by the amount of terephthalate consumed. Data are the mean ± SD of •OH production (average from three runs) in pmol/10 mg test dust. Normalization: LD-ug, 100%; LD-jm, 193%; LD-jm, 1367%; TiO2 121%; and quartz 76%. Graph plotted from data from Lam et al. (Citation2022).
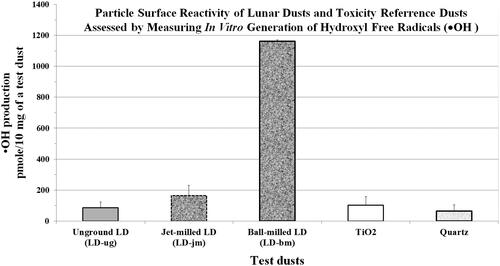
Harrington, Schmidt, et al. (Citation2017) also endorsed the hypothesis that the psROS is a crucial determinant of the particle-induced lung diseases. After joining NASA, Harrington conducted a mouse ITI study to test this long-accepted hypothesis using seven ground dusts (six derived from meteorites and one from a terrestrial basalt) containing various levels of psROS (Harrington, McCubbin, et al. Citation2017). This study was conducted in collaboration with the toxicology laboratory at New York University to support NASA’s manned deep-space missions by assessing the correlation between the dusts’ psROS and pulmonary toxicity, and the correlation between iron levels of the dusts and pulmonary toxicity. The investigators found no correlation between psROS of the dusts and the dusts’ toxicity in the lungs; iron level also played an insignificant role in toxicity. Caston et al. (Citation2018) investigated ground LD simulant with increased ●OH in cell culture studies and found that ●OH had no toxicological impact on the cells.
1.6. Currently accepted mechanisms do not explain many of the toxicological findings in the lungs of dust-exposed animals
The highly toxic ●OH species and other free radicals are known to play a critical pathological role in lesions induced by ionizing radiation. As noted above, some particle researchers postulated that the free radicals generated by particles could play an important role in the toxicity in the lung (Fubini et al. Citation1989, Citation1990, Citation1995; Donaldson et al. Citation1996; Fubini Citation1998). Fubini et al. (Citation1987) proposed that free radicals on the surface of silica can react with H2O and O2, which subsequently trigger macrophage-mediated reactions leading to fibrosis. It has long been known that ionizing radiation can induce free radicals from H2O and O2 that pose oxidative stress to the biological system (Smith Citation1962; Riley Citation1994). The speculation that particle-derived free radicals are key to pathogenesis in the lung of dust-exposed animals (Fubini et al. Citation1989, Citation1995; Donaldson et al. Citation1996) can be inferred from radiation toxicity studies. So why do the levels of psROS of dust have no impact on the dust toxicity in the lung as shown in studies like those of Lam et al. (Citation2022) and Harrington, McCubbin, et al. (Citation2017)? It is noteworthy that prolonged radiation exposure will ionize H2O in its path continuously producing free radicals extracellularly and intracellularly that could damage cellular structures. The short-lived and limited amounts of psROS on particles lose their activity rapidly in lung fluid that contains antioxidants (Vallyathan et al. Citation1988). Furthermore, unlike ●OH and other free radicals produced intranuclearly by ionizing radiation, psROS from the particles cannot enter the nucleus and damage DNA.
Vallyathan et al. (Citation1988) of the National Institute for Occupational Safety and Health (NIOSH) reported that the half-life of ROS generated by fresh-milled quartz (Qz-jm) was ∼30 h in the air and only a few minutes when the dust was placed in phosphate-buffered saline. It is likely that the half-life of psROS is even shorter in the presence of surfactant, antioxidants, and antioxidative enzymes in the lung. Rats given a bolus ITI dose (12 mg/rat) of quartz dust (not freshly ground) had time-dependent increases in incidences of lung tumors (17% (11 months), 32% (17 months), and 86% (26 months)), whereas rats instilled with iron oxide in the same study had no tumors (Saffiotti Citation1992). The short half-life of psROS could not explain this long-term particle-induced toxicity in the lung. Furthermore, iron oxide is more likely than quartz to generate free radicals, whereas the latter is known to elicit more neutrophilic inflammation than the former. The chronic presence of particle-elicited neutrophils produced oxidants that induced mutations in alveolar epithelial cells (AECs) of the rats assessed 15 months after bolus instillation with quartz or other dusts (Driscoll, Deyo, et al. Citation1997). Auten et al. (Citation2002) reported oxidants from neutrophils injured the AECs and damaged their DNA in hyperoxia-exposed neonatal rats. These authors hypothesized that neutrophilic oxidants could be the source of oxidative stress leading to toxicity in the lungs.
Oberdörster et al. (Citation1992) showed that neutrophilic inflammation and lung lesions occurred in rats after they were instilled with ultrafine TiO2 particles. However, when rats were instilled with the same amount of this TiO2 that was contained (phagocytized) in host AMs, no inflammation or toxicity occurred. Oberdörster concluded that internalization of particles by AMs prevented lung neutrophilic inflammation and toxicity. Saffiotti (Citation1992) of the National Cancer Institute instilled 37 rats with a single dose of 12 mg quartz (Min-U-Sil 5); 11 rats had lung tumors when examined 11 months after the bolus quartz instillation. When Syrian golden hamsters were examined 12 months after they were each instilled with 20 mg quartz, the quartz particles were extensively phagocyted by AMs resulting in little toxicity, and the adjacent epithelium generally remained unaffected with no significant indication of persistent epithelial hyperplasia. No fibrosis and no cancer were observed in these hamsters. Others have also shown that chronic exposures to dusts resulted in severe alveolar neutrophilia and severe lung lesions including cancer in rats but not in hamsters. In summary, the proposed mechanism of pathogenesis of lung disease based on psROS of the dust, or unique crystalline silica chemistry such as silanols or negative surface charge (Lapp and Castranova Citation1993; Castranova Citation1998) fails to fully explain the differences in toxicological responses for hamsters and rats exposed to quartz and other PSPs. A mechanism based on ROS and cytokines generated by AMs (Castranova et al. Citation1988) also do not provide a satisfactory explanation of the drastically different response in rats vs. hamsters.
1.7. Where do we stand after 30 years of research in particle toxicology?
The mechanisms underlying differences in toxicological responses and tumorigenicity in the lungs of rats and hamsters exposed to PSPs and the implications for human hazard identification and risk assessment have been debated by toxicologists for more than 30 years (Mauderly et al. Citation1987, Citation1994; Muhle et al. Citation1989; Hext Citation1994; Heinrich et al. Citation1995; Bermudez et al. Citation2002, Citation2004). A critical regulatory question is which animal species (rats, mice, or hamsters) is the most relevant to assess human risk from inhaled particles and for toxicity and bioassay studies. To assess the current state of scientific understanding, Drs. Paul Borm and Kevin Driscoll interviewed 27 toxicology experts and experts on risk assessment and published their findings in a commentary paper (Borm and Driscoll Citation2019). Based on the gathered opinions, the authors concluded that, “… in many cases… we do not fully understand the rat lung cancer mechanism after exposure to poorly soluble particles…. Is the rat lung response to high doses of particles like titanium dioxide and carbon black unique to this species, or is the rat a human-relevant, sensitive species?” The authors advocated that, “we believe it is important to re-activate the public debate … safety assessment [of inhaled particles].” In a follow-up public expert workshop, experts concluded, based on the current state of the science, that using lung tumors in rats exposed to very high concentrations of PSPs should be seriously questioned for assessing human risk (Driscoll Citation2022).
1.8. An association between neutrophils and particle-induced lung diseases has not been fully investigated
The association between alveolar neutrophilia and dust-induced lung diseases, including cancer, has been recognized for a long time (Driscoll Citation1996; Morrow et al. Citation1996); however, the causal pathological role of neutrophils in particle-induced lung diseases has not been fully investigated or elucidated. In this article, we propose a mechanistic hypothesis of particle-induced pulmonary toxicity that centers on the role of AECs and the recruited neutrophils. This mechanism addresses several toxicological questions including species-specific differences in response to exposure of poorly soluble low-toxicity dusts such as TiO2 and CB.
1.9. No correlation exists between results of particles studies using in in vitro cultures and in vivo animal studies
Warheit’s team conducted comparative in vitro and in vivo toxicity studies of five mineral dusts (Sayes et al. Citation2007). The five dusts were ITI into rats and applied to three in vitro pulmonary assays including coculture of human AMs and rat type II AECs. A large spectrum of biomarkers of toxicity was assessed in the dust-treated cell cultures and cocultures and in the lungs of dust-instilled rats. The authors concluded that “The end points selected to assess oxidative stress and macrophage function yielded little useful information…When considering the range of toxicity end points to 5 different particle types, the comparisons of in vivo and in vitro measurements demonstrated little correlation.” Seagrave et al. (Citation2003) conducted a similar comparative study employing an AM-AEC coculture system and drew the same conclusion that “… the rank order of potency from the in vitro assays in general did not correspond with the previous rankings from in vivo comparisons of the same samples [diesel-related PMs].”
These results also raise questions about the role of particle-activated AMs in producing toxicity to the AECs. The absence of neutrophils in the AM-AEC cocultures may be why Warheit’s team and Seagrave’s Lovelace group did not find a correlation between results of the in vitro and in vivo studies. Driscoll, Deyo, et al. (Citation1997) reported no increases in mutations in RLE6TN cells (rat alveolar type II AEC cell line) that were incubated directly with quartz, CB, and TiO2. However, AECs isolated from the lungs of rats that were instilled 15 months earlier with these dusts had increases in mutations. The increases in mutations detected in RLE6TN cells that were incubated with BAL cells harvested from the lungs of the quartz-instilled rats were attributed mainly to oxidants produced by neutrophils (). Driscoll and co-workers’ findings indicate that particle-elicited alveolar neutrophils induced mutagenesis of AECs in the lung. The EPA (Citation2021) has called for adopting new approach methodologies to reduce the use of animals in toxicity studies. Collectively, the research on lung inflammation after particle exposure, species-specific differences in response, and mutations suggest neutrophils should be incorporated into the in vitro approaches for particle toxicological assays.
Figure 2. (A) Mutagenic effect of alveolar neutrophils or macrophages from quartz-instilled rats on epithelial cells in culture. (B) Relationship between the hprt mutation frequency in alveolar epithelium cells and severity of particle elicited inflammation (% neutrophils) in lungs of rats instilled with the test dusts at 10 mg/kg or 100 mg/kg. Standard deviations are not plotted; refer to original data for accuracy. Data courtesy of Driscoll, Deyo, et al. (Citation1997).
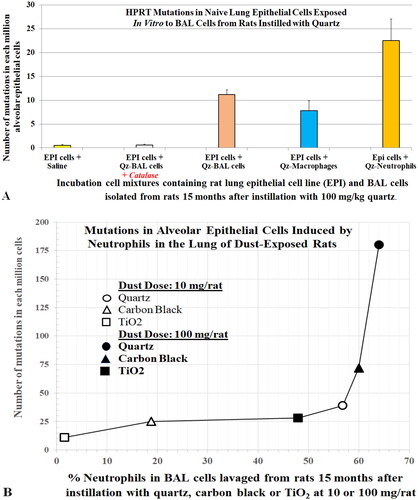
1.10. Neutrophilia is the most common toxicological index for lung diseases
Unlike AMs, the short-lived alveolar neutrophils are difficult to isolate and study in vitro. Furthermore, no alveolar neutrophil cell lines exist due to their short half-life. The role of neutrophils in the pathogenesis of lung diseases has not been fully elucidated. Neutrophilic inflammation is often seen in the lungs of dust-exposed rats that have severe lesions including fibrosis, as well as in many other chronic human lung diseases (Liu et al. Citation2017; Herrero-Cervera et al. Citation2022). The number of neutrophils in lung samples is commonly reported as an index of inflammation and is a common biomarker of toxicity (Oberdörster et al. Citation2005). After human volunteers inhaled concentrated ambient PM, more neutrophils were detected in their BALF (Ghio et al. Citation2000). Sputum samples from human subjects exposed to DPM and ozone contained more neutrophils than those from subjects exposed to the DPM alone (Bosson et al. Citation2007). Increases in neutrophils were observed in BAL samples obtained from volunteers exposed to NO2 and in bronchial mucosa biopsies from ozone-exposed subjects (Aris Citation1993; Helleday et al. Citation1994). Neutrophil influx into the lungs was observed in guinea pigs exposed to sulfuric acid (Amdur and Chen Citation1989). Thus, exposures to airborne oxidative pollutants, even of non-PM pollutants, such as O3, NO2, and sulfuric acid, can trigger pulmonary neutrophilic infiltration and fibrosis (Last et al. Citation1994; Michaudel et al. Citation2018). The pattern of lung damage induced by the PM and oxidative gases is similar, with the primary target being the alveolar epithelium. Unlike gaseous pollutants, insoluble particles will accumulate and remain in the lung with repeated exposures. Alveolar neutrophilia is commonly observed with all these exposures or co-exposures and is considered as an early, important toxicity biomarker. However, neutrophilia has scarcely been considered as a key contributor to particle-induced pathogenesis of lung disease in animals or as a major factor contributing to species-specific differences in particle-induced pathogenesis and carcinogenesis.
1.11. Oxidants from dust-elicited neutrophils are associated with lung lesions
As mentioned above, Driscoll, Deyo, et al. (Citation1997) showed that alveolar neutrophils elicited by in vivo exposure to quartz, CB, and TiO2 could induce mutations in naïve AECs ex vivo. The mutagenic effects were due to inflammatory cell-derived oxidants. Supporting a role for oxidants was the observation that the antioxidant catalase significantly attenuated the mutagenic activity of the particle-elicited rat lung inflammatory cells. Increase in mutation frequency (mf) in AECs induced per million BAL neutrophils increased with the ITI dose of the particles. In vivo assessment of AECs from rats exposed to these PSPs showed quartz was more mutagenic to epithelial cells (mf: Rat-Qz > Rat-CB > Rat-TiO2). Both the ex vivo and in vivo results implicate oxidative stress in this effect. Indeed, it has been determined that neutrophils produce about 2.5 times more ROS than macrophages. Driscoll (Citation1996) concluded that particle-induced neutrophilia in the lung and oxidant-triggered mutations in epithelial cells were key factors in lung carcinogenesis after exposure to PSPs.
Auten et al. (Citation2002) reported a hyperoxia-induced neutrophil influx in the lungs of neonatal rats exposed to 95% oxygen. These rats showed impaired lung development and signs of oxidative DNA damage. When the rats were treated with anti-cytokine-induced neutrophil chemoattractant (CINC), the lesions and the DNA damage in the lung parenchymal cells were prevented. The authors concluded that “neutrophil influx during hyperoxia damages DNA by nicking and oxidation, and that blocking neutrophil influx can prevent this… Neutrophil-mediated oxidative DNA damage may contribute to abnormal lung development in newborns subjected to significant oxidative stress.”
In agreement with Auten’s results showing neutrophil-generated ROS caused DNA damage of AECs of hyperoxia-exposed neonatal rats, Knaapen et al. (Citation1999) reported a correlation between neutrophilic production of oxidative species and damage to DNA in AECs. It is noteworthy that the half-life for neutrophils is several hours and for macrophages, it is ∼15 days (∼360 h). Furthermore, these short-lived neutrophils can undergo secondary necrosis and release their oxidants and other inflammatory molecules (see detail in Section 3.7). With high concentration and prolonged dust exposure, the number of neutrophils recruited into the lung greatly exceeds that of the macrophages. Thus, the macrophages’ ability to remove the dead neutrophils can be overwhelmed. These factors suggest that neutrophils can be a major endogenous source of oxidants in the lung.
1.12. A newly proposed unifying mechanism centered on the concerted actions of AECs and the recruited neutrophils
The results of the study on quartz, CB, and TiO2 discussed above allowed Driscoll (Citation1996) to establish the links between particle-induced inflammation and oxidative mutagenic events in AECs and to propose that inflammation plays a critical role in the mechanism by which particles induce pulmonary pathology in rats.
The NASA LD toxicity assessment team reported standard toxicity biomarkers in BALF and pulmonary histopathology in rats exposed to airborne LD for 4 weeks (Lam et al. Citation2013) and in rats that had been ITI with five test dusts (three LDs, quartz, and TiO2) (Lam et al. Citation2022). Presented in this paper are our follow-up measurements of the oxidant contents of BAL neutrophils from these exposed rats. The results from the rats in the ITI study show that neutrophilic oxidants increased in dose-dependent, time-dependent, and dust-dependent (Qz > LD > TiO2) fashions. Levels of IL-6 and TNFα, two cytokines involved in neutrophil recruitment and activation, were higher in the BALF of rats exposed to LD by inhalation. Furthermore, expression of many of the proinflammatory genes increased in the AECs of these LD-exposed rats (Zhang et al. Citation2023). For example, CXCL2 gene (codes for neutrophil chemokine) expression in the lung increased with the exposure concentration and post-exposure time. All these biomarkers correlated with the lung histopathological changes in the LD-exposed rats (Lam et al. Citation2013, Citation2022). These findings provide evidence that oxidants released from the recruited neutrophils led to the pathogenesis of disease in the lung.
Our experimental results on neutrophilic oxidants reported here and the results of others shed light on the toxicological roles of neutrophils and led us to propose a refined mechanism of particle-induced pathogenesis of lung disease where particles deposit on the alveolar surface stimulating surface receptors, such as scavenger receptors, on AECs. The inflicted AECs synthesize and release cytokines, which recruit neutrophils and subsequently activate the neutrophils to increase and release oxidants and proteinases. These harmful cellular products could be released from the large number unphagocytized neutrophils that undergo secondary necrosis in lungs with severe neutrophilia (see Section 3.7). The continuous release of oxidants and harmful proteinases by the alveolar neutrophils contributes to the persistent oxidative stress that leads to histopathological changes in the lung. As presented in Section 3 of this paper, our refined mechanism of particle toxicity would help explain why nanoparticles are more toxic than micron-size particles of the same exposure mass concentration, and why dust-induced lesions progress with time in the lung of exposed rats. Furthermore, this proposed mechanism provides a basis for species-specific differences in responses to particle toxicity (i.e. why the same dust at the same concentration induces more severe lesions, including cancer, in rats than hamsters) and explains the existence of thresholds in dose–response curves of particle toxicity. This proposed mechanism should be useful for assessing human health risk from exposures to poorly soluble mineral dust and environmental PM.
2. Materials and methods
2.1. General notes for materials and methods section
Some of the information in this section that are reported in the present paper have not been reported or published elsewhere. Others are briefly described here and more detailed information can be found in previous publications (Lam et al. Citation2013, Citation2022; Zhang et al. Citation2023).
2.2. Laboratory animals
Fischer 344 adult male rats (∼8–10 weeks old) were purchased from Charles River Laboratories (Raleigh, NC). The animals were housed in pairs in an AAALAC-approved animal facility at the NIOSH (Morgantown, WV) or NASA Johnson Space Center (JSC, Houston, TX) and had free access to water and standard laboratory rodent chows. Both facilities provided HEPA-filtered air and a 12-h light/dark cycle. These studies were approved by the NIOSH or the NASA Institutional Animal Care and Use Committee and the guidelines and approved test protocols were followed.
2.3. Test dusts of respirable sizes for rat ITI studies
2.3.1. LD samples
An Apollo-14 lunar regolith (200 g; stored in nitrogen from the time it was collected from the moon) was used for the present study. The composition of the lunar regolith by weight is 48% SiO2 (amorphous), 20% Al2O3, 12% CaO, 7.5% FeO, 7% MgO, and 2% TiO2 (McKay et al. Citation2015). A fine dust fraction was aerodynamically separated from this regolith using a cyclone, and the respirable dust was captured on a paper membrane filter and was designated lunar dust, unground, respirable size (LD-ug) for the current study. Aliquots of the coarser dust that was captured in the cyclone were ground using a jet-mill or a zirconia-ball mill (see Lam et al. Citation2022 for grinding procedures). Respirable dusts of the ground samples were similarly isolated using a cyclone. The particle size distributions were determined using a Microtrac (Haan, Germany). The mass median diameter (MMD) of the unground fine dust was 2.1 (+0.6/–0.9) µm; jet-milled lunar respirable dust (LD-jm) was 2.5 (+1.0/–0.7) µm; the MMD for the ball-milled lunar respirable dust (LD-bm) was 1.8 (+0.5/–0.9) µm (McKay et al. Citation2015).
2.3.2. Quartz dust and TiO2
Quartz dust, a fine-sized crystalline silica (Min-U-Sil 5; MMD 1.6 µm), was obtained by NIOSH from U.S. Silica (Berkeley Springs, WV) many years ago and has been used in multiple studies published by NIOSH investigators. The TiO2 (rutile pigment R-100, consisting of ∼99% TiO2, ∼1% alumina) used in the present study was produced by DuPont Company (Newark, DE) and was a gift from Dr. D. Warheit. The average primary particle size of this dust was about 0.4 µm. Both reference dusts were used without further treatment and considered to be aged dusts.
2.4. Measurement of hydroxyl radicals on the dusts
Hydroxyl radicals (●OH), the most powerful oxidant among the ROS (Wardman Citation1989), are stable enough to be detected in an aqueous system. Terephthalate is a sensitive probe for ●OH (Saran and Summer Citation1999; Wallace et al. Citation2009), although it is possible that it detects other types of ROS as well and is not specific to ●OH (Turci et al. Citation2015). The test dusts were assessed for chemical reactivity by measuring the amount of ●OH generated per unit weight of dust in the presence of terephthalate (Wallace et al. Citation2009). Each dust was tested in triplicate by adding an equal amount of dust to a terephthalate solution. The resultant fluorescence, which was proportional to the amount of ●OH present, was spectrophotometrically determined.
2.5. Intratracheal instillation of dusts
The NASA lunar geology team coded the five test dusts (three LDs, TiO2, and quartz) as A, B, C, D, and E, and weighed each aliquot of dust samples under nitrogen in an astromaterial hood. The dust toxicity testing team and the pathologists were blinded to the identity of the codes. Each of the test dusts was suspended in 10% Survanta® (which was used to disperse the LD samples) in normal saline and vortexed slightly immediately before instilling into the rats (six rats/group). Each anesthetized rat was instilled with 0.4 mL of this dispersion medium containing 0, 1, 2.5, or 7.5 mg of a test dust.
2.6. LD inhalation exposures
The details of the LD nose-only inhalation study and the assessment of BALF biomarkers of toxicity and histopathology were previously reported (Lam et al. Citation2013). Briefly, groups of five rats were exposed to 0, 2.1, 6.8, 20.8, and 60.6 mg/m3 of LD for 4 weeks (6 h/d, 5 d/week). The mass median aerodynamic diameter (MMAD) of the aerosolized dust was 2.4 µm. One day or 1, or 4, or 13 weeks after the inhalation exposure, the right lungs were lavaged and BALF was assessed for biomarkers of toxicity, and a section of the median lobe of the lavaged right lung was collected from each animal for gene profiles and toxicogenomic analyses (Zhang et al. Citation2023). The left lung was fixed with formalin and prepared for histopathology study (Lam et al. Citation2013).
2.7. Collection of BALF samples
To assess biomarkers of toxicity in BALF samples, rats were euthanized with an overdose of Sleepaway (containing pentobarbital; Fort Dodge Animal Health, Fort Dodge, IA) at 1 or 4 weeks after the dust instillation. Following NIOSH in-house protocols (Roberts et al. Citation2012), the lung was lavaged three times with 6 mL of phosphate-buffered saline. The first lavage was centrifuged, and the supernatant was collected and assessed for acellular biomarkers in BALF. The cell pellets of the first and subsequent lavages were combined and suspended in 1 mL of HEPES-buffered solution for assessment of cell numbers and differentials.
2.8. Assessment of BAL cells
Aliquots of cells were placed on microscope slides and centrifuged in a Shandon CytoSpin II; the cells were stained with Wright-Giemsa dye solutions. Cell differentials (in percentage) for neutrophils and macrophages were determined by visually counting 300 cells. The numbers of macrophages and neutrophils in the BALF sample from a rat were obtained by multiplying the total number of BAL cells by the percentage of macrophages or neutrophils, respectively. All BAL cell and acellular assays followed NIOSH standard protocols (Roberts et al. Citation2012).
2.9. Chemiluminescence (CL) assay for measuring of ROS levels in BAL cells
The CL assay, which used zymosan to stimulate inflammatory cells to produce CL, can indirectly measure ROS generated by human blood neutrophils, monocytes, or rat BAL cells (Castranova et al. Citation1990; Antonini et al. Citation1994). The CL assay was used to measure the oxidant production by BAL cells, as described by Porter et al. (Citation2002). Briefly, BAL cell aliquots were incubated at 37 °C for 20 min. Luminol (5-amino-2,3-dihydro-1,4-phthalazinedione) solution was added to the BAL cell suspensions to achieve a final luminol concentration of 0.08 mg/mL. CL was measured at 390–620 nm for 15 min using an automated luminometer (Berthold AutoLumat Plus LB 953, Gaithersburg, MD). Zymosan (2 mg/mL) was added, and CL was then measured. Zymosan-stimulated CL was determined as counts per min per million BAL cells. This assay was performed at NIOSH (Morgantown, WV), where ITI experiments were conducted. For the follow-up LD inhalation studies (Lam et al. Citation2013), all experiments including the CL assay using a Berthold Centro LB 960 luminometer (Gaithersburg, MD) were conducted at the NASA JSC (Houston, TX).
2.10. Collection of BALFs from LD-exposed rats and assessment of BALF cytokines
Concentrations of the several cytokines in BALFs were determined by multiplex array. The rat Fluorokine MAP Multi-Analyte Profiling immunoassay was performed according to the manufacturer’s instructions (R&D Systems, Minneapolis, MN). This array, which analyzes several cytokines simultaneously using distinct bead populations that fluoresce at different intensities along a single emission wavelength, was performed in a 96-well plate and was analyzed using a Luminex 100 instrument.
2.11. Collection of lung tissue and isolation of total RNA for gene activation study
After the right lung of each animal was lavaged, a section of the median lobe of the right lung was removed and snap-frozen in liquid nitrogen, preserving the tissue for extraction of RNA. Total RNA was isolated from each of these samples (<20 mg) using Qiagen Mini RNeasy Kit (Qiagen, Valencia, CA) according to the protocol recommended by the manufacturer. The total RNA was treated with DNase I to remove any trace contamination of genomic DNA prior to subsequent analyses. The concentration and integrity of the purified RNA were measured using Nanodrop™ 2000 (Thermo Fisher Scientific, Waltham, MA) and an Agilent Bioanalyzer (Agilent Technologies, Inc., Santa Clara, CA). RNA integrity number of all the samples ranged from 9.9 to 10 (see more details in Zhang et al. Citation2023).
2.12. Real-time polymerase chain reaction (RT-PCR) analysis of four chemokines and fibrosis-related genes
The RT2 Profiler PCR Array technique (Qiagen, Valencia, CA) was used to measure expression of fibrosis-related genes in the control group of animals and the group exposed to the highest LD exposure concentration at different time points after exposure. The RT-PCR technique was also used to measure expression of four chemokines in all the RNA samples isolated from all the air and LD-exposed rats that were sacrificed at the four time points after exposure. For each analysis, the cDNAs were synthesized from 1 µg of total RNA from each sample using a QuantiTect Reverse Transcription kit or a RT2 PCR array first strand kit and primers for Ccl12, Ccl3, Cxcl2, and Cxcl5 (Qiagen, Valencia, CA). Primers for Ccl12, Ccl3, CXCL2, and Cxcl5 were purchased from Qiagen (Valencia, CA). The expression of β-actin and Rpl13a served as reference controls for analyses of both fibrosis-related and chemokines encoding genes. Within these gene families of interest, the level of expression of individual genes was assessed in cDNA synthesized from total RNA using a Bio-Rad CFX96 real-time PCR system (Hercules, CA) and Qiagen’s SyBR Green kit (Valencia, CA). Reverse transcription negative reactions were run on each plate to confirm the absence of genomic DNA contamination. Relative expression values were calculated by Qiagen’s Ct analysis using the average of two reference genes and normalized to experimental controls for fold changes. All the data are presented as average fold changes ± SD (see more details in Zhang et al. Citation2023).
3. Results and discussion
3.1. Toxicity of lunar dust and assessment of exposure to lunar dust containing particle surface ROS
3.1.1. Comparison of the toxicity of LD with terrestrial dusts and an assessment of impact of psROS on dusts’ toxicity
The United States is planning return missions to the Moon for long-duration exploration and research. During lunar surface expeditions, astronauts could be exposed to fine LD that could contain high levels of psROS. To assess risk of in situ LD exposures and to investigate the toxicity of LD using samples of lunar regolith collected 50 years ago whose psROS might have become passivated during the long storage, the NASA LD geology team (McKay et al. Citation2015) ground (by jet mill and by ball mill) fine aliquots of an Apollo-14 lunar regolith to produce two dust samples (LD-jm and LD-bm) with increased or restored levels of psROS (). These two LDs together with the unground LD were toxicologically compared with TiO2 and quartz in rats (Lam et al. Citation2022). The results () showed that the pulmonary toxicity of these three respirable LDs was indistinguishable, despite their 14-fold difference in particle-generated hydroxyl free radicals (●OH; the most toxic of the ROS and stable enough for detection). LD was moderately toxic, more toxic than TiO2. Quartz was the most toxic among the five test dusts () but contained the least amount of psROS (). The overall results revealed that psROS had no significant impact on the dust’s toxicity in the lung (see Lam et al. Citation2022 for details).
Figure 3. Rats were intratracheally instilled with lunar dusts (LD), TiO2, and quartz (SiO2) at 0 (control), 1, 2.5, or 7.5 mg/rat. The lungs were lavaged 1 or 4 weeks after the dust instillation. Numbers of alveolar macrophages (A) and neutrophils (B) were determined. ROS of total BAL cells was assessed by chemiluminescence (C). X-axis labels: jet-milled LD: LD-jm (green); unground LD: LD-ug (blue); ball-milled LD: LD-bm (red); bars of lighter color-shades represent 1 week; bars of darker color-shades represent 4 weeks. Each bar is the mean ± SD from six rats.
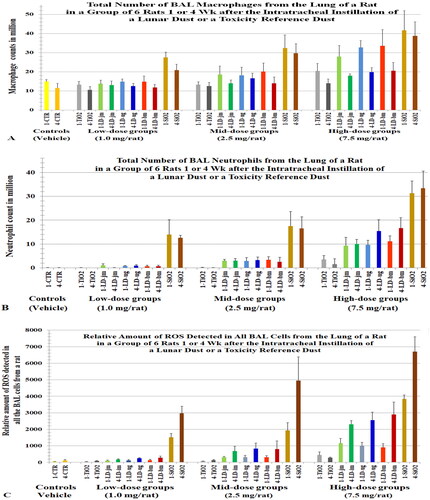
Figure 4. Relative ROS levels in BAL cells from rats instilled with lunar dusts (LD), TiO2, and quartz (SiO2) at 0 (control), 1, 2.5, or 7.5 mg/rat. The lungs were lavaged 1 or 4 weeks after the dust instillation. ROS in BAL cells were assessed by a chemiluminescence assay. X-axis labels: jet-milled LD: LD-jm (green): unground LD: LD-ug (blue); ball-milled LD: LD-bm (red); bars of lighter color-shades represent 1-week groups; bars of darker color-shades represent 4-week groups. Each bar is the mean ± SD from six rats.
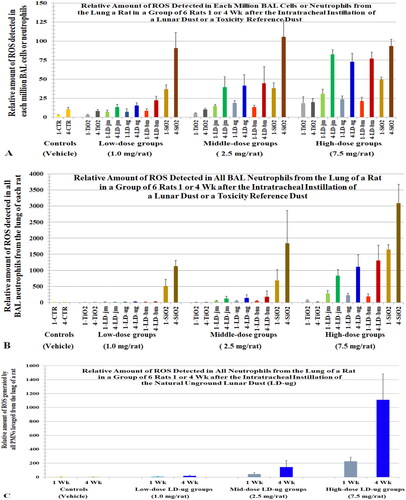
Figure 5. (A) A replica of (levels of ROS in all BAL cells) plotted here to ease comparison. (B) ROS levels in all BAL neutrophils () were multiplied by 2 and plotted here () to compare with the ROS levels of all BAL cells (). Cells were lavaged from rats 1 or 4 weeks after they instilled with lunar dusts (LD), TiO2, and quartz (SiO2) at 0 (control), 1, 2.5, or 7.5 mg/rat. Note, we initially assumed that the level of oxidants generated by a neutrophil = the level of oxidants generated by a BAL cell; however, data from Driscoll, Deyo, et al. (Citation1997) showed that the oxidative capacity of a neutrophil is twofold of that of a BAL cell from rats exposed to quartz. X-axis labels: jet-milled LD: LD-jm (green): unground LD: LD-ug (blue); ball-milled LD: LD-bm (red); bars of lighter color-shades represent 1-week groups; bars of darker color-shades represent 4-week groups.
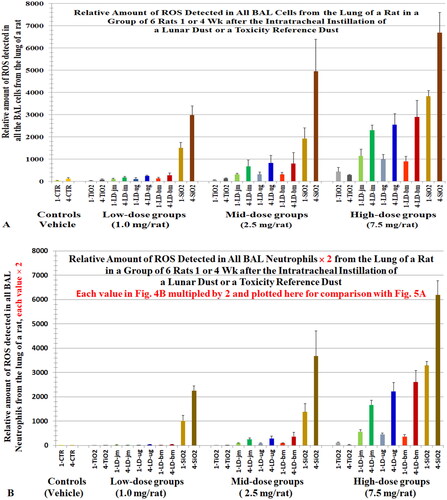
Harrington of NASA’s geology group collaborated with a particle research team from New York University to investigate the toxicity of six ground meteorite dusts and a ground terrestrial basalt dust containing various amounts of ROS and iron. The results of their mouse ITI study led the authors to conclude that, “The MORB [terrestrial basalt] demonstrated higher geochemical reactivity [i.e. ROS & soluble iron levels] than most of the meteorite samples but caused the lowest acute pulmonary inflammation (API)… there is no direct correlation between a particle’s ability to generate ROS acellularly and its ability to generate API.” This 7-dust study in mice (Harrington, McCubbin, et al. Citation2017) and the 5-dust study in rats reported here and in Lam et al. (Citation2022) show that psROS did not contribute to the toxicity of the dusts in the lung. These results nullify the long-held hypothesis that psROS is the key determinant of particle-induced pathogenesis of lung disease.
3.1.2. Risk to astronauts on the moon from exposure to LD containing free radicals
Because astronauts on the moon could be exposed to LD containing ROS, articles by Vandette (Citation2018) and Specktor (Citation2018) have claimed that LD is highly toxic based on the findings by Caston et al. (Citation2018) that ground LD simulant (a terrestrial volcanic ash) contained free radicals. Caston concluded that, “the ability of the simulants to produce ROS …was not correlated with the observed cytotoxic or genotoxic effects” in their human cell culture studies. Studies of rats exposed to authentic Apollo14 LD revealed the LD is in fact only moderately toxic (Lam et al. Citation2013). Although LD is expected to contain psROS in situ, our 5-dust ITI study showed that psROS does not contribute significantly to the pulmonary toxicity of LD (Lam et al. Citation2022), which will alleviate some of the concerns of the NASA engineers responsible for designing a clean lunar living-quarter and those of the Artemis astronauts. In light of these data, NASA has established exposure limit for LD for expeditions on the moon of up 6 months. The LD permissible exposure limit was based on the data of the no-observable-adverse-effect-level (NOAEL) for rats exposed to airborne LD for 4 weeks (Lam et al. Citation2013). This LD exposure limit is 20-fold lower than the NOAEL and was approved by a NASA-appointed Non-Advocate Review Committee consisting of prominent particle toxicologists.
3.2. Alveolar epithelial cells are the key lung cells recruiting neutrophils into the lung
It is not easy to isolate AECs from the lung; however, it is relatively easy to obtain AMs and show that they can produce cytokines. Therefore, cytokines found in BALF are often thought to come exclusively from macrophages. It is well established that many cell types in the lung can produce inflammatory cytokines including endothelial cells, epithelial cells, and resident macrophages (many near the interface with the external environment; Boyle Citation2005). When particles land deep in the lung, they first contact the AECs. However, AECs (the “victims”) are seldom thought to be involved in sending out “SOS” cellular messengers to recruit inflammatory cells.
Studies of infectious diseases indicate that the AECs synthesize cytokines to recruit inflammatory cells into the lung when exposed to microbial particles (Bello-Irizarry et al. Citation2012; Alon et al. Citation2021). The results of a study conducted on several mineral dusts by Finkelstein et al. (Citation1997) that examined the proinflammatory cytokine gene activation in lung tissues also indicated that particle-inflicted AECs were involving in recruiting the leukocytes to the lung of dust-exposed animals. The authors noted that, “effects on the epithelium are due to direct interactions with particles, not a result of macrophage-derived mediators.” These data indicate AECs, not macrophages, are the key cell-type in the lung that releases cytokines to recruit neutrophils after particle exposure. Further support for the direct role of epithelial cells in expression of particle-induced inflammatory cytokine comes from Driscoll et al. (Citation1996); Driscoll, Carter, et al. (Citation1997). The authors concluded that “… rat lung epithelial cells are key contributor to chemokine expression in the lung after exposure to α-quartz and potentially other noxious particles” (Driscoll et al. Citation1996).
3.3. Contribution of neutrophils to oxidative stress and disease in the lung
3.3.1. Lung toxicity correlated with alveolar neutrophils in dust-exposed rats
The causal relationship between alveolar neutrophilia and lung pathology has been well demonstrated in studies with TiO2. Lee et al. (Citation1985) reported that rats exposed to 250 mg/m3 pigmentary or µm-sized TiO2 (µm-TiO2; primary size (ps) <1 µm) for 2 years developed tumors in the lungs. Heinrich et al. (Citation1995) also reported that rats developed tumors when chronically exposed to 10 mg/m3 ns-TiO2 (ps 14 nm). Bermudez et al. (Citation2002, Citation2004) exposed rats to 250 mg/m3 of µm-TiO2 (ps < 1 µm) or 10 mg/m3 of ns-TiO2 (ps 2 nm) for 13 weeks and showed that neutrophils accounted for 83% and 65% of recovered BAL cells, respectively. Alveolar epithelial hyperplasia and metaplasia, and septal fibrosis were also observed. In these studies, the severity of lung lesions correlated with the levels of BAL neutrophils. Driscoll, Deyo, et al. (Citation1997) reported that increased mf in AECs of rats exposed to either quartz, CB, or TiO2 was dose- and dust-dependent (mf: Qz > CB > TiO2) and the mutations were attributed mainly to the oxidants produced by the recruited neutrophils (). During persistent particle-induced inflammation, continuous release of oxidants from neutrophils could lead to lung lesions, mutagenesis, and carcinogenesis (Driscoll Citation1996).
3.3.2. The concerted responses of particle-afflicted AECs and the recruited neutrophils start the disease process
Presented in the current manuscript are experimental data from LD studies assessing the toxicological role of oxidants generated by alveolar neutrophils. These results and relevant data from other published research support the relationship between neutrophilic inflammation and persistent oxidative stress in the lungs is a key factor in the AOP of dust-induced lung disease. Our proposed AOP of dust toxicity in the lung involves the concerted responses of the particle-stimulated AECs and the recruited alveolar neutrophils: dust exposure stimulates cytokine release from AECs, which recruit the inflammatory cells to the lung, and the persistent neutrophilia is a source of continuous release of oxidants and other harmful cellular contents that lead to pulmonary pathogenesis.
3.3.3. Level of oxidants produced by BAL cells including neutrophils estimated using the CL assay that requires zymosan
3.3.3.1. Measuring alveolar neutrophils’ oxidant levels with the CL assay used for blood neutrophils
The CL assay, with stimulates test cells with zymosan, has been used to measure levels of ROS generated by human and rat neutrophils and macrophages (Castranova et al. Citation1985, Citation1990; Antonini et al. Citation1994; Porter et al. Citation2002). A NIOSH study employed this CL assay to measure oxidants generated by BAL cells obtained from silica exposed rats (Porter et al. Citation2002). The authors attributed the CL signal generated by the BAL cells to macrophages only. Support for their claim that macrophages respond to unopsonized zymosan but neutrophils (isolated from dust-exposed animals) do not was based on the studies by Allen (Citation1977) and Hill et al. (Citation1977) showing human blood neutrophils cannot respond to unopsonized zymosan unless opsonized by C3 compliment. It is noteworthy that the studies of Allen (Citation1977) and Hill et al. (Citation1977) were conducted on untreated normal human blood neutrophils and showed that zymosan would need to be opsonized by C3 in serum unless in the presence of bivalent cations (Ca2+ and Mg2+).
3.3.3.2. Neutrophils in the presence of macrophages can interact with zymosan and undergo an oxidative burst
Ezekowitz et al. (Citation1985) showed that macrophages can secrete C3 to opsonize zymosan. After studying a macrophage–neutrophil co-culture in the presence of unopsonized zymosan, Ezekowitz et al. (Citation1985) concluded that “[(1)] Mϕ complement and/or other products enable the PMN to react with zymosan particles in the absence of serum; [(2)] PMN are attracted to zymosan-bearing Mϕ, aggregate, and degranulate with resultant destruction of macrophages as well as PMN, presumably a result of the vigorous respiratory burst; [(3)] PMN clustered around Mϕ-containing zymosan and released large amounts of O2–.” Neutrophil and PMN were used interchangeably by these authors in their paper.
3.3.3.3. Neutrophils can interact with un-opsonized zymosan
Several groups reported that human neutrophils can phagocytize unopsonized zymosan or yeast (Ross et al. Citation1985; Makni-Maalej et al. Citation2013; Gupta-Wright et al. Citation2017). Yuen et al. (Citation2016) noted that “Neutrophils also carry key components of the complement alternative pathway (AP) such as properdin or complement factor P (CFP), complement factor B (CFB), and C3.”
3.3.3.4. TNFα can activate neutrophils to secret C3 to opsonize zymosan
Botto et al. (Citation1992) and Camous et al. (Citation2011) showed that TNFα-activated neutrophils produced and secreted C3. Our NASA LD toxicity assessment team detected TNFα in the BALF from our LD-exposed rats (Crucian et al. Citation2014). These results support a mechanism whereby the recruited neutrophils in the lungs of dust-exposed rats could be activated by TNFα and other mediators that enabled neutrophils, like macrophages, to produce C3. C3 from the alveolar neutrophils and macrophages of dust-exposed rats could opsonize zymosan, triggering both neutrophils and AMs to release O2 in a CL assay.
3.3.3.5. Alveolar neutrophils from dust-exposed rats differ from neutrophils from normal human blood
Human blood neutrophils and monocytes are not activated. Monocytes entering the lung are activated and become macrophages. Driscoll, Deyo, et al. (Citation1997) showed that the oxidants released from alveolar neutrophils that were isolated from dust-exposed rats caused mutations in naïve RLE6TN cells (rat alveolar type II AEC cell line) in vitro (). The mf increased with the oxidant contents of the alveolar neutrophils ([Ox]Nu), which correlated with the dose and cytotoxicity of the dust ([Ox]Nu: Rat-Qz > Rat-LD > Rat-TiO2) (). Therefore, neutrophils in the lungs of dust-treated animals have been activated or modulated by cytokines and other cellular mediators. The alveolar neutrophils from dust-exposed rats would differ from untreated blood neutrophils from humans in the ability to interact with unozonized zymosan.
3.3.3.6. Levels of CL produced by neutrophils
The data presented suggest that neutrophils can interact with zymosan without the zymosan being pre-opsonized with C3 or serum. The NASA toxicology team used the NIOSH CL assay protocol to measure ROS in BAL cells. Because it is difficult to separate neutrophils from macrophages in studies involving a large number of animals (our dust ITI study conducted at NIOSH used 192 rats (five dusts, three doses and two time points, plus control groups), Lam et al. Citation2022). NASA researchers performed the standard cell differential counts of BAL cell preparations. The CL assay estimated the oxidant levels per million BAL cells. In the dust-exposed rats, BAL cells consisted of essentially macrophages (Mϕ) and neutrophils; therefore, it was conservatively assumed that oxidant level per BAL cell ([Ox]BC) = oxidant level per macrophage ([Ox]Mϕ) = oxidant level per neutrophil ([Ox]Nu). It is noteworthy that Driscoll, Deyo, et al. (Citation1997) showed in a quartz-exposed rat, the oxidant level in a neutrophil was about 2.5-fold higher than that in a macrophage, and BAL cells enriched with neutrophils were more oxidatively mutagenic than the same number of BAL cells containing a higher percentage of macrophages, which indicates [Ox]Nu > [Ox]Mϕ.
3.4. Levels of ROS in BAL neutrophils harvested from the rats in the ITI studies correlated with the exposure doses and cytotoxicity of the test dusts
Using the CL assay on BAL cells harvested from rats 1 or 4 weeks after they were instilled with one of five test dusts (Lam et al. Citation2022) or one day, 1, 4, or 13 weeks after the 4-week LD inhalation exposure (Lam et al. Citation2013), the relative levels of ROS produced by each million neutrophils or per neutrophil ([Ox]Nu) was estimated, assuming [Ox]Nu = [Ox]BC, as noted above. The following are our findings on ROS relevant to the dust-induced lung toxicity.
3.4.1. BAL cells counts and levels of ROS generated by BAL cells from rats instilled with five test dusts
The number of macrophages and neutrophils in BALF samples from the rats in the ITI studies are shown in (number of neutrophils, η). The estimated relative levels of ROS generated by the total BAL cells are shown in and the relative level of ROS per million BAL cells or neutrophils (value per neutrophil: [Ox]Nu) are shown ; the sum of ROS generated by total BAL neutrophils (∑[Ox]Nu = η × [Ox]Nu) are shown in . shows that ROS generated by all the BAL neutrophils increased with instilled dose and exposure duration (∑[Ox]Nu: 4 weeks > 1 week). The data for rats instilled with unground LD, shown in , have been replotted in to better illustrate that the neutrophilic oxidants increased with dose and with the time that the instilled particles remained in the lung (∑[Ox]Nu: 4 weeks > 1 week). These dose- and time-dependent patterns were similarly reflected in the pathological changes detected in the lung (Lam et al. Citation2022).
3.4.2. The levels of ROS in BAL neutrophils correlated with the toxicity of test dusts
The levels of ROS in BAL neutrophils harvested from the lungs of the rats in the NASA 5-dust ITI study increased following the order of cytotoxicity of the dusts (i.e. Qz > LD > TiO2). Rats instilled with quartz showed the highest levels of ROS per million cells, and those instilled with TiO2 had the lowest levels (). All three LDs produced intermediate levels of ROS per million BAL neutrophils. These neutrophilic oxidative biomarkers were consistent with the pulmonary pathology and with some other toxicity biomarkers in the lungs produced after exposure to these dusts (Lam et al. Citation2022). It is noteworthy that the neutrophilic oxidants, inferred from the extent of oxidative mutation, in dust-exposed rats increased with the cytotoxicity of the dusts (Qz > CB > TiO2) as reported by Driscoll, Deyo, et al. (Citation1997).
3.4.3. The total amount of oxidants generated by BAL cells (∑[Ox]BC) correlated more closely with the number of neutrophils than with the number of macrophages
In the 5-dust ITI study, the total amount of oxidants in all BAL cells () correlated more closely with the total number of neutrophils () than with the total number of macrophages (). shows that the amount of oxidants detected in all BAL cells (∑[Ox]BC) of the rats exposed to the high dose for 4 weeks were higher than these of the rats exposed to the high dose for 1 week. However, the rats exposed to the high dose for 4 weeks had less macrophages () than the rats exposed to the high dose for 1 week.
3.4.4. Total oxidants in BAL cells (∑[Ox]BC) vs. 2-times the ROS in all neutrophils (2 × ∑[Ox]Nu)
In the mutation study mentioned above, Driscoll, Deyo, et al. (Citation1997) showed that a million neutrophils were about 2- to 2.5-fold more oxidatively mutagenic than the same number of BAL cells or AMs, respectively (). We stated earlier that we had assumed for our calculation that the level of oxidants per BAL cell ([Ox]BC) = level of oxidant per macrophage and ([Ox]Mϕ) = level of oxidant per neutrophil ([Ox]Nu). However, this likely underestimated the level of oxidants from a neutrophil relative to a mixed BAL cell population and a macrophage population. Assuming a million neutrophils generated twofold more oxidants than the same number of BAL cells (i.e. [Ox]Nu = 2 × [Ox]BC), we replotted the levels oxidants generated by all neutrophils for the ITI study (: ∑[Ox]Nu yielding : 2 × ∑[Ox]Nu). For the doses that showed toxicity in the ITI dust study, the profile or pattern of the bar graphs (2 × ∑[Ox]Nu) in closely matches the ∑[Ox]BC profile in . These suggest that the neutrophils contributed more than macrophages to the oxidants detected in all BAL cells (∑[Ox]BC).
3.5. Levels of ROS in BAL neutrophils harvested from the rats in the LD inhalation studies increased with the LD exposure concentrations and post-exposure time
3.5.1. BAL cell counts and levels of ROS generated by BAL neutrophils from rats exposed to airborne LD
We also determined the differential cell counts and ROS levels in BAL cells from the inhalation-exposed rats. The number of macrophages and neutrophils (η) are shown in , respectively. shows the ROS values estimated from the total BAL cells. The relative neutrophilic oxidant per cell ([Ox]Nu) or per million cells and the total neutrophilic oxidants (∑[Ox]Nu = η × [Ox]Nu) are shown in , respectively. These neutrophilic oxidants (∑[Ox]Nu = η × [Ox]Nu), which reflect oxidative stress produced by the recruited neutrophils in the lung, correlated with the pulmonary toxicity observed in the LD inhalation study (Lam et al. Citation2013).
Figure 6. Rats were exposed to lunar dust by inhalation at 0, 2.1, 6.8, 20.8, or 60.6 mg/m3 for 4 weeks and the right lungs were lavaged thereafter at 1 d and 1, 4, and 13 weeks. BAL cells were harvested; macrophages and neutrophils were counted and plotted in (A) and (B), respectively. ROS generated by all BAL cells were assessed by chemiluminescence. Each bar is the mean ± SD from five rats.
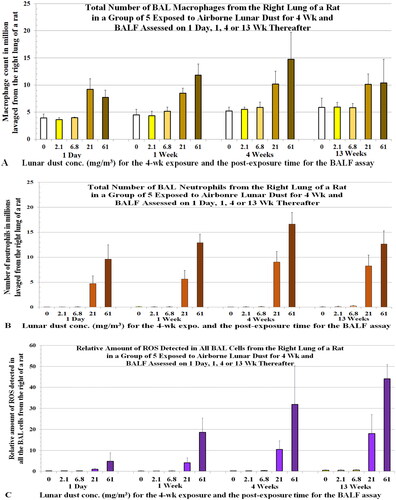
Figure 7. Rats were exposed to lunar dust by inhalation at 0, 2.1, 6.8, 20.8, or 60.6 mg/m3 for 4 weeks. The right lungs were lavaged thereafter at 1 d and 1, 4, and 13 weeks. (A) The relative amount of ROS from all BAL cells or neutrophils (estimated by a chemiluminescence assay) and (B) the relative amount of ROS detected in all neutrophils from each of the groups of animals. Each bar is the mean ± SD from five rats.
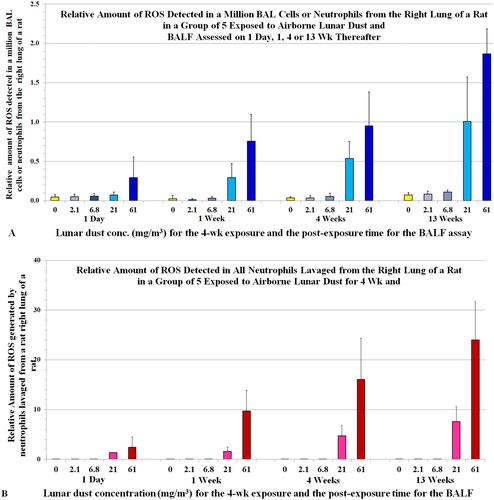
3.5.2. The concentration-dependent and post-exposure time-dependent increases in levels of ROS in BALF closely correlated with neutrophil counts
shows the ROS values estimated from the total BAL cells from rats in the LD inhalation study. The levels of BAL ROS () correlate closely with BAL neutrophil counts (), and less with macrophage counts (). This finding suggests the neutrophils produced more of the ROS in the BAL cells than macrophages did. Furthermore, the BAL cells from rats exposed to 60.6 mg/m3 had higher levels of oxidants than those from rats exposed to 20.8 mg/m3 for all the post-exposure time points; this finding is clearly reflected in the neutrophil counts () but not in the microphage counts (). The relative level of ROS per million BAL cells or neutrophils (value per neutrophil: [Ox]Nu) is shown in , whereas shows that ROS is generated by all the BAL neutrophils. Both figures show neutrophil oxidants increased with exposure concentration and post-exposure time. These data were also consistent with our histopathological findings (Lam et al. Citation2013).
Similar to Section 3.4 (the ITI study), we replotted the data of the levels of oxidants generated by all neutrophils (: ∑[Ox]Nu) to yield the profile of 2 × ∑[Ox]Nu (). closely matches with that of ∑[Ox]BC () for the two high LD inhalation concentrations (21.8 and 60.6 mg/m3) that produced lung toxicity. These suggest that the neutrophils contributed more than macrophages to the oxidants detected in all BAL cells (∑[Ox]BC). The LD at exposure concentrations of 2.1 and 6.8 mg/m3 that resulted in no neutrophil recruitment and toxicity in the lung were the NOAELs of the 4-week LD inhalation (Lam et al. Citation2013).
Figure 8. (A) Relative amount of ROS in all BAL cells of rats one day or 1, or 13 weeks after they were exposed to airborne lunar dust (a replica of plotted here to ease comparison. (B) ROS levels in all BAL neutrophils were multiplied by 2 and plotted here to compare with the ROS levels of all BAL cells (). Note, we initially assumed that the level of oxidants generated by a neutrophil = the level of oxidants generated by a BC; however, data from Driscoll, Deyo, et al. (Citation1997) showed that the oxidative capacity of a neutrophil is twofold of that of a BAL cell from rats exposed to quartz.
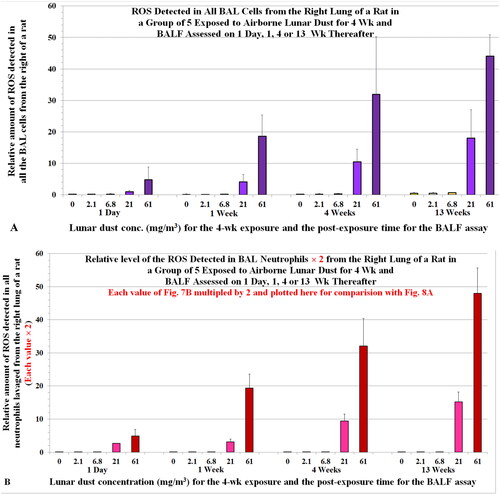
We also observed a dose-dependent and time-dependent increase in oxidant level per million BAL cells (or per cell, [Ox]BC) in the LD inhalation study, which correlated with the number of neutrophils (correlation coefficient r = ∼0.8), but not with the number of macrophages (r = 0.46) (Zhang et al. Citation2023). These findings further suggest that the oxidants detected in the BAL cells in the lungs of rats that have substantial inflammation are derived mainly from the alveolar neutrophils.
3.5.3. The level of oxidants in BAL cells correlates more closely with the number of neutrophils than the number of macrophages in studies reported by others
Castranova’s team instilled rats with residual oil fly ash and Listeria monocytogenes and found that the total CL generated by all neutrophils was greater than that generated by all macrophages despite the number of BAL neutrophils being much fewer than the number of macrophages (Roberts et al. Citation2007). Albrecht et al. (Citation2005) assessed ROS by measuring hydrogen peroxide in the lungs of quartz-instilled rats and found that this measure correlated with the total number of neutrophils but not with the total number of macrophages in the BAL cells. When rats were instilled with quartz and BAL cells were examined 15 months later, Driscoll, Deyo, et al. (Citation1997) reported the amount of oxidants per neutrophil was 2.5 times higher than the amount per macrophage. Taken together, these data support that, in dust-exposed rats with substantial and persistent lung neutrophilia, such as those observed in the TiO2 inhalation studies of Bermudez et al. (Citation2002, Citation2004), activated neutrophils are the major contributors to pulmonary oxidative stress and lung injury.
3.6. Molecular and genotoxic events in AECs – the roles of cytokines and neutrophils in particle-induced pathogenesis
3.6.1. Neutrophil-associated gene products IL-1β and TNFα were detected in LD-exposed rats
BALF samples collected from rats at 1 d, 1, 4, and 13 weeks after the 4-week LD inhalation exposure displayed concentration-dependent and post-exposure time-dependent increases in concentrations of IL-1β and TNFα (). Both TNFα and IL-1β are cytokines known to activate neutrophils to produce oxidants. Ferrante (Citation1992) noted, “TNFs are direct stimuli for the neutrophil respiratory burst and weak stimuli of lysosomal enzyme release. The TNFα augment the oxidative burst and lysosomal enzyme release…”
Figure 9. Rats were exposed to lunar dust by inhalation at 0, 2.1, 6.8, 20.8, or 60.6 mg/m3 for 4 weeks and the right lungs were lavaged thereafter at 1 d and 1, 4, and 13 weeks. BALFs were assessed for cytokines. (A) IL-1β levels. (B) TNF-α levels. Each data point contains the mean ± SD from five rats.
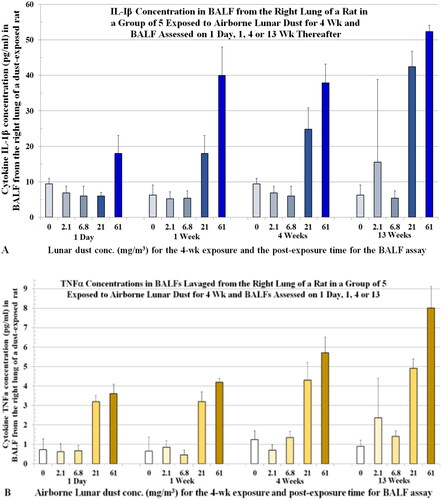
3.6.2. Gene expression in the lungs of rats exposed to quartz reveals the mechanism of particle-induced lung disease
In a toxicogenomic study conducted at NIOSH in which rats were exposed to crystalline silica for five days at 15 mg/m3, Sellamuthu et al. (Citation2013) reported that “Genes involved in oxidative stress, inflammation, respiratory diseases, cancer, and tissue remodeling and fibrosis were significantly differentially expressed in the rat lungs.” The authors concluded that studies of gene expression profiling and bioinformatics (toxicogenomics) on lung tissues of dust-exposed rats can reveal the initiation, progression, and mechanism of pathogenesis.
3.6.3. Lunar dust exposure induced AECs to upregulate the neutrophil chemokine gene CXCL2
Using standard toxicogenomic assays, the activation of proinflammatory and fibrotic genes was assessed in the lung tissues of the rats in the LD inhalation study. shows the dose- and time-dependent curves of the activation profiles of the CXCL2 gene (measured as increased gene product CXCL2 mRNA) in rat tissues after the AMs and other leukocytes were removed by lavage. The pattern of cytokine increases () is similar to that of CXCL2 genes (). shows the levels the oxidants from all neutrophils harvested from the lungs of rats exposed to each of the four LD concentrations for 4 weeks and assessed up to 13 weeks after the dust exposure. Note that the profiles for the CXCL2 gene and levels of oxidants are similar. These findings suggest that the recruitment and activation of neutrophils in the lung, resulting from the interaction between the dust particles and AECs, leads to expression of the CXCL2 gene. As noted above, BALF TNFα and IL-1β cytokines () similarly increased in a dose- and time-dependent manner. These findings suggest that AECs exposed to dust particles also up-regulate genes for neutrophil-associated cytokines TNFα and IL-1β.
Figure 10. Rats were exposed to lunar dust by inhalation at 0, 2.1, 6.8, 20.8, or 60.6 mg/m3 for 4 weeks and the right lungs were lavaged thereafter at 1 d and 1, 4, and 13 weeks. Post-lavage lung tissues were assessed for CXCL2 gene expression (A). Relative levels of ROS from all BALF neutrophils were estimated by a chemiluminescence assay (B). Each point represents the average data from five rats.
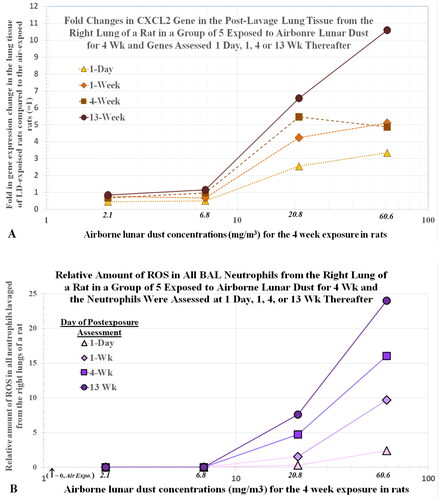
3.6.4. LD-induced activation of CXCL2 gene in the lung tissue and the function of CXCL2 chemokine
The CXCL2 gene (located on chromosome 4 in humans) is the template for biosynthesis of a cluster of CXC chemokines. CXCL2 RNA, which was detected in elevated levels in the LD inhalation study mentioned above, codes for the cytokine-induced neutrophil chemoattractant 3 (CINC-3, also known as MIP-2).
Neutrophils are known to cause bronchopulmonary dysplasia and lung injury in premature human babies (Lorant et al. Citation1999); these babies usually need hyperoxia therapy. Searches have long been conducted for drugs (such as inhibitors of IL-6 and CINC) that block neutrophil influx in the lung to alleviate the neutrophil-induced lung damage (Rudloff et al. Citation2017). Auten et al. (Citation2002) reported that neonatal rats that were exposed to 95% oxygen immediately after birth and assessed eight days later had neutrophil influx into the lung and showed impaired lung development and signs of oxidative DNA damage. When hyperoxia-exposed neonatal rats were treated with anti-CINC to block neutrophil recruitment on day 3 and day 4, a significant reduction in DNA strand breaks and nucleic acid oxidation in lung parenchymal cells was observed. The authors concluded that “neutrophil influx during hyperoxia damages DNA by nicking and oxidation, and that blocking neutrophil influx can prevent this. … Neutrophil-mediated oxidative DNA damage may contribute to abnormal lung development in newborns subjected to significant oxidative stress … neutrophil-generated ROS contribute significantly to nucleic acid damage in newborn rat lungs…” Similarly, Chang et al. (Citation2009) reported that rats exposed to 95% O2 at birth had lung injury when examined on day 14. The levels of TNF-α and IL-6 mRNA were elevated. Blocking these neutrophil-associated cytokines reduced the lung toxicity. Auten et al. (Citation2002) provide evidence that oxidants from neutrophils are genotoxic and can trigger oxidative DNA damage.
The activation of the lung gene of rats in the LD inhalation study () and the results of the neonatal rat studies suggest that high oxygen concentration in the air causes irritation or some damage to the AECs (likely to the cell membrane) triggering the AECs to activate the CXCL2 gene(s) to produce CINC cytokine(s), which recruit the neutrophils to the lung. The AECs also increase the production of IL-1β, IL-6, and TNFα, which can stimulate neutrophils’ oxidant production. Koto et al. (Citation1997) used anti-CINC to block neutrophil influx and lung toxicity in O3-exposed rats and reported that, “Ozone exposure [1 ppm for 3 h] induced a significant neutrophilia [O3: 16 × 104; air: 4.2 × 104] in BALF at 24 h after exposure; prior treatment with a goat anti-CINC antibody (1 mg, intravenously) suppressed the neutrophilia [3.1 × 104] …”
3.6.5. CXCL2 gene code for cytokine-induced neutrophil chemoattractant 3 – a functional homologue of the human cytokine IL-8 (CXCL8)
The CXCL2 cytokine is a functional homologue of the human CXCL8, which is also known as IL-8 or neutrophil chemotactic factor (Watanabe et al. Citation1991; Mitsuhashi et al. Citation1999). Beside recruiting neutrophils, IL-8 also activates neutrophils (Baggiolini et al. Citation1989). Human AECs released IL-8 when incubated with quartz, asbestos, amphiboles, or TiO2 (Rosenthal et al. Citation1994; Hetland et al. Citation2001; Monteiller et al. Citation2007). IL-8 is the cytokine primarily responsible recruiting neutrophils into lungs of cystic fibrosis (CF) patients (see discussion below). Jeon et al. (Citation2002) demonstrated that the in vitro migration of human neutrophils followed a directional concentration gradient of IL-8. It can be inferred that cytokines released by dust-exposed AECs will recruit neutrophils to the exact sites (sources of cytokine release) of the deposited particles or injury (dust-inflicted AECs), and the greater the exposure or injury, the more neutrophils will arrive.
3.6.6. Oxidants from neutrophils are genotoxic and can cause oxidative damage to DNA
In the aforementioned study of anti-CINC to block neutrophil recruitment (Auten et al. Citation2002) conducted by the Neonatal-Perinatal Research Institute of Duke University, neonatal rats exposed to 95% O2 for eight days had neutrophil influx and about fivefold more 8-OH-2-deoxyguanosine (OHdG, a marker for DNA oxidative damage) in their AECs (∼32% OHdG) than the air-exposed neonates (∼6% OHdG). When neutrophil influx into the lung was blocked by anti-CINC treatment on day 3 and day 4 after the hyperoxia exposure, this reduced the formation of OHdG to about 8%, which was close to the level of air-exposed neonatal rats. Bronchiolar epithelial cells in the same rats had similar levels of DNA oxidative damage as the AECs. The authors also measured DNA nicking or breaks in AECs using the TUNEL (terminal deoxynucleotidyl transferase dUTP nick end labeling) assay and determined that DNA breaks induced in AECs by exposures to 95% O2, 95% O2 + anti-C1NC, or air were about 1, 0.02, and 0.2 florescence units, respectively. Auten et al. (Citation2002) concluded that “… that blocking neutrophil influx significantly reduces DNA strand breaks and nucleic acid oxidation in lung parenchymal cells. We conclude that neutrophil-generated ROS contribute significantly to nucleic acid damage in newborn rat lung even during very high ambient oxygen.” These data show neutrophilic oxidant is not only cytotoxic, but also genotoxic.
3.6.7. Oxidants from alveolar neutrophils caused mutations in AECs in the lung of dust-exposed rats
The CXCL2 expression data from LD-exposed lung tissues presented herein, the data on neutrophil-induced lesions and oxidative DNA damage that can be blocked with anti-CINC in the lung of neonatal rats (Auten et al. Citation2002), and the results on oxidants from alveolar neutrophils induced mutations in AECs of dust-exposed rats (Driscoll, Deyo, et al. Citation1997) help elucidate the genomic/molecular mechanisms of pathogenesis of lung diseases including cancer in rats chronically exposed to high concentrations of dusts. illustrates the process of dust-inflicted AECs activating their proinflammatory genes (chiefly CXCL2) to produce chemokines (CINC chemokine) that recruit neutrophils and neutrophil-targeting cytokines (chiefly TNFα, IL-1β, and IL-6) that activate neutrophils, leading to lung disease.
Figure 11. Molecular and genomic mechanism of pathogenesis. Dust lands on the alveolar epithelial cells and triggers the cells to up-regulate the proinflammatory genes to produce chiefly CXCL2 chemokine (cytokine-induced neutrophil chemoattractant 2 (CINC-2)) and TNFα, IL-1β, IL6 cytokines. CINC chemokine recruits neutrophils while TNFα and IL-1β activate neutrophils to produce oxidants, leading to alveolar neutrophilia. Neutrophilic oxidants damage the lung and DNA. Persistent neutrophilia in chronically and heavily dust exposed rodents (particular the rats) could result in lung lesions including fibrosis, hyperplasia, metaplasia, and even cancer. OS: oxidative stress. Total amount of neutrophilic oxidants: ∑[Ox]Nu = [Ox]Nu × η.
![Figure 11. Molecular and genomic mechanism of pathogenesis. Dust lands on the alveolar epithelial cells and triggers the cells to up-regulate the proinflammatory genes to produce chiefly CXCL2 chemokine (cytokine-induced neutrophil chemoattractant 2 (CINC-2)) and TNFα, IL-1β, IL6 cytokines. CINC chemokine recruits neutrophils while TNFα and IL-1β activate neutrophils to produce oxidants, leading to alveolar neutrophilia. Neutrophilic oxidants damage the lung and DNA. Persistent neutrophilia in chronically and heavily dust exposed rodents (particular the rats) could result in lung lesions including fibrosis, hyperplasia, metaplasia, and even cancer. OS: oxidative stress. Total amount of neutrophilic oxidants: ∑[Ox]Nu = [Ox]Nu × η.](/cms/asset/e2b18eea-0611-47be-9189-3cd5d382469d/itxc_a_2258925_f0011_c.jpg)
3.7. Oxidant and proteolytic enzymes from dying short-lived neutrophils contributing to pathogenesis
3.7.1. Half-life of recruited neutrophils
Egger et al. (Citation1988) reported that, at the site of inflammation, rat neutrophils have a lifespan of 20 min during the intensive period when they are recruited to the lung and a lifespan of 5.5 h after the onset of inflammation. These authors also noted that “cell death and disintegration is evidently due to self-destructive peroxidation.” Generally, neutrophils have a half-life of several hours and a lifespan of 1–3 days in tissue (Egger et al. Citation1988; Robinson Citation1998; Knudsen et al. Citation2004; Summers et al. Citation2010). Scheel-Toellner et al. (Citation2004) proposed that accumulation of ROS is the primary factor limiting neutrophil life span. By comparison, the half-life of macrophages was reported by several groups to be 10–30 days (Fritsch and Masse Citation1992; Murphy et al. Citation2008).
3.7.2. The number of recruited alveolar neutrophils greatly outnumber the recruited AMs in rats exposed to very high concentration of dust
Rats exposed to 250 mg/m3 of TiO2 for 2 years developed lung tumors (Lee et al. Citation1985). Bermudez et al. (Citation2002) exposed rats to the same TiO2 concentration for 13 weeks and reported large influxes of neutrophils into their lungs. Based on the data reported by Bermudez et al. (Citation2002), it can be estimated the lung of a TiO2-exposed rat that had about 32 million BAL cells (100%) would have ∼26.5 million (83%) neutrophils and ∼5.5 million (∼17%) macrophages. Assuming that the average half-lives of neutrophils and macrophages are 5 h and 15 days (or 360 h), respectively, in a TiO2-exposed rat from this Bermudez study, roughly 2.75 million (i.e. half of the ∼5.5 million) macrophages would have died (or exited the lung) and would have been replaced by incoming macrophages every 15 days. In the same 15-d period, about 954 million neutrophils would also have died and been replenished (i.e. half of the ∼26.5 million (or ∼13.25 million) would have died and would have been replaced every 5 h (∼954 million neutrophils in 15 d (or 360 h)). Macrophages would become overburdened by the large amount of dust particles as well as dying neutrophils in the lung. To remove the dying neutrophils, each macrophage would have to phagocytize ∼400 neutrophils (972 million neutrophils ÷ 2.5 million macrophages = 389). The macrophages themselves might have been injured by toxic particles or overwhelmed by the presence of a large number of particles. Morrow (Citation1988) pointed out that phagocytosis decreases when the volume of phagocytized composite exceeds 6% of normal macrophage volume, and it completely ceases when the volume reaches 60%. These inflicted or particle-laden macrophages would not be effective in removing such a large number of dying neutrophils.
3.7.3. Unphagocytized apoptotic alveolar neutrophils undergo secondary necrosis releasing oxidants and other harmful cellular contents
The unphagocytized apoptotic neutrophils undergo secondary necrosis and release their oxidants and proteolytic enzymes, which would further damage local tissue (Robinson Citation1998; Liu et al. Citation2003; Rydell-Törmänen et al. Citation2006; Quint and Wedzicha Citation2007; Silva et al. Citation2008; Arandjelovic and Ravichandran Citation2015). Zhang et al. (Citation2002) pointed out that “… excessive apoptosis may overwhelm clearance processes and cause secondary necrosis of apoptotic bodies.” The particle-induced cellular events in the lungs of rats heavily and chronically exposed to PSPs leads to the prolonged release of oxidants ([Ox]Nu) from the neutrophils as they undergo necrosis and are replenished. The oxidants subsequently injure the AECs and damage their DNA (Auten et al. Citation2002), which could initiate hyperplastic and metaplastic reactions that could progress to metaplasia and cancer.
3.8. AMs and their role in dust-induced pulmonary pathology
If the dust burden in the lung is small, macrophages with engulfed particles migrate to the mucociliary apparatus or escalators and are transported out of the lung. However, if the lung is overburdened with particles, the dust exposure overwhelms the ability of macrophages to remove particles. Moreover, the particle-laden macrophages can also contribute to lung pathogenesis. These particle-overloaded macrophages are less mobile and therefore less likely to move out of the lung before dying. Some of the dead macrophages would be engulfed by incoming macrophages. Aggregates of dead macrophages would progress in the development of granulomas, as is typically seen in the lungs of rodents heavily exposed to dust and in the lungs of pneumoconiosis patients (Chong et al. Citation2006). These dead macrophages can also release their own harmful contents and ingested particles to the surrounding epithelium, damaging the epithelial cells and triggering release of more cytokines by afflicted AECs; the released cytokines would augment recruitment of inflammatory cells and promote proliferation of fibroblasts. Fibrosis, a common manifestation of chronic and heavy dust exposure, is a cellular defense process in which fibroblasts produce large amounts of collagen to wall off areas of inflammation and oxidative injury. Typically, granulomas are surrounded by fibrotic tissue. If dust particles and neutrophil recruitment persist in the lung, the granulomatous inflammation and fibrosis will progress with time, and the disease state could progress to massive fibrosis.
3.9. Progress of pathogenesis with time
A 12 mg/rat bolus dose of quartz instilled into male rats resulted in tumor incidence of 0% (after 6 months), 17% (after 11 months), 32% (after 17 months), and 86% (after 26 months) (Saffiotti Citation1992). These results illustrate that lesions progress with time, particularly in rats instilled with large doses of dust. NIOSH toxicity data () show that when rats were exposed to 15 mg/m3 quartz the number of neutrophils and amount of fibrosis increased with exposure duration (Castranova et al. Citation2002; Porter et al. Citation2004). Castranova et al. (Citation2002) reported a time-dependent increase of reactive nitrogen species (RNS: NOx) in BAL cells harvested from the exposed rats (). These increases continued even during post-exposure periods as shown in (Porter et al. Citation2004).
Figure 12. Rats were exposed to 15.3 mg/m3 quartz for up to 116 days. (A) number of neutrophils (value × 5 million/rat) and NOx (reactive nitrogen species) levels (treated minus control) in BALF and lung fibrosis increased with duration of dust exposure. Data courtesy of Castranova et al. (Citation2002). (B) BAL neutrophils (values in million) and fibrosis were assessed in a subset of the animals exposed for 20, 40, or 60 days, 36 days thereafter. Data courtesy of Porter et al. (Citation2004).
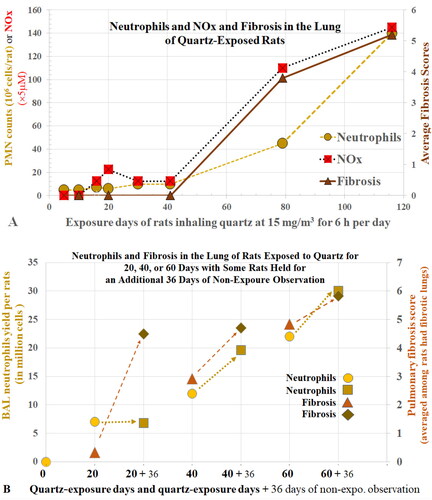
A 2-year exposure to 250 mg/m3 of TiO2 produced cancer in rats (Lee et al. Citation1985). When Bermudez et al. (Citation2002) exposed rats to the same TiO2 concentration for 13 weeks, 83% of the BAL cells were neutrophils; 65% of BAL cells were neutrophils even 1 year after the termination of TiO2 exposure. The small decrease in number of neutrophils was due to the decreased TiO2 lung burden. Rats exposed to a lower concentration of TiO2 had less neutrophils. Generally, the lesions correlated with the severity of alveolar neutrophilia. Levels of neutrophil oxidants increased with concentration and exposure time in rats exposed to airborne LD (). This pattern paralleled the histopathology and levels of some of the BALF toxicity biomarkers.
As mentioned above, Auten et al. (Citation2002) reported that neutrophils in hyperoxia-exposed neonatal rats caused lung lesions and oxidative DNA damage. Blocking neutrophil recruitment prevented lung lesions and oxidative DNA damage. All these data suggest that a persistent presence of neutrophils, or more accurately, neutrophilic oxidants (Σ[Ox]Nu = η × [Ox]Nu) or oxidative stress, is the driving force or underlaying factor of the disease progression and carcinogenesis. Saffiotti (Citation1992) reported neutrophil influx in the rats treated with crystalline silica study. Porter et al. (Citation2004) showed that once a threshold lung burden of silica is achieved (as in the Saffiotti study) the number of alveolar neutrophils will continue to increase with time after exposure. In addition, the oxidative DNA damage induced by neutrophils accumulates with time, and errors in DNA repair processes increase as animals get older, resulting in increased tumor incidence with time. This progressive disease pattern would not occur with exposure to low concentrations of low-toxicity dust that do not produce persistent alveolar neutrophilia.
3.10. Effects of dust particle size and role of alveolar neutrophils in dust-induced lung diseases
3.10.1. Difference in neutrophil counts in rats exposed to ns-TiO2 and µmTiO2
Rats chronically exposed to 10 mg/m3 of ns-TiO2 (ps 15–40 nm) (Heinrich et al. Citation1995) or to 250 mg/m3 of µm-TiO2 (pigmentary dust, ps < 1 µm) (Lee et al. Citation1985) developed tumors and severe lesions in the lung that were not observed in rats exposed to 50 or 10 mg/m3 µm-TiO2. Understanding how ns-TiO2 causes cancer in the lung whereas coarser pigmentary particles (µm-TiO2) at the same exposure concentration (10 mg/m3) did not produce cancer and lesions could reveal why nanoparticles are more toxic than the same mass concentration of micron-sized particles of the same material. Bermudez et al. (Citation2002, Citation2004) exposed rats to 10 mg/m3 of ns-TiO2 (ps 0.02 µm) or µm-TiO2 (ps < 1 µm) for 13 weeks and found that neutrophils accounted for ∼65% or ∼3% of BAL cells, respectively, at the end of the 13-week exposures. Rats exposed to ns-TiO2 had severe epithelial proliferative and metaplastic changes in their lungs (dust burden = 11 mg/g tissue), whereas rats exposed to µm-TiO2 had no lesions (dust burden in the lung = 7 mg/g tissue).
3.10.2. ns-TiO2 has more particles and a greater surface area than the same concentration of µmTiO2, which results in greater toxicity in the lung
Why does a 13-week exposure to ns-TiO2 dust particles give rise to so many neutrophils in the lung, whereas a 13-week exposure to the same concentration of µm-TiO2 dust does not, as shown in the study of Bermudez et al. (Citation2002, Citation2004)? Assuming the dust particles are spherical, for the same dust mass, a 10-fold decrease in particle diameter would result in a 1000-fold increase in the particle number and 100-fold increase in total particle surface area. Hypothetically, we can visualize the AECs that cover the alveolar surface as squares on a checkerboard and the dust particles as darts. Thus, many more ns particles (or darts) would impact many more squamous AECs (or hits on the checkerboard squares) than the same mass concentration of micron-sized particles. More particle hits would stimulate more AECs to synthesize and release chemokines to recruit more neutrophils (η). Oberdörster et al. (Citation2005) reported that the number of neutrophils in a rats’ lung was more closely correlated with the total particle surface area than the mass of the instilled dusts, i.e. the number of neutrophils increased in direct proportion to the total surface area contacted by the dust particles. Driscoll (Citation1996) showed that tumor incidence in dust-exposed rats correlated more closely with total particle surface area than with mass of the exposed dust ().
Figure 13. Lung tumor incidence in rats that were chronically exposed to poorly soluble dusts. Doses were estimated based on surface particle area (m2) of the dust that deposited in the lung of an exposed rat. Data are courtesy of Dr. Driscoll. Refer to original data (Driscoll Citation1996) for accuracy.
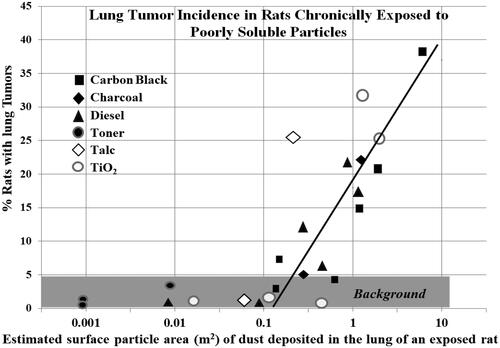
3.10.3. When more ACEs are involved, more cytokines are released, which recruit and activate more neutrophils, leading to greater oxidative stress in the lung
Crushing a particle just 10-time smaller in diameter will produce 1000 fine particles and greatly increase total surface area for particle–cell interaction, which would afflict many more ACE cells. The ns-TiO2 exposure afflicted more ACEs than the µm-TiO2 exposure, leading to greater amounts of cytokines being released in the lungs of ns-TiO2 exposed rats to recruit more neutrophils (η). A neutrophil in the lung of a ns-TiO2 exposed rat is subjected to more cytokines (such as TNFα or IL-1β) prompting it to produce more oxidants [Ox]Nu and proteolytic enzymes than a neutrophil in the lungs of a µm-TiO2 exposed rat. These events lead to greater oxidative stress in the rats exposed to ns-TiO2, i.e. greater total oxidants from all the neutrophils (Σ[Ox]Nu = η × [Ox]Nu) (). The greater level of chronic oxidative stress derived from persistently recruited neutrophils would explain why 2-year exposures to 10 mg/m3 ns-TiO2 rats resulted in a high incidence of cancer and severe lesions (Heinrich et al. Citation1995), whereas rats exposed to the same 10 mg/m3 µm-TiO2 had neither cancer nor lesions (Lee et al. Citation1985).
3.11. Animal species differences in toxicological responses between dust-exposed rats and hamsters
3.11.1. Macrophages are more effective in removing particles from the lungs of hamsters than rats
The hamsters used in toxicology studies were introduced from Syria. Their ancestors lived in arid or dry area burrowing their homes/holes in the desert (Miedel and Hankenson Citation2015). It has been speculated that the burrowing desert hamsters have evolved to be more capable than rats in handling dust particles in the lung (Creutzenberg et al. Citation1998). This would imply that macrophages of the desert-dwelling hamster are more capable or efficient than rat macrophages in clearing dust particles from the lung. Bermudez et al. (Citation2002) exposed hamsters and rats to the same concentrations of pigment-grade TiO2 (10, 50, and 250 mg/m3) for 13 weeks. At the end of the 13-week exposure, the lung burden (dust per gram dry lung tissue (g-dlt)) of the rats and hamsters exposed to 250 mg/m3 were 120 and 114 mg/g, respectively. However, 13 and 45 weeks after the exposure, the lung dust burden in rats were 98 and 95 mg/g, respectively, whereas the corresponding values in hamsters were 30 and 5 mg/g. For the 50 mg/m3 TiO2 exposure, dust burdens in the rats’ lungs 0, 13, and 52 weeks after the exposure were 46, 34, and 24 mg/g, respectively; the corresponding values for hamsters were 15, 1, and 0 mg/g (). These data indicate hamsters are more efficient in clearing particles from the lung and suggest exposure to the same concentration of dust induces sustained neutrophilic inflammation in rats leading to lung disease, but not in hamsters.
Figure 14. (A) TiO2 burden in the lung of rats and hamsters that were exposed to pigment TiO2 for 13 weeks. (B) Percentage of neutrophils in the BAL cells the lung of TiO2-exposed rats and hamsters. Each point is the average data from five animals. Black arrows indicate the Y-axis with the values for the curve. Data (no standard deviation) presented here are courtesy of Dr. Warheit and were replotted from data presented in Bermudez et al. (Citation2002).
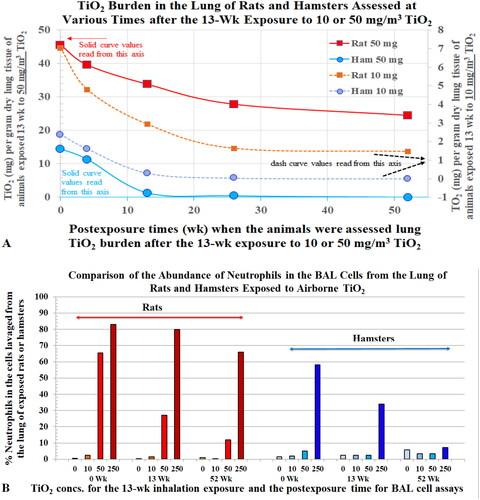
3.11.2. Hamster macrophages are more effective than rat macrophages in removing particles, resulting in less lung injury in the former
Saffiotti (Citation1992) conducted a species comparative carcinogenesis study by intratracheally instilling 20 mg of silica into each hamster and 12 mg into a rat. The hamsters were examined 12 months after the bolus instillation, and no cancer was found, whereas the 11 of the 37 rats had tumor. The author concluded that, in hamsters, “silica was extensively phagocytized by pulmonary macrophages (as in the other species), but very little if any evidence appeared of the toxic effect of silica on the macrophages… absence of macrophage necrosis, … No significant amount of fibrosis … The adjacent epithelium generally remained unaffected, and no significance of persistent epithelial hyperplasia was found.” The author stated that there were no lung tumors in the Fe2O3 treated rats. Fe2O3 was probably used as low-toxicity reference dust in the rat study. Neutrophilic inflammation was observed in the lung of the silica-exposed rats. Saffiotti (Citation1992) did not report seeing neutrophils in the lung of hamsters and stated “only a moderate lymphocytic reaction was occasionally seen, little progression occurred over a period of 12 months…. The adjacent epithelium generally remained unaffected.” The findings that the particles were extensively taken up by macrophages with apparently little to no neutrophil recruitment does not support the postulation that macrophages are the key lung cells that produce chemokines to recruit neutrophils.
3.11.3. Particle burden in the lung and the role of neutrophils in species-specific differences in pathogenesis
3.11.3.1. Rats exposed to very high concentrations of TiO2 had more alveolar neutrophils than hamsters and neutrophilia persisted in rats’ lungs after the termination of dust exposure
A 2-year exposure to 250 mg/m3 of TiO2 induced cancer in rats (Lee et al. Citation1985). Bermudez et al. (Citation2002) conducted an inhalation toxicity study in rats and hamsters using the same concentrations of this pigmentary TiO2 to investigate species-specific differences in dust-induced pathogenesis. The study showed that neutrophils accounted for ∼83% (31.8 × 106) and 58% (19 × 106) of the BAL cells in rats and hamsters, respectively, at the end of a 13-week exposure to 250 mg/m3 µm-TiO2. Neutrophils still accounted for about 65% of BAL cells in rats, relative to 5% in hamsters (control 3%), 1 year after the termination of dust exposure.
3.11.3.2. After exposure to the same concentration of TiO2, rats had more particles and neutrophils in their lungs than hamsters
Thirteen and 48 weeks after the 13-week subchronic exposure of 250 mg/m3 of TiO2, the TiO2 burden in hamsters’ lungs was ∼52 mg/g-dlt and ∼10 mg/g-dlt, respectively. The corresponding values for rats were ∼98 mg/g-dlt and ∼90 mg/g-dlt. One year after the termination of dust exposure, neutrophils still accounted for about 65% of rats’ BAL cells, relative to 5% in hamsters (control 3%) (). The severity of pulmonary lesions correlated with the particle burden and number of neutrophils in the lungs. After a 13-week exposure to 50 mg/m3 of TiO2, the TiO2 burden in the lungs of the hamsters and rats was 15 mg/g-dlt and 47 mg/g-dlt, respectively (), and the average number of BAL neutrophils in hamsters and rats were 6% and 66%, respectively () (Bermudez et al. Citation2002). These data show that after exposure to the same concentration of TiO2, hamsters had less TiO2 burden, less neutrophils, and less toxicity in their lungs than the rats. The data from Bermudez et al. (Citation2002) and Saffiotti (Citation1992), described in the preceding two sections, suggest that the desert-dwelling hamsters’ macrophages are more efficient than the rats’ macrophages in phagocytizing the dust particles, removing them from alveolar epithelium, and ridding them from the lung. Less TiO2 particles on hamsters’ AECs would trigger less chemokines being released to recruit neutrophils.
3.11.3.3. Rats had more neutrophils in their lungs than hamsters after exposure to the same concentration of CB
Elder et al. (Citation2005) exposed rats and hamsters to 50 mg/m3 of ns-CB for 13 weeks and found that neutrophils accounted for 48% and 15% of the BAL cells from these animals, respectively. The authors noted that hamsters exhibited much less toxicity than the rats. Carter and Driscoll (Citation2001) and Seiler et al. (Citation2001) also reported that rat lungs had more neutrophils than the hamster lungs after instillation with comparable doses of quartz, and the number of neutrophils correlated with pulmonary lesions.
3.11.4. Species-specific differences in oxidative contents of alveolar neutrophils
Carter and Driscoll (Citation2001) measured biomarkers of toxicity, including the number of neutrophils, and the level of ROS and RNS in BALF samples isolated from rats and hamsters seven days after the animals were instilled with 0.2, 2, or 20 mg of quartz (). From their data (), it can be calculated that the rat/hamster ratios of nitric oxide (an index of RNS) generated by 105 BAL cells were ∼3.0, ∼8.5, and ∼8.3, for the 0.2, 2, or 20 mg doses, respectively. The rat/hamster ratio of superoxide anions (an index of ROS) was ∼2 for all three quartz-dosed groups. As discussed above, Driscoll, Deyo, et al. (Citation1997) showed that, in ITI dust-exposed rats, alveolar neutrophils generated 2.5 times more oxidants than macrophages on a per cell basis. The difference in levels of RNS and ROS in the lungs of dust-exposed rats and hamsters could help explain the species differences in response to particulates.
Figure 15. (A) Comparison of toxicity biomarkers (neutrophil counts and LDH) and (B) oxidant production (superoxide anion, nitric oxide anion, and malonaldehyde) in BAL cells collected from rats and hamsters seven days after they were instilled with saline, or quartz (at 0.2, 2.0, or 20 mg). N = 4–6 animals. Data courtesy of Dr. Driscoll (Carter and Driscoll Citation2001).
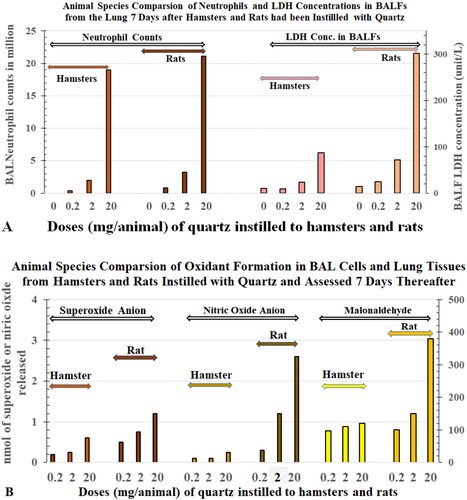
The higher levels of oxidants [Ox]Nu found in rats could partially explain why the BALF LDH concentrations (an index of pulmonary toxicity) () were higher in rats (302 U/L) than the hamsters (87 U/L) after the animals were instilled with 20 mg TiO2. The macrophages of rats and hamsters did not have time to clear the particles out of the lung during the seven days of observation after the acute bolus was instilled: therefore, the average number of BAL neutrophils (η) in the rats (34.8 million) was not substantially different from that of the hamsters (29.5 million). The overall results show that the toxicity depends on the number of neutrophils (η) as well as their oxidant content per cell ([Ox]Nu), that is, all the neutrophilic oxidants (∑[Ox]Nu × η).
The above studies (Bermudez et al. Citation2002; Elder et al. Citation2005) show that the same inhalation-exposure concentrations or same/comparable ITI doses of test dusts induced a greater influx of neutrophils (η) into the rat lung than the hamster lung, coupling with greater oxidant (ROS and RNS) production per neutrophil ([Ox]Nu) (Carter and Driscoll Citation2001). The products of these two neutrophilic biomarkers (η × [Ox]Nu), i.e. the total neutrophil-derived oxidative stress (=Σ[Ox]Nu = η × [Ox]Nu), could explain why, when rats and hamsters inhaled dust of micrometer sizes (such as quartz, talc, or diesel particles) or nano sizes (CB or TiO2) at the same concentrations and for the same durations or were ITI with comparable doses, the rats sustained more severe lesions and lung cancer (Saffiotti Citation1992; NTP Citation1993; Heinrich et al. Citation1995; Bermudez et al. Citation2002, Citation2004).
3.12. Neutrophilia induced by particle-inflicted AECs leads to lung pathogenesis in dust-exposed rats
In the 5-dust ITI lung biomarkers study (BAL cells assessed 1 and 4 weeks after the dust exposure) and 4-week LD inhalation study (BAL cells assessed one day and 1, 4, and 13 weeks after exposure), the oxidant production by BAL cells was measured. The data of both studies, reported here, show time-dependent increases in [Ox]Nu and Σ[Ox]Nu ( and ). At the onset of dust exposure and before the inflammatory responses are substantial, the insults to AECs are produced predominately by dust particles. The macrophages, the antioxidants, and protective enzymes keep the particle-induced toxicity in check and prevent it from going beyond a threshold level. The macrophages are capable of phagocytizing dust particles and apoptotic neutrophils as part of the defense process. Even if macrophages fail to phagocytize some dying neutrophils, which results in oxidant release, the low levels of neutrophilic oxidants are neutralized or destroyed by the antioxidants.
With prolonged dust exposures or greater concentrations of dust, particles on the alveolar surface exceed the amount that can be removed by macrophages. When the threshold has been exceeded, more particles interact with the AECs resulting in greater release of cytokines to recruit and activate neutrophils, which produce excessive oxidants that overwhelm the antioxidant defense. The unphagocytized dead neutrophils then release oxidants, proteolytic enzymes, and other inflammatory products, adding further injury to AECs. The particle-inflicted damage to AECs and the cytokines released by AECs are amplified by the demise of recruited short-lived neutrophils.
As exposure progresses, necrotic neutrophils add yet another insult to the lung epithelium, which is already overburdened by the dust particles. This further amplifies release of cytokines from AECs. This release of more cytokines leads to recruitment of more inflammatory cells in the lungs of rats heavily exposed to dust. Incoming neutrophils are activated by increased levels of cytokines to produce more oxidants. Thus, the product (Σ[Ox]Nu) = η × [Ox]Nu) increases with exposure concentration and exposure duration. Driscoll, Deyo, et al. (Citation1997) reported that BAL cells harvested from rats 15 months after they were instilled with quartz, CB, or TiO2 showed dose- and dust-dependent increases in the number of neutrophils (η) and oxidants per neutrophil (i.e. Qz-[Ox]Nu > CB-[Ox]Nu > TiO2-[Ox]Nu). In other words, the lungs of quartz instilled rats had the highest total neutrophil-derived oxidative stress (=Σ[Ox]Nu = η × [Ox]Nu) and TiO2 had the least. During persistent particle-induced inflammation, continuous release of oxidants from neutrophils could lead to excessive cell damage resulting in lung lesions, mutagenesis, and carcinogenesis, as shown in the rats () (Driscoll Citation1996; Driscoll, Deyo, et al. Citation1997).
3.13. Thresholds and non-linear dose responses in toxicity – relationship with neutrophilic oxidants
As discussed above, dust-induced lesions in the lungs can be attributed mainly to the oxidative stress (or Σ[Ox]Nu) derived from neutrophils. The profiles of Σ[Ox]Nu in the lungs of rats inhaling LD for 4 weeks and assessed 1 d, 1 week, 4 weeks, and 13 weeks thereafter are shown in . When the Σ[Ox]Nu levels shown in were plotted against the LD exposure concentrations on a logarithmic scale (like Driscoll’s cancer incidence curve; , Driscoll Citation1996), we obtained a hockey stick like curve with a threshold (). identifies that the point of departure or threshold for Σ[Ox]Nu occurs in the region between 4 and 7 mg/m3. Histopathological and biomarker assessments of the LD inhalation identified 6.8 mg/m3 as the NOAEL or threshold, which was used for establishing a NASA LD exposure limit (Lam et al. Citation2013). and also show increases in Σ[Ox]Nu with time and duration of exposure to the two LD concentrations (20.8 and 60.6 mg/m3) that produced lung lesions, which progressed over time after the 4-week inhalation exposure (Lam et al. Citation2013).
It is well established that mutations can lead to cancer. When Driscoll, Deyo, et al. (Citation1997) plotted mf induced in AECs against the percentage of neutrophils in BAL cells, they obtained a hockey stick like curve (). Because alveolar neutrophils in the high-dose group were more oxidatively mutagenic per million cells (i.e. produced more oxidants per cell) than neutrophils in the low-dose group, the hockey stick like curve () of mf vs. neutrophil percentage or number (η) in BALF did, in fact, reflect mf vs. the total oxidants produced by the neutrophils (i.e. η × [Ox]Nu or Σ[Ox]Nu). Driscoll, Deyo, et al. (Citation1997) concluded that the non-linear relationship between mf and inflammatory cells, particularly neutrophils, is concordant with the relationship between tumorigenic responses and particle-induced inflammation in the rat lungs.
The NIOSH rat silica studies discussed above also showed that increases in RNS, number of neutrophils, and fibrosis with the duration of exposure illustrate the hockey stick like curves with thresholds in response () (Castranova et al. Citation2002; Porter et al. Citation2004). Morrow et al. (Citation1996) noted that in chronic and heavy dust-exposed rats, neutrophil inflammation, and fibrosis occur before tumorigenesis. Driscoll (Citation1996) reported that the dose–response curve of the combined tumor incidences in rats that chronically inhaled several relatively inert PSPs (CB, µm-TiO2, diesel, talc, and toner) was also nonlinear with a hockey stick like shape that was “consistent with the existence of a threshold” ().
The reason why the shape of toxicity curves () like a hockey stick with a threshold is that the toxicological outcomes depend not only on neutrophil count (η) but also the oxidant per neutrophil [Ox]Nu. The product of these two neutrophil biomarkers (Σ[Ox]Nu = η × [Ox]Nu) represents the total neutrophilic oxidants or oxidative stress in the lung
3.14. Risk assessment of dust exposures in humans, and neutrophils in human lung diseases
3.14.1. Alveolar neutrophilia is commonly seen in human lung diseases
Alveolar neutrophils play a pivotal role in pathogenesis and carcinogenesis in dust-exposed rats. Humans also have increased numbers of neutrophils in their lungs after exposure to airborne oxidative pollutants or PM, and neutrophil infiltration is associated with human lung diseases. In a study of patients with chronic obstructive pulmonary diseases, Quint and Wedzicha (Citation2007) stated, “… If neutrophils are not cleared efficiently by apoptosis … impaired phagocytosis leads to decreased neutrophil clearance and increased secondary necrosis.” This statement is similar to a comment made by Warheit and colleagues that apoptotic cells progressed into necrotic cells in the lung of dust-exposed rats (Zhang et al. Citation2002).
Strzyz (Citation2017) noted, “apoptosis can progress to secondary necrosis if apoptotic cells are not efficiently removed by phagocytic cells.” The harmful effects of necrotic cells have been pointed out by Arandjelovic and Ravichandran (Citation2015) that “Failures in clearing apoptotic cells at early stages of death and their progression to a secondary necrotic state can induce tissue inflammation due to the release of cellular contents or exposure of otherwise sequestered intracellular moieties.” With respect to the harmful effects of neutrophils, Iba et al. (Citation2013) summed up and stated, “Neutrophil necrosis is probably one of the major causes of tissue damage during infection.” In a review article on the role of neutrophils in common human respiratory diseases, Liu et al. (Citation2017) concluded that “there are still many unanswered questions on the role of neutrophils in lung cancer, pulmonary fibrosis, cystic fibrosis, bronchitis, and bronchiolitis.” It is noteworthy that many human lung diseases are caused by exposure to airborne pollutants and are associated most consistently with PM (Dockery et al. Citation1993; Pope et al. Citation2002; Krewski et al. Citation2003; Laden Citation2019). The results of our studies of dusts described here may help delineate how neutrophils promote or cause these human lung diseases.
3.14.2. Patients who have idiopathic progressive pulmonary fibrosis also have pulmonary neutrophilia and a high incidence of cancer
A study of 18 patients with idiopathic progressive pulmonary fibrosis (IPF) showed that neutrophils accounted for 0.2–42.6% (mean 9.8%) of their total BAL cells, fibrosis worsened in patients who had higher percentages of neutrophils (>10%) and/or eosinophils (>5%) (Haslam et al. Citation1980). The results of an epidemiology study in United Kingdom showing a fivefold increase in lung cancer in IPF patients compared with the general public led the authors (Le Jeune et al. Citation2007) to conclude that, “This study provides further evidence for a marked increase in the incidence of lung cancer in people with IPF.”
3.14.3. Patients with cystic fibrosis also have pulmonary neutrophilia and a high incidence of cancer
Cystic fibrosis is a genetic, chronic disease characterized by increasing airway obstruction and neutrophilic inflammation. Because the AECs in the lungs of CF patients contain mutant genes, these abnormal lung parenchymal cells release sustained, elevated levels of proinflammatory cytokines (most notably IL-8) that recruit an abundance of neutrophils (Eidelman et al. Citation2001; Bodas and Vij Citation2010). Infants with CF who had no infections had elevated levels of IL-8 and neutrophils in their BALF samples (Khan et al. Citation1995). As noted in a review of CF by Jacquot et al. (Citation2008), “Activated neutrophils present in airways produce large amounts of proteases and reactive oxygen species (ROS).” The massive neutrophil influx results in an increased release of oxidants and proteolytic enzymes that eventually lead to fibrosis (Eidelman et al. Citation2001; Hector et al. Citation2014). Rousset-Jablonski et al. (Citation2022) reported that the lung cancer incidence in persons with CF (including those with lung transplants) was 40-fold (fourfold without lung transplants) higher than the French general public in the years of 2006–2017. The persistently high neutrophil influx contributes to ROS and elevated proteases in the lung that lead to progressive fibrosis in IPF patients and CF patients that eventually lead to cancer. This is similar to neutrophil-mediated pathogenesis and fibrosis in rats that are heavily exposed to dusts.
3.15. The mechanisms of pathogenesis of lung disease in humans and rats are similar
In a review article, Wynn (Citation2011) of NIH’s National Institute of Allergy and Infectious Diseases, noted that the initial involvement of proinflammatory cytokines IL-1β, TNFα, CXC chemokines, and TGF-β1 led to the recruitment of neutrophils that can quickly evolve to a progressive fibrosis. The author further stated, “recruitment of neutrophils to the BAL is an important predictor of early mortality in IPF patients.” As noted above, IPF patients and CF patients have a high incidence of fibrosis and cancer.
With respect to the disease process in rats that were chronically exposed to PSP, Morrow et al. (Citation1996) pointed out tumors were observed only at exposure levels that resulted in a pronounced (neutrophilic) inflammatory and fibrotic response in the lung. Apparently, similar mechanisms of pathogenesis of lung disease in humans and rats occur if prolonged insults to the lung lead to severe pulmonary neutrophilia that progresses to fibrosis and eventually cancer. It is noteworthy that the mechanisms that occur in rats that are chronically exposed to high concentrations of a low-toxicity PSP that overwhelm the lung’s dust clearing ability and antioxidant capacity would not operate in humans or rats that were sporadically exposed to low concentrations of PSP that do not trigger excessive neutrophil influx in the lung.
3.16. Risk assessment of dust exposure in humans – choice of suitable animal model in dust studies
Humans are exposed to PM that includes combustion particulates in the environment and occupational exposure to dusts such as CB, talc, silica, TiO2, and other PSPs. Bermudez et al. (Citation2002) noted, “Findings of fibrosis, bronchoalveolar hyperplasia, metaplasia, and pulmonary tumorigenesis following exposures to high doses of PSP for long periods of time appear to be unique to the rat relative to other rodent species.” Mauderly (Citation1997) pointed out that rats are more sensitive to particle-induced carcinogenesis than Syrian hamsters, and thus the use of hamsters for carcinogenicity assays could yield “false negatives”. On the other hand, Borm et al. (Citation2004) pointed out that “the rat is a particularly sensitive species to oxidative injury, whereas the hamster has been suggested to be more like humans in its antioxidant defense and oxidant generation…”. Saffiotti (Citation1992) postulated that “each of these animal models may represent a subset of the human population, with subjects at different levels of susceptibility to either pneumoconiotic disease or lung cancer.” In a recent commentary article, containing the pooled opinions of 27 environmental health experts, Borm and Driscoll (Citation2019) reported no consensus regarding the applicability of using rats exposed to high doses of PSP to assess human risk. Subsequently, a workshop was held in Edinburgh, Scotland in 2019 to address the topic of PSP, rat lung cancer, and human risk (Driscoll and Borm Citation2020), and the consensus of the experts was “rat lung cancer occurring only under conditions of lung particle overload does not imply a cancer hazard for humans under non-overloading conditions”.
The overall results of dust studies by Bermudez et al. (Citation2002, Citation2004) (TiO2), Elder et al. (Citation2005) (CB), Driscoll, Deyo, et al. (Citation1997) (TiO2, CB, Qz), Carter and Driscoll (Citation2001), and Seiler et al. (Citation2001) show that after exposure to comparable doses of ITI dusts or inhalation exposure to the same concentrations of a test dust, neutrophil infiltration (η) in the lung was more severe in rats than in hamsters. Carter and Driscoll (Citation2001) showed that when these species were exposed to the same concentrations of quartz, the oxidant levels (ROS and RNS) in the same number of neutrophils were 2–8.5 times higher in rats that in hamsters. As pointed out above, the oxidative stress in the lung is mainly attributed to neutrophils and therefore, can roughly be expressed as oxidative stress = Σ[Ox]Nu = [Ox]Nu × η. These findings indicate that values of [Ox]Nu and numbers of neutrophils (η) are higher in rats than hamsters exposed to the same dust concentration. Thus, the greater oxidative stress or Σ[Ox]Nu in the rat lung would explain why rats are more sensitive than hamsters exposed to the same concentration of dust, because the number of neutrophils and their oxidant levels in the lungs appear to dictate the course of pathogenesis.
Perhaps Syrian golden hamsters, which have been living in the dusty desert for millions of years, have evolutionarily benefited from more macrophages than rats or a greater ability of these macrophages to remove particles from their lungs () (Bermudez et al. Citation2002). Alternatively, the AECs of the desert burrowers may be more accustomed to or tolerant to inhaled PM and synthesize less chemokines to recruit fewer neutrophils. If indeed these postulated adaptations to a dusty environment have occurred (Creutzenberg et al. Citation1998), this may be a basis for asserting that hamsters are a less suitable model for assessing risk to humans from dust exposures.
3.17. Risk to humans from exposure to low concentrations of dust extrapolated using data from animals exposed to high concentrations of dust
When animals are chronically exposed to high concentrations of low-cytotoxicity PSPs, the large number of inflicted AECs will constantly release elevated levels of cytokines to recruit and activate a substantial number of neutrophils. Above a threshold, prolonged inflammation, oxidant stress, and damage progress to pathogenesis. Although less AECs are affected during exposures to lower concentrations of more cytotoxic dusts (such as quartz), the injury and intensity of the response of the individual AECs will be greater; subsequently a greater release of cytokines leads to recruitment and activation of a large number of neutrophils at lower doses. If the induced response is above the threshold for inflammation and oxidant stress, it may progress to lung injury, epithelial hyperplasia, fibrosis, and, under extreme conditions, cancer. However, when animals are exposed to low concentrations of low-cytotoxicity dusts, or a very low concentration of highly toxic dust, macrophages can phagocytize the relatively low numbers of particles and the neutrophils as they undergo apoptosis, resulting in a level of inflammation and oxidant stress below a threshold, allowing repair and recovery. The dust-induced cancer data compiled by Driscoll (Citation1996) and the Σ[Ox]Nu data presented here () show dose–response curves consistent with the existence of a threshold (a hockey stick like dose–response curve) indicating low-dose dust exposures produced little or no injury. Exposures for prolonged periods to high or toxic levels of dusts can produce severe and persistent pulmonary inflammation characterized by high neutrophil influx leading to severe lesions (including cancer). As was the conclusion at the 2019 Edinburgh Workshop on the hazards and risks of poorly soluble low-toxicity particles, such animal data would very likely over-predict the risks to humans exposed to these dusts at much lower concentrations and non-overloading levels (Driscoll and Borm Citation2020; Driscoll Citation2022). Results of studies on PSPs using bolus high ITI doses that overwhelm dust clearance and produce persistently high neutrophil inflammation have little value for human risk assessment. Exposure limits on dusts and other airborne PM should set at levels that avoid overwhelming the capacity of macrophages to phagocytize and clear dust particles (Morrow Citation1988), thus, preventing neutrophil-derived oxidants and proteases from reaching levels exceeding the lung’s defense capacity.
4. Conclusions
4.1. A proposed pathogenic mechanism associated with particle-induced lung injury
Upon review and assessment of the results of the LD and numerous other studies, we propose a general mechanism by which particles cause pathological changes in the lung. The genomic and molecular events induced by particle exposures are presented in . The mechanism of pathogenesis of lung diseases is depicted in . After entering the lung, respirable dust particles build up on the alveolar epithelium. The unphagocytized particles stimulate or interact with AECs (e.g. binding to scavenger receptors); toxic particles such as quartz, can cause irritation or damage to the cells in situ. The inflicted AECs, through signaling cascades, augment their gene expression machinery to increase cytokine production, as detected in the lung tissues of LD-treated rats (). Chemokines released by AECs recruit inflammatory cells (AMs and neutrophils) to the locations of injury, where they further contribute to the inflammatory response. Some cytokines (such as those detected in BALF; ) activate the recruited cells to increase oxidant () and proteolytic enzyme production. The greater the cytotoxicity of the particles and the higher the concentration of dust, the more AECs are afflicted, the more neutrophils are recruited, and the more oxidant stress and damage are incurred. With continued exposures, the cycle continues. Each incoming neutrophil is subjected to collectively greater cytokine activation signals in the lung to produce more oxidants and proteases.
Figure 16. Diagram illustrating a proposed mechanism of particle-induced pathogenesis and carcinogenesis in the lungs that is mediated by epithelial cells and neutrophils. Sequence of events leading to dust-induced pathogenesis: (1) particles enter the alveoli and deposit on alveolar epithelial cells (AECs, light blue), causing some irritation or injury. (2) The dust-inflicted AECs turn on gene processes that produce elevated levels of cytokines, which recruit blood leukocytes (chiefly monocytes (brown





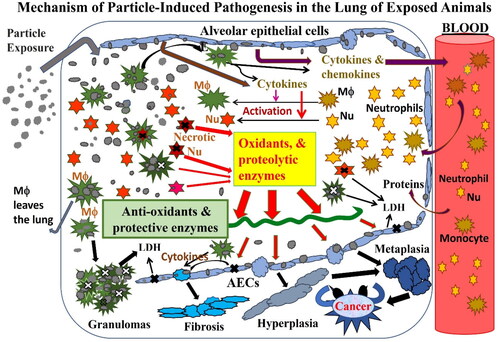
Neutrophils are short-lived, and dead neutrophils would normally be phagocytized by macrophages. When the dust particles in the lung are below a threshold level, macrophages can remove them, and antioxidants effectively protect the lung. Above a threshold lung burden, macrophages can no longer clear all particles and the dead neutrophils. The release of harmful products from dead neutrophils together with production of oxidants from newly recruited neutrophils overwhelm the antioxidants and the protective enzymes in the lung, leading to lung damage (). When large numbers of particle-laden macrophages are unable to leave the lungs, they cluster to form granulomas or giant cell aggregates. The live macrophages release cytokines while dead macrophages release oxidants and destructive cellular contents, causing localized damage and recruitment of more neutrophils. Therefore, above a dust burden threshold, a cycle of AEC insult and inflammatory cell recruitment and oxidant production cause proliferation and activation of fibroblasts, leading to an increase in collagen production to wall off local injury. The significant and persistent increase of connective tissue manifests as fibrosis. The damage or death of type I AECs leads to regenerative proliferation and hyperplasia of type II AECs, and in severe cases, type II AEC metaplasia and eventually cancer. Driscoll, Deyo, et al. (Citation1997) showed dose-dependent and dust-dependent increases in the number of alveolar neutrophils (η) and oxidants (per cell, [Ox]Nu) in neutrophils harvested from dust-exposed rats. The oxidants released from the neutrophils in situ induced mutations in the type II AECs of these rats (). It is well known that mutations can lead to cancer (Driscoll Citation1996).
Thus, the extent of insult to AECs and the magnitude and persistence of neutrophilic recruitment and subsequent oxidative stress collectively reflect the toxic properties of the dust and the exposure dosimetry (the magnitude of the dose or concentration and the duration of exposure). Toxic quartz reaches a threshold at a relatively low lung burden, whereas the threshold for a low-toxicity dust, such as TiO2, is reached at a higher lung burden. Species-specific responses to a given dust in the lung reflect different degrees of particle-induced neutrophil recruitment and oxidant generation, which result in different susceptibility to pneumoconiosis. Furthermore, these concerted responses of AECs and neutrophils illustrate why nanoparticles, by virtue of greater number and greater surface area per unit mass, are generally more toxic than micrometer-sized particles in the lungs, and how quartz produces more lesions than TiO2. As neutrophils die, they cause more damage to AECs that, in turn, causes the AECs to recruit more neutrophils. This cycle of AEC damage, neutrophilic recruitment, oxidant production, and injury explain why dust-related human fibrotic lung diseases (such as silicosis or progressive fibrosis) sometimes worsen or progress with time even after the dust exposure ended (Seaton Citation1983). The primary mechanism that interrupts the disease process is the elimination of dust particles by dissolution and by macrophages. Antioxidants and protective enzymes reduce the harmful effects of neutrophilic products. However, high burdens of low-toxicity dust particles or low burdens of high-toxicity dusts induce activation of cytokines from AECs, neutrophil recruitment, and release of oxidants by these recruited neutrophils, which overwhelms antioxidant defenses. The persistent oxidative tissue damage leads to lung disease.
Abbreviations | ||
AEC | = | alveolar epithelial cell |
AMs | = | alveolar macrophages |
AOP | = | adverse outcome pathway |
BALF | = | bronchoalveolar lavage fluid |
CARB | = | California Air Resources Board |
CB or BC | = | carbon black |
CF | = | cystic fibrosis |
CINC | = | cytokine-induced neutrophil chemoattractant |
CL | = | chemiluminescence |
DE | = | diesel exhaust |
DPM | = | diesel particulate matter |
IARC | = | International Agency for Research on Cancer |
IPF | = | idiopathic progressive pulmonary fibrosis |
ITI | = | intratracheally instilled |
JSC | = | Johnson Space Center |
LD | = | lunar dust |
LD-ug | = | lunar dust, unground, respirable size |
LD-bm | = | lunar dust, zirconia ball-milled, respirable size |
LD-jm | = | lunar dust, jet-milled, respirable size |
mf | = | mutation frequency |
MMD | = | mass median diameter |
NASA | = | National Aeronautics and Space Administration |
NIOSH | = | National Institute for Occupational Safety and Health |
NOAEL | = | no-observable-adverse-effect-level |
ns | = | nano-sized |
NTP | = | National Toxicology Program |
•OH | = | hydroxyl radicals |
PM | = | particulate matter |
PMN | = | polymorphonuclear leukocytes or neutrophils |
PSP | = | poorly soluble particles |
ps | = | primary size |
psROS | = | particle surface reactive oxygen species |
Qz | = | quartz |
Qz-jm | = | quartz ground by jet mill |
RNS | = | reactive nitrogen species |
ROS | = | reactive oxygen species |
RT-PCR | = | real-time polymerase chain reaction |
TNFα | = | tumor necrosis factor alpha |
WHO | = | World Health Organization |
(η) | = | number of neutrophils in BALF or the whole lung recovered from a rat |
[Ox]BC | = | oxidants in a BAL cell estimated by chemiluminescence |
[Ox]Mϕ | = | oxidants in an alveolar macrophage |
[Ox]Nu | = | oxidants in an alveolar neutrophil |
Σ[Ox]Nu | = | [Ox]Nu × η, all the oxidants in the neutrophils in BALF or the whole lung |
Acknowledgements
The LD toxicity studies were funded by the NASA Human Research Program Grant through NASA’s JSC and Wyle Bioastronautics Contract #NAS9-02078. This project was funded by the NASA Human Research Program. Under the NASA-NIOSH government interagency arrangement/agreement, the NIOSH provided (without compensation) scientific staff and technological expertise to conduct the LD intratracheal instillation studies in rats, the participation of the principal investigator of the NASA LD studies, at the NIOSH facility. The results of CL (oxidant) assay of the particle-recruited neutrophils in the lungs of exposed rats, are a central pillar of the present paper. The oxidant assay was only one of several toxicological biomarkers assessed in NIOSH Health Effects Laboratory Division under the supervision of Dr. Vincent Castranova, who retired from NIOSH in 2014. Dr. Castranova has contributed greatly to the science specifically the neutrophil-centered mechanism of toxicity, and preparation of the current manuscript as an independent scientist. Overall, the present LD studies and other NASA LD toxicological studies were overseen and reviewed by the NASA-assembled Lunar Airborne Dust Toxicity Assessment Group and Non-Advocate Review Committee consisting of national experts in toxicology, environmental sciences, and lunar geology. The authors gratefully acknowledge the NASA Astromaterial Samples Curator for providing an Apollo-14 lunar regolith sample, from which the NASA lunar geology team isolated the respirable LDs for our animal toxicity studies. The authors thank the members of the Lunar Airborne Dust Toxicity Assessment Group and Non-Advocate Review Committee for their advice and technical support on the LD toxicity project. Technical assistance from S. Bassett, C. Gonzalez, and staff of the NIOSH Health Effects Laboratory Division (Morgantown, WV), NASA-JSC Clinical Laboratory, and Histology Laboratory of the University of Texas Medical Center (Houston) is also gratefully acknowledged. The authors also thank K. George, C. Tapia (KBR), and H. Garcia (formerly KBR/JSC Toxicology Group) for editorial review and assistance. May-Ying Lam is acknowledged for contributing graphic artwork. The author gratefully acknowledges the review comments provided by Dr. Cohen and the external reviewers who were anonymous to the authors.
Declaration of interest
This project was funded by the NASA Human Research Program. The authors participated in the development of the paper as individual professionals and have sole responsibility for the writing and content of the paper. None of the authors have been involved in the last 5 years with regulatory or legal proceedings related to the contents of the paper. The findings and conclusions in this report are those of the authors and do not necessarily represent the views of the NASA, NIOSH, the University of Texas Health Science Center at Houston or any other entities. No potential conflict of interest was reported by the author(s). SPECIAL DECLARATION OF INTERESTS DISCLOSURE STATEMENT by the Editor-in-Chief of CRT, Dr. Roger O. McClellan, was a member of the NASA-assembled Lunar Airborne Toxicity Assessment Group advising on the experimental design and the conduct of the LD toxicity studies in rodents. He is also a contributing coauthor of this paper. In the interest of transparency and to avoid any conflicts of interest, he recused himself from the review process for this paper following its submission to CRT for publication consideration. The review process for this paper was assigned to a member of the Journal’s Editorial Advisory Board, Samuel Cohen PhD, MD who is knowledgeable of the toxicology of respiratory toxicants and respiratory disease processes pathology. The publication fees and cost of open access for this article were paid by NASA.
References
- Albrecht C, Knaapen AM, Becker A, Höhr D, Haberzettl P, van Schooten FJ, Borm PJ, Schins RP. 2005. The crucial role of particle surface reactivity in respirable quartz-induced reactive oxygen/nitrogen species formation and APE/Ref-1 induction in rat lung. Respir Res. 6(1):129–137. doi: 10.1186/1465-9921-6-129.
- Allen RC. 1977. Evaluation of serum opsonic capacity by quantitating the initial chemiluminescent response from phagocytizing polymorphonuclear leukocytes. Infect Immun. 15(3):828–833. doi: 10.1128/iai.15.3.828-833.1977.
- Alon R, Sportiello M, Kozlovski S, Kumar A, Reilly EC, Zarbock A, Garbi N, Topham DJ. 2021. Leukocyte trafficking to the lungs and beyond: lessons from influenza for COVID-19. Nat Rev Immunol. 21(1):49–64. doi: 10.1038/s41577-020-00470-2.
- Amdur MO, Chen LC. 1989. Furnace-generated acid aerosols: speciation and pulmonary effects. Environ Health Perspect. 79:147–150. doi: 10.1289/ehp.8979147.
- Antonini JM, Van Dyke K, Ye Z, DiMatteo M, Reasor MJ. 1994. Introduction of luminol-dependent chemiluminescence as a method to study silica inflammation in the tissue and phagocytic cells of rat lung. Environ Health Perspect. 102(Suppl. 10):37–42. doi: 10.1289/ehp.94102s1037.
- Arandjelovic S, Ravichandran KS. 2015. Phagocytosis of apoptotic cells in homeostasis. Nat Immunol. 16(9):907–917. doi: 10.1038/ni.3253.
- Aris RM, Christian D, Hearne PQ, Kerr K, Finkbeiner WE, Balmes JR. 1993. Ozone-induced airway inflammation in human subjects as determined by airway lavage and biopsy. Am Rev Respir Dis. 148(5):1363–1372. doi: 10.1164/ajrccm/148.5.1363.
- Auten RL, Whorton MH, Mason NS. 2002. Blocking neutrophil influx reduces DNA damage in hyperoxia-exposed newborn rat lung. Am J Respir Cell Mol Biol. 26(4):391–397. doi: 10.1165/ajrcmb.26.4.4708.
- Baggiolini M, Walz A, Kunkel SL. 1989. Neutrophil-activating peptide-1/interleukin 8, a novel cytokine that activates neutrophils. J Clin Invest. 84(4):1045–1049. doi: 10.1172/JCI114265.
- Bello-Irizarry SN, Wang J, Olsen K, Gigliotti F, Wright TW. 2012. The alveolar epithelial cell chemokine response to pneumocystis requires adaptor molecule MyD88 and interleukin-1 receptor but not toll-like receptor 2 or 4. Infect Immun. 80(11):3912–3920. doi: 10.1128/IAI.00708-12.
- Bermudez E, Mangum JB, Asgharian B, Wong BA, Reverdy EE, Janszen DB, Hext PM, Warheit DB, Everitt JI. 2002. Long-term pulmonary responses of three laboratory rodent species to subchronic inhalation of pigmentary titanium dioxide particles. Toxicol Sci. 70(1):86–97. doi: 10.1093/toxsci/70.1.86.
- Bermudez E, Mangum JB, Wong BA, Asgharian B, Hext PM, Warheit DB, Everitt JI. 2004. Pulmonary responses of rats, mice, and hamsters to subchronic inhalation of ultrafine titanium dioxide particles. Toxicol Sci. 77(2):347–357. doi: 10.1093/toxsci/kfh019.
- Bodas M, Vij N. 2010. The NF-kappaB signaling in cystic fibrosis lung disease: pathophysiology and therapeutic potential. Discov Med. 9(47):346–356.
- Borm PJ, Driscoll KE. 2019. The hazards and risks of inhaled poorly soluble particles—where do we stand after 30 years of research? Part Fibre Toxicol. 16(1):11. doi: 10.1186/s12989-019-0294-4.
- Borm PJ, Schins RP, Albrecht C. 2004. Inhaled particles and lung cancer, Part B: paradigms and risk assessment. Int J Cancer. 110(1):3–14. doi: 10.1002/ijc.20064.
- Bosson J, Pourazar J, Forsberg B, Adelroth E, Sandström T, Blomberg A. 2007. Ozone enhances the airway inflammation initiated by diesel exhaust. Respir Med. 101(6):1140–1146. doi: 10.1016/j.rmed.2006.11.010.
- Botto M, Lissandrini D, Sorio C, Walport MJ. 1992. Biosynthesis and secretion of complement component (C3) by activated human polymorphonuclear leukocytes. J Immunol. 149(4):1348–1355.
- Boyle JJ. 2005. Macrophage activation in atherosclerosis: pathogenesis and pharmacology of plaque rupture. Curr Vasc Pharmacol. 3(1):63–68. doi: 10.2174/1570161052773861.
- Brain JD. 1980. Macrophage damage in relation to the pathogenesis of lung diseases. Environ Health Perspect. 35:21–28. doi: 10.1289/ehp.803521.
- Camous L, Roumenina L, Bigot S, Brachemi S, Frémeaux-Bacchi V, Lesavre P, Halbwachs-Mecarelli L. 2011. Complement alternative pathway acts as a positive feedback amplification of neutrophil activation. Blood. 117(4):1340–1349. doi: 10.1182/blood-2010-05-283564.
- CARB. 2022. Overview: diesel exhaust & health. The California Air Resources Board; [assessed 2022 Dec]. https://ww2.arb.ca.gov/resources/overview-diesel-exhaust-and-health.
- Carter JM, Driscoll KE. 2001. The role of inflammation, oxidative stress, and proliferation in silica-induced lung disease: a species comparison. J Environ Pathol Toxicol Oncol. 20(Suppl. 1):12. doi: 10.1615/JEnvironPatholToxicolOncol.v20.iSuppl.1.30.
- Caston R, Luc K, Hendrix D, Hurowitz JA, Demple B. 2018. Assessing toxicity and nuclear and mitochondrial DNA damage caused by exposure of mammalian cells to lunar regolith simulants. Geohealth. 2(4):139–148. doi: 10.1002/2017GH000125.
- Castranova V, Bowman L, Reasor MJ, Lewis T, Tucker J, Miles PR. 1985. The response of rat alveolar macrophages to chronic inhalation of coal dust and/or diesel exhaust. Environ Res. 36(2):405–419. doi: 10.1016/0013-9351(85)90034-9.
- Castranova V, Jones T, Barger M, Afshari A, Frazer DG. 1990. Pulmonary responses of guinea pigs to consecutive exposures to cotton dust. In: Jacobs R, Wakelyn P, Domelsmith L, editors. Proceedings of the 14th Cotton Dust Research Conference; Jan 10–13; Las Vegas (NV): National Cotton Council; p. 131–135.
- Castranova V, Porter D, Millecchia L, Ma JY, Hubbs AF, Teass A. 2002. Effect of inhaled crystalline silica in a rat model: time course of pulmonary reactions. Mol Cell Biochem. 234–235 (1–2):177–184.
- Castranova V, Rabovsky J, Tucker JH, Miles PR. 1988. The alveolar type II epithelial cell: a multifunctional pneumocyte. Toxicol Appl Pharmacol. 93(3):472–483. doi: 10.1016/0041-008x(88)90051-8.
- Castranova V. 1998. Particles and the airways: basic biological mechanisms of pulmonary pathogenicity. Appl Occup Environ Hyg. 13(8):613–616. doi: 10.1080/1047322X.1998.10390122.
- Chang YS, Kim YJ, Yoo HS, Sung DK, Kim SY, Kang S, Park WS. 2009. Alpha-phenyl-N-tert-butylnitrone attenuates hyperoxia-induced lung injury by down-modulating inflammation in neonatal rats. Exp Lung Res. 35(3):234–249. doi: 10.1080/01902140802573007.
- Chong S, Lee KS, Chung MJ, Han J, Kwon OJ, Kim TS. 2006. Pneumoconiosis: comparison of imaging and pathologic findings. Radiographics. 26(1):59–77. doi: 10.1148/rg.261055070.
- Creutzenberg O, Bellmann B, Muhle H, Dasenbrock C, Morrow P, Mermelstein R. 1998. Lung clearance and retention of toner, TiO2, and crystalline silica, utilizing a tracer technique during chronic inhalation exposure in Syrian golden hamsters. Inhal Toxicol. 10:731–751.
- Crucian B, Quiriarte H, Nelman M, Lam C-W, James JT, Sams C. 2014. Pulmonary and systemic immune response to chronic lunar dust inhalation. NASA Technical Report Server. [accessed 2019 May]. http://ntrs.nasa.gov/search.jsp?R=20140013073.
- Dockery DW, Pope CA, Xu X, Spengler JD, Ware JH, Fay ME, Ferris BG, Speizer FE. 1993. An association between air pollution and mortality in six US cities. N Engl J Med. 329(24):1753–1759. doi: 10.1056/NEJM199312093292401.
- Domínguez-Rodríguez A, Báez-Ferrer N, Abreu-González P, Rodríguez S, Díaz R, Avanzas P, Hernández-Vaquero D. 2021. Impact of desert dust events on the cardiovascular disease: a systematic review and meta-analysis. J Clin Med. 10(4):727. doi: 10.3390/jcm10040727.
- Donaldson K, Beswick PH, Gilmour PS. 1996. Free radical activity associated with the surface of particles: a unifying factor in determining biological activity? Toxicol Lett. 88(1–3):293–298. doi: 10.1016/0378-4274(96)03752-6.
- Driscoll KE, Borm PJA. 2020. Expert workshop on the hazards and risks of poorly soluble low toxicity particles. Inhal Toxicol. 32(2):53–62. doi: 10.1080/08958378.2020.1735581.
- Driscoll KE, Carter JM, Hassenbein DG, Howard B. 1997. Cytokines and particle-induced inflammatory cell recruitment. Environ Health Perspect. 105(Suppl. 5):1159–1164. doi: 10.1289/ehp.97105s51159.
- Driscoll KE, Deyo LC, Carter JM, Howard BW, Hassenbein DG, Bertram TA. 1997. Effects of particle exposure and particle-elicited inflammatory cells on mutation in rat alveolar epithelial cells. Carcinogenesis. 18(2):423–430. doi: 10.1093/carcin/18.2.423.
- Driscoll KE, Howard BW, Carter JM, Asquith T, Johnston C, Detilleux P, Kunkel SL, Isfort RJ. 1996. Alpha-quartz-induced chemokine expression by rat lung epithelial cells: effects of in vivo and in vitro particle exposure. Am J Pathol. 149(5):1627–1637.
- Driscoll KE. 1996. The role of inflammation in the development of rat lung tumors in response to chronic particle exposure. Inhal Toxicol. 8:139–153.
- Driscoll KE. 2022. Review of lung particle overload, rat lung cancer, and the conclusions of the Edinburgh Expert Panel—it’s time to revisit cancer hazard classifications for titanium dioxide and carbon black. Front Public Health. 10:907318. doi: 10.3389/fpubh.2022.907318.
- Egger G, Spendel S, Porta S. 1988. Characteristics of ingress and life span of neutrophils at a site of acute inflammation, determined with the Sephadex model in rats. Exp Pathol. 35(4):209–218. doi: 10.1016/s0232-1513(88)80090-2.
- Eidelman O, Srivastava M, Zhang J, Leighton X, Murtie J, Jozwik C, Jacobson K, Weinstein DL, Metcalf EL, Pollard HB. 2001. Control of the proinflammatory state in cystic fibrosis lung epithelial cells by genes from the TNF-alphaR/NFkappaB pathway. Mol Med. 7(8):523–534. doi: 10.1007/BF03401858.
- Elder A, Gelein R, Finkelstein JN, Driscoll KE, Harkema J, Oberdörster G. 2005. Effects of subchronically inhaled carbon black in three species. I. Retention kinetics, lung inflammation, and histopathology. Toxicol Sci. 88(2):614–629. doi: 10.1093/toxsci/kfi327.
- EPA. 2021. New approach methods work plan. Washington (DC): Office of Chemical Safety and Pollution Prevention, Office of Research and Development, U.S. Environmental Protection Agency. https://www.epa.gov/system/files/documents/2021-11/nams-work-plan_11_15_21_508-tagged.pdf.
- Ezekowitz RA, Sim RB, MacPherson GG, Gordon S. 1985. Interaction of human monocytes, macrophages, and polymorphonuclear leukocytes with zymosan in vitro. Role of type 3 complement receptors and macrophage-derived complement. J Clin Invest. 76(6):2368–2376. doi: 10.1172/JCI112249.
- Ferrante A. 1992. Activation of neutrophils by interleukins-1 and -2 and tumor necrosis factors. Immunol Ser. 57:417–436.
- Finkelstein JN, Johnston C, Barrett T, Oberdörster G. 1997. Particulate–cell interactions and pulmonary cytokine expression. Environ Health Perspect. 105(Suppl. 5):1179–1182. doi: 10.1289/ehp.97105s51179.
- Fritsch P, Masse R. 1992. Overview of pulmonary alveolar macrophage renewal in normal rats and during different pathological processes. Environ Health Perspect. 97:59–67. doi: 10.1289/ehp.929759.
- Fubini B, Bolis V, Giamello E, Pugliese L, Volante M. 1989. The formation of oxygen reactive radicals at the surface of the crushed quartz dusts as a possible cause of silica pathogenicity. In: Mossman BT, Bégin RO, editors. Effects of mineral dusts on cells. NATO ASI series. Vol. 30. Berlin, Heidelberg: Springer; p. 205–214.
- Fubini B, Bolis V, Giamello E. 1987. The surface chemistry of crushed quartz dust in relation to its pathogenesis. Inorg Chim Acta. 138(3):193–197. doi: 10.1016/S0020-1693(00)81222-0.
- Fubini B, Giamello E, Volante M, Bolis V. 1990. Chemical functionalities at the silica surface determining its reactivity when inhaled. Formation and reactivity of surface radicals. Toxicol Ind Health. 6(6):571–598.
- Fubini B, Hubbard A. 2003. Reactive oxygen species (ROS) and reactive nitrogen species (RNS) generation by silica in inflammation and fibrosis. Free Radic Biol Med. 34(12):1507–1516. doi: 10.1016/s0891-5849(03)00149-7.
- Fubini B, Mollo L, Giamello E. 1995. Free radical generation at the solid/liquid interface in iron containing minerals. Free Radic Res. 23(6):593–614. doi: 10.3109/10715769509065280.
- Fubini B. 1998. Surface chemistry and quartz hazard. Ann Occup Hyg. 42(8):521–530. doi: 10.1016/s0003-4878(98)00066-0.
- Ghio AJ, Kim C, Devlin RB. 2000. Concentrated ambient air particles induce mild pulmonary inflammation in healthy human volunteers. Am J Respir Crit Care Med. 162(3 Pt 1):981–988. doi: 10.1164/ajrccm.162.3.9911115.
- Giannadaki D, Pozzer A, Lelieveld J. 2014. Modeled global effects of airborne desert dust on air quality and premature mortality. Atmos Chem Phys. 14(2):957–968. doi: 10.5194/acp-14-957-2014.
- Gupta-Wright A, Tembo D, Jambo KC, Chimbayo E, Mvaya L, Caldwell S, Russell DG, Mwandumba HC. 2017. Functional analysis of phagocyte activity in whole blood from HIV/tuberculosis-infected individuals using a novel flow cytometry-based assay. Front Immunol. 8:1222. doi: 10.3389/fimmu.2017.01222.
- Hamilton RF Jr, Thakur SA, Holian A. 2008. Silica binding and toxicity in alveolar macrophages. Free Radic Biol Med. 44(7):1246–1258. doi: 10.1016/j.freeradbiomed.2007.12.027.
- Harrington AD, McCubbin FM, Kaur J, Smirnov A, Galdanes K, Schoonen MAA, Chen LC, Tsirka SE, Gordon T. 2017. Pulmonary inflammatory responses to acute meteorite dust exposures—implications for human space exploration. Lunar Planet Sci. XLVIII:2922.
- Harrington AD, Schmidt MP, Szema AM, Galdanes K, Tsirka SE, Gordon T, Schoonen MAA. 2017. The role of Iraqi dust in inducing lung injury in United States soldiers—an interdisciplinary study: Iraqi dust and U.S. soldiers’ lung injury. Geohealth. 1(5):237–246. doi: 10.1002/2017GH000071.
- Haslam PL, Turton CW, Heard B, Lukoszek A, Collins JV, Salsbury AJ, Turner-Warwick M. 1980. Bronchoalveolar lavage in pulmonary fibrosis: comparison of cells obtained with lung biopsy and clinical features. Thorax. 35(1):9–18. doi: 10.1136/thx.35.1.9.
- Hector A, Griese M, Hartl D. 2014. Oxidative stress in cystic fibrosis lung disease: an early event, but worth targeting? Eur Respir J. 44(1):17–19. doi: 10.1183/09031936.00038114.
- Heinrich U, Fuhst R, Rittinghausen S, Creutzenberg O, Bellmann B, Koch W, Levsen K. 1995. Chronic inhalation exposure of Wistar rats and two different strains of mice to diesel engine exhaust, carbon black, and titanium dioxide. Inhal Toxicol. 7(4):533–556. doi: 10.3109/08958379509015211.
- Helleday R, Sandström T, Stjernberg N. 1994. Differences in bronchoalveolar cell response to nitrogen dioxide exposure between smokers and nonsmokers. Eur Respir J. 7(7):1213–1220. doi: 10.1183/09031936.94.07071213.
- Herrero-Cervera A, Soehnlein O, Kenne E. 2022. Neutrophils in chronic inflammatory diseases. Cell Mol Immunol. 19(2):177–191. doi: 10.1038/s41423-021-00832-3.
- Hetland RB, Schwarze PE, Johansen BV, Myran T, Uthus N, Refsnes M. 2001. Silica-induced cytokine release from A549 cells: importance of surface area versus size. Hum Exp Toxicol. 20(1):46–55. doi: 10.1191/096032701676225130.
- Hext PM. 1994. Current perspectives on particulate induced pulmonary tumours. Hum Exp Toxicol. 13(10):700–715. doi: 10.1177/096032719401301009.
- Hill HR, Hogan NA, Bale JF, Hemming VG. 1977. Evaluation of nonspecific (alternative pathway) opsonic activity by neutrophil chemiluminescence. Int Arch Allergy Appl Immunol. 53(6):490–497. doi: 10.1159/000231790.
- [IARC] International Agency for Research on Cancer. 1989. Diesel and gasoline engine exhausts and some nitroarenes. IARC monographs on the evaluation of carcinogenic risks to humans. Vol. 46. Lyon; Lyon, France: International Agency for Research on Cancer; World Health Organization.
- [IARC] International Agency for Research on Cancer. 2013. IARC: outdoor air pollution a leading environmental cause of cancer deaths. Press release (17 October 2013); [accessed 2019 May]. Lyon, France: International Agency for Research on Cancer, World Health Organization. https://www.iarc.fr/wp-content/uploads/2018/07/pr221_E.pdf.
- Iba T, Hashiguchi N, Nagaoka I, Tabe Y, Murai M. 2013. Neutrophil cell death in response to infection and its relation to coagulation. J Intensive Care. 1(1):13. doi: 10.1186/2052-0492-1-13.
- Jacquot J, Tabary O, Le Rouzic P, Clement A. 2008. Airway epithelial cell inflammatory signalling in cystic fibrosis. Int J Biochem Cell Biol. 40(9):1703–1715. doi: 10.1016/j.biocel.2008.02.002.
- Jeon NL, Baskaran H, Dertinger SK, Whitesides GM, Van de Water L, Toner M. 2002. Neutrophil chemotaxis in linear and complex gradients of interleukin-8 formed in a microfabricated device. Nat Biotechnol. 20(8):826–830. doi: 10.1038/nbt712.
- Johnston CJ, Driscoll KE, Finkelstein JN, Baggs R, O'Reilly MA, Carter J, Gelein R, Oberdörster G. 2000. Pulmonary chemokine and mutagenic responses in rats after subchronic inhalation of amorphous and crystalline silica. Toxicol Sci. 56(2):405–413. doi: 10.1093/toxsci/56.2.405.
- Khan TZ, Wagener JS, Bost T, Martinez J, Accurso FJ, Riches DW. 1995. Early pulmonary inflammation in infants with cystic fibrosis. Am J Respir Crit Care Med. 151(4):1075–1082. doi: 10.1164/ajrccm/151.4.1075.
- Knaapen AM, Seiler F, Schilderman PA, Nehls P, Bruch J, Schins RP, Borm PJ. 1999. Neutrophils cause oxidative DNA damage in alveolar epithelial cells. Free Radic Biol Med. 27(1–2):234–240. doi: 10.1016/s0891-5849(98)00285-8.
- Knudsen E, Benestad HB, Seierstad T, Iversen PO. 2004. Macrophages in spleen and liver direct the migration pattern of rat neutrophils during inflammation. Eur J Haematol. 73(2):109–122. doi: 10.1111/j.1600-0609.2004.00263.x.
- Kolling A, Ernst H, Rittinghausen S, Heinrich U. 2011. Relationship of pulmonary toxicity and carcinogenicity of fine and ultrafine granular dusts in a rat bioassay. Inhal Toxicol. 23(9):544–554. doi: 10.3109/08958378.2011.594458.
- Koto H, Salmon M, Haddad el-B, Huang TJ, Zagorski J, Chung KF. 1997. Role of cytokine-induced neutrophil chemoattractant (CINC) in ozone-induced airway inflammation and hyperresponsiveness. Am J Respir Crit Care Med. 156(1):234–239. doi: 10.1164/ajrccm.156.1.9606095.
- Krewski D, Burnett RT, Goldberg MS, Hoover BK, Siemiatycki J, Jerrett M, Abrahamowicz M, White WH. 2003. Overview of the reanalysis of the Harvard Six Cities Study and American Cancer Society Study of particulate air pollution and mortality. J Toxicol Environ Health A. 66(16–19):1507–1551. doi: 10.1080/15287390306424.
- Laden F. 2019. A tale of six cities: the Landmark Harvard Six Cities Study. Environ Epidemiol. 3:221. doi: 10.1097/01.EE9.0000608272.94008.7b.
- Lam C-W, Castranova V, Zeidler-Erdely PC, Renne R, Hunter R, McCluskey R, Scully RR, Wallace WT, Zhang Y, Ryder VE, et al. 2022. Comparative pulmonary toxicities of lunar dusts and terrestrial dusts (TiO2 & SiO2) in rats and an assessment of the impact of particle-generated oxidants on the dusts’ toxicities. Inhal Toxicol. 34(3–4):51–67. doi: 10.1080/08958378.2022.2038736.
- Lam C-w, Scully RR, Zhang Y, Renne RA, Hunter RL, McCluskey RA, Chen BT, Castranova V, Driscoll KE, Gardner DE, et al. 2013. Toxicity of lunar dust assessed in inhalation-exposed rats. Inhal Toxicol. 25(12):661–678. doi: 10.3109/08958378.2013.833660.
- Lapp NL, Castranova V. 1993. How silicosis and coal workers’ pneumoconiosis develop—a cellular assessment. Occup Med. 8(1):35–56.
- Laskin DL, Malaviya R, Laskin JD. 2019. Role of macrophages in acute lung injury and chronic fibrosis induced by pulmonary toxicants. Toxicol Sci. 168(2):287–301. doi: 10.1093/toxsci/kfy309.
- Laskin DL, Sunil VR, Gardner CR, Laskin JD. 2011. Macrophages and tissue injury: agents of defense or destruction? Annu Rev Pharmacol Toxicol. 51(1):267–288. doi: 10.1146/annurev.pharmtox.010909.105812.
- Last JA, Sun WM, Witschi H. 1994. Ozone, NO, and NO2: oxidant air pollutants and more. Environ Health Perspect. 102(10):179–184.
- Le Jeune I, Gribbin J, West J, Smith C, Cullinan P, Hubbard R. 2007. The incidence of cancer in patients with idiopathic pulmonary fibrosis and sarcoidosis in the UK. Respir Med. 101(12):2534–2540. doi: 10.1016/j.rmed.2007.07.012.
- Lee KP, Trochimowicz HJ, Reinhardt CF. 1985. Pulmonary response of rats exposed to titanium dioxide (TiO2) by inhalation for two years. Toxicol Appl Pharmacol. 79(2):179–192. doi: 10.1016/0041-008x(85)90339-4.
- Liu CY, Liu YH, Lin SM, Yu CT, Wang CH, Lin HC, Lin CH, Kuo HP. 2003. Apoptotic neutrophils undergoing secondary necrosis induce human lung epithelial cell detachment. J Biomed Sci. 10(6):746–756. doi: 10.1007/BF02256327.
- Liu J, Pang Z, Wang G, Guan X, Fang K, Wang Z, Wang F. 2017. Advanced role of neutrophils in common respiratory diseases. J Immunol Res. 2017:6710278. doi: 10.1155/2017/6710278.
- Lorant DE, Albertine K, Bohnsack JF. 1999. Chronic lung disease of early infancy: role of neutrophils. In Bland RD, Coalson J, editors. Chronic lung disease in early infancy, lung biology in health and disease. New York: Marcel Dekker; p. 793–812.
- Makni-Maalej K, Chiandotto M, Hurtado-Nedelec M, Bedouhene S, Gougerot-Pocidalo MA, Dang PM, El-Benna J. 2013. Zymosan induces NADPH oxidase activation in human neutrophils by inducing the phosphorylation of p47phox and the activation of Rac2: involvement of protein tyrosine kinases. Biochem Pharmacol. 85(1):92–100. doi: 10.1016/j.bcp.2012.10.010.
- Mauderly JL, Jones RK, Griffith WC, Henderson RF, McClellan RO. 1987. Diesel exhaust is a pulmonary carcinogen in rats exposed chronically by inhalation. Fundam Appl Toxicol. 9(2):208–221. doi: 10.1093/toxsci/9.2.208.
- Mauderly JL, Snipes MB, Barr EB, Belinsky SA, Bond JA, Brooks AL, Chang IY, Cheng YS, Gillett NA, Griffith WC, et al. 1994. Pulmonary toxicity of inhaled diesel exhaust and carbon black in chronically exposed rats. Part I: neoplastic and nonneoplastic lung lesions. Res Rep Health Eff Inst. 68(Pt 1):1–97.
- Mauderly JL. 1997. Relevance of particle-induced rat lung tumors for assessing lung carcinogenic hazard and human lung cancer risk. Environ Health Perspect. 105(Suppl. 5):1337–1346. doi: 10.1289/ehp.97105s51337.
- McKay DS, Cooper BL, Taylor LA, James JT, Thomas-Keprta K, Pieters CM, Wentworth SJ, Wallace WT, Lee TS. 2015. Properties of potentially toxic, respirable-size lunar dust. Acta Astronaut. 107:163–176. doi: 10.1016/j.actaastro.2014.10.032.
- Michaudel C, Mackowiak C, Maillet I, Fauconnier L, Akdis CA, Sokolowska M, Dreher A, Tan HT, Quesniaux VF, Ryffel B, et al. 2018. Ozone exposure induces respiratory barrier biphasic injury and inflammation controlled by IL-33. J Allergy Clin Immunol. 142(3):942–958. doi: 10.1016/j.jaci.2017.11.044.
- Miedel EL, Hankenson FC. 2015. Biology and diseases of hamsters. Lab Anim Med. Published online 2015 Jul 10:209–245. doi: 10.1016/B978-0-12-409527-4.00005-5.
- Mitsuhashi H, Hata J, Asano S, Kishimoto T. 1999. Appearance of cytokine-induced neutrophil chemoattractant isoforms and immunolocalization of them in lipopolysaccharide-induced acute lung inflammation in rats. Inflamm Res. 48(11):588–593. doi: 10.1007/s000110050508.
- Monteiller C, Tran L, MacNee W, Faux S, Jones A, Miller B, Donaldson K. 2007. The pro-inflammatory effects of low-toxicity low-solubility particles, nanoparticles and fine particles, on epithelial cells in vitro: the role of surface area. Occup Environ Med. 64(9):609–615. doi: 10.1136/oem.2005.024802.
- Morrow PE, Haseman JK, Hobbs CH, Driscoll KE, Vu V, Oberdörster G. 1996. The maximum tolerated dose for inhalation bioassays: toxicity vs overload. Fundam Appl Toxicol. 29(2):155–167. doi: 10.1093/toxsci/29.2.155.
- Morrow PE. 1988. Possible mechanisms to explain dust overloading of the lungs. Fundam Appl Toxicol. 10(3):369–384. doi: 10.1016/0272-0590(88)90284-9.
- Mossman BT, Borm PJ, Castranova V, Costa DL, Donaldson K, Kleeberger SR. 2007. Mechanisms of action of inhaled fibers, particles and nanoparticles in lung and cardiovascular diseases. Part Fibre Toxicol. 4(1):4–14. doi: 10.1186/1743-8977-4-4.
- Muhle H, Takenaka S, Mohr U, Dasenbrock C, Mermelstein R. 1989. Lung tumor induction upon long-term low-level inhalation of crystalline silica. Am J Ind Med. 15(3):343–346. doi: 10.1002/ajim.4700150309.
- Murphy J, Summer R, Wilson AA, Kotton DN, Fine A. 2008. The prolonged life-span of alveolar macrophages. Am J Respir Cell Mol Biol. 38(4):380–385. doi: 10.1165/rcmb.2007-0224RC.
- Nightingale JA, Maggs R, Cullinan P, Donnelly LE, Rogers DF, Kinnersley R, Chung KF, Barnes PJ, Ashmore M, Newman-Taylor A. 2000. Airway inflammation after controlled exposure to diesel exhaust particulates. Am J Respir Crit Care Med. 162(1):161–166. doi: 10.1164/ajrccm.162.1.9908092.
- Nordenhäll C, Pourazar J, Blomberg A, Levin JO, Sandström T, Adelroth E. 2000. Airway inflammation following exposure to diesel exhaust: a study of time kinetics using induced sputum. Eur Respir J. 15(6):1046–1051. doi: 10.1034/j.1399-3003.2000.01512.x.
- [NTP] National Toxicology Program. 1993. Toxicology and carcinogenesis studies of talc (CAS No. 14807-96-6) (non-asbestiform) in F344/N rats and B6C3F1 mice (inhalation studies). National Toxicology Program Tech. Rept. No. 507. Bethesda (MD): National Institutes of Health.
- [NTP] National Toxicology Program. 2000. 13th report on carcinogens. USA: National Toxicology Program, U.S. Department of Health and Human Services; [accessed 2019 May]. https://ntp.niehs.nih.gov/ntp/roc/content/profiles/dieselexhaustparticulates.pdf.
- Oberdörster G, Ferin J, Gelein R, Soderholm SC, Finkelstein J. 1992. Role of the alveolar macrophage in lung injury: studies with ultrafine particles. Environ Health Perspect. 97:193–199. doi: 10.2307/3431353.
- Oberdörster G, Oberdörster E, Oberdörster J. 2005. Nanotoxicology: an emerging discipline evolving from studies of ultrafine particles. Environ Health Perspect. 113(7):823–839. doi: 10.1289/ehp.7339.
- Pohlen M, Carroll D, Prisk GK, Sawyer AJ. 2022. Overview of lunar dust toxicity risk. NPJ Microgravity. 8(1):55. doi: 10.1038/s41526-022-00244-1.
- Pope CA 3rd, Burnett RT, Thun MJ, Calle EE, Krewski D, Ito K, Thurston GD. 2002. Lung cancer, cardiopulmonary mortality, and long-term exposure to fine particulate air pollution. JAMA. 287(9):1132–1141.
- Porter DW, Hubbs AF, Mercer R, Robinson VA, Ramsey D, McLaurin J, Khan A, Battelli L, Brumbaugh K, Teass A, et al. 2004. Progression of lung inflammation and damage in rats after cessation of silica inhalation. Toxicol Sci. 79(2):370–380. doi: 10.1093/toxsci/kfh110.
- Porter DW, Millecchia L, Robinson VA, Hubbs A, Willard P, Pack D, Ramsey D, McLaurin J, Khan A, Landsittel D, et al. 2002. Enhanced nitric oxide and reactive oxygen species production and damage after inhalation of silica. Am J Physiol Lung Cell Mol Physiol. 283(2):L485–L493. doi: 10.1152/ajplung.00427.2001.
- Quint JK, Wedzicha JA. 2007. The neutrophil in chronic obstructive pulmonary disease. J Allergy Clin Immunol. 119(5):1065–1071. doi: 10.1016/j.jaci.2006.12.640.
- Riley PA. 1994. Free radicals in biology: oxidative stress and the effects of ionizing radiation. Int J Radiat Biol. 65(1):27–33. doi: 10.1080/09553009414550041.
- Roberts JR, Mercer RR, Chapman RS, Cohen GM, Bangsaruntip S, Schwegler-Berry D, Scabilloni JF, Castranova V, Antonini JM, Leonard SS. 2012. Pulmonary toxicity, distribution, and clearance of intratracheally instilled silicon nanowires in rats. J Nanomater. 2012:398302–398317. doi: 10.1155/2012/398302.
- Roberts JR, Young SH, Castranova V, Antonini JM. 2007. Soluble metals in residual oil fly ash alter innate and adaptive pulmonary immune responses to bacterial infection in rats. Toxicol Appl Pharmacol. 221(3):306–319. doi: 10.1016/j.taap.2007.03.022.
- Robinson JP. 1998. Oxygen and nitrogen reactive metabolites and phagocytic cells. In: Robinson JP, Babcock GF, editors. Phagocyte function: a guide for research and clinical evaluation. New York (NY): Wiley-Liss; p. 217–252.
- Rosenthal GJ, Germolec DR, Blazka ME, Corsini E, Simeonova P, Pollock P, Kong LY, Kwon J, Luster MI. 1994. Asbestos stimulates IL-8 production from human lung epithelial cells. J Immunol. 153(7):3237–3244.
- Ross GD, Thompson RA, Walport MJ, Springer TA, Watson JV, Ward RH, Lida J, Newman SL, Harrison RA, Lachmann PJ. 1985. Characterization of patients with an increased susceptibility to bacterial infections and a genetic deficiency of leukocyte membrane complement receptor type 3 and the related membrane antigen LFA-1. Blood. 66(4):882–890. doi: 10.1182/blood.V66.4.882.882.
- Rousset-Jablonski C, Dalon F, Reynaud Q, Lemonnier L, Dehillotte C, Jacoud F, Berard M, Viprey M, Van Ganse E, Durieu I, et al. 2022. Cancer incidence and prevalence in cystic fibrosis patients with and without a lung transplant in France. Front Public Health. 10:1043691. doi: 10.3389/fpubh.2022.1043691.
- Rudloff I, Cho SX, Bui CB, McLean C, Veldman A, Berger PJ, Nold MF, Nold-Petry CA. 2017. Refining anti-inflammatory therapy strategies for bronchopulmonary dysplasia. J Cell Mol Med. 21(6):1128–1138. doi: 10.1111/jcmm.13044.
- Rydell-Törmänen K, Uller L, Erjefält JS. 2006. Direct evidence of secondary necrosis of neutrophils during intense lung inflammation. Eur Respir J. 28(2):268–274.
- Saffiotti U. 1992. Lung cancer induction by crystalline silica. In: d’Amato R, Slaga TG, Farland W, Henry C, editors. Relevance of animal studies to the evaluation of human cancer risk. New York (NY): Wiley-Liss, Inc.; p. 51–59.
- Salvi SS, Nordenhall C, Blomberg A, Rudell B, Pourazar J, Kelly FJ, Wilson S, Sandström T, Holgate ST, Frew AJ. 2000. Acute exposure to diesel exhaust increases IL-8 and GRO-alpha production in healthy human airways. Am J Respir Crit Care Med. 161(2 Pt 1):550–557. doi: 10.1164/ajrccm.161.2.9905052.
- Saran M, Summer KH. 1999. Assaying for hydroxyl radicals: hydroxylated terephthalate is a superior fluorescence marker than hydroxylated benzoate. Free Radic Res. 31(5):429–436. doi: 10.1080/10715769900300991.
- Sayes CM, Reed KL, Warheit DB. 2007. Assessing toxicity of fine and nanoparticles: comparing in vitro measurements to in vivo pulmonary toxicity profiles. Toxicol Sci. 97(1):163–180. doi: 10.1093/toxsci/kfm018.
- Scheel-Toellner D, Wang K, Craddock R, Webb PR, McGettrick HM, Assi LK, Parkes N, Clough LE, Gulbins E, Salmon M, et al. 2004. Reactive oxygen species limit neutrophil life span by activating death receptor signaling. Blood. 104(8):2557–2564. doi: 10.1182/blood-2004-01-0191.
- Seagrave J, Mauderly JL, Seilkop SK. 2003. In vitro relative toxicity screening of combined particulate and semivolatile organic fractions of gasoline and diesel engine emissions. J Toxicol Environ Health A. 66(12):1113–1132. doi: 10.1080/15287390390213881.
- Seaton A. 1983. Coal and the lung. Thorax. 38(4):241–243. doi: 10.1136/thx.38.4.241.
- Seiler F, Rehn B, Rehn S, Bruchs J. 2001. Significant differences in the cellular and molecular reactions of rat and hamster lung after quartz exposure. Toxicol Lett. 119(1):11–19. doi: 10.1016/s0378-4274(00)00289-7.
- Sellamuthu R, Umbright C, Roberts JR, Cumpston A, McKinney W, Chen BT, Frazer D, Li S, Kashon M, Joseph P. 2013. Molecular insights into the progression of crystalline silica-induced pulmonary toxicity in rats. J Appl Toxicol. 33(4):301–312. doi: 10.1002/jat.2733.
- Selley L, Phillips DH, Mudway I. 2019. The potential of omics approaches to elucidate mechanisms of biodiesel-induced pulmonary toxicity. Part Fibre Toxicol. 16(1):4. doi: 10.1186/s12989-018-0284-y.
- Silva MT, do Vale A, dos Santos NM. 2008. Secondary necrosis in multicellular animals: an outcome of apoptosis with pathogenic implications. Apoptosis. 13(4):463–482. doi: 10.1007/s10495-008-0187-8.
- Smith D. 1962. Free radical in irradiated biology material and systems. Annu Rev Nucl Sci. 12(1):577–602. doi: 10.1146/annurev.ns.12.120162.003045.
- Specktor B. 2018. Moon dust is super toxic to human cells; [accessed 2023 Jan]. Live Science; [accessed 2018 Mar 17]. https://www.livescience.com/62590-moon-dust-bad-lungs-brain.html.
- Strzyz P. 2017. Cell death: pulling the apoptotic trigger for necrosis. Nat Rev Mol Cell Biol. 18(2):72–72. doi: 10.1038/nrm.2017.1.
- Summers C, Rankin SM, Condliffe AM, Singh N, Peters AM, Chilvers ER. 2010. Neutrophil kinetics in health and disease. Trends Immunol. 31(8):318–324. doi: 10.1016/j.it.2010.05.006.
- Turci F, Corazzari I, Alberto G, Martra G, Fubini B. 2015. Free-radical chemistry as a means to evaluate lunar dust health hazard in view of future missions to the moon. Astrobiology. 15(5):371–380. doi: 10.1089/ast.2014.1216.
- Valderrama A, Zapata MI, Hernandez JC, Cardona-Arias JA. 2022. Systematic review of preclinical studies on the neutrophil-mediated immune response to air pollutants, 1980–2020. Heliyon. 8(1):e08778. doi: 10.1016/j.heliyon.2022.e08778.
- Vallyathan V, Shi X. 1997. The role of oxygen free radicals in occupational and environmental lung diseases. Environ Health Perspect. 105(1):165–177.
- Vallyathan V, Shi XL, Dalal NS, Irr W, Castranova V. 1988. Generation of free radicals from freshly fractured silica dust. Potential role in acute silica-induced lung injury. Am Rev Respir Dis. 138(5):1213–1219. doi: 10.1164/ajrccm/138.5.1213.
- Vandette K. 2018. Moon dust is extremely toxic and poses health hazards for astronauts. Nature.Science.Life; [accessed 2023 Jan]. Earth.com; [accessed 2018 Dec 19]. https://www.earth.com/news/moon-dust-toxic-health/.
- Wallace WT, Taylor LA, Liu Y, Cooper BL, McKay D, Chen B, Jeevarajan AS. 2009. Lunar dust and lunar simulant activation and monitoring. Meteorit Planet Sci. 44(7):961–970. doi: 10.1111/j.1945-5100.2009.tb00781.x.
- Wardman P. 1989. Reduction potentials of one-electron couples involving free-radicals in aqueous-solution. J Phys Chem Ref Data. 18(4):1637–1755. doi: 10.1063/1.555843.
- Watanabe K, Koizumi F, Kurashige Y, Tsurufuji S, Nakagawa H. 1991. Rat CINC, a member of the interleukin-8 family, is a neutrophil-specific chemoattractant in vivo. Exp Mol Pathol. 55(1):30–37. doi: 10.1016/0014-4800(91)90016-q.
- [WHO] WHO Regional Office for Europe. 2012. Health effects of black carbon. Copenhagen: WHO Regional Office for Europe; [accessed 2022 Dec]. http://www.euro.who.int/__data/assets/pdf_file/0004/162535/e96541.pdf.
- Wynn TA. 2011. Integrating mechanisms of pulmonary fibrosis. J Exp Med. 208(7):1339–1350. doi: 10.1084/jem.20110551.
- Yanagisawa R, Takano H, Ichinose T, Mizushima K, Nishikawa M, Mori I, Inoue K-I, Sadakane K, Yoshikawa T. 2007. Gene expression analysis of murine lungs following pulmonary exposure to Asian sand dust particles. Exp Biol Med. 232(8):1109–1118. doi: 10.3181/0612-RM-311.
- Yuen J, Pluthero FG, Douda DN, Riedl M, Cherry A, Ulanova M, Kahr WH, Palaniyar N, Licht C. 2016. NETosing neutrophils activate complement both on their own nets and bacteria via alternative and non-alternative pathways. Front Immunol. 7:137. doi: 10.3389/fimmu.2016.00137.
- Zhang DD, Hartsky MA, Warheit DB. 2002. Time course of quartz and TiO2 particle-induced pulmonary inflammation and neutrophil apoptotic responses in rats. Exp Lung Res. 28(8):641–670.
- Zhang Y, Story M, Yeshitla S, Wang X, Scully RR, Theriot C, Wu HL, Ryder VE, Lam CW. 2023. Persistent changes in expression of genes involved in inflammation and fibrosis in the lungs of rats exposed to airborne lunar dust. Inhal Toxicol. 35(5–6):139–156. doi: 10.1080/08958378.2023.2172485.