Abstract
This scoping review provides an overview of publications reporting adverse effects on the intestines of the food additives carrageenan (CGN) (E 407)/processed Eucheuma seaweed (PES) (E 407a) and carboxymethylcellulose (CMC) (E 466). It includes evidence from human, experimental mammal and in vitro research publications, and other evidence. The databases Medline, Embase, Scopus, Web of Science Core Collection, Cochrane Database of Systematic Reviews and Epistemonikos were searched without time limits, in addition to grey literature. The publications retrieved were screened against predefined criteria. From two literature searches, 2572 records were screened, of which 224 records were included, as well as 38 records from grey literature, making a total of 262 included publications, 196 on CGN and 101 on CMC. These publications were coded and analyzed in Eppi-Reviewer and data gaps presented in interactive maps. For CGN, five, 69 and 33 research publications on humans, experimental mammals and in vitro experiments were found, further separated as degraded or native (non-degraded) CGN. For CMC, three human, 20 animal and 14 in vitro research publications were obtained. The most studied adverse effects on the intestines were for both additives inflammation, the gut microbiome, including fermentation, intestinal permeability, and cancer and metabolic effects, and immune effects for CGN. Further studies should focus on native CGN, in the form and molecular weight used as food additive. For both additives, randomized controlled trials of sufficient power and with realistic dietary exposure levels of single additives, performed in persons of all ages, including potentially vulnerable groups, are needed.
1. Introduction
Despite the existing regulations aimed to secure the safety of additives in our food, among the general public there are persons who worries about health risks from intake of food additives. The topic of potential adverse effects of food additives also appears intermittently in the media. In this scoping review, literature published on adverse effects on the intestines of two commonly used food additives, carrageenan (CGN) and carboxymethylcellulose (CMC), have been compiled and presented, in order to get an overview of this issue.
1.1. Regulation of food additives
According to Regulation (EC) No 1333/2008 (Citation2008b), a food additive is defined as “any substance not normally consumed as a food in itself and not normally used as a characteristic ingredient of food, whether or not it has nutritive value, the intentional addition of which to food for a technological purpose in the manufacture, processing, preparation, treatment, packaging, transport or storage of such food results, or may be reasonably expected to result, in it or its by-products becoming directly or indirectly a component of such foods.” Only food additives included in the Community list in Annex II of this regulation may be placed on the market as such and used in foods under the conditions of use specified therein, listed in the food categories (FC) to which they may be added.
At present, 406 food additives are present in the European Union (EU) database of food additives (Regulation (EC) No 1333/2008 Citation2008a). All food additives are identified by an E number and food additives are included in the ingredient lists of foods in which they are used. Product labels must identify both the function of the additive in the finished food and the specific substance used by referring either to its E number or name. Food additives are grouped into 27 functional groups, of which those that are used to maintain product consistency comprise a heterogeneous group of more than 80 food additives. They include emulsifiers, which make it possible to mix ingredients that are not naturally miscible, for instance water and fat in mayonnaise, gelling agents, thickeners and stabilizers.
In Europe, food additives on the market are authorized by the EU in accordance with Annexes II and III to Regulation (EC) No 1333/2008 (Citation2008b) on food additives, after risk assessments by the European Food Safety Authority (EFSA). At the international level, the Joint FAO/WHO Expert Committee on Food Additives (JECFA) is responsible for evaluating the safety of food additives. Thus, food additives should not, on the basis of the scientific evidence available, pose a safety concern to the health of the consumer at the level of use proposed.
1.2. Carrageenan (CGN) (E 407)/processed Eucheuma seaweed (PES) (E 407a)
One controversial food additive is carrageenan (CGN) (E 407), which has been used as thickener, gelling agent, stabilizer, emulsifier and glazing agent (EFSA Citation2018a). It is obtained from seaweeds of the families Gigartinaceae, Solieriaceae, Hypneaceae and Furcellariaceae of the class Rhodophyceae (red seaweeds), by extraction with water or dilute aqueous alkali (Commission Regulation (EU) No. 231/2012 (Citation2012); EFSA Citation2018a). Over time, the sources for the production of CGN have changed as well as some of the algae names. As summarized by EFSA (Citation2018a), the industrial sources of CGN are mainly the species Eucheuma cottonii, E. spinosum, Chondrus crispus, C. ocellatus, Hypnea musciformis and several Gigartina, Iridaea and Furcellaria species, which are harvested either in nature or produced by seaweed farming.
The closely related food additive processed Eucheuma seaweed (PES) (E 407a) has structural similarities with CGN (E 407) (EFSA Citation2018a). The industrial sources of E 407a are strains of the red seaweeds E. cottonii and E. spinosum, from which the additive is obtained by aqueous alkaline treatment at high temperature (Commission Regulation (EU) No. 231/2012 (Citation2012); EFSA Citation2018a). Processed Eucheuma seaweed (E 407a) is distinguished from CGN (E 407) by its higher content of cellulosic matter (15%) and because the manufacturing does not include solubilisation and precipitation during processing (EFSA Citation2018a). The terms carrageenan (CGN) and processed Eucheuma seaweed (PES) are restricted in use only for the non-hydrolysed or otherwise chemically degraded polymers.
US FDA (Citation2023a) has listed that CGN and its ammonium, calcium, potassium or sodium salts may be safely used when it is the refined hydrocolloid prepared by aqueous extraction from the following species of the families Gigartinaceae and Solieriaceae of the class Rodophyceae: Chondrus crispus, C. ocellatus, Eucheuma cottonii, E. spinosum, Gigartina acicularis, G. pistillata, G. radula and G. stellata, and if it is a sulfated polysaccharide with dominant hexose units of which are galactose and anhydrogalactose with 20–40% sulfate content on a dry-weight basis. Furthermore, they inform that the food additive is used or intended for use in the amount necessary for an emulsifier, stabilizer or thickener in foods, except for those standardized foods that do not provide for such use.
The chemical name of CGN (E 407) is sulfate esters of polygalactose (sulfated polygalactans), and CGN consists mainly of the potassium, sodium, magnesium and calcium sulfate esters of galactose and 3,6-anhydrogalactose polysaccharides (Commission Regulation (EU) No. 231/2012 (Citation2012); EFSA Citation2018a). These hexoses are alternately linked α-1,3 and β-1,4 in the copolymer. The three major prevalent polysaccharides in CGN are designated k-, ι-, λ-CGN () depending on the number and position of sulfate groups on the disaccharide repeating unit; k-CGN with one sulfate group at C4 of the β-D-galactose, ι-CGN with one sulfate group at C4 of the β-D-galactose and one sulfate group at C2 of the α-3,6-anhydro-D-galactose, and λ-CGN with one sulfate group at C2 of the β-D-galactose and two sulfate groups at C2 and C6 of the α-D-galactose (Marburger Citation2003; Dräger et al. Citation2011; EFSA Citation2018a). In the processed Eucheuma seaweed (E 407a), the main polysaccharide is κ-CGN when produced from E. cottonii and primarily ι-CGN when produced from E. spinosum (EFSA Citation2018a). The CAS number for native CGN (E 407) is 9000-07-1, for κ-CGN 11114-20-8, for ι-CGN 9062-07-1 and for λ-CGN 9064-57-7 (EFSA Citation2018a). Degraded CGN, often called poligeenan (PGN), has CAS number 53973-98-1. No CAS number had been assigned to processed Eucheuma seaweed (E 407a).
Figure 1. Structural formulas of κ-, ι- and λ-CGN. The names κ-, ι- and λ-CGN do not reflect definitive chemical structures but only general differences in the composition and degree of sulfation at specific locations in the polymer (EFSA Citation2018a, reused with permission from EFSA).
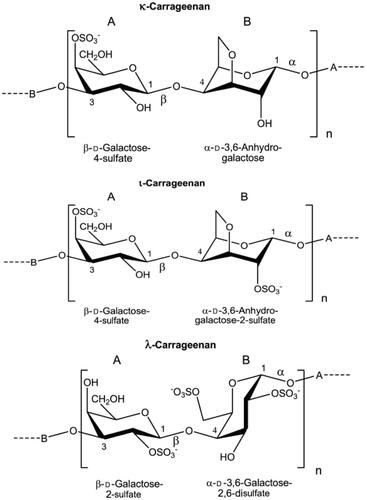
The molecular weight (MW) of CGN depends on the extraction method used (Weiner Citation1991). Industrial food grade (native) CGN is extracted with water and has average MW of 200–400 kDa. The MW is not specified for E 407 and E 407a in the EU regulation, however, according to industry, E 407 is defined as having a weight-average MW of 200–800 kDa (EFSA Citation2018a). The low MW CGN (MW fraction below 50 kDa) should be <5% of the total composition of commercial products E 407 and E 407a (Commission Regulation (EU) No. 231/2012 (Citation2012)). Degraded CGN, such as PGN, C16 and Ebimar, are not authorized as food additives in EU. PGN is prepared from ι-CGN by acid hydrolysis at low pH (0.9–1.3) and high temperature (>80°C) for several hours and has a MW of 10–20 kDa (EFSA Citation2018a). C16 is artificially formed from ι-CGN by acid hydrolysis with 0.1 M sulfuric acid at 60 °C for 1.5 h (EFSA Citation2018a) with MW of about 20 kDa (Benitz et al. Citation1973). Ebimar is a commercial degraded CGN, mostly λ-CGN, with MW of about 20 kDa (Evans et al. Citation1965).
Maximum permitted levels (MPL) of CGN (E 407) and PES (E 407a) have been defined in Annex II of Regulation (EC) No 1333/2008 (Citation2008b) with amendments. They are authorized food additives in the EU at quantum satis (QS) levels in many foods, or in some foods with specific MPLs. In food safety regulations in the EU, QS is a catch-all restriction for food ingredients, especially food additives, which are harmless enough to have no specific quantity restriction. E 407 is used for example in ice cream, salad dressing, jam and marmalade, fruit or vegetable spreads, cheese, sauces and processed meats, and E 407a is used in meat preparation (EFSA Citation2018a). The group acceptable daily intake (ADI) for CGN and PES is 75 mg/kg body weight (bw) per day (EFSA Citation2018a).
1.3. Carboxymethylcellulose (CMC) (E 466)
Another controversial food additive is carboxymethylcellulose (CMC) (E 466), also called sodium carboxy methyl cellulose or cellulose gum, used as emulsifier, stabilizer, binder, thickener and gelling agent in foods. It is the partial sodium salt of a carboxymethyl ether of cellulose (), where the cellulose is obtained from natural strains of fibrous plant material (Commission Regulation (EU) No. 231/2012 (Citation2012)). It has CAS no. 9004-32-4. E 466 is authorized as a food additive in the EU at QS in most food categories (EFSA Citation2018b). Typical foods and beverages that contain CMC are for instance ice cream, salad dressings, cheese, bakery wares and various processed foods. A numerical ADI value for the non-modified and modified celluloses, including E 466, was found not to be needed because of no safety concern at the reported uses and use levels (EFSA Citation2018b).
Figure 2. The chemical structure of CMC, in which R = H or CH2COOH (EFSA Citation2020, reused with permission from EFSA).
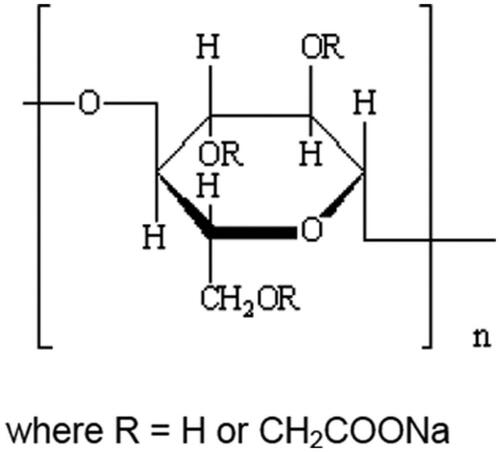
US FDA (Citation2023b) has defined sodium carboxymethylcellulose as the sodium salt of CMC not less than 99.5% on a dry-weight basis, with maximum substitution of 0.95 carboxymethyl groups per anhydroglucose unit and with a minimum viscosity of 25 centipoises for 2% by weight aqueous solution at 25 °C. This substance is generally recognized as safe (GRAS) when used in accordance with good manufacturing practice.
1.4. Objectives
In this work, an extensive scoping review on adverse effects on the intestines of the food additives CGN (E 407)/PES (E 407a) and CMC (E 466) has been conducted. The study population was the general healthy population of both sexes and all age groups, as well as patients with intestinal diseases. Regarding concept and context, the adverse effects were limited to three main hypotheses: inflammation, including effects on barrier dysfunction or permeability of the intestines, autoimmune effects or effects on the intestinal microbiome, without further limitations in geography, publication year or other factors. The data on adverse health effects were obtained from the three usual streams of such information; human studies, experimental animal studies and in vitro studies. The objectives of this scoping review were to obtain an overview of the available evidence and to identify knowledge gaps for further research on and risk assessments of these food additives.
2. Methodology
As part of planning of this scoping review, a protocol was made but not published, however, all the same information is included in this section. This scoping review was guided by the framework for scoping reviews originally proposed by Arksey and O’Malley (Citation2005) with later enhancements and updates of the methodology (Levac et al. Citation2010; Daudt et al. Citation2013; Munn et al. Citation2018; Peters et al. Citation2021a, Citation2021b; Page et al. Citation2021a, Citation2021b). In addition, the latest version of guidance and checklist specific for scoping reviews (Tricco et al. Citation2018) together with guidance for systematic reviews (Page et al. Citation2021a, Citation2021b) were consulted (Appendix A, PRISMA-ScR checklist filled in for this scoping reviews).
2.1. Systematic literature searches
First, a pilot search was performed in December 2021 in the database Medline (OVID) to get a quick overview of the topic of interest (not shown). Further, an extensive peer-reviewed literature search in several databases (Appendix B, First literature search) was performed in order to obtain publications on the three lines of evidence; human studies, experimental animal studies and in vitro studies.
The text words contained in the titles and abstracts of relevant articles in the pilot search and the MeSH and other index terms describing the articles were used to develop a full search strategy in collaboration with the library staff at the Norwegian Institute of Public Health (NIPH). The search strategy, including all identified key words and index terms, was adapted for each of the included six databases, Medline (OVID), Embase (OVID), Scopus (Elsevier B.V.), Web of Science Core Collection [SCI-EXPANDED & SSCI] (Clarivate), Cochrane Database of Systematic Reviews [CDSR, CENTRAL] (Wiley) and Epistemonikos (Epistemonikos foundation) by a research librarian, before peer-review by a second librarian. The searches were run on 31.01.22, except for the Cochrane Database, which was run on 28.01.22. No restrictions were included in the searches related to publication date to obtain as much information as possible. Also, no restrictions were used for type of publications in order to be as comprehensive as possible. Reviews, both systematic and narrative, were included. Conference abstracts were included, representing new data, possibly not yet published as full-text articles. Additionally, commentaries, editorial articles, letters to the editor etc. were included to get an understanding of the reasons for the controversy around these food additives. However, in this review more emphasis was put on the original research publications (human, experimental animals and in vitro studies). The searches were limited to the languages English, Norwegian, Danish or Swedish. Duplicates were removed in EndNote v. 20 (Clarivate, AZ, USA), before the search outcome was uploaded in the Eppi-Reviewer (ER) software (version 4.13.0.2) (Evidence for Policy and Practice Information and Co-ordinating Centre (EPPI-Centre), Institute of Education, University of London, UK) for further analyses.
A repeated literature search in the same databases under the same conditions as the first search was performed by the NIPH library to obtain additional newer publications on December 7 and 8, 2022 (Appendix C, Repeated literature search).
2.2. Grey literature
Relevant risk assessment reports were searched for in October 2022 at the web sites of EFSA, JECFA, National Institutes of Health (NIH, USA), European Medicines Agency (EMA), the Federal Institute for Risk Assessment (BfR, Germany), French National Institute for Industrial Environment and Risks (INERIS), French Agency for Food, Environmental and Occupational Health and Safety (ANSES), National Institute for Public Health and the Environment (RIVM, the Netherlands) and the EU Commission Joint Research Centre (JRC).
Master and doctoral theses were searched for in PROSPERO (International prospective register of systematic reviews), Cristin (Current Research Information System in Norway), Oria (a search engine for search in the Norwegian academic libraries’ resources) and System for Information on Grey Literature in Europe (SIGLE) in November-December 2022.
Grey literature was also searched in December 2022 in Google Scholar using the search terms (carrageenan OR carboxymethylcellulose) AND intestin*. However, since this search returned approximately 19000 and 18500 publications, respectively, many of which were already obtained by the library database searches, were books not available unless purchased or were irrelevant to the scope of this work, only the first 100 hits for each food additive were scrutinized for inclusion. Many of the publications were found in several sources, i.e. in Google Scholar and in references list of already included publications.
2.3. Selection of evidence
The obtained publications were screened on two levels using predefined inclusion and exclusion criteria (). Titles and abstracts were screened independently by two reviewers for assessment against the inclusion criteria in ER. Thereafter, publications that full-filled the inclusion criteria were read in full-text by two independent reviewers. Conflicts between the two reviewers were resolved by discussions or by a third reviewer, on both stages of the selection process.
Table 1. Inclusion and exclusion criteria used for selection of publications obtained from the literature searches.
The inclusion criteria comprised adverse effects on the intestines of the food additives CGN (E 407)/PES (E 407a) and CMC (E 466). From the pilot search, three main hypotheses on adverse effects on the intestines of these two food additives appeared to be prevalent and search terms to cover these were therefore included in the main search strategy: 1) effects on barrier dysfunction or permeability of the intestines, often including inflammation, 2) autoimmune effects or 3) effects on the intestinal microbiome. Studies mainly exploring positive health effects of varying substances or treatments were screened when they included a group only given CGN or CMC. Studies on adverse effects on the general healthy human population of both sexes and all age groups without any geographical limitations, or in experimental mammals, were included. Publications which studied diseases of the intestinal tract, such as inflammatory bowel disease (IBD) or irritable bowel syndrome (IBS) in patients or animal models, were included. IBD is an immune-mediated, chronic inflammatory disease that affects the gastrointestinal tract and is a common term for Crohn’s disease (CD) and ulcerative colitis (UC) (Park et al. Citation2020).
The results of the literature searches and the study inclusion/exclusion process are presented in the PRISMA-ScR flow diagram (), including the reasons for exclusion at the full-text level (see also Appendix D, Publications excluded at full-text level with reasons). The pdfs of the included full-text publications were obtained directly from the internet or ordered from the NIPH library and uploaded into ER. No authors were contacted for additional information about their publications.
Figure 3. PRISMA flow chart of literature identification, selection and inclusion for the scoping review, guided by Tricco et al. (Citation2018), Page et al. (Citation2021a, Citation2021b).
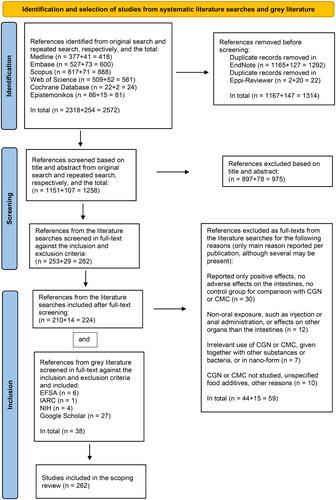
2.4. Data extraction and analyses
The categories of information in the studies relevant to full-fill the study objectives were extracted from the included full-text publications and sorted into standardized categories, called codes in ER, using frequency and cross-table functions in this software. The codes are presented in Appendix E, Eppi-Reviewer Code book. Two authors categorized each publication independently of each other. Disagreements were resolved by discussion.
The data included, i.e. the codes, were the food additive, CGN (E 407)/PES (E 407a) or CMC (E 466). The food additives were further coded in sub-categories, for CGN; κ-, ί- or λ-CGN, unspecified CGN, degraded CGN (including PGN, C16, Ebimar), modified CGN, and for CMC; non-modified CMC or modified CMC (Appendix E, Eppi-Reviewer Code book). This definition of modified CMC is used in a more general sense and is not consistent with the group “chemically modified celluloses” to which E 466 belongs, as defined by EFSA (Citation2018b). Other codes were publication type (human, animal, in vitro or other types of studies, conference abstracts), human study design (systematic review, randomized controlled trial (RCT), non-randomized controlled study, prospective/retrospective cohort study, case-control study, cross-sectional study, case report, incidence/prevalence study), experimental animal study (species), in vitro studies (human or animal cells or microbiota, or other in vitro studies), other types of studies (toxicokinetics, non-systematic reviews, commentaries/editorials/letters, studies with data relevant for exposure and risk assessments), human study population (general healthy population (children, adolescents, adults)) or patients (with IBD, IBS, other diseases)) and publication year. Lastly, formulated hypotheses, suggested mechanisms, explanations for an effect or similar statements in the publications for adverse effects on the intestines were noted for each publication. They were first grouped in three main hypotheses (effects on barrier dysfunction or permeability of the intestines, including inflammation, autoimmune effects, effects on the intestinal microbiome), but were later divided in more groups since other hypotheses were also mentioned in the included publications (as shown in and ). Several publications contained more than one type of data, such as from both experimental animal and in vitro studies. For a few publications in addition to the conference abstracts, the coding was based only on the title, abstract and content list (for books), since full-text was not available.
2.5. Presentation of the results
This scoping review presents the various categories of information from the included publications relevant for the research questions in text, tables and figures. The figures were made in Excel from the data extracted into various categories by using frequency and cross-table functions in ER. In addition, two interactive research maps were made with EPPI-Mapper included in the ER software. These maps were made by exporting the data extracted in ER as a JSON data file (https://www.json.org/json-en.html).
3. Results
3.1. Number of relevant publications obtained
From the first main literature search, 2318 publications were obtained from all six databases, which after removal of duplicates in EndNote was reduced to 1165. After import into ER, two additional duplicates were observed, reducing the number of publications obtained to 1151 that were screened at the level of title and abstract according to the inclusion and exclusion criteria (). These publications were handled in the ER software as shown in . Of these, 253 publications were considered potentially relevant and were assessed in the full-text version according to the same inclusion and exclusion criteria. In the follow-up second literature search, 254 publications were initially obtained, which after removal of duplicates in EndNote and ER gave 107 publications, increasing the total to 1258 publications that were screened at the level of title and abstract. From the publications obtained from the second literature search, 29 were further screened at the full-text level, increasing the total number at this step to 282 publications. After full-text screening, 210 and 14 publications from the first and second literature search, respectively, in total 224, were retained. Thirdly, from the examination of title/abstract and full-text in the same step of grey literature from many sources, six risk assessments from EFSA, one risk assessment from the International Agency for Research on Cancer (IARC), four publications from NIH and 27 publications from Google Scholar, in total 38, were included after examination (). From all this literature, in total 262 publications were finally included in this scoping review and were coded in ER for data extraction. Of these, 196 were on CGN and 101 on CMC.
The distribution of all types of publications per five-year-periods is shown for CGN and CMC in , showing that research interest in CGN has been ongoing since 1961, whereas CMC publications are mostly from the last decade, when also CGN interest has increased substantially.
The reasons for exclusion at full-text level of 59 publications are listed in Appendix D. Publications excluded at full-text level with reasons. Studies that only looked for positive health effects of these food additives were outside the scope of this review, or did not have a control group to compare the food additives with, were excluded. During the screening process, it became apparent that in many studies, CGN was injected in animal paws or lung tissues to induce inflammation in order to test natural substances or drugs that could reduce or eliminate inflammation. Although this non-oral administration route was not relevant for food additives, it was apparent from these studies that CGN could induce inflammation in the skin or lungs in various animal models. In other publications, the food additives were administered intrarectally. Thus, these studies using non-oral administration routes were outside our inclusion criteria and the publications were excluded. Another large group that was excluded were studies where CMC was used as an adhesive under operative procedures in the intestines or other organs, or was used as a component of new delivery systems for drugs. Additional publications that were excluded for not meeting the inclusion criteria studied effects of these food additives on other parts of the body than the intestines or examined effects of other treatments, substances or bacteria together with CGN or CMC, or were in nano form.
In the following, the results will be described for each food additive separately, and for the various categories of publications separately if not stated otherwise; original research publications (humans, experimental animals, in vitro), toxicokinetics and absorption, distribution, metabolism and excretion (ADME) publications, non-systematic (narrative) reviews, commentaries/editorials/letters, conference abstracts, data relevant for exposure (from all types of publications) and risk assessment opinions. For all codes, there is often overlap between alternatives in the categories, since many publications report more than one type of food additive, type of publication, such as both animal and in vitro studies, more than one hypothesis for adverse effects and so on. When stated in the publications, the MW of the CGN types was included in the text.
3.2. Publications on carrageenan (CGN) (E 407)/processed Eucheuma seaweed (PES) (E 407a)
Since most of the publications on CGN from the literature searches did not specify the EU food additive numbers (E 407 or E 407a), the publications have not been divided accordingly, but described together. Although the main objective of this scoping review was to elucidate the potential adverse effects of the food additive E 407/E 407a, containing native, non-degraded CGN, it become apparent from an early look at the literature that even with native, non-degraded CGN there were numerous publications reporting adverse effects, and therefore, both categories, degraded and native CGN, were included for comparison in this review. Data were obtained on the three most prevalent polysaccharides of CGN (κ-CGN, ί-CGN and λ-CGN), in addition to degraded CGN (sometimes called poligeenan (PGN), C16 or Ebimar) and CGN modified in various ways. In the following text, “degraded” is native written when it is clear from the publication that a degraded CGN (with lower MW than CGN) has been used. If “degraded” is not stated in the text and there are no further specifications to indicate that CGN was degraded, the results are assumed to be from native CGN (i.e. not degraded). Studies in which the type of native CGN used were not reported were categorized as unspecified CGN. Overlap among these categories was often the case in the publications.
No time limits were used for the literature searches to obtain as many as possible of the relevant publications. The number of research publications studying degraded CGN showed a peak around 1971–1995 and a higher peak around 2006–2023 (). The first period represents publications using CGN to induce inflammation in experimental animal models, in which various anti-inflammatory substances and treatments could be tested. Publications on native CGN are also present from those early years but a higher number is published more recently, around 2011–2023.
The number of research publications studying the various types of CGN are shown in . Somewhat more publications were using κ- or λ-CGN than ι-CGN. A lot of the publications stated that they used CGN, without specifying what form of CGN they studied. An even higher number of publications was found for degraded CGN, sometimes studied together with native CGN in the same publications. No research publications used modified CGN. However, in a review, it was described that red seaweed CGNs may be chemically modified with one or more substitutions in the same molecule in different ratios, resulting in new types of CGNs called “hybrid CGNs” (Cian et al. Citation2015). In addition, an abstract described investigations on refined food grade κ-CGN with 10% Na+ or 10% Ca2+, which affect the gel formation of CGN (Farag et al. Citation2018).
Figure 6. The number of research publications studying the various types of CGN as specified in the publications. Modified CGN was only mentioned in one review publication and one abstract.
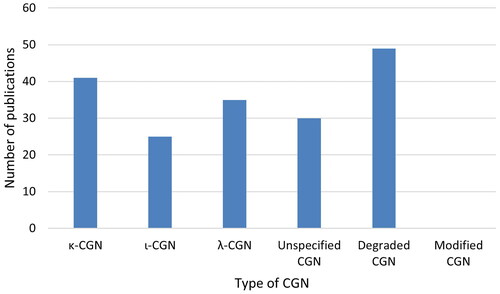
The distribution of various types of publications on CGN (original research publications on human studies, experimental animal studies and in vitro studies, as well as some other types of studies) is shown in . Only five studies reported research on humans (excluding publications on exposure), in patients with intestinal diseases. The highest number of publications was on various species of experimental animals (69), with in vitro studies as the second largest category (33), studying microbiota from humans or animals ex vivo-in vitro or describing mechanistic data in human or animal primary cells or cell lines. Twenty publications included some data on exposure or intake of CGN or its presence in foods, three publications gave information on ADME and two risk assessment opinions of CGN were available. The main results of the original research publications on humans, animals and in vitro are described briefly in the following.
Figure 7. The distribution of various types of publications on CGN (degraded and native). The human studies (without studies of exposure), experimental animal studies and in vitro studies are original research publications. Exposure studies comprise all types of publications that contain information on levels in food, exposure etc., including from review publications and conference abstracts. In addition, publications on CGN containing information on ADME and risk assessments were included.
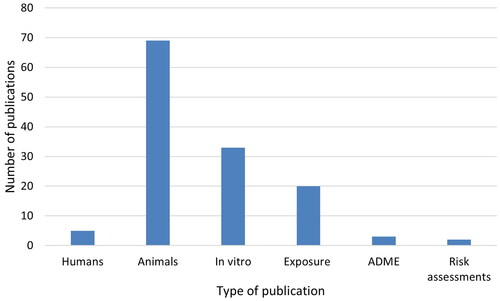
3.2.1. Human studies
Only five publications reported human studies on adverse effects of CGN on the intestines, two using degraded CGN and three using native CGN, all in adult patients. No publications were found on adverse effects of degraded or native CGN on healthy adults, or on children or adolescents.
3.2.1.1. Human studies of degraded CGN
Two older studies on adult patients without control groups were included using degraded CGN. In the first study, 40 patients of both genders with gastrointestinal ulcerations were given a degraded crude extract of CGN, mostly λ-CGN (Ebimar, MW of about 20 kDa) (Evans et al. Citation1965). One patient had mild diarrhea and three complained of constipation. One patient needed surgery for continuing severe pain and vomiting after two months. In the second study, six patients who suffered from malignant disease of the colon were given degraded CGN (Grasso et al. Citation1973). No signs of any ulceration in the samples of normal gut were seen and degraded CGN was not detected in the colon by histochemistry or other methods.
3.2.1.2. Human studies of native CGN
One relevant small randomized double-blind, placebo-controlled, multicenter, clinical trial was found (Bhattacharyya et al. Citation2017). They examined whether patients of both sex (>18 years) diagnosed with UC in remission would have a longer interval to relapse if they followed a diet without CGN vs. after oral exposure to equal parts of 100–200 mg food grade κ-, ι- and λ-CGN in gelatin capsules. The study continued until relapse or one year of participation. Of the 12 patients completing the study, three of five who received CGN relapsed, whereas none of the seven patients receiving placebo relapsed (p = 0.046). In the CGN-exposed group only, interleukin (IL)-6 and fecal calprotectin levels were increased. This clinical trial was included in the only systematic review found, on dietary interventions for induction and maintenance of remission in IBD by Limketkai et al. (Citation2019). These authors calculated a risk ratio with 95% confidence interval (CI) of 0.5 (0.15, 1.64) for the above-mentioned clinical trial and it was given very low score for certainty of the evidence (GRADE) and a high risk of bias.
In an older clinical study without control group, λ-CGN powder was given as treatment to 22 patients with peptic ulcers (17 men and 5 women) (Heineken Citation1961). The only adverse side-effect reported from CGN was one case of dizziness.
3.2.2. Animal studies
Among the included publications reporting adverse effects on the intestines of CGN, in total 69 were experimental animal studies. The numbers of publications on each animal species studied were rats (25), mice (18), guinea pigs (20), rabbits (7), hamsters (3), ferrets (1), pigs (2) and monkeys (3). Among the animal studies, a large proportion of the studies (23) was performed with unspecified native CGN, whereas the various types of CGN were more similarly represented, with 25, 15 and 19 publications for κ-, ι- and λ-CGN, respectively. The number of publications on experimental animals that had used degraded CGN was 38, whereas a somewhat lower number (31) had studied native (non-degraded CGN). Five publications studied both degraded and native CGN in animals (Watt and Marcus Citation1969; Benitz et al. Citation1973; Grasso et al. Citation1973; Mankes and Abraham Citation1975; Pintauro and Gilbert Citation1990).
3.2.2.1. Animal studies of degraded CGN
Nine publications reported studies on rats using degraded CGN. The endpoints studied were ulceration and mucosal injuries (Grasso et al. Citation1973; Benard et al. Citation2010), diarrhea and uptake in tissues (Grasso et al. Citation1975), role of intestinal bacterial flora (Hirono et al. Citation1981), intestinal permeability (Delahunty et al. Citation1987) and colitis, followed by squamous metaplasia and finally adenomas, adenocarcinomas, squamous cell papillomas and squamous cell carcinomas in the colorectum, as well as presence of CGN in macrophages in the lamina propria mucosa and submucosa of the colorectum (Ishioka et al. Citation1987).
In a model for IBD, rats were sensitized by a s.c. injection of degraded λ-CGN and Freund’s complete adjuvant followed by oral administration of the same CGN solution in drinking water, where the degraded λ-CGN caused significant small intestinal injury shown by ulceration, abnormal villous pattern, inflammation, microgranulomas and crypt abscesses, resembling human IBD (Moyana and Lalonde Citation1990; Moyana et al. Citation1994). Prior sensitization aggravated the effects of CGN. Further, a study suggested that oxygen free radicals from intestinal macrophages played a role in this intestinal injury (Moyana and Lalonde Citation1991).
Three publications reported studies on mice using degraded CGN, inducing bloody diarrhea, pericryptal inflammation and marked dilatation of the caecum and ascending colon (Fath et al. Citation1984). Combined treatment of the low MW (∼4.5 kDa) κ-CGN oligosaccharides (KCO) with KCO-degrading bacteria (Bacteroides xylanisolvens and E. coli) led to greater pro-inflammatory effects in the colon and rectum of mice than either KCO or bacteria alone (Yin et al. Citation2021). Of several strains of mice, DBA/2J was the most sensitive to colitis induced by degraded λ-CGN (MW 30 kDa) (Hata et al. Citation2006). However, β-catenin-accumulated crypts, putative precancerous lesions in colon, were not increased after initiation with azoxymethane (AOM), indicating lack of a promotor effect of degraded λ-CGN in mice.
All the 20 publications reporting studies on guinea pigs were from 2005 or earlier, and used degraded CGN, of which the three following publications also studied native CGN. In both guinea pigs given 1% undegraded CGN or 5% degraded CGN in drinking water for 3–4 weeks, ulcerative lesions in the caecum and colon, and fecal occult blood, were observed, with more extensive damage and higher incidence of ulceration in the guinea pigs given degraded CGN (Watt and Marcus Citation1969). Both 5% solutions of degraded and native CGN in drinking water produced ulceration in the caecum and colon of guinea pigs, with more serious effects in those given degraded CGN, such as severe diarrhea, blood in the feces, submucosal oedema, haemorrhage and ulceration (Grasso et al. Citation1973). Guinea pigs were administered 0.2% undegraded κ-CGN, 0.2% undegraded ι-CGN, 1% degraded κ-CGN or 1% degraded ι-CGN in the drinking water to investigate drug-metabolizing enzyme activities relevant for cancer promotion (Pintauro and Gilbert Citation1990). The undegraded κ-CGN and undegraded ι-CGN increased small intestinal cytochrome P-450 levels and benzo[a]pyrene hydroxylase activities, whereas the degraded κ-CGN and ι-CGN did not.
Most of the remaining publications studying guinea pigs also reported effects of degraded CGN (0.5–5% in drinking water) for from two-three days up to six weeks on colitis and ulcerations in the intestines, sometimes with occult blood in the feces, diarrhea or decreased body weight (Watt and Marcus Citation1971; Van der Waaij et al. Citation1974; Grasso et al. Citation1975; Boxenbaum and Dairman Citation1977; Onderdonk et al. Citation1977; Olsen and Poulsen Citation1980; Onderdonk et al. Citation1981; Jensen et al. Citation1984; Onderdonk et al. Citation1984; Langman et al. Citation1985; Marcus et al. Citation1989; Kitsukawa et al. Citation1992 (using MW 30 kDa); Marcus et al. Citation1992; Fujita and Sakurai Citation1995; Xiong et al. Citation2005). The inflammatory response was often associated with infiltration of macrophages or mononuclear phagocytes in the intestinal mucosa.
The majority of the studies did not specify the form of degraded CGN used. However, in guinea pigs given either degraded κ-, ι- or λ-CGN in drinking water for three weeks, the order of potency was ι- > λ- > κ-CGN for decreasing effect on body weight gain (Norris et al. Citation1981). When the effects on colitis of degraded Eucheuma spinosum (almost entirely ι- and nu-CGN) and degraded Eucheuma cottonii (almost entirely κ- and mu-CGN) given in drinking water to guinea pigs were compared, degraded E. spinosum CGN induced mild to moderate colitis, while E. cottonii consistently induced severe colitis in colon and rectum (Langman et al. Citation1985).
Some publications on guinea pigs also studied the influence of intestinal microflora. UC was significantly reduced by elimination of the family of Enterobacteriaceae (Van der Waaij et al. Citation1974). There were shifts in the major microbiota populations during development of the ulcerative lesions in guinea pigs (Onderdonk et al. Citation1977). Two pools of feces each containing 10 bacterial strains were obtained from conventional guinea pigs administered a solution of degraded CGN, which caused ulcerations in their ceca and large intestines (Onderdonk et al. Citation1981). When these samples were given by orogastric intubation to germfree guinea pigs all these animals also developed such ulcerations, whereas control animals did not. Further studies showed that certain strains of Bacteroides vulgatus were capable of inducing immune enhancement of UC induced by degraded CGN (Onderdonk et al. Citation1984). Immune animals which received B. vulgatus from a patient with inflammatory bowel disease, but not from a clinically normal person, had more intestinal lesions than non-immune animals.
Guinea pigs treated with degraded ι-CGN excreted more PEG-900 than controls, suggesting increased intestinal permeability (Delahunty et al. Citation1987).
All the seven publications reporting studies on rabbits were from 1996 or earlier. Most of the publications studied inflammation and ulceration caused by degraded CGN (0.3–5%) in drinking water or by gastric intubation for 4–9 weeks or even up to 7 months (Grasso et al. Citation1973; Aoki Citation1978; Al-Suhail et al. Citation1984a; Al-Suhail et al. Citation1984b; Kitano et al. Citation1996 (MW 30 kDa)). Ulcerations resembled those seen in guinea pigs and in patients with UC, and CGN was present within macrophages. A focal, high-grade dysplasia was seen in the colonic mucosa of rabbits sensitized to degraded CGN by an injection and exposed to degraded CGN in drinking water for 28 months (Kitano et al. Citation1986). A similar rabbit experiment with λ-CGN (30–33 kDa) suggested impairment of the immunoglobulin (Ig)A-regulated local immune response and an abnormality in the differentiation of immunoglobulin-secreting cells (Matsumoto et al. Citation1988).
One publication on hamsters given degraded CGN in the diet for six months and ferrets given degraded CGN by gastric intubation for 28 days did not observe ulceration and other adverse changes that were observed in guinea pigs and rabbits (Grasso et al. Citation1973).
One publication on pigs administered degraded CGN (20–30 kDa) in the drinking water found no effects on stool consistency or levels of IL-1β, IL-8, IL-6, IL-10 or tumor necrosis factor-(TNF)-α (Munyaka et al. Citation2016). However, CGN decreased bacterial species richness (α-diversity) and shifted community composition. At the phylum level, an increase in Proteobacteria and Deferribacteres, and a decrease in Firmicutes, Actinobacteria, Bacteroidetes and Tenericutes, were observed.
One publication on two squirrel monkeys given 1.5% degraded CGN by gastric intubation for 28 days revealed no ulceration and other adverse changes (Grasso et al. Citation1973). In another publication, Rhesus monkeys (Macaca mulatta) were administered degraded CGN (C16, MW 20 kDa) or native, largely κ-CGN, in drinking water for 7–14 weeks (Benitz et al. Citation1973). The monkeys given 0.5 (n = 2) or 1% (n = 2) C16 solution gained weight, but in those on 2% C16 (about 2.9 g/kg bw per day, n = 6) weight loss was considerable, and they lost blood from the intestinal tract and developed anaemia. Pathological changes in the colon ranged from shallow mucosal erosions to multiple crypt abscesses. The severity of these effects was dose-dependent, but some reversal was indicated when allowed to recover for 20–24 weeks. The six monkeys given 1% native CGN (about l–3 g/kg bw per day) all gained weight and remained in good condition. Thus, a marked difference was observed between the effects of degraded (C16) and native CGN in monkeys. Another study on Rhesus monkeys compared effects of C16, 2% degraded ι-CGN (MW ca. 20 kDa), administered to six monkeys in drinking water for 10 weeks, and 1% undegraded κ- and λ-CGN mixture (MW ca. 800 kDa) administered to six monkeys in drinking water for 10 weeks (Mankes and Abraham Citation1975). Degraded CGN, but not native CGN, was detected in lysosomes in submucosal macrophages, altering the functional state of the lysosomes. Necrosis and leucocyte infiltration were also observed in the macrophages.
3.2.2.2. Animal studies of native CGN
Seventeen publications reported studies on rats using native CGN. No ulceration or other adverse changes were observed in rats given 5% native CGN in the diet for 56 days, as found also for degraded CGN (Grasso et al. Citation1973). In rats given 50 g/kg of ι-CGN for 50 days, increased weight of the cecal contents and decreased cecal bacterial population, as well as decreased rate of reduction of several nitro-containing compounds, were observed (Rowland et al. Citation1983). In a model of IBD, rats given λ-CGN in drinking water gradually developed intestinal lesions, which were morphologically similar to those in human UC, and the proliferative response of splenocytes to known mitogens was significantly diminished (Pricolo et al. Citation1996). In rats which orally consumed 1% κ-CGN-containing PES (407a) for four months, inflammation developed in the small intestine, which was associated with a strong overexpression of the DNA repair enzyme O-6-methylguanine-DNA methyltransferase (MGMT) in enterocytes and in stroma, mainly on the surface of villi (Tkachenko et al. Citation2018b). In the same model, the CGN-induced inflammation was accompanied by higher levels of TNF-α, IL-1β and heat shock protein-90α upregulation in the intestinal mucosa (Tkachenko et al. Citation2020). In rats exposed to PES in drinking water (140 mg/kg bw per day) for two weeks, altered small and large intestinal morphology, infiltration of lamina propria in the small intestine with macrophages, higher systemic levels of inflammation markers (C-reactive protein and middle molecules), changes in the lipid order of the phospholipid bilayer in the cell membranes of leukocytes, as well as activation of apoptosis in these cells, were demonstrated (Pogozhykh et al. Citation2021). In the same rat model, low-grade inflammation, as indicated by increased T lymphocytes and macrophages in the colonic lamina propria, was induced (Onishchenko et al. Citation2022). Among the endothelial-mesenchymal transition (EMT) markers, fascin and vimentin were overexpressed in both stromal and epithelial cells, while E-cadherin was upregulated in the stroma and downregulated in epithelia.
In rats administered CGN in drinking water for four weeks, CGN reduced the protective properties of mucin, accelerated death of epithelial cells and led to interstitial inflammation in the small intestine, together with decreased activity of poly (ADP-ribose) polymerase (PARP), increased activity of apoptosis signal-regulating kinase 1 (ASK-1) and high percentage of DNA fragmentation in the small intestine (Gubina-Vakyulyk et al. Citation2015). Increased death of epithelial cells was accompanied by activation of proliferation, but not sufficient for complete regeneration of the epithelial cover of villi. Similarly treated rats with gastroenterocolitis showed activation of apoptosis in the intestinal epithelium, with changes in the polar regions of the enterocyte membranes, but not in more hydrophobic regions (Tkachenko et al. Citation2018a).
In rats given 5, 20 or 50 g ι-CGN per kg diet (0.5, 2 and 5%) for 30 days, all doses caused similar cecal enlargement and decrease in concentration of bacteria per gram of cecal content (Mallett et al. Citation1985). Rats fed the highest dose showed a significant decrease in enterobacteria, staphylococci, streptococci and total facultative anaerobes as well as in lactobacilli and the total microscopic count. Proportions of Enterobacteria and lactobacilli were relatively increased, while the proportion of staphylococci was decreased. There were no differences in degree of cecal enlargement and decrease in cecal bacterial numbers and concentrations between κ-, ι- or λ-CGN.
Intestinal permeability was determined indirectly by orally administration to rats of a poorly absorbed dye, phenol red, and measuring its recovery in feces and urine (Shiau and Chang Citation1986). CGN type I (80% κ-CGN, 20% λ-CGN) or CGN type II (ι-CGN) were fed as 5 and 15% of the diet for 31 days. Both CGN increased permeability dose-dependently, with some differences, with adaptation apparently taken place at four weeks. The urinary excretion 96 h after administration in drinking water of two polyethylene glycol markers was measured in rats after feeding CGN type II (20%) for 4 weeks (Elsenhans and Caspary Citation1989). The ratio of PEG 4000 to PEG 900 in the urine increased after CGN feeding, showing that CGN could influence the intestinal permeability of larger molecules.
The promoter effect of native CGN (Viscarin 402) upon colon carcinogenesis induced by AOM or methylnitrosourea (MNU) was studied in rats (Watanabe et al. Citation1978). CGN enhanced the incidence of colonic tumors in the AOM- and MNU-treated rats. No tumors were found with control diet, but one of 15 rats (7%) had a colonic adenoma after CGN diet without carcinogen. To study carcinogenic effects of native CGN (Gelcarin, largely composed of κ-components and also λ-CGN (MW ca. 800 kDa), doses of 0.5, 2.5 or 5% (average daily intake 360, 1998 and 4022 mg/kg, respectively) were given in the diet to rats for life (Rustia et al. Citation1980). A trend toward increased incidence of benign mammary tumors in females and testicular neoplasms in males occurred at the 2.5% CGN dose, however, no statistically significant increases in tumor incidences were seen. The tumors of the gastrointestinal tracts of the rats were one leiomyoma of the intestine and three squamous cell papillomas of the forestomach with the middle CGN dose. In conclusion, native CGN had no carcinogenic effects on rats throughout their life spans. The initiating and promoting effects of native κ-CGN (MW 345 kDa, type I) were studied in conventional rats and germ-free rats gavaged with human Bacteroides sp. and human fecal samples from healthy children given CGN-containing desserts (Taché et al. Citation2000). The initiating effect of κ-CGN was studied by scoring preneoplastic aberrant crypt foci (ACF) in the colon of rats given κ-CGN (10% gel and 0.25% liquid in drinking water) for eight days. κ-CGN alone did not initiate ACF. The promoting effect of κ-CGN was studied by comparing the multiplicity of ACF (number of aberrant crypts/focus) in rats receiving drinking water (controls), liquid κ-CGN (0.25% in water) or κ-CGN gel (2.5% in water) for 100 days, after tumor initiation with AOM. In conventional rats, 2.5% κ-CGN gel promoted growth of ACF, whereas 0.25% liquid κ-CGN did not. In rats with human microflora, no promoting effect of 2.5% κ-CGN gel was observed. This indicated that rat microflora, but not human gut microflora, might be involved in colonic tumor promotion by κ-CGN.
The effects of ι-CGN were studied in rats given diets supplemented with 5% of the seaweed Sarconema filiforme for eight weeks (du Preez et al. Citation2020). The rats had lower, although not significant, food intake and body weight with S. filiforme. The S. filiforme supplementation also modulated gut microbiota without changing the Firmicutes to Bacteroidetes ratio. In rats fed a high-fat diet and injected with streptozotocin to induce diabetes, supplementation with 270 mg/kg unspecified CGN did not affect glycemic control-related parameters: fasting blood glucose, glycosylated serum proteins, oral glucose tolerance test, insulin and the homeostasis model of assessment of insulin resistance (HOMA-IR)) (Nie et al. Citation2021). However, CGN increased serum glucagon-like peptide (GLP)-1, decreased leptin and increased some unsaturated fatty acids in diabetic rats.
Fifteen publications reported studies on mice using native CGN. Mice given 5, 20 or 50 g of ι-CGN per kg diet for 30 days showed cecal enlargement and decreased concentration of bacteria per gram of cecal content (Mallett et al. Citation1985). Two % CGN in drinking water for 4 weeks induced a marked diffuse inflammatory response in the colon, associated with infiltration of leucocytes and macrophages, and a two-fold increase in prostaglandins in mice (Zijlstra et al. Citation1992).
Both systemic and intestinal inflammatory responses of undegraded κ-, λ-CGN in drinking water (total CGN intake ca. 11.5 mg/30 g mouse) for 30 days were examined in B-cell lymphoma/leukemia (Bcl10) wild-type, heterozygous and null mice (Bhattacharyya et al. Citation2013). Adverse effects in caecum and intestines were seen in the wild-type mouse, but not in Bcl10 null mice, consistent with reduced inflammation in the absence of Bcl10. Fecal calprotectin and circulating keratinocyte chemokine (KC), nuclear RelA and RelB, phospho(Thr559)-NF-κB-inducing kinase (NIK) and phospho(Ser36)-IκBα in the colonic epithelial cells were significantly lower in the CGN-treated Bcl10 null mice than in controls. IL10-deficient mice exposed to CGN in a germ-free environment showed an increase in activation of the canonical pathway of NF-κB (RelA) activation, but without increase in RelB or phospho-Bcl10. In mice given undegraded CGN in drinking water for four weeks (Bhattacharyya et al. Citation2014b), CGN decreased arylsulfatase B activity in colonic epithelial cells, with subsequent effects on C4S, galectin-3, Sp1 and Wnt9A, which may have significant effects on Wnt-initiated signaling and related cellular processes.
Mice were treated with κ-CGN in doses of 1.7, 8.3 and 41.7 mg/kg bw by for 14 days prior to the administration of 2,4,6-trinitrobenzene sulfonic acid (TNBS) to test effects of CGN on TNBS-induced inflammation (Wu et al. Citation2016a). κ-CGN pretreatment increased body weight loss, mortality rate and colonic inflammation, associated with oxidative stress and activation of the Toll-like receptor (TLR)4-NF-κB and MAPK/ERK1/2 pathway. In a similar study, κ-CGN administration prior to induction of colitis by oxazolone showed increased body weight loss, decreased survival and aggravated colonic inflammation (Wu et al. Citation2016b). Secretion of IL-4, IL-10, TNF-α and IL-6 also increased after κ-CGN exposure, associated with an activation of the TLR4-NF-κB pathway, a decreased ratio of regulatory T cells and the induction of Th2-dependent immune responses.
In another inflammation model, mice given κ-CGN (1.7, 8.3 and 41.7 mg/kg diet, average MW 1000 kDa) and then later were inoculated with Citrobacter freundii bacteria, the dose-dependent decreases in body weight and survival were higher with CGN than without, indicating that CGN aggravated the bacteria-induced gut inflammation (Wu et al. Citation2017; Wu et al. Citation2021). Mice with high dietary λ-CGN consumption showed altered colonic microbiota composition that resulted in degradation of the colonic mucus layer, increased fecal LPS level and decreased short-chain fatty acids (SCFA), affecting integrity of the intestinal barrier. These effects could be reproduced in germ-free mice by fecal transplantation from mice given the high λ-CGN dose, but not in germ-free mice fed the high λ-CGN dose. A similar study with κ-CGN (average MW 198 kDa) in mice did not cause significant inflammatory symptoms, but it reduced SCFA and decreased thickness of the mucus layer by altering microbiota composition (Wu et al. Citation2022). Administration of the pathogenic bacterium Citrobacter rodentium further aggravated the inflammation and mucosal damage in the presence of κ-CGN. Mucus layer degradation and altered SCFA levels could be reproduced by fecal transplantation from κ-CGN-fed mice, but not from germ-free κ-CGN-fed mice. The results suggested that κ-CGN may not be directly inflammatory but creates an environment that favors inflammation by perturbation of gut microbiota composition and metabolism, and facilitates expansion of pathogens.
The diet appears to modulate the effects of CGN. In mice exposed to 0.02% κ-CGN together with dairy cream, no adverse outcomes were seen on lipid metabolism, intestinal permeability or liver damage, but a higher expression of endoplasmic reticulum (ER) stress genes in the colon was observed (Milard et al. Citation2018). κ-CGN (average MW 640.80 ± 11.53 kDa) reduced colon length and induced more serious deepening of the crypts in mice on a high-sucrose diet (Gao et al. Citation2022). κ-CGN on a high-sucrose/high-salt diet induced more serious reduction in goblet cells and increased permeability of the intestines, and reduced anti-inflammatory bacteria and increased harmful bacteria, associated with decrease of anti-inflammatory colonic metabolites.
CGN may affect metabolic parameters. Mi et al. (Citation2020) found that a 45% fat diet with 0.5% κ-CGN (average MW 365 kDa) and normal drinking water increased the general disease activity index (DAI), myeloperoxidase (MPO) activity, a marker for infiltration of inflammatory cells, and mRNA expression of TLR4 in colon of mice, whereas a 45% fat diet with 5% κ-CGN, but no κ-CGN in drinking water, had no such effects. No signs of colitis were observed on a 10% fat diet regardless of the mode of vehicle used for κ-CGN (diet or drinking water), except that 5% κ-CGN in 10% fat diet and normal drinking water increased the DAI. Moreover, the κ-CGN-induced colitis on high-fat diet was correlated with increases of the harmful bacteria Alistipes finegoldii and Bacteroides acidifaciens, whereas the anti-inflammatory bacterium Akkermansia muciniphila was increased in the group given 10% fat diet with 0.5% κ-CGN in normal drinking water. Hence, the inflammatory property of CGN is influenced greatly by its intake form via modification of host intestinal microecology. In a further study, in obese mice given 5% κ-CGN for 6 weeks, genes involved in the inflammatory pathways or tight junction protein encoding were not significantly dysregulated by κ-CGN (Zhang et al. Citation2021). However, κ-CGN decreased expression of genes for adipocytokines, lipogenesis, lipid absorption and transport, and increased expression of genes for of adipolysis and oxidation. The food-grade κ-CGN was not absorbed or significantly degraded in the digestive tract of the obese mice. In another study on mice fed a 60% fat diet, 0.2% or 1% κ-CGN significantly reduced body weight gain, fat mass, adipocyte size, fasting blood glucose and blood lipids (Wang et al. Citation2021). κ-CGN improved high-fat diet-induced obesity by the AMPK and PPARγ signaling pathway. The level of TNF-α and LPS in the low dose CGN group, but not in the high dose group, was significantly lower than that in the high-fat diet group. The abundance of Firmicutes, Proteobacteria, Lachnospiraceae and Desulfovibrionaceae was positively correlated with body weight, fasting blood glucose, serum low density lipoprotein cholesterol (LDL-c), total cholesterol (TC) and triglyceride levels, whereas Bacteroidetes were negatively correlated with LDL-c and TC levels, while positively with fasting blood glucose.
Comparable activities of colitis were induced by κ-, ι- and λ-CGN given in drinking water for six weeks to mice (Shang et al. Citation2017). All three forms of CGN decreased the anti-inflammatory bacterium Akkermansia muciniphila in the gut microbiota.
Three publications on guinea pigs compared native and undegraded CGN (Watt and Marcus Citation1969; Grasso et al. Citation1973; Pintauro and Gilbert Citation1990) and are described above in Section 3.2.2.1. There were no publications studying native CGN in rabbits or in ferrets.
There were two publications on hamsters using native CGN. Hamsters given 5, 20 or 50 g of ι-CGN per kg diet for 30 days showed cecal enlargement and decreased concentration of bacteria per gram of cecal content (Mallett et al. Citation1985). To study carcinogenic effects of native CGN (Gelcarin), largely composed of κ- and also λ-CGN (MW ca. 800 000), doses of 0.5, 2.5 or 5% in the diet (average daily intake 370, 2162 and 3719 mg/kg, respectively) were given to hamsters for life (Rustia et al. Citation1980). The tumors of the gastrointestinal tracts of the hamsters were one squamous cell papilloma in each treated group, which were not significantly different from controls. Thus, native CGN had no carcinogenic effects on hamsters exposed throughout their life spans.
One publication studied pigs administered native CGN (average MW 200 kDa) orally in doses of 50, 200 and 500 mg/kg bw per day for 12 weeks (Poulsen Citation1973). No effects of CGN were seen on behavior, body weight gain, feed utilization, haematology, blood chemistry, urine analysis or organ weights. No UC or erosions of the mucous membrane of caecum and colon were observed. However, CGN decreased the total counts of aerobic bacteria in the colon and rectum, and the number of Lactobacilli in the rectum.
Two publications compared native and degraded CGN in Rhesus monkeys (Benitz et al. Citation1973; Mankes and Abraham Citation1975) and are described above in Section 3.2.2.1.
3.2.3. In vitro studies
In total, 33 publications with in vitro experiments reported adverse effects on the intestines of CGN. Among the in vitro studies, 8 studies were performed with unspecified native CGN, whereas there were 19, 10 and 16 publications with κ-, ι- and λ-CGN, respectively. The numbers of publications on in vitro studies that had used degraded CGN were 14, whereas 26 had studied native (non-degraded CGN). Seven publications studied both degraded and native CGN in vitro.
Six publications were studying microbiota from human feces (three on degraded, three on native CGN), whereas the majority (21) of the publications reported studies on human primary cells or cell lines (three on degraded, 12 on native, seven on both types of CGN), some also including non-human mammalian cells. Four publications studied mammalian fecal microbiota (two on degraded, two on native CGN) and eight publications used mammalian primary cells or cell lines (three on degraded, five on native CGN).
3.2.3.1. In vitro studies on degraded CGN
Seven in vitro studies were using degraded CGN only. In addition, seven publications included data on both degraded and native CGN, described in this and/or the section on native CGN.
When fermentation of CGN, i.e. breakdown of carbohydrates by human fecal microbiota that cannot be digested by the body’s enzymes, was screened in an in vitro batch fermentation system, seven of eight human colonic microbiota fecal samples showed that high MW κ-CGN (450 or 100 kDa) remained undegraded, whereas low MW κ-CGN, i.e. κ-CGN oligosaccharides (KCO, ∼4.5 kDa), were degraded (Yin et al. Citation2021). Sulfate groups were not removed during degradation. The concentrations of propionic and butyric acids were significantly increased, and pH decreased, after KCO fermentation. Bacteroides xylanisolvens and Escherichia coli isolates from the fecal samples appeared to degrade KCO synergistically, with B. xylanisolvens being the primary degrading bacteria. Studies of in vitro fermentation by human gut microbiota of two κ-CGN oligosaccharides (MW <3000 kDa with different degree of polymerization (DP)) obtained from simulated gastric digestion, called KO3 and KO6, showed that both were readily degraded (Sun et al. Citation2019). Both promoted pro-inflammatory bacteria Prevotella, while inhibited anti-inflammatory bacteria Bacteroides and Parabacteroides. The KO3 with larger DP improved SCFA production and strongly increased the growth of Bifidobacterium and Lactobacillius, whereas KO6 with smaller DP reduced SCFA production and strongly increased the abundance of mucin-producing bacteria Prevotellaceae. Another study also showed that κ-CGN oligosaccharides were degraded by Bacteroides xylanisolvens and E. coli isolated from human gut microbiota (Li et al. Citation2017).
Human peripheral blood monocytes (PBM) and human THP-1 monocytic cells were cultured in the presence of either 10 or 40 kDa degraded CGN prepared from native ι-CGN in vitro (Benard et al. Citation2010). Degraded CGN inhibited THP-1 cell proliferation and arrested the cells in G1 phase. Degraded CGN also increased Intercellular Adhesion Molecule 1 (ICAM-1) expression in both PBM and THP-1 cells, with the strongest effect seen with the 40 kDa degraded CGN, and stimulated monocyte aggregation. Degraded CGN also stimulated expression and secretion of TNF-α in both cell types. All these effects involved NF-kB activation and indicated that degraded CGN led to an inflammatory phenotype of the monocytes.
Associated with inflammatory effect on human colon cancer HT29 cells, supernatants (50, 100 and 200 µl/ml) from fermentation of κ-CGN oligosaccharides KO3 and KO6 promoted secretions of IL-1β, TNF-α, secretory IgA and mucin2 in a dose-dependent way, indicating that smaller κ-CGN oligosaccharides might have stronger influence on inflammation (Sun et al. Citation2019).
Native λ-CGN activated the Wnt/β-catenin signaling pathway, shown by increased expression of Wnt9A, suppressed Dickkopf 3 and RHOU genes, and increased accumulation of β-catenin, and suppressed the expression and secretion of bone morphogenetic protein-4 (BMP4) in human NCM460 cells (Bhattacharyya et al. Citation2007). This suggested a role of λ-CGN in malignant transformation in the human intestine. Native and degraded κ-CGN had similar reducing effect on BMP4 and both had lower effect on BMP4 reduction than native λ-CGN.
In NCM460 cells and primary human colonic epithelial cells that were exposed to low levels (1–10 mg/L) of undegraded high MW CGN for 1–8 days, increased cell death, reduced cell proliferation and cell cycle arrest were reported (Bhattacharyya et al. Citation2008a). After 6–8 days of CGN exposure, the percentage of cells reentering G0–G1 decreased and the percentages of cells in S and G2-M phases increased. Increases in activated p53, p21 and p15 followed CGN exposure, consistent with CGN-induced cell cycle arrest. However, the antiproliferative response, as decline in BrdU incorporation, occurred after exposure to both undegraded κ-, ι- and λ-CGN as well as degraded κ-CGN. No evidence of apoptosis was seen, but the data was consistent with necrosis.
Shorter, degraded κ-CGNs induced higher IL-8 levels than longer disaccharides (Bhattacharyya et al. Citation2010c). Hydrolysis of different forms of high MW CGN (κ-, ι-, λ-CGN) by the enzyme α-1→(3,6)-galactosidase reduced increases in IL-8 and BCL10 in NCM460 cells, but α-1→6-galactosidase, β-1→4-galactosidase and β-1→3,6-galactosidase had no effect. In contrast, specific κ-CGNases or ι-CGNases, which hydrolyze β-1,4-galactosidic bonds, increased IL-8 and BCL10, because of increased exposure of the immunogenic α-1→3-galactosidic epitope of CGN to TLR4. Thus, the CGN-induced innate immune response in NCM460 cells was modified by enzymes that hydrolyze distinct galactosidic bonds.
Food grade κ-, ι- and λ-CGN were mixed with milk (whey), soy or egg protein isolates, and tested in a semi-dynamic in vitro digestion model, which showed varying levels of disturbance of gastric digestive proteolysis and a significant decrease in pepsin activity (Fahoum et al. Citation2017). Further, in human Caco-2 cells, samples of physiologically digested CGN affected the epithelial barrier function, including redistribution of the tight-junction protein Zo-1, changes in cellular F-actin architecture and increased monolayer permeability of macromolecules. Moreover, CGN also increased the pro-inflammatory IL-8 receptor CXC Motif Chemokine Receptor 1 (CXCR1).
Degraded λ-CGN with MW of 10–40 kDa induced inflammation via both NF-κB and activation protein (AP)-1, and increased the inflammatory effects of LPS though AP-1 activation in the human THP-1-derived macrophage cell line and the murine RAW264.7 macrophage cell line (Chen et al. Citation2014). Degraded λ-CGN was more effective than native λ-CGN in the activation of macrophages to secrete TNF-α.
Degraded and undegraded CGN of several varieties were compared in several human cell lines (Ariffin et al. Citation2014). Food grade κ-CGN (FGKC), dried sheet κ-CGN (DKC), commercial grade κ-CGN (CGKC), food grade ι-CGN (FGIC) and commercial grade ι-CGN (CGIC) were dissolved in hydrochloric acid and water to prepare degraded and undegraded CGN, respectively, with concentration range of 62.5–2000.0 μg/ml. FGKC and DKC were PES (E 407a). Degraded FGKC, DKC and CGKC reached the concentration inhibiting 50% of cell viability (IC50) in 24, 48 and 72 h in Caco-2 (human epithelial colorectal adenocarcinoma), FHs 74 Int (normal human small intestinal), HepG2 (human hepatocellular carcinoma) and Fa2N-4 (immortalized human hepatocyte) cell lines. Degraded FGIC and CGIC were only toxic in Fa2N-4 cells. Apoptosis was demonstrated in degraded κ-CGN treated Caco-2, FHs 74 Int, HepG2 and Fa2N-4 cells. Chromatin condensation and nuclear fragmentation were seen in Caco-2 and HepG2 cell, whereas DNA ladder was only found in HepG2 cells. Degraded κ-CGN also inactivated PCNA, Ki-67 and survivin genes in HepG2. On the other hand, cells treated with undegraded FGKC, DKC, CGKC, FGIC and CGIC showed no cytotoxic effect after the same analyses as with degraded CGN.
PGN (MW <20 kDa) at concentrations of 1 and 10 µg/ml showed a 3.5- and 7-fold induction of IL-8 in human HepG2 cells in the absence of serum protein, but no induction of IL-8 was observed when serum protein was present (McKim et al. Citation2016).
Partially hydrolysed (degraded) CGN (up to 1.5 mg/ml) retarded growth and caused cell death in the rat ileum epithelial cell line IEC18, as well as inhibited DNA synthesis, disrupted cellular junctions and subsequently caused cell membrane injury (up to 5 mg/ml) (Ling et al. Citation1988).
Pools of feces containing ten bacterial strains obtained from conventional guinea pigs administered 5% degraded CGN caused cytotoxic responses in Vero cells (African green monkey kidney cells) and WI-38 cells (human lung fibroblasts), increased presence of long-chain fatty acids (LCFA) in the cell culture supernatants and increased chemotactic activity (Onderdonk et al. Citation1981).
κ-CGN oligosaccharides (KCO) (MW 1-7 kDa) were obtained by enzymatic hydrolysis (Han et al. Citation2019). Culture medium was supplemented with KCO and fermented by pig fecal microbiota in vitro. KCO increased the concentration of a total of seven SCFAs (only butyric acid significantly). KCO had no obvious effects on fecal microbiota structure after the fermentation. However, the bacterial populations at the phylum level showed a mild increase in the abundance of Bacteroidetes in KCO culture and a total of 50 taxa displayed significant differences vs. controls. KCO increased the abundance of butyric acid producing bacteria, such as Coprococcus_1, Ruminococcaceae and Roseburia, and opportunistic pathogenic bacteria, including Peptococcus, Clostridium and Bacteroides.
3.2.3.2. In vitro studies on native CGN
The majority of the in vitro studies had only used native (undegraded) CGN (19). In addition, seven publications compared degraded and native CGN, described also in Section 3.2.3.1. CGN (κ-, ι- and λ-) had strong detrimental effect on human microbiota, using feces obtained from a healthy person maintained ex vivo in the dynamic stable MiniBioReactor Array model under anaerobic conditions (Naimi et al. Citation2021). CGN altered microbiota density and composition, and induced bioactive levels of flagellin in human embryonic kidney HEK cells with this donor. In a study of in vitro batch fecal fermentation using samples from 13 young healthy donors (seven women and six men), κ-CGN had no effects on production of any SCFA or branch chain fatty acids (BCFA), but increased the abundance of Escherichia/Shigella and inhibited the growth of f Bifidobacterium in the human gut microbiome (Gerasimidis et al. Citation2020). Effects on CGN in fecal samples from 20 healthy persons and 10 UC patients (4 men and 6 women, adults) were studied by Gibson et al. (Citation1991). Fermentation of CGN stimulated sulfide production in the colitis patients, and increased growth and activities of sulfate-reducing bacteria, which were higher in the healthy controls.
Studies in the human colonocyte cell line NCM460 cells and human colonic epithelial cell line HT-29 showed that λ-CGN increased expression of colonic Wnt9A through Sp-1-mediated transcriptional effects involving arylsulfatase B, chondroitin 4-sulfate and galectin-3 (Bhattacharyya et al. Citation2014b).
Type IV λ-CGN, essentially a pure λ form, increased Bcl10, IκBα phosphorylation, total and nuclear NF-κB and secretion of IL-8 in the NCM460 cell line (1 µg/ml) and ex vivo normal human colon epithelial tissues (10 µg/ml), implicating this signaling pathway in intestinal inflammation induced by CGN (Borthakur et al. Citation2007). When comparing IL-8 secretion between the native κ-, ι- and λ-CGNs (0.1, 1 and 10 µg/ml) in NCM460 cells, the response was highest for λ-, next for κ- and then ι-CGN. Further, in NCM460 and HT-29 cell lines, it was shown that NF-κB binding to the BCL10 promoter involved the components RelA and RelB, and could lead to prolonged activation of the λ-CGN-induced inflammation by a transcriptional mechanism involving a NF-κB-BCL10 loop (Borthakur et al. Citation2012).
λ-CGN increased production of reactive oxygen species (ROS) in NCM460 cells and affected the Bcl10-mediated pathway, which involves the innate immune response, and was responsible for the majority of the effects of CGN in the inflammatory pathway leading to IL-8 (Bhattacharyya et al. Citation2008b). Both the ROS and Bcl10 pathways increased NF-κB nuclear translocation and IL-8 secretion. The Bcl10-NFκB-IL-8 inflammatory pathway affected by CGN in NCM460 cells was mediated by TLR4, indicating that CGN-induced inflammation in human colonocytes proceeds via innate immunity (Bhattacharyya et al. Citation2008c). IL-8 secretion in response to κ-, ι- and λ-CGN after treatment with carrageenase and galactosidase enzymes in NCM460 cells was compared and it was found that λ-CGN produced a higher IL-8 response than κ-CGN or ι-CGN (Bhattacharyya et al. Citation2010c). Another study suggested that λ-CGN drives both the NCM460 and mouse embryonic fibroblast (MEF) cell lines stimulated with TNF-α, a potent proinflammatory cytokine, towards inflammation rather than towards apoptotic cell death (Bhattacharyya et al. Citation2010b). Lastly, in NCM460 cells, an increase in nuclear RelA following exposure to λ-CGN was partially inhibited by exogenous IL-10 (the canonical pathway), whereas the increase in nuclear RelB was unaffected by exogenous IL-10 (the non-canonical pathway) (Bhattacharyya et al. Citation2013). In human HT-29 cells, κ-CGN did not stimulate IL-8 alone, but increased LPS-induced IL-8 secretion via the Bcl10-NFκB pathway and acted in synergy with LPS to increase the expression of MD-2 and CD14 (Wu et al. Citation2017).
A CGN food grade blend, separate κ-, λ- or ι-CGN, or a CGN non-gelling mix of κ- and λ-CGN were reported not to bind to and activate TLR4 signaling or reduce the LPS response when co-administered, in a HEK293 (immortalized human embryonic kidney cells)-TLR4 reporter cell-line model (McKim et al. Citation2015). The various forms of CGN were reported to bind tightly to serum proteins. These results were different from results of other publications, and it should be noted that the work was funded by FMC Corporation, a producer of CGN.
Several publications studied effects of CGN on the intestinal barrier and its permeability. Choi et al. (Citation2012) studied effects of CGN on the pro-inflammatory transcription factors NF-κB and early growth response gene 1 (EGR-1), evaluated in terms of human intestinal epithelial barrier integrity using the human cell lines HT-29, HCT-8 and Caco-2. Both pro-inflammatory transcription factors were elevated by CGN, but only NF-κB activation was shown to be involved in the induction of IL-8. The integrity of the in vitro epithelial cell monolayer under the CGN exposure was maintained by both activated NF-κB and EGR-1. Suppression of NF-κB or EGR-1 aggravated barrier disruption by CGN, which was associated with reduced gene expression of tight junction component zonula occludens-1 (Zo-1) and its irregular localization in the epithelial monolayer. Further, CGN also upregulated the expression of the macrophage inhibitory cytokine 1 (MIC-1) that promoted epithelial cell apoptosis. The counterbalance between MIC-1 and the pro-survival protein activating transcription factor 3 (ATF3) appeared critical for deciding the fate of enterocytes under CGN exposure, when studied in human HCT-8 and HCT-116 intestinal epithelial cells, CMT-93 mouse colon cancer cells and primary mouse intestinal epithelial cells (Choi et al. Citation2014).
In an in vitro co-culture system of human intestinal epithelial Caco-2 cells and human macrophage-like THP-1 cells, κ-CGN resulted in apoptosis, reduced transepithelial electrical resistance of the Caco-2 cell monolayer and increased secretion of TNF-α, IL-1β or IL-6, depending on CGN concentration (Jiang et al. Citation2013).
CGN’s physiochemical properties, such as zeta-potential, may affect gastric proteolysis, potentially leading to reduced accessibility of proteins, peptides and amino acids in the intestines (Fahoum et al. Citation2017). Different commercial CGN samples varied in their zeta-potential, arising from their different degree of sulfation, throughout a range of pH values as well as with varying CaCl2 concentrations Throughout the tested pH range, κ-CGN was the least negatively charged, ι-CGN was intermediately charged, while λ-CGN was the most negatively charged of the CGN samples at typical luminal concentrations of CaCl2.
κ-, λ- or ι-CGN exposure reduced the activity of several sulfatases and increased N-acetylgalactosamine-containing glycosaminoglycan (GAG) content and their disaccharide composition in several human and mammalian intestinal and mammary epithelial cell lines (Yang et al. Citation2012). The results suggested that CGN inhibition of sulfatase activity led to re-distribution of the cellular GAG composition with increase in di-sulfated chondroitin sulfate, with potential consequences for cell structure and function.
In a standard Caco-2 absorption model, CGN was reported not to affect permeability or cytotoxicity (McKim et al. Citation2016). Further, in the two human intestinal cell lines HT-29 and HCT-8, CGN did not induce IL-8, IL-6 or monocyte chemoattractant protein-1 (MCP-1) (CCL2) or produced cellular toxicity. The TLR4 agonist LPS produced weak induction of IL-8 in HT-29 cells and no induction in HCT-8 cells. No effect on oxidative stress was observed in HT-29 cells with κ-CGN. In the human liver cell line HepG2, λ-CGN had no effect on the expression of IL-8, IL-6 or MCP-1. These results were different from results of other publications, and it should be noted that the work was funded in part by FMC Corporation, a producer of CGN, and the International Food Additives Council (IFAC), a global association representing manufacturers and end-users of food ingredients, including food additives.
Among the publications on non-human microbiota, Harmuth-Hoene and Schwerdtfeger (Citation1979) found that in rat feces, dry matter digestibility and apparent protein digestibility, as well as nitrogen retention and trypsin inhibition, were decreased with 10% CGN in the diet during eight days. These effects were attributed to the partial breakdown by intestinal microbiota and/or their ability to partially inhibit the activity of proteolytic enzymes in the gastrointestinal tract.
In an ex vivo-in vitro experiment, the proliferative responses of splenocytes from rats given 2% λ-CGN in drinking water to the mitogens concanavalin A (Con A, to stimulate T cells), lipopolysaccharide (LPS, to stimulate B cells) and pokeweed mitogen (PWM, to stimulate both T and B cells) were significantly diminished compared with splenocytes from control rats (Pricolo et al. Citation1996). The proliferative responses were fully restored with a specific inhibitor of nitric oxide synthase, involving nitric oxide in the immunosuppression.
In rats given a fibre-free purified diet supplemented with 5, 20 or 50 g of ι-CGN per kg diet for 30 days, all doses caused reduced activities of azoreductase, β-glucosidase, β-glucuronidase, nitrate reductase and total nitroreductase per gram of cecal content in vitro (Mallett et al. Citation1985). Mice treated similarly also had decreased activities of β-glucuronidase and total nitroreductase, whereas hamsters had increased activities of β-glucuronidase and nitrate reductase, but decreased activity of azoreductase and total nitroreductase per gram of cecal content.
λ-CGN affected the Bcl10-NFκB-IL-8 inflammatory pathway via TLR4, since binding of λ-CGN to TLR4 was markedly reduced in RAW 264.7 mouse macrophages after exposure with TLR4 blocking antibody (HTA-125), whereas binding to 10ScNCr/23 mouse macrophages, which are deficient in the genetic locus for TLR4, was absent (Bhattacharyya et al. Citation2008c).
The four publications comparing native and degraded CGN are described in Section 3.2.3.1. (Bhattacharyya et al. Citation2007; Bhattacharyya et al. Citation2008a; Ariffin et al. Citation2014; Chen et al. Citation2014). Three other publications also included data on both degraded and native CGN, and are present in both sections (Bhattacharyya et al. Citation2010c; McKim et al. (Citation2016); Fahoum et al. Citation2017).
3.2.4. Hypotheses on adverse effects of CGN
Many publications examined more than one specific hypothesis or had several objectives for their work, and many publications examined the same hypotheses or effects, thus, a complete list would be very repetitive. The number of research publications studying degraded or native CGN in relation to various hypotheses on adverse effects is shown in . Most of the hypotheses were studied both with degraded and native CGN, with some differences. The number of studies on inflammation was higher with degraded (43) than native CGN (35), reflecting the use of degraded CGN as an experimental inflammation model. The number of studies of native CGN was higher than of degraded CGN for most of the other endpoints, especially permeability of the intestinal barrier (10 vs. 6), effects on the gut microbiome (16 vs. 11), metabolic effects, such as obesity and metabolic syndrome (9 vs. 3), and effects on cell proliferation, apoptosis or cell cycle (9 vs. 3), respectively, i.e. reflecting the more wide-spread interest in native CGN in relation to different health effects.
3.2.5. Data relevant for exposure
Twenty publications had some information relevant for exposure to food grade CGN. In reviews, CGN (E 407) was reported in 4% of all food products in the food supply in EU (Cox et al. Citation2021) and the range of content of CGN in some commonly consumed food groups was reported to vary from 0.01 g to 4 g per 100 g food (Tobacman Citation2001).
The estimated intake of CGN has increased over time. In reviews by David et al. (Citation2018) and Liao et al. (Citation2021), it was reported that in a FDA report (1972), the daily CGN consumption in USA was reported to be 45 mg per day, an industrial survey on the use of food additives (1977) estimated CGN intake of 100 mg per day for individuals >2 years, and the CGN intake was estimated to rise to 2.5 mg per day in 1989 and further up to 7.7 g per day (0–1 mg/kg bw) in South Florida in 2003. In other reviews, the estimated range of intake of CGN reported for the general population was 18–42 mg/kg bw per day and for infants given formula 6–160 mg/kg bw per day (Weiner Citation2014) and the average daily intake of CGN in a Western diet (USA) was estimated to be 20 to 200 mg/day (Martino et al. Citation2017). In an abstract, it was reported that CGN is consumed at levels of about 250 mg/day in the average diet (Bhattacharyya and Tobacman Citation2012).
In a review, it was reported that CGN was consumed by 77.5% of the consumers among 106 489 adults in the French NutriNet-Santé cohort study (2009–2020) (Chazelas et al. Citation2021). Mean intake per person per day was approximately 46 mg per day. The mean ± standard deviation (SD) intake was 0.71 ± 1.07 mg/kg bw per day and the 95th percentile intake was 2.70 mg/kg bw per day.
CGN or PES were not considered to be used in combination in the same foods and their exposure could therefore be assessed independently (EFSA Citation2018a). Several different exposure scenarios were used in the calculations of their exposure by EFSA. The mean maximum level exposure to CGN and PES was estimated based on the maximum reported use levels provided by industry, since CGN and PES are authorized as QS in almost all FC, and ranged from 21.8 mg/kg bw per day in the infants to 581.4 mg/kg bw per day in toddlers (EFSA Citation2018a). The 95th percentile of exposure to CGN and PES ranged from 58.1 mg/kg bw per day in the elderly to 999.1 mg/kg bw per day in children. The Panel further concluded that in the refined brand-loyal exposure scenario the exposure estimates exceeded, by up to about 10-fold, the temporary ADI of 75 mg/kg bw per day at the 95th percentile for all population groups and at the mean for all population groups except for infants and the elderly, which may be a safety concern (EFSA Citation2018a). In a reply to a comment, David et al. (Citation2019) presented a figure of total levels of estimated exposure to CGN from foods in various age groups based on an EFSA survey of European countries (referring to Supplementary material in EFSA Citation2018a) where the exposures in mg/kg bw per day were exceeding the ADI for toddlers, other children and adolescents.
Average exposure to unspecified CGN in children of both sex (n = 138, mean age 14.2 ± 2.8 years) with CD was quantified from three 24-h recall days over two years obtained by telephone (Lee et al. Citation2018). A total of 1325 unique foods were recorded and 5% of the foods contained CGN. Mean ± SD frequencies of exposures per day were 0.58 ± 0.63 (range 0–3.67) of CGN. The frequency of exposure to CGN in 20 patients with CD using 7-day food diaries analyzed for habitual intake was 0.09 ± 0.15 (mean ± SD) per day (Sandall et al. Citation2020).
In an abstract, the exposures to selected food additives per day in children with IBD and the association with IBD relapse were reported (Ho et al. Citation2021). In this single-centre observational cohort study, children 8–18 years of age with CD or UC in remission were enrolled. Detailed 24-h dietary recalls were collected at baseline, between 3–6, 7–10, 11–14 months and at time of relapse. A food list was generated, and a database of product ingredient lists was evaluated to quantify the frequency of dietary exposure to 10 food additives, including CGN (and CMC). In total, 22 participants with IBD (15 CD, 7 UC) were enrolled, of which six later dropped out. Mean age was 12.8 ± 3.5 years, with average disease duration 2.2 ± 1.9 years. Disease relapse occurred in 5/16 (31%) of participants; 4 with CD and 1 with UC. There were no statistical differences between number of exposures per day or intake of food additives between the remission and relapse groups.
An abstract reported emulsifier exposure using baseline dietary data from adults and children with IBD enrolled in the Australian Inflammatory Bowel Disease Microbiome (AIM) study, a multicentre, prospective, longitudinal cohort study (Reveley et al. Citation2022). Baseline dietary intake was measured using a 3-day prospective food diary. Food products were evaluated for the presence of six emulsifiers, including CGN (and CMC), using ingredient lists. Complete data were available for 370 participants (102 with CD, 75 with UC and 193 healthy controls; 337 adults and 33 children). There was no difference in emulsifier exposure between those with UC or CD, and healthy controls. Mean exposure was 2.7 occasions per day (range 0–6.0) for those with UC, 2.8 occasions (range 0–10.7) for those with CD and 2.4 occasions for healthy controls (range 0–7.7). Children with IBD had significantly higher exposure to emulsifiers than adults (p < 0.01). Daily occasions of exposure (range) to CGN in patients with IBD were 0.4 (0–1.7) in children (n = 21) and 0.2 (0–1.7) in adults (n = 156).
Three abstracts reported on previous and current consumption of nine food additives including CGN (and CMC), determined in 156 participants (92 with CD, 21 first-degree relatives of patients with CD (FDR), 21 non-related healthy household members of patients with CD (HHM) and 22 healthy controls (HC)) (Zhang et al. Citation2022a, Citation2022b, Citation2022c). No significant differences in food additive consumption were observed among the CD, FDR, HHM and HC groups in the first two abstracts, whereas the third abstract reported that while the FDR of CD patients consumed less food additives, no significant differences were observed between the CD, HHM, and HC groups.
One publication on use of emulsifiers in processed foods mentioned CGN and exposure, but without providing any new quantitative data on exposure to CGN (Laster et al. Citation2019).
Sixty-one exclusive enteral nutrition (EEN) formulas used to induce remission in active CD contained 56 food additives (median per formula was 11) (Logan et al. Citation2020). CGN (unspecified) was present in 21.8% of the formulas.
The International Organization for the Study of Inflammatory Bowel Disease (IOIBD) has recommended that “it may be prudent to reduce intake of processed foods containing CGN” (with evidence level very low, agreement 92.3%) in both UC and CD, based on data from epidemiology, animal models and one very small RCT (Levine et al. Citation2020).
3.2.6. Absorption, distribution, metabolism and excretion (ADME) studies
Three publications contained some information about CGN and ADME. In a review article, it was summarized that CGN was not significantly degraded in the gastrointestinal tract and was excreted unchanged in the feces (Weiner Citation2014). CGN was not significantly absorbed, was not metabolized and did not affect nutrient absorption.
The ADME data on CGN was sufficient to show that high MW CGN (MW of ca. 800 kDa) was not absorbed intact, while low MW CGN (<88 kDa) was found in tissues of animals after oral administration (EFSA Citation2018a). No data were available on ADME of processed Eucheuma seaweed, but EFSA did not expect it to behave differently from CGN, based on their chemical structure. The conditions (duration, pH, temperature) for potential hydrolysis of CGN in the gastrointestinal tract were considered less extreme than the mild acidic conditions which resulted in the formation of chemically degraded CGN. The theoretical possibility that more limited degradation of CGN could occur under conditions representative of the in vivo situation remains uncertain although this had not been observed to any significant extent in the available in vivo ADME studies. It was also noted that the tested CGN differed in MW distribution and EFSA considered that existing results did not allow for evaluation of kinetic differences between the various types of native CGN (κ-, ι- and λ-) and their corresponding low MW fractions.
However, in an abstract, it was reported that E. coli ST871 in feces from healthy men could efficiently metabolize degraded κ-CGN in vitro and the promoter effect of degraded κ-CGN on colon tumorigenesis included the E. coli ST871-mediated metabolite pyrocatechol (Wang et al. Citation2022).
3.2.7. Risk assessment opinions
Two risk assessments of CGN were available. EFSA Panel on Food Additives and Nutrient Sources added to Food (ANS) reevaluated CGN (E 407) and PES (E 407a) as food additives (EFSA Citation2018a). The no observed adverse effect level (NOAEL) was 3400–3900 mg/kg bw per day, the highest dose tested, in a subchronic toxicity rat study performed with CGN nearly in compliance with the EU specification for E 407. No adverse effects were detected in chronic toxicity studies with CGN in rats up to 7500 mg/kg bw per day (the highest dose tested). The NOAEL values of sodium and calcium CGNs for prenatal developmental dietary toxicity studies were the highest doses tested, 3000 and 5000 mg/kg bw per day for rats and hamster, respectively. The safety of PES was considered sufficiently covered by the toxicological evaluation of CGN. They concluded that there was no concern regarding carcinogenicity of CGN, and no concern for genotoxicity of CGN and PES. However, EFSA concluded that the existing group ADI for the two food additives E 407 and E 407a of 75 mg/kg bw per day should be considered temporary because of the remaining uncertainties regarding chemistry (type and MW), exposure assessment, and biological and toxicological data, awaiting a new risk assessment with new data within five years.
In this risk assessment (EFSA Citation2018a), they evaluated both human studies, animal studies and in vitro studies, of which many are included in this scoping review. In addition, 48 documentations were submitted to EFSA from industry and other institutions not publicly available.
Previous risk assessments of these food additives (CGN, and also CMC) from JECFA, and the Scientific Committee on Food (SCF), the main committee providing the European Commission with scientific advice on food safety before being replaced by EFSA, are cited and referenced in the EFSA opinion summarized above for CGN, and below for CMC, and are not described here.
Native (undegraded) CGN has been classified by the IARC as Group 3, i.e. unclassifiable with respect to carcinogenicity in humans, whereas degraded CGN (PGN) is classified as Group 2B, a possible human carcinogen, based on animal data (IARC Citation1987).
3.2.8. Non-systematic (narrative) reviews, commentaries/editorials/letters and conference abstracts
No other scoping review/scoping study or systematic review on effects of CGN on the intestines in line with our objectives was detected among the published literature. However, 54 non-systematic review publications on CGN with varying scope and perspectives, published between 1973 and 2023, were included. Most of these review publications (36) were only on CGN (Watt and Marcus Citation1973; Watt and Marcus Citation1975; Anver and Cohen Citation1976; MacPherson and Pfeiffer Citation1976; Watt and Marcus Citation1981; Onderdonk Citation1985a, Citation1985b; Strober Citation1985; Whittaker and Freeman Citation1988; Nicklin and Miller Citation1989; Yoshida and Murata Citation1990; Weiner Citation1991; Kim and Berstad, Citation1992; Elson et al. Citation1995; Michel and Macfarlane Citation1996; Wirtz and Neurath Citation2000; Tobacman Citation2001; Cohen and Ito Citation2002; Hibi et al. Citation2002; Tobacman Citation2003; Kanneganti et al. Citation2011; Neurath Citation2012; Goyal et al. Citation2014; McKim Citation2014; Weiner Citation2014; Cian et al. Citation2015; Wells et al. Citation2017; David et al. Citation2018; Shang et al. Citation2018; Dey Citation2019; McKim et al. Citation2019; Gkikas et al. Citation2020; Gotteland et al. Citation2020; Liao et al. Citation2021; Liu et al. Citation2021; Li et al. Citation2023). They were concerned with health effects and safety of CGN and were often reviews on animal models. Many reviews were on effects of CGN on IBD, UC and colitis, some on colitis-associated carcinogenesis, microbiota or immunological effects, others mainly reviewed the use of CGN as pharmaceuticals, nutritional or functional food sources or prebiotics. Of the included reviews on CGN, 18 publications also included CMC (Liu et al. Citation2015; Martino et al. Citation2017; Paula Neto et al. Citation2017; Kikut et al. Citation2018; Halmos et al. Citation2019; Laster et al. Citation2019; Marion-Letellier et al. Citation2019; Vo et al. Citation2019; Zinöcker Citation2019; Bancil et al. Citation2021; Borsani et al. Citation2021; Cox et al. Citation2021; Sasson et al. Citation2021; Tan and Nie Citation2021; De Siena et al. Citation2022; Levine et al. Citation2022; Liu et al. Citation2022; Kuang et al. Citation2023) and reviewed effects on intestinal inflammation and disease, microbiota, intestinal permeability, obesity and metabolic dysregulation, or allergy.
Fourteen publications on CGN in the category commentaries/editorials/letters were published in the period 1969–2022. One of the main issues in these publications (as well as in original research publications) seems to be the differences between native and degraded CGN. A distinction is made between the food grade CGN of high MW and degraded CGN, which are not the same substances as commercial food grade CGN (Marcus and Watt Citation1969; Tobacman Citation2002a, Citation2002b; Bixler Citation2017; David et al. Citation2019; Weiner and McKim Citation2019). Another issue is whether the research from experimental animal models (and in vitro model systems) is representative of effects of CGN in humans being exposed to CGN as a food additive or as medication (Bonfils Citation1970; Marcus and Watt Citation1970; Mottet Citation1972). Other emphasize conclusions on evaluations of safety of CGN already performed by health authorities (Carthew Citation2002; Kirsch Citation2002). Three were on adverse effects on the intestines of several food additives, also including CMC (Zinöcker and Lindseth Citation2019; Cox and Sandall Citation2021; Wellens et al. Citation2022).
The 23 conference abstracts on CGN found in the searches, published 1984–2022, were mostly reporting studies on unspecified CGN, either degraded or native, and mostly in animals or in vitro. The effects of CGN in these abstracts were often reported also as full publications (Shiau and Chang Citation1984; Madhère et al. Citation1994; Axelsson and Midtvedt Citation1997; Sabljic et al. Citation2002; Bhattacharyya et al. Citation2010a; Bhattacharyya and Tobacman Citation2011; Bhattacharyya and Tobacman Citation2012; Bhattacharyya et al. Citation2014a; Fahoum et al. Citation2015; Jimenez Loayza et al. Citation2019; Kang et al. Citation2022; Wang et al. Citation2022). In addition, four abstracts were on both CGN and CMC (Schooth et al. Citation2020; Zhang et al. Citation2022a, Citation2022b, Citation2022c).
However, seven abstracts reporting on human studies were not obtained as full publications. In Cochrane Library, a randomized controlled clinical trial was listed (Cochrane Central Register of Controlled Trials Citation2021). The inclusion criteria were UC, clinical remission verified by normal fecal calprotectin 2, age 18‐64 years, with no biological medications or systemic cortisone usage for relapse. The intervention was CGN 2‐2.5 g/day vs. a corresponding dose of oat fibre, starting with a 7‐day run‐in period (low CGN diet, instructed by a dietician), then either a CGN period or an oat fibre (control) period for 7 days, followed by at least 14 days of wash-out, then the other treatment (either CGN or oat fibre), altogether 35 days. The primary outcome studied was colitis activity measured using the Simple Clinical Colitis Assessment Index (SCCAI) at baseline and the 7th day of both treatment periods. Secondary outcomes were gastrointestinal symptoms, laboratory tests using blood, urine and stool samples at baseline and at the end (7th day) of both treatment periods measuring fecal calprotectin, hs‐CRP, S‐FABP‐2 (intestinal permeability marker), U‐creatinine, U‐Albumin, F‐albumin, F‐IgG and F‐intestinal alkaline phosphatase (IAP). Macronutrient and fibre intake will be measured using a 3‐day food diary at the end of both treatment periods. No results were reported.
Two abstracts on a randomized, double-blind, placebo-controlled, cross-over trial (registered as NCT02629705) were published by Wagner et al. (Citation2018a, Citation2018b). Healthy males (n = 20) were randomly allocated to 14 days of CGN (250 mg twice daily) or matching placebo. Participants had a mean (± SD) age of 27.6 ± 4.8 years and a BMI of 24.4 ± 2.6 kg/m2. After a wash-out period of 30 ± 7 days, they received the other compound. At the end of each treatment phase, participants underwent an oral glucose tolerance test (OGTT), a hyperinsulinemic-euglycemic clamp with labeled glucose (ISIclamp), whole-body magnetic resonance (MR)-tomography and 1H-MR-spectroscopy, blood immune cell phenotyping and a lactulose-mannitol test for investigating intestinal permeability. Intestinal permeability increased after CGN vs. placebo (p = 0.03). Whole-body insulin sensitivity index (ISI), both ISIclamp and OGTT, did not differ between CGN and placebo. No changes of hepatic fat content, body fat mass, fat distribution, liver transaminases or inflammatory cytokines were observed.
In addition, an abstract not covered by a full publication reported results of modified CGNs. The effects of κ-CGN gels modified with Na+ and Ca2 were studied in neonatal rats, which received a daily oral dose of pure refined food grade κ-CGN (500 mg/kg bw per day), κ-CGN with 10% Na+, κ-CGN with 10% Ca2+ or no κ-CGN as control group, for 14 weeks (Farag et al. Citation2018). All groups showed normal growth without differences in body weight or feed consumption, and no mortality or pathological symptoms were observed. Serum biochemical parameters showed increase (p < 0.05) in total cholesterol with κ-CGN + Ca compared with controls, whereas no differences were observed in total glycerides, total lipids, high or low density lipoprotein cholesterol, liver enzymes, urea, creatinine or haematological parameters between any groups. Serum antioxidant biomarkers in rats treated with κ-CGN + Ca showed decreased (p < 0.05) superoxide dismutase (SOD) and glutathione reductase (GR) activities vs. controls, but the other two groups did not. Rats treated with κ-CGN showed increase (p < 0.05) in malondialdehyde (MDA), whereas no differences were detected between κ-CGN + Na, κ-CGN + Ca and controls. Catalase activity (CA) was not affected in any groups. In contrast to serum, levels of liver antioxidants showed no differences for SOD, GR, MDA or CA between any groups. Histological investigation showed minor changes in rat colon mucosa of κ-CGN and κ-CGN + Na, such as focal destruction of crypts of Lieberkuhn, where some were replaced by cellular infiltration, loss of colonic crypt integrity seen as loss of vacuolated cytoplasm, presence of dense small nuclei and lymphocytic aggregation in the submucosa.
One abstract reported that E 407a promoted ROS generation by leukocytes and induced intestinal inflammation associated with macrophage and T-lymphocyte infiltration after two weeks of daily oral exposure to 140 mg/kg bw in rats (Tkachenko et al. Citation2021).
Two abstracts reporting exposure to food additives, including CGN, per day in children with IBD and the association with IBD relapse (Ho et al. Citation2021) and exposure using baseline dietary data from adults and children with IBD (Reveley et al. Citation2022) are described in Section 3.2.5.
3.3. Publications on carboxymethylcellulose (CMC) (E 466)
Few publications on CMC were found until the period 1991–2023 and the number increased especially after 2016 (). Only two publications were investigating modified CMC, which are described in more detail below (Bär et al. Citation1995, see Section 3.3.6.) and Andreev-Andrievskiy et al. Citation2021, see Section 3.3.3).
Figure 9. The number of research publications studying non-modified or modified CMC per 5-year periods.
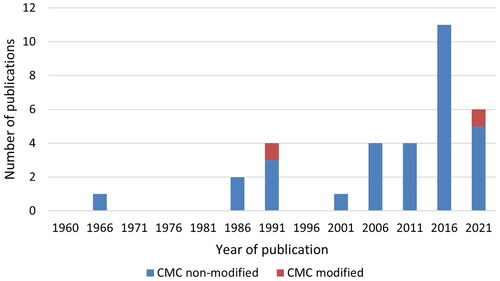
The distribution of various types of original research publications on CMC (human studies, experimental animal studies and in vitro studies) is shown in . Only three human studies were available. As for CGN, the highest number of publications was on various experimental animals (20), with in vitro studies as the second largest category (14), studying microbiota from humans or animals ex vivo-in vitro or describing mechanistic data in human or animal primary cells or cell lines. Seventeen publications of various types contained some information relevant for exposure to CMC, two had some information on toxicokinetics and ADME, and five risk assessment opinions by EFSA on CMC were found. The main results of the original research publications on humans, animals and in vitro are described briefly in the following.
Figure 10. Distribution of various types of publications on CMC. The human studies (without studies of exposure), experimental animal studies, in vitro studies and other types of studies (one toxicokinetic study), are original research publications. Exposure studies comprise all types of publications that contain information on levels in food, exposure etc., including from review publications and conference abstracts. In addition, publications on CMC containing information on toxicokinetics and ADME, and risk assessments, were included.
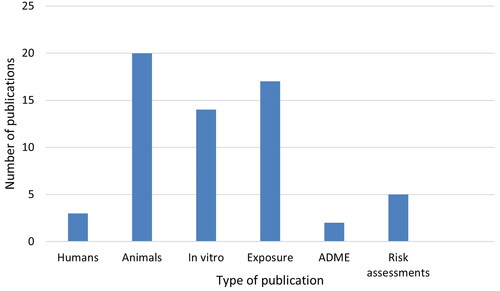
3.3.1. Human studies
Only three publications contained original research in humans relevant for effects of CMC. A randomized, double-blind, controlled feeding study included healthy adults (18–60 years) of both sexes kept first on an emulsifier-free diet at home for three days and thereafter divided into two groups and given either a CMC-free diet (n = 9) or a CMC-rich diet (15 g/day of CMC) (n = 7) for 11 days (Chassaing et al. Citation2022a). In the CMC group, an increased disturbance of the intestinal microbiota with reduced bacteria diversity, increased stomach discomfort, and reduction of SCFA and free amino acids in feces, were observed. An increased amount of bacteria in the normally sterile inner mucus layer of the intestine was found in two of the test subjects, indicating intestinal inflammation. According to the authors, the amount of CMC used was somewhat higher than the CMC intake for most persons, but that those who consume a lot of processed food would have an intake of CMC comparable to in this study.
In a study of five healthy male volunteers (age range 24–58 years) given 15 g CMC daily for 23 days after a 7-day control period, no adverse effects were noted either on plasma biochemistry, haematology, urinalysis, glucose tolerance, serum cholesterol, triglyceride and phospholipids, or breath hydrogen and breath methane concentrations (Anderson et al. Citation1986). In addition, some inconsistent changes were observed on intestinal transit time, fecal wet and dry weights, fecal bile acids, fecal fat and excretion of neutral sterols, which were considered physiological and not adverse.
One case report ascribed anaphylaxis to CMC in a 14-year-old girl (Ohnishi et al. Citation2019). She had four episodes of anaphylaxis and the common ingredient in the foods eaten in all episodes (pizza, cheeseburger, ice lolly and a half-frozen beverage) was CMC. She tested positive for CMC in skin prick tests with a CMC solution and CMC-containing foods, and did not react to a CMC-free ice lolly from the same manufacturer as eaten before. No other publications were found on adverse effects of CMC in children or adolescents.
3.3.2. Animal studies
The 20 included publications on adverse effects of CMC on the intestines in experimental animals were mostly on mice (13) and/or rats (7), as well as one study on pigs.
CMC in drinking water was administered for 12 weeks to wild-type mice and to two strains, IL-10−/− and TLR5−/−, that are sensitive to effects on microbiota composition and inflammation (Chassaing et al. Citation2015). CMC induced low-grade inflammation and obesity/metabolic syndrome in wild-type mice and induced profound colitis in the sensitive strains. CMC-induced metabolic syndrome was associated with microbiota encroachment, changes in species composition and increased pro-inflammatory potential. Further, use of germ-free mice and fecal transplants indicated that these changes in microbiota were necessary and sufficient for both low-grade inflammation and metabolic syndrome. In another study, when the CMC-treated M-SHIME human microbiota (see Section 3.3.3) was transferred to germ-free mice, many of the host and microbial alterations observed in mice directly treated with CMC were observed (Chassaing et al. Citation2017a). In a third study, gnotobiotic wild-type and IL-10-/- mice were colonized with adherent-invasive E. coli (AIEC), associated with Crohn’s disease, and subsequently administered CMC in drinking water for 12 weeks (Viennois et al. Citation2020). AIEC colonization of germfree and altered Schaedler flora (ASF) mice resulted in chronic intestinal inflammation and dysregulations of metabolism after CMC exposure. In IL-10-/- mice, AIEC mono-colonization resulted in severe intestinal inflammation in response to CMC. Transcriptomic analysis revealed that CMC directly induced expression of genes that mediate AIEC virulence and promotion of inflammation.
Effects of CMC on ex-germ-free IL10-/- mice colonized by pooled fecal transplant from three patients with active IBD were investigated by Rousta et al. (Citation2021). In mice which received CMC in drinking water for four weeks, CMC increased fecal lipocalin 2 (Lcn-2) levels, histological inflammatory scores and inflammatory cytokine gene expression in colon. CMC did not affect bacterial composition but significantly decreased Caudoviricetes (bacteriophages). Thus, CMC promoted aggressive inflammation without changing bacterial composition. Twenty to 27-week-old IL-10 gene-deficient mice received either CMC or water orally for 3 weeks (Swidsinski et al. Citation2009). CMC-treated IL-10 gene-deficient mice demonstrated a massive bacterial overgrowth, distention of spaces between villi filled with bacteria, adherence of bacteria to the mucosa and migration of bacteria to the bottom of the crypts of Lieberkuhn. Leukocytes migrated into the intestinal lumen in four of the seven CMC-exposed mice. The changes were similar to those observed in CD in humans and were absent in control animals.
As opposed to other studies, in IL-10-/- mice which develop spontaneous colitis, exposure to CMC by daily gavage for 14 days caused reduction of blood in the feces, feces consistency score, histopathological injury score, colon weight/length ratio and activity of MPO vs. controls (Ung et al. Citation2010). However, CMC did not decrease the levels of the inflammatory cytokines interferon (IFN)-γ or IL-17 in colon or caecum.
Exposure of mice to CMC in drinking water for 12 weeks induced chronic intestinal inflammation, increased adiposity and caused sex-dependent changes in gut microbiota composition (Holder et al. Citation2019). Additionally, CMC treatment altered anxiety-like behaviours in males and reduced social behaviour in females, followed by changed expression of neuropeptides associated with regulation of feeding as well as social and anxiety-related behaviour.
Exposure of mice to CMC in drinking water for 15 weeks caused inflammation, reduced mucus thickness and increased the intestinal permeability measured by increased passage of the permeability tracer fluorescein isothiocyanate (FITC)-dextran into serum (Wu et al. (Citation2020).
When mice received oral CMC (0.45 ml of a 0.05% solution) daily for 5 days, two of 11 mice died vs. none of ten in the controls, mean body weight was reduced by 3.6% and level of C-reactive protein was increased (Nabarawi Citation2014). CMC in drinking water for 12 weeks administered to adult mice increased body weight and adipose tissue but did not affect fasting blood glucose (Sandall et al. Citation2020). CMC also decreased colon length, a proxy marker for intestinal inflammation, compared to controls.
Increased risk of colorectal cancer associated with IBD coined the term “colitis-associated cancer” and the concept that inflammation promotes cancer (Viennois et al. Citation2017). A colitis-associated cancer model was obtained by treating mice with the colon carcinogen AOM and dextran sulfate sodium (DSS) to induce inflammation. CMC in drinking water for ca. 14 weeks exacerbated tumor development (both number of tumors and tumor area) and altered the microbiota metagenome, characterized by increased levels of LPS and flagellin. In a later study, the effect of CMC on cancer initiation and progression in a genetic model of intestinal adenomas using mice with the multiple intestinal neoplasia (Min) mutation in the tumor suppressor gene adenomatous polyposis coli (Apc), ApcMin/+ mice, was investigated (Viennois and Chassaing Citation2021). CMC in drinking water for 15 weeks increased small intestinal tumor development, apparently independent of chronic intestinal inflammation, but it was associated with CMC’s effects on proliferation in the intestinal epithelium and intestinal microbiota composition.
Long-term exposure of rats and mice to dietary levels of 10 or 100 g/kg of CMC (Edifas B) for 104 and 100 weeks, respectively, had little adverse influence on the general health and survival of the animals (McElligott and Hurst Citation1968). The haematological parameters in the rats were also unaffected. The rats appeared to compensate for the lower nutritional value of the diet by increased food consumption, but in the later stages of the experiment retardation of growth was apparent. Histological examination revealed no intestinal abnormality or evidence of the passage of Edifas B through the intestinal wall, and there was no evidence that the substance was carcinogenic, in either species.
The protective mucus layer in the intestines acts as a barrier that protects the underlying epithelial layer against microorganisms and chemicals. When rat intestines were directly exposed to 1% CMC for 30 min., mucus pore size decreased, which resulted in significantly slower E. coli speed and passive diffusion rates of nanoparticles through mucus (Lock et al. Citation2018).These results indicated that acute exposure to CMC may impact barrier and structural properties of intestinal mucus, affect interactions between intestinal lumen contents, microbes and underlying tissue, which may contribute to development of intestinal inflammation. Three concentrations of CMC (0.1%, 0.2% and 0.5%) could all increase absorption of a drug with low intestinal permeability, shown by intestinal perfusion in rats (Valizadeh et al. Citation2015).
Some older publications studied effects of CMC on various physiological parameters of the intestines and feces in rats, and reported effects on cell proliferation, tissue hypertrophy - mostly caused by the increased bulk of feces and diarrhea (Wyatt et al. Citation1988; Toyoda et al. Citation1994; Juśkiewicz and Zduńczyk Citation2004).
In addition, a study on toxicokinetics in rats by Bär et al. (Citation1995) is described under Section 3.3.6.
In weaned piglets given CMC in the diet for 13 days, aerobe bacteria were transiently higher at day 7 but coliform counts remained unchanged and β-haemolytic E. coli were virtually absent (Lallès et al. Citation2006). This indicated that CMC had pro-absorptive effects on the small intestine, possibly due to the absence of pathogenic E. coli.
3.3.3. In vitro studies
In total, 14 publications on in vitro experiments on CMC were included, using fecal microbiota from humans (8) or animals (rat and pig) (2), or cell lines from humans (4) or primary cells from animals (rat and mouse) (3). All except one in vitro publication (Andreev-Andrievskiy et al. Citation2021) studied non-modified CMC.
Effects of Kagocel, a synthetic CMC derivative copolymerized with gossypol, were studied in murine Peyer’s patches lymphocytes from the small intestine of mice (Andreev-Andrievskiy et al. Citation2021). The lymphocytes were stimulated with concanavalin A (Con A), or with Con A and TLR3 ligand poly I:C to mimic viral infection. After 24 h of stimulation, the cells were treated with Kagocel. Expression of genes involved in the inflammatory response, antiviral defense, lymphocyte survival and proliferation (C1qa, C2, C3, Ccl21a, Il11, Il1b, Il23a, Il5, Ltb4r2, Alox15, Pla2g4a, Ptger1, Mapkapk5, Hras, Ifna1, Tlr2, Mrc1 and Mx2) was upregulated in Kagocel-treated lymphocytes. Transcription factors (CEBPs, IRF, NFκB, RXR, Stat, Tead4 and ZSCAN) and master regulators (cIAP, CIKS, dock9, MEKK1, FXR, IKK, IRAK, TRAF, dsRNA:TLR3:TRIF) were also identified. The changes in gene expression pattern and the outcome of bioinformatics analysis suggested that pattern recognition receptors, TLRs and dectin-1, were the key mediators of Kagocel’s effects on the immune system, possibly involving the interferon autocrine loop. Kagocel upregulated genes of various components of the innate immune defense system.
Three publications reported studies of CMC and intestinal permeability. Concentrations of 0.02%, 0.1%, 0.5% and 1% CMC, claimed to be commonly used in the food industry, did not affect intestinal permeability studied ex vivo in the Ussing diffusion chamber system using small intestinal fragments from rats and in the human colorectal adenocarcinoma-derived cell line Caco-2, or the stimulation of the human dendritic cell-like myeloid leukemia-derived MUTZ-3 cell line (Oscarsson et al. Citation2020). Testing of CMC in mucus-producing rat intestinal cell cultures, human Caco-2 cells and mucus-producing human colon HT29-MTX cells in a monolayer showed that CMC decreased mucus pore size and reduced diffusion of various particles through the mucus (Lock et al. Citation2018). CMC also resulted in partial removal of the mucus layer, making it thinner with fewer globular mucus aggregates, which may contribute to exposure of the epithelium to intestinal contents and increase bacterial translocation, resulting in intestinal inflammation. Lastly, exposure of AIEC to CMC in vitro increased the motility and ability to adhere to human intestine-407 epithelial cells, but invasion ability was not affected (Viennois et al. Citation2020). Transcriptomic analysis revealed that CMC directly induced expression of clusters of genes that mediate AIEC virulence in a dose-dependent way and promoted inflammation.
Four publications studied changes by CMC on the microbiota. In an ex vivo-in vitro study on feces from pigs, dietary CMC administered to growing pigs resulted in a distinctive bacterial community (Metzler-Zebeli et al. Citation2010). CMC affected the taxonomic composition and metabolic features of the fecal microbiota, as shown by increases in total bacteria, Bacteroides-Prevotella-Porphyromonas and Clostridium cluster XIVa, and Enterobacteriaceae and increased prevalences of E. coli virulence factors in feces.
The mucosal simulator of the human intestinal microbial ecosystem (M-SHIME) is a dynamic in vitro model which simulates the lumen- and mucus-associated human intestinal microbial ecosystem. It maintains a complex stable human microbiota in the absence of a host. In this system, CMC increased the pro-inflammatory potential of the human fecal microbiota, demonstrated by increased levels of bioactive flagellin (Chassaing et al. Citation2017a). In another model system, CMC had adverse effect on human microbiota composition when using feces obtained from a healthy person and maintained ex vivo in the MiniBioReactor Array model, by directly changing the microbiota composition and gene expression (Naimi et al. Citation2021). CMC had only modest effects on levels of flagellin and LPS measured in human embryonic kidney HEK cells with this donor.
Seven publications reported on fermentation of CMC in vitro. CMC was barely fermented, as shown by no changes in MW, and showed similar total SCFA production as the controls when tested with feces from three healthy adult donors (one man and two women) (He et al. Citation2022). However, CMC increased the relative abundance of the Firmicutes phylum. At the operational taxonomic unit (OTU) level, CMC could apparently favour the growth of certain bacterial families, but which one(s) changed with the donor.
Using fecal microbiota from 10 adult humans (four women and six men), 0.005%, 0.05% and 0.5% CMC did not affect the structure of the cell population and even increased total cell count at higher concentrations, although the fraction of living cells was unaffected by all CMC concentrations (Miclotte et al. Citation2020). CMC had small impact on microbial composition in all three concentrations and had no effect on the general microbial metabolic activity, including SCFA production. The effects of CMC on several parameters, including flagellin levels, varied strongly depending on the microbiota donor.
In a study of in vitro batch fecal fermentation, CMC showed no or minimal effects on the broad composition and fermentation capacity of the fecal microbiome, had no effects on the production of any SCFA or BCFA, and had no significant effects on the growth of broad bacterial populations, when using fecal samples from 13 young healthy donors (seven women and six men) (Gerasimidis et al. Citation2020).
In a study of in vitro fermentation of CMC using fecal samples from 10 healthy adult donors (5 of each sex), CMC increased acetic acid, butyric acid and propionic acid, as well as a total of six SCFA (Chen et al. Citation2020). With CMC, significantly lower Shannon diversity index than with controls was observed, indicating lower microbial diversity. After CMC exposure, there was a higher relative abundance of Fusobacteria, Bacteroides and Proteabacteria, and a relative decrease in Firmicutes, at the phyla level. There were clear spatial separation and clustering of the fermentation bacteria from the CMC-treated vs. control groups. Unique microbiota changes after CMC treatment were increased abundance of Escherichia-Shigella and Lachnoclostridium, and decreased abundance of Bifidobacterium, Streptococcus, Fecalibacterium and Ruminococcus_torques_group.
In a study on fibre composition of CMC, the majority of CMC was soluble fibre and it was estimated that about 55% of the glucose residues in CMC had at least one carboxymethyl group substitution and therefore would not be available for fermentation by gut bacteria (Bliss et al. Citation2013). Further, in vitro incubation was studied with feces from 13 adult persons (nine women and four men) with fecal incontinence and adverse gastrointestinal symptoms, before and after consumption of CMC given in muffins and juice twice daily for 20–21 days, or a placebo without CMC. The results indicated that prior consumption of a specific fibre did not increase its degradation by fecal bacteria.
In vitro fermentation of CMC with feces from three healthy adult male donors resulted in formation of the SCFAs acetate, butyrate and propionate during 24 h (Bourquin et al. Citation1993).
In vitro fermentation with slurries of cecal contents from rats fed a fibre-free diet prepared under anaerobic conditions showed that CMC was poorly fermented, and thus, not significantly degraded (Wyatt et al. Citation1988).
3.3.4. Hypotheses on adverse effects of CMC
The number of research publications studying CMC in relation to various hypotheses on adverse effects is shown in . Effects on the gut microbiome as well as inflammation are studied in the highest number of publications, with 19 and 17 publications, respectively. Metabolic effects (11) and fermentation (10) are the next most common topics, followed by intestinal physiology, permeability of the intestinal barrier and cancer, with 5, 5 and 4 publications, respectively.
3.3.5. Data relevant for exposure
Seventeen publications had some information relevant for exposure to CMC. In a review, celluloses, including CMC, were reported to be present in 2% of all food products in the food supply in EU (Cox et al. Citation2021).
Mean dietary exposure to CMC in the population aged >2 years in USA was estimated to be 30, 27, 25 and 24 mg/kg bw per day in the time periods 1999–2002 and 2003–2010, both in the NHANES study, and 1999–2002 and 2003–2010, both in the NTP NET-NID study, respectively (Shah et al. Citation2017). The 90th percentile dietary exposure to CMC was estimated to be 70, 60, 53 and 53 mg/kg bw per day and the percentages of users of CMC were 95, 100, 100 and 100, in the same time periods and studies, respectively. This study was also reported in review publications by Laster et al. (Citation2019), Laudisi et al. (Citation2019) and Cox et al. (Citation2021).
In EFSA (Citation2018b), total exposure was only indicated for all the ten evaluated microcrystalline, powdered and modified celluloses together, being around 660–900 mg/kg bw per day, not for E 466 alone.
The incidence and prevalence of IBD are increasing globally, both in Western countries and Asia, although thought to be lower in Asia, whereas prevalences in Europe are as high as those in USA, according to a review by Park et al. (Citation2020). These increases are also seen in children. The increases in IBD were hypothesized to be caused by numerous environmental factors, including dietary factors, such as CMC, and nutritional factors.
Average exposure to CMC in children of both sexes (n = 138, mean age 14.2 ± 2.8 years) with CD was quantified using data from three 24-h recall days over two years obtained by telephone (Lee et al. Citation2018). A total of 1325 unique foods were recorded and 0.4% of the foods contained CMC. Mean ± SD frequencies of exposures to CMC per day were 0.05 ± 0.13 (range 0–0.67). The frequency of exposure to CMC (mean ± SD) in 20 patients with CD using 7-day food diaries analyzed for habitual intake was 0.14 ± 0.30 per day (Sandall et al. Citation2020).
In an abstract, the exposures to selected food additives, including CMC (and CGN), per day in children with IBD and the association with IBD relapse were reported (Ho et al. Citation2021) (see Section 3.2.5.). An abstract reported emulsifier exposure using baseline dietary data from adults and children with IBD enrolled in the Australian AIM study, a multicenter, prospective, longitudinal cohort study (Reveley et al. Citation2022) (see Section 3.2.5.). It was reported that daily occasions of exposure (range) to CMC in patients with IBD were 0.3 (0–3.0) in children (n = 21) and 0.1 (0–1.7) in adults (n = 156). Three abstracts determined previous and current consumption of nine food additives, including CMC (and CGN), in patients with CD, their first-degree relatives, their non-related healthy household members and 22 healthy controls (Zhang et al. Citation2022a, Citation2022b, Citation2022c) (see Section 3.2.5.).
Exposure was mentioned, but without providing quantitative data on CMC, in a review by Martino et al. (Citation2017) and in an exposure publication by Chazelas et al. (Citation2021).
CMC was present in 12.7% of sixty-one EEN formulas used to induce remission in active CD (Logan et al. Citation2020).
The International Organization for the Study of Inflammatory Bowel Disease (IOIBD) has recommended that “it may be prudent to limit intake of CMC” (with evidence level very low, agreement 92.3%) in both UC and CD, based on data from epidemiology, animal models and one very small RCT (Levine et al. Citation2020).
3.3.6. Toxicokinetics and ADME studies
E 466 was not absorbed intact, not fermented and was excreted intact via feces (>90%) in rats, rabbits and humans (EFSA Citation2018b, Citation2020).
Only one study included both modified, i.e. partially hydrolysed CMC, depolymerized with a cellulase-containing enzyme preparation from Trichoderma longhibrachiatum (CMC-ENZ), and non-modified CMC, studying toxicokinetics of the two CMC variants (Bär et al. Citation1995). Five % of each CMC type were administered in the diet to rats for two weeks, followed by a single gavage of 14C-CMC-ENZ or 14C-CMC (500 mg/kg bw). About 95% of the label was excreted in feces, <2% in urine, <1% with CO2 and a small fraction was retained in the body (CMC, 0.58%; CMC-ENZ, 0.75%). Tissue retention of 14C was highest in the liver after both treatments. Only about 49% and 65% of fecal 14C was extracted with water in the 14C-CMC- and 14C-CMC-ENZ-dosed rats, respectively. Gel permeation chromatography of the dosing solutions and the fecal extracts revealed that CMC was depolymerized during intestinal passage, whereas CMC-ENZ was excreted nearly unchanged. Consequently, the MW distribution of the 14C-CMC and 14C-CMC-ENZ fecal excretion products was similar. The authors concluded that there was no toxicologically relevant difference between the disposition of CMC and CMC-ENZ in rats.
3.3.7. Risk assessment opinions
Five relevant risk assessments of CMC had been performed by EFSA. Sodium CMC (E 466) was evaluated together with other cellulose food additives in the group “chemically modified celluloses” by the ANS Panel (EFSA Citation2018b), based on read-across between all the celluloses because of their structural, physicochemical and biological similarities. Note that modified CMC in this and other EFSA opinions does not have the same meaning as in other publications (Bär et al. Citation1995), and, thus, CMC was not coded as modified for the EFSA opinions in ER. The acute toxicity of celluloses was low and there was no genotoxic or carcinogenic concern. Short-term and subchronic dietary toxicity studies at levels up to 10% in the feed did not indicate specific adverse effects. In chronic toxicity studies, the NOAEL values ranged up to 9000 mg/kg bw per day of celluloses. Adverse effects on reproductive performance or developmental effects were not observed with celluloses at doses greater than 1000 mg/kg bw by gavage (often the highest dose tested). EFSA concluded that there was no need for a numerical ADI value and that there would be no safety concern at the reported uses and use levels for the evaluated celluloses, including for E 466.
The EFSA Panel on Additives and Products or Substances used in Animal Feed (FEEDAP) assessed sodium CMC as a feed additive and concluded that it was safe for all animal species and that the use of this substance in animal nutrition was of no concern for consumer safety (EFSA Citation2020). Likewise, the EFSA Panel on Food Contact Materials, Enzymes, Flavorings and Processing Aids (CEF) assessed (sodium) CMC and concluded that it did not raise a safety concern for the consumer when used as a component of a mixture in active food contact materials intended used as moisture and liquid absorbers in the packaging of perishable foods to extend their shelf-life (EFSA Citation2012, Citation2018c).
As a follow-up of the assessment by the ANS Panel in 2018 (EFSA Citation2018b), the Panel on Food Additives and Flavorings (FAF) assessed the safety of E 466 for its uses as a food additive in food for infants below 16 weeks of age belonging to FC 13.1.5.1 (Dietary foods for infants for special medical purposes and special formulae for infants) and addressed the issues previously identified when used in food for the general population, including the safety assessment for FC 13.1.5.1 and FC 13.1.5.2 (Dietary foods for babies and young children for special medical purposes) (EFSA Citation2022). The business operators declared that E 466 was not used in food for infants below 16 weeks of age and in FC 13.1.5.1. Due to the lack of data, an assessment was not performed for this FC and age group. The business operators did not provide data to support the uses of E 466 in FC 13.1.5.2. Due to the almost unchanged database compared to in the previous evaluation, the FAF Panel confirmed the previous conclusion that the available data was still not sufficient for an adequate assessment of the safety of use of E 466 in infants and young children consuming foods belonging to the FC 13.1.5.2.
3.3.8. Non-systematic (narrative) reviews, commentaries/editorials/letters and conference abstracts
No other scoping review/scoping study or systematic review on effects of CMC on the intestines in line with our objectives was detected among the published literature. In total, 26 narrative reviews on CMC (all non-modified) were included. The reviews on CMC were published more recently, between 2015 and 2023, compared with many of those on CGN. Eight narrative reviews on CMC were included, reviewing effects on inflammatory bowel diseases, the microbiome or CMC as an obesogenic chemical (Glade and Meguid Citation2016; Viennois and Chassaing Citation2018; Laudisi et al. Citation2019; Partridge et al. Citation2019; Goens and Micic Citation2020; Park et al. Citation2020; Rinninella et al. Citation2020; Heindel et al. Citation2022). In addition, 18 publications, also including CGN, reviewed effects of CMC on intestinal inflammation and disease, microbiota, intestinal permeability, obesity and metabolic dysregulation, or allergy (Liu et al. Citation2015; Martino et al. Citation2017; Paula Neto et al. Citation2017; Kikut et al. Citation2018; Halmos et al. Citation2019; Laster et al. Citation2019; Marion-Letellier et al. Citation2019; Vo et al. Citation2019; Zinöcker Citation2019; Bancil et al. Citation2021; Borsani et al. Citation2021; Cox et al. Citation2021; Sasson et al. Citation2021; Tan and Nie Citation2021; De Siena et al. Citation2022; Levine et al. Citation2022; Liu et al. Citation2022; Kuang et al. Citation2023).
The nine commentaries/editorials/letters on CMC published 2015–2022 all discussed non-modified CMC. Two of them (Bordon Citation2015; Cani Citation2015) concerned the published study on CMC by Chassaing et al. (Citation2015) and two concerned the publication by Chassaing et al. (Citation2022a); Wellens et al. (Citation2022) and the answer by Chassaing et al. (Citation2022b). One discussed CMC in relation to IBD in Australia (Niewiadomski Citation2018), whereas four were on adverse effects on the intestines of several food additives and also mentioned CGN (Zinöcker Citation2019; Zinöcker and Lindseth Citation2019; Cox and Sandall Citation2021; Wellens et al. Citation2022).
All the 24 conference abstracts on CMC published 2005–2022 were reporting studies on non-modified CMC, mostly in animals or in vitro. Two abstracts (Cochrane Central Register of Controlled Trials Citation2018; Chassaing et al. Citation2020) on a randomized double-blind, controlled feeding experiment with CMC had later been published in full (Chassaing et al. Citation2022a). Fourteen abstracts were on one or more effects of CMC on intestinal inflammation, microbiome and metabolic changes, intestinal permeability, mucin-producing cells, or obesity, metabolic syndrome and thermogenesis (Bowen-Yacyshyn et al. Citation2005; Ung et al. Citation2009; Yacyshyn et al. Citation2011; Chassaing and Gewirtz Citation2013; Chassaing and Gewirtz Citation2014; Chassaing et al. Citation2016; Chassaing et al. Citation2017b; Chassaing and Gewirtz Citation2017a; Chassaing and Gewirtz Citation2017b; Behr et al. Citation2018; Xu et al. Citation2019; Zheng et al. Citation2019; Zheng et al. Citation2020; Zangara et al. Citation2021). In addition, four abstracts included both CMC and CGN (Schooth et al. Citation2020; Zhang et al. Citation2022a, Citation2022b, Citation2022c).
Four additional abstracts reported human data not yet presented in full publications. The exposures to selected food additives per day in children with IBD and the association with IBD relapse were reported (Ho et al. Citation2021). The results in this abstract were described for ten food additives together, including CMC (and CGN) (see Section 3.2.5.).
In an abstract by Kang et al. (Citation2021), it was examined in vitro how CMC affected CD mucosa-associated microbiota (CD-MAM) in microbial cultures with cryopreserved biopsies from five CD patients. These cultures were inoculated in medium with 0.1% CMC (a concentration found in food) or without additive (control). The addition of CMC appeared to have only modest effects on the CD-MAM communities from 4 of 5 CD patients. However, in one person, CMC resulted in large expansions in the relative abundance of Bacteroides, Proteus, Morganella, the family Enterobacteriaceae and Enterococcus, with a concordant reduction in the Streptococcus lineages.
Emulsifier exposure was examined using baseline dietary data from adults and children with IBD enrolled in the Australian AIM study, a multicenter, prospective, longitudinal cohort study, and compared with exposure of healthy controls (Reveley et al. Citation2022). The results in this abstract were described for six food additives together, including CMC (and CGN) (see Section 3.2.5.).
An abstract reported generation of a gnotobiotic human intestinal organoid microcosm system (HIOMS) as an in vitro model of the human gut microbiome (Xu et al. Citation2020). The organoids were microinjected with a live mixture of four functionally distinctive bacteria species, Akkermansia muciniphila, Bacteroides acidifaciens, B. cellulosilyticus and B. intestinalis, supplied with CMC and co-cultured for 48 h. A stable colonization of A. muciniphila and B. intestinalis was demonstrated and their abundance was not affected by CMC. However, CMC increased α-fucosidase activity by 360%.
3.4. Evidence presented as interactive research maps
The included evidence was presented as interactive research maps, which give a visual overview of where in this area there is quite a lot, some or non-existent scientific knowledge. The first interactive research map on evidence found in the 262 included publications () is showing various types of CGN and CMC in the columns, type of publications in the rows and hypotheses on the adverse effects on the intestines as bubbles. The size of the bubbles indicates the number of studies in each square.
Figure 12. Image of the interactive research map showing various types of CGN and CMC in the columns, type of publications in the rows and hypotheses on the adverse effects on the intestines as bubbles. The size of the bubbles indicates the number of studies in each square. From this map, available and missing data in the various categories can be easily seen. The map can be downloaded from Supplementary materials Figure 1 be used interactively.
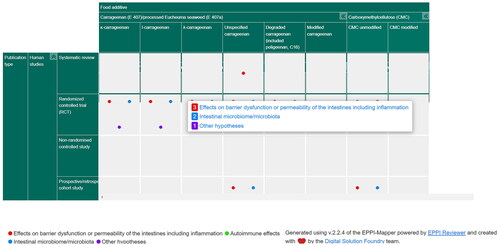
The second interactive research map () is showing various types of CGN and CMC in the columns, human populations (healthy general population or patients) in the rows and hypotheses on the adverse effects on the intestines as bubbles.
Figure 13. Image of the interactive research map showing various types of CGN and CMC in the columns, human populations (healthy general population or patients) in the rows and hypotheses on the adverse effects on the intestines as bubbles. The size of the bubbles indicates the number of studies in each square. From this map, available and missing data in the various categories can be easily seen. The map can be downloaded from Supplementary materials Figure 2 to be used interactively.
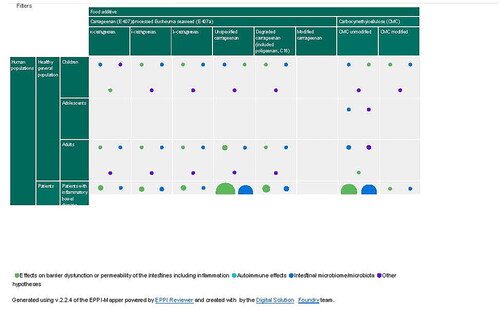
From these maps, available and missing data in the various categories can be easily seen. The research gaps that can be identified from the maps indicate where future research may be useful to carry out in relation to human (healthy persons or patients), animal and in vitro studies (publication types), and which potential adverse effects in the gut should be studied in more detail resulting from the consumption of CGN and CMC.
The interactive research maps are available as HTML files and can be downloaded by clicking on the links below and displayed in any browser, without the need for login. To use the maps interactively, one can click anywhere in a column, row or bubble and access all studies that address the particular food additive, publication type etc.
4. Discussion
4.1. Methodological aspects
Summaries of results from individual studies and across similar studies are usually not included in scoping reviews (Munn et al. Citation2018). However, to get an overview of the existing knowledge of the adverse effects of CGN and CMC, short summaries of the individual publications were included. This was considered particularly necessary for CGN, since some commentaries indicated that adverse effects were only caused by degraded CGN and not by native CGN. Therefore, summaries were made for comparison of effects between native and degraded CGN, as discussed in Section 4.3.1.
Many of the traditional, narrative review publications reported data on both CGN and CMC, as well as on several other food additives. In addition to more general information about these food additives, they gave relevant information about the concerns for adverse health effects, although some mainly focused on positive effects. The commentaries, editorial articles and letters also gave insight into the controversies regarding their potential adverse health effects. A summary of some arguments for or against the likelihood that CGN may have adverse effects can be found in Liao et al. (Citation2021). The conference abstracts were included to pick up newer research indicating adverse effects, however, the reported results were most often covered also by full publications. As opposed to in this scoping review, a future full systematic review on adverse effects of these food additives would include only the primary research publications and available systematic reviews.
4.2. Number of various categories of publications on CGN and CMC
In total, 2572 publications from two literature searches were screened against predefined inclusion/exclusion criteria, and 224 of them were included in this review. In addition, 38 publications from grey literature sources were included, making a total of 262 investigated publications. Of these, 196 and 101 were on CGN and CMC, respectively. The potential adverse effects of CGN or CMC on the intestines have been studied mainly in individual research publications, mostly performed in experimental animals or in vitro. For CGN, five, 69 and 33 studies on humans, experimental animals and in vitro experiments were found, of which two and three, 38 and 31, and 14 and 26 publications studied degraded and native (undegraded) CGN, respectively, in the three publication categories. For CMC, three human studies, 20 experimental animal studies and 14 in vitro studies were obtained. Only one systematic review was found, which was relevant for native CGN in humans, although a few were listed as planned studies (scored as abstracts) in the Cochrane database, but not yet published. Publications (of all types) having information relevant for exposure were 20 and 17 for food grade CGN and CMC, respectively. Publications with data on toxicokinetics and/or ADME were three and two, and risk assessment opinions were two and five, for CGN and CMC, respectively.
High numbers of non-systematic (narrative) review articles were obtained, 54 on CGN and 26 on CMC, reflecting the ongoing controversy about potential adverse effects of these and other food additives, as well as reviewing their use as pharmaceuticals, nutritional or functional food sources or prebiotics. Many of these reviews discussed food additives in general and did not focus specifically on CGN or CMC. Insight into the controversial issues was also gained from the commentaries/editorials/letters, 14 on CGN and nine on CMC. Conferences abstracts, 23 on CGN and 24 on CMC, were included in order to obtained more recent hypotheses, however, most of these were already available as full publications.
The included publications presented several hypotheses on how the two food additives may be associated with adverse effects on the intestines, often studying effects related to several hypotheses in the same publications, and most often, several publications had been studying the same hypotheses and effects.
No other scoping review/scoping study or systematic review on effects of CGN and CMC on the intestines in line with our objectives was detected by search in Medline up to June 12, 2023.
4.3. Main findings on effects of CGN relevant for human intestinal health
Effects on inflammation and effects on the microbiome, including fermentation, were the most studied endpoints both with degraded and native CGN in the research publications across the three evidence streams, whereas permeability of the intestinal barrier was somewhat less studied (), obviously reflecting the search terms used in the literature searches. Higher number of publications had studied inflammation with degraded CGN, because of its use as a model for inflammation, and somewhat higher number had studied the microbiome with native CGN. Effects on the immune system and cancer were also studied with both variants of CGN.
Among the research publications, only five human studies of CGN and effects on the intestines were included, all on adult patients with gastrointestinal conditions, three on native CGN and two on degraded CGN. In the randomized double-blind, placebo-controlled, clinical trial by Bhattacharyya et al. (Citation2017), including 12 UC patients in remission given 100 mg food grade κ-, ι- and λ-CGN or placebo in gelatin capsules, three of five who received CGN relapsed, whereas none of the seven patients receiving placebo relapsed. However, limited weight can be put on this study because of the low number of participants. When it was included in a systematic review on dietary interventions of remission in IBD, Limketkai et al. (Citation2019) calculated a risk ratio with 95% CI of 0.5 (0.15, 1.64) for this study and a very low score for certainty of the evidence (GRADE) and high risk of bias. In the other three human studies in patients with peptic ulcers or malignant disease, which were quite old and without control groups, few and mild adverse effects (dizziness, mild diarrhea, constipation, but no ulceration) were reported either with native or degraded CGN (Heineken Citation1961; Evans et al. Citation1965; Grasso et al. Citation1973). No research publications were found on adverse effects of degraded or native CGN on healthy adults or on children or adolescents.
In addition, of the three publications on human studies that were available only as abstracts, two reported results on a randomized, double-blind, placebo-controlled, cross-over trial (Wagner et al. Citation2018a, Citation2018b), in which 20 healthy males were given 250 mg CGN or placebo twice daily for 14 days. Intestinal permeability increased after CGN vs. placebo (p = 0.03), whereas whole-body insulin sensitivity index (ISI), ISIclamp and OGTT did not differ between the groups. No changes of hepatic fat content, body fat mass, fat distribution, liver transaminases or inflammatory cytokines were observed.
Current understanding of the pathogenesis of IBD suggests an interaction between genetic susceptibility of the immune system to changes in microbiota, likely due to environmental exposures that trigger a dysregulated immune response (Niewiadomski Citation2018). The rapid rise in incidence of IBD over the last few decades also strongly points to the environment playing a significant role, which may include diet in general or food additives specifically. Dietary interventions in patients with IBD are difficult to conclude upon with certainty, since there are many different factors that contribute to the symptoms, and the impact of a complex and varying diet in general is difficult to evaluate. Participants in studies on food additive-free diets most often abstain from foods with many different additives, making it difficult to pin-point any adverse effects to one specific food additive.
Among the 69 publications with studies of CGN in experimental animals, 38 and 31 publications investigated effects of degraded and native CGN, respectively. Several species of experimental animals were used in both groups of studies, in decreasing numbers, rats, guinea pigs, mice, rabbits, hamsters, monkeys, pigs and ferrets. Most of the publications did not give a clear reason for the choice of animal model, however, the large use of guinea pigs and rabbits was mainly from earlier years when animal models of inflammation with degraded CGN were established and investigated for testing of anti-inflammatory drugs or other treatments. The three publications using monkeys, likely being most similar to humans, were all from the 1970s. In two of the studies, adverse effects (intestinal mucosa ulceration, and effects on lysosomes and macrophages, respectively) were observed in Rhesus monkeys given 2% degraded CGN (C16), but not when given native CGN (Benitz et al. Citation1973; Mankes and Abraham Citation1975), whereas a study on two squirrel monkeys given 1.5% degraded CGN for 28 days did not find any adverse effects (Grasso et al. Citation1973).
A large variety of doses and exposure times were used in the animal studies. Concentrations of degraded CGN in diet or drinking water used were from 0.2% to 10% with exposure from 1–2 weeks up to 28 months - the longest studying ulcerations in rabbit (Kitano et al. Citation1986), whereas native CGN was used in concentrations of 0.02% to 20% with exposure from one week up to lifetime - the longest studying cancer in rats and hamsters (Rustia et al. Citation1980). Compared to the exposure from foods consumed by humans, these highest concentrations may not be relevant for studies of effects on human intestinal health of food additives.
Some studies described a model for IBD where rats were sensitized by a s.c. injection of a solution of degraded CGN and Freund’s complete adjuvant followed by oral administration of the same CGN solution in drinking water (Moyana and Lalonde Citation1990, Citation1991). Although not directly relevant for humans exposed to CGN in food, prior sensitization aggravated the ulceration and inflammation in the intestines. Thus, the studies showed that the immune system is involved in these effects. In another inflammation model, mice were given CGN and then later were inoculated with Citrobacter freundii or Citrobacter rodentium bacteria (Wu et al. Citation2017, Citation2021, Citation2022). The results indicated that CGN aggravated the bacteria-induced gut inflammation, which may be relevant for humans having bacterial infections in their gastrointestinal tract. As well as when exposed together with pathogenic microorganisms, synergistic effects of CGN on inflammation were observed when it was given before other inflammatory chemical agents such as trinitrobenzene sulfonic acid (Wu et al. Citation2016a) and oxazolone (Wu et al. Citation2016b).
The order of potency was ι- > λ- > κ-CGN for decreasing effect on body weight gain in guinea pigs given 3% of either degraded κ-, ι- and λ-CGN in drinking water for three weeks, although the mean daily CGN consumption per group was in the reverse order, indicating that potency was not related to intake concentration (Norris et al. Citation1981). Comparable activities of colitis shown by histological score and TNF-α serum levels were induced by native κ-, ι- and λ-CGN given in drinking water for six weeks to mice, and all three decreased the anti-inflammatory gut bacterium Akkermansia muciniphila, although they showed some other differences on the microbiome (Shang et al. Citation2017). Regarding mechanistic differences between the three main forms of CGN, when comparing IL-8 secretion between the native κ-, ι- and λ-CGNs in human cells in vitro, the response was highest for λ-, next for κ- and then ι-CGN (Borthakur et al. Citation2007). There is at present not sufficient evidence to understand potential differences in adverse effects between the various CGN forms.
In total, 33 publications with in vitro experiments reported adverse effects on the intestines of CGN, of which 14 and 26 studies had used degraded and native CGN, respectively. Human colonic microbiota samples from feces studied in an in vitro batch fermentation system showed that high MW κ-CGN (450 or 100 kDa) remained undegraded, whereas low MW κ-CGN, i.e. KCO (∼4.5 kDa) were degraded (Yin et al. Citation2021). In vitro fermentation by human gut microbiota of two κ-CGN oligosaccharides (MW <3000 kDa with different DP), called KO3 and KO6, obtained from simulated gastric digestion, were both readily degraded (Sun et al. Citation2019). A third study also showed that κ-KCO were degraded by Bacteroides xylanisolvens and E. coli isolated from human gut microbiota (Li et al. Citation2017). Thus, at least in vitro, human fecal bacteria appear to be able to degrade CGN oligosaccharides to even lower MW, but apparently not the high MW CGN which is used in food additives.
Some in vitro studies found that native CGN activated the Wnt/β-catenin signaling pathway in human cells, suggesting a role in malignant transformation in the intestine (Bhattacharyya et al. Citation2007; Bhattacharyya et al. Citation2014b). However, when native CGN (Gelcarin, largely composed of κ- and λ-CGN, with MW of approximately 800 kDa), in doses of 0.5, 2.5 or 5% given in the diet to rats and hamsters for life, no significant increase in tumor incidence was seen (Rustia et al. Citation1980). The potential risk of intestinal cancer in humans from a low intake of CGN as food additive needs updated studies, both regarding promoter and initiating effects.
Regarding molecular mechanisms for the adverse effects of CGN, λ-CGN induced inflammation via activation of NF-κB and AP-1 pathways in macrophages and enhanced LPS-induced TNF-α secretion through AP-1 (Chen et al. Citation2014). In this study, degraded λ-CGN was much stronger than native λ-CGN in the activation of macrophages to secrete TNF-α. Native λ-CGN increased Bcl10, IκBα phosphorylation, total and nuclear NF-κB, and secretion of IL-8 in human cell lines, implicating this signaling pathway in intestinal inflammation induced by CGN (Borthakur et al. Citation2007). NF-κB binding to the BCL10 promoter involved the components RelA and RelB, and could lead to prolonged activation of the λ-CGN-induced inflammation by a transcriptional mechanism involving a NF-κB-BCL10 loop (Borthakur et al. Citation2012). κ-CGN did not stimulate IL-8 alone, but increased LPS-induced IL-8 secretion via the Bcl10-NFκB pathway and acted in synergy with LPS to increase the expression of MD-2 and CD14 (Wu et al. Citation2017). Thus, these signaling pathways seems to be important for the effects of CGN, although one publication did not support this view (McKim et al. Citation2015).
4.3.1. Degraded versus non-degraded (native) CGN
Degraded forms of CGN, including PGN, are not approved as food additives in the EU, nor are they used for this purpose in other countries such as USA (EFSA Citation2018a). Thus, the degraded CGN may be regarded as not relevant for the discussion on food additives and human intestinal health, since as such they are not indicative of the potential adverse effects of CGN used in regulated food additives today. It was argued in the literature obtained that the lack of understanding of the difference between non-degraded and degraded CGN, and the misuse of CGN terms, may have contributed to the confusion and misinterpretation of the results in many of the studies, leading to the worries about adverse health effects in the general population and also among scientists (David et al. Citation2018, Citation2019; Weiner and McKim Citation2019). Likewise, it could be argued that degraded CGN and PGN should not have been included in this scoping review. However, the distinction between CGN of different MW was not clear to the authors before this work started. Furthermore, it become apparent from an early look at the literature that even with native, non-degraded CGN there were numerous publications reporting apparently adverse effects. The degraded forms of CGN were therefore included in this review for comparison with native CGN and to get a more complete overview of the controversial issues.
Thus, one of the major controversial issues in this field seems to be differences in health risk from degraded CGN vs. native CGN. A distinction is made between the food grade forms of CGN of high MW (MW 200–800 kDa) used in the food additives E 407/E 407a (EFSA Citation2018a), which are claimed not to be absorbed from the intestinal tract nor being degraded during transit, and degraded CGN such as PGN, C16 and Ebimar (MW 10–20 kDa). The degraded CGN forms are made by low pH (<2.0) and high temperature (>80 °C), and are often used to induce inflammation and ulcerations in the intestinal tract of rats, guinea pigs and rabbits as experimental models of inflammation in general or human IBD. These CGN forms are not the same substances as commercial food grade CGN. The low MW of degraded CGN forms reduces the strength of protein binding, which allows absorption from the intestines (McKim et al. Citation2019). However, in many of the publications, the MW of the CGN (and the form of CGN) was not specified.
The next issue is whether the presence of low MW components in high MW food grade CGN or possible generation of low MW CGN following food processing or gastric hydrolysis of native CGN may cause adverse effects in humans. Trace amounts of native CGN have been shown to cross the intestinal barrier and are demonstrated within intestinal lamina propria cells and macrophages, and are absorbed via Peyer’s patches and cecal lymph nodes in animals (Mankes and Abraham Citation1975; Ishioka et al. Citation1987; Nicklin and Miller Citation1989). Based on the ADME data available, EFSA (Citation2018a) concluded that CGN with high MW, for example κ-/λ-CGN with MW of about 800 kDa studied in Rhesus monkeys (Mankes and Abraham Citation1975), was not absorbed, while low MW CGN (<88 kDa) was found in tissues (liver, intestines) and in urine of rats and guinea pigs after oral administration.
Based on the data provided to EFSA (Citation2018a) after a call for data, the overlap between the low MW-average MW tail of food-grade CGN and high MW-average MW tail of PGN is expected to increase with the decrease of the weight-average MW of the CGN. An example was given: for CGN with the weight-average MW of about 770 kDa, the overlap would encompass from ca. 30 kDa up to ca. 200 KDa molecules. Thus, although for a commercially available CGN with a weight-average MW of 200 kDa, the preparation would encompass a larger fraction with a MW <50 kDa. EFSA concluded that it could not be excluded that commercial CGN preparations may contain considerable amounts of low MW molecules when used as food additive. Therefore, with respect to the definition of E 407 and E 407a in the Commission Regulation (EU) No. 231/2012 (Citation2012), the weight-average MW range should be specified in a narrow way avoiding a significant overlap with the MW range of PGN. Furthermore, there is an urgent need to develop a validated method to quantify the low MW CGN with the limit of 5% (EFSA Citation2018a). Overall, EFSA considered that the information available suggested that the ulceration and bleeding in the intestines reported in some studies having tested native CGN could be associated with the presence of low MW CGN (EFSA Citation2018a).
Regarding risk of cancer, this distinction in MW of CGN is made by IARC, since native (undegraded) CGN has been classified as Group 3, i.e. unclassifiable with respect to carcinogenicity in humans, whereas degraded CGN (PGN) is classified as Group 2B, a possible human carcinogen, based on animal data (IARC Citation1987).
Information about the stability of high MW CGN in food when exposed to various temperature and pH conditions under the authorized food use is also limited and contradictory (EFSA Citation2018a).
4.4. Main findings on effects of CMC relevant for human intestinal health
The three types of research publications reported effects on intestinal microbiota, including studies of fermentation of CMC, inflammatory potential, metabolic effects, effects on intestinal physiology, permeability of the intestinal barrier and cancer ().
Only three human studies on CMC were found, of which a recent study (Chassaing et al. Citation2022a) indicated that CMC in high, but realistic doses, disturbed the intestinal microbiota and led to inflammation. However, the number of subjects in this study was low (in total 16) and they were exposed only for 11 days, thus, such a study needs to be repeated with higher numbers of participants exposed for longer time. In an older study (Anderson et al. Citation1986) with five healthy men given 15 g CMC daily for 23 days, only some inconsistent changes in fecal physiology were observed. One other study (Ohnishi et al. Citation2019) reported that CMC appeared to have caused anaphylaxis in a young girl, although this appears to be a low risk.
Of the 20 experimental animal studies on CMC, most of them used continuous doses of 1–2% CMC in drinking water or other oral exposure in two to 15 weeks, see for instance Viennois et al. (Citation2020) and Rousta et al. (Citation2021). If this is to be transferable to humans, it means that all the food consumed daily contains this concentration of CMC, which is likely an overestimation. However, a high intake of CMC may be possible in some individuals depending on their dietary habits, such as if eating a lot of processed foods. Also of relevance, it is not known whether some persons are more susceptible to adverse effects of CMC than others. The sensitivity to CMC may possibly be species-specific, so that typical laboratory animals such as rodents may react differently to CMC than humans.
As inflammation is implicated in intestinal cancer, some studies tested CMC in various animal models of cancer promotion. One % CMC in drinking water for ca. 14 weeks exacerbated both number of tumors and tumor area after initiation of mice with the colon carcinogen AOM and exposure to DSS to induce inflammation (Viennois et al. Citation2017). In another cancer model, using ApcMin/+ mice, having a mutation (Min, for multiple intestinal neoplasia) in the tumor suppressor gene Apc, involved in the Wnt/β-catenin signaling pathway of colorectal cancer also in humans (Washington and Zemper Citation2019), 1% CMC in drinking water for 15 weeks increased small intestinal tumor development, apparently independent of chronic intestinal inflammation, but associated with CMC’s effects on proliferation in the intestinal epithelium and intestinal microbiota composition (Viennois and Chassaing Citation2021). Thus, there are indications that CMC may act as a tumor promoter in the colon, at least at these doses given in drinking water.
The literature search resulted in 14 in vitro studies on CMC, most of which used human microbiota or cells. Of the six in vitro publications that reported on fermentation of CMC using human donors, four found no effects such as changes in MW or increased formation of SCFA (Bliss et al. Citation2013; Gerasimidis et al. Citation2020; Miclotte et al. Citation2020; He et al. Citation2022), whereas two studies reported increased individual or total SCFA (Bourquin et al. Citation1993; Chen et al. Citation2020). Also, for changes in the microbiota composition, the results varied between the publications. Overall, it appeared that the resulting effects may vary strongly depending on the feces microbiota donor, possibly indicating individual susceptibility in the population.
4.5. Strengths and limitations of this scoping review
This scoping review provides an overview of type of research published on adverse effects on the intestines of the food additives CGN/PES (E 407/E 407a) and CMC (E 466). The strength of this scoping review is that it is based on extensive literature searches comprising six library databases as well as grey literature, without any time limit. The publications have been selected in a systematic way, to provide a transparent overview of the current literature available about potential adverse effects of CGN and CMC on the intestines. It also included data from three evidence streams; human data, experimental animal data and in vitro data. As such, it is a good starting point for performing a systematic review of adverse effects of these food additives. In addition, missing data within this research area are identified and illustrated in the interactive research maps ( and ), which can be used for planning of further research employing studies in humans or animals on presence of such effects and in vitro studies for the mechanisms behind the effects.
A limitation of this scoping review is that quality assessments of the included studies and evaluation of risk of bias of the overall body of publications were not performed. However, the objective of this work was not to give a final answer to whether these food additives constitute a risk to intestinal health or not. In general, the aim of scoping reviews is to provide extensive and transparent overviews of knowledge on a specific topic, in which the literature is systematically identified, selected and presented in text and visually, rather than performing a qualitative or quantitative synthesis of the data in order to assess the causality, or size and severity of potential adverse effects of a stressor. Thus, the quality assessment step is regarded as not strictly necessary for scoping reviews as opposed to in systematic reviews of causal relationship between, as for this topic, the food additives CGN and CMC, and their adverse effects on the intestines (Munn et al. Citation2018; Peters et al. Citation2021b; Pollock et al. Citation2022).
Not all the data categorized from the publications fitted one ER code precisely because of overlap within the publications of more than one study design, several streams of data, several animal species etc. Thus, the coding system in ER used to categorize the publications may not be entirely consistent and exclusive, although the aim of each category (code) was to be as specific as possible. This mixture of types of data within the publications means that the total numbers of publications in a category are often larger than what would be expected from merely addition of numbers.
This scoping review is limited to effects on the intestines, not other organs. The literature searches did not include all languages. Thus, although extensive, this scoping review is not considered a complete overview of publications on adverse effects of CGN and CMC.
4.6. Evidence gaps and implications of the study findings for further research
Two interactive research maps showing various types of CGN and CMC in the columns, type of publications or human populations in the rows, and hypotheses on the adverse effects on the intestines as bubbles are illustrated in and . These maps can be used interactively via the links in Section 3.4. The size of the bubbles indicates the number of studies in each square, and thus, available and missing data in the various categories can be easily seen. The references to the publications constituting this map can be downloaded directly from the map.
In a review, David et al. (Citation2019) highlighted three unresolved information gaps for CGN. These were 1) insufficient information on the current levels of public exposure to CGN, 2) a better understanding of the link between CGN’s physicochemical properties, its impact on digestive proteolysis and the effects on the microbiome and inflammation, and 3) how the digestion of CGN in the intestines may differentially affect susceptible subjects in the population, such as children or elderly persons, or patients with intestinal diseases.
The evidence analyzed in this scoping review suggests that CGN, both degraded and native, and CMC, may cause adverse effects on intestinal health, predominantly based on studies in experimental animals and in vitro studies. These adverse effects include decreased barrier function and/or increased permeability of the intestines, leading to influx of bacteria or chemicals, causing inflammation, often concomitantly with potentially adverse changes in the intestinal microbiota. However, since very few such publications were available on human subjects, more research is needed in this area before one can draw any firm conclusions on the effects on humans. Also, the risk of cancer from food additives acting as tumor promoters in animal models is more uncertain in humans. There is a lack of human studies on the healthy general population, especially children and adolescents, on whom very few publications were found, and better data are also needed for patients with various intestinal disease conditions. The human studies should preferably be RCT as this is defined as the gold standard for human studies, and of longer duration to examine the effects of long-term exposure to the food additives. Studies using CGN or CMC as a dietary supplement compared to a placebo supplement are probably not comparable with the real-life dietary exposure to additives in foods. The results may be more realistic by comparing a diet with or without a specific additive.
Older studies finding adverse effects, especially inflammation, from degraded CGN are not representative of the CGN authorized for use as a food additive (E 407/E 407a) today. However, many publications conclude that adverse effects are also found in studies on native CGN. Compared to the exposure from foodstuffs consumed by humans, the highest concentrations of CGN or CMC used in the experimental animal studies may not be relevant for studies of effects on human intestinal health. Both CGN and CMC are allowed at QS in most food categories, however, there is a lack of data on actual use levels in each food category, as well as updated information on consumption of each category of food for all age groups in the population.
Knowledge on the real-life exposure to the food additives in all population groups is of importance for their risk assessment, as for all other chemical substances. Dietary studies are difficult to perform, and it must be taken into account that the intake of food additives will vary from individual to individual, over time and across national borders. National food surveys should include data on concentrations of various food additives present in the consumed foods, as well as intake of the relevant foods. A problem is that such national surveys are performed rather rarely, and thus, may not be representative of new food products on the market and the food additives therein, for example such as the recently increased number of highly processed vegan food products made to replace meat products.
Another issue is whether the research from experimental animals and in vitro model systems is representative of effects of CGN or CMC in humans when being exposed to them as food additives. Especially, it is questionable whether exposure to CGN or CMC in drinking water used in many animal experiments as the sole source of liquid is representative of dietary exposure to additives in humans from varying types of foods. Food has a more complex matrix where the additives may be bound to proteins, affecting their absorption differently from water (EFSA Citation2018a).
5. Conclusions
Of the two food additives investigated in this scoping review, CGN appears to be the one for which further research is most urgently needed. Based on the information obtained, it appears to be reasonably established that degraded CGN (with low MW), including the artificially manufactured products such as PGN, C16 or Ebimar, is absorbed and may be present in various animal tissues. Degraded CGN has been extensively used as an experimental animal model of inflammation. Thus, degraded CGN appears clearly adverse in experimental animals, causing colitis and possibly cancer, and likely may do so also in humans, depending on the actual exposure (administration route, matrix and dose). The role of native, non-degraded CGN is less clear, however, a substantial number of publications reported adverse effects on the intestines also of this form of CGN in experimental animals, supported by plausible mechanistic data from in vitro studies. Also for CMC, the available animal studies indicate that it may have potentially adverse effects on the intestines, supported by mechanistic data from in vitro studies. However, the relevance of these studies for human intake of CMC as a food additive is unclear. For both food additives, their role in reducing the intestinal barrier and increasing permeability of the intestines to chemicals or bacteria, and their impact on the gut microbiota, needs further clarification.
6. Recommendations for further work on adverse effects of CGN and CMC
For further research of potential adverse health effects of CGN, we recommend to focus the studies on the forms of CGN used as food additives, i.e. food-grade CGN, according to Commission Regulation (EU) No. 231/2012 (Citation2012), and to characterize and specify exactly which form(s) of CGN being present and their levels, including the distribution of MW within the product.
For both CGN and CMC, new human studies, preferably RCT, should have sufficient power, realistic dietary intake levels, performed in healthy persons of all age groups, and also in potentially vulnerable population groups such as young children, pregnant women and elderly persons, as well as in relevant patient groups, such as those with IBS, IBD, CD and UC. One additive instead of several should be studied at the time in order to be able to conclude on adversity of a specific additive. Good quality, longer-term animal studies with dietary exposure to human relevant doses would also still be useful, and the choice of animal model should be justified.
Future research should be performed in a transparent and systematic way, with the hypothesis under scrutiny stated up front, to gain trust from the public. In a future systematic review on these food additives, quality assessments of the included studies should be performed to better interpret and understand their impact on the overall evidence. Future risk assessments of CGN and CMC should include studies now available on permeability and disturbance of barrier properties of the intestines and effects on the microbiome, representing relatively more recently occurring hypotheses on potentially adverse effects than inflammation.
Abbreviation | ||
ACF | = | aberrant crypt foci |
ADI | = | acceptable daily intake |
ADME | = | absorption, distribution, metabolism and excretion |
AIEC | = | adherent-invasive E. coli |
AIM | = | Australian Inflammatory Bowel Disease Microbiome study |
AOM | = | azoxymethane |
APC | = | adenomatous polyposis coli |
BCFA | = | branch chain fatty acids |
Bcl/BCL | = | B-cell lymphoma/leukemia |
bw | = | body weight |
CD | = | Crohn’s disease |
CGN | = | carrageenan |
CMC | = | carboxymethylcellulose, DP: degree of polymerization |
EC | = | European Commission |
EEN | = | exclusive enteral nutrition |
EFSA | = | European Food Safety Authority |
EGR-1 | = | early growth response gene 1 |
ER | = | Eppi-Reviewer |
EU | = | European Union |
FC | = | food category |
GAG | = | glycosaminoglycan |
HTML | = | hypertext markup language |
IAP | = | intestinal alkaline phosphatase |
IARC | = | International Agency for Research on Cancer |
IBD | = | inflammatory bowel disease |
IBS | = | irritable bowel syndrome |
Ig | = | immunoglobulin |
IL | = | interleukin |
IOIBD | = | International Organization for the Study of Inflammatory Bowel Disease |
JECFA | = | Joint FAO/WHO Expert Committee on Food Additives |
KCO | = | κ-CGN oligosaccharides |
LPS | = | lipopolysaccharide |
MPL | = | maximum permitted level |
MPO | = | myeloperoxidase |
M-SHIME | = | mucosal simulator of the human intestinal microbial ecosystem |
MW | = | molecular weight |
NIPH | = | Norwegian Institute of Public Health |
NOAEL | = | no observed adverse effect level |
PBM | = | peripheral blood monocytes |
PES | = | processed Eucheuma seaweed |
PGN | = | poligeenan |
QS | = | quantum satis |
RCT | = | randomized controlled trial |
ROS | = | reactive oxygen species |
SCF | = | Scientific Committee on Food |
SCFA | = | short-chain fatty acids |
SD | = | standard deviation |
TLR | = | Toll-like receptor |
TNF | = | tumor necrosis factor |
UC | = | ulcerative colitis |
Acknowledgments
The authors would like to thank Ragnhild Agathe Tornes, the NIPH library, for performing the literature searches. Håkon Valen and Gunn Elisabeth Vist at NIPH, and Zak Ghouze at University College London, are thanked for good advice and help with EPPI-Reviewer. The authors gratefully acknowledge the comments of the Editor and the external reviewers selected by the Editor who were anonymous to the authors.
Declaration of interest
This research did not receive any grants from funding agencies in the public, commercial or not-for-profit sectors, and the work was funded only by regular employment and engagements of the authors at NIPH. The motivation for this work was questions to NIPH from the public about the safety of these food additives. The authors report no conflicts of interests. The authors have not participated in and do not anticipate participation in any legal, regulatory or advocacy proceedings related to the contents of the paper.
References
- Al-Suhail AA, Reid PE, Culling CFA, Dunn WL, Clay MG. 1984a. Studies of the degraded carrageenan-induced colitis of rabbits. I. Changes in the epithelial glycoprotein O-acylated sialic acids associated with ulceration. Histochem J. 16(5):543–553. doi: 10.1007/bf01041354.
- Al-Suhail AA, Reid PE, Culling CFA, Dunn WL, Clay MG. 1984b. Studies of the degraded carrageenan-induced colitis of rabbits. II. Changes in the epithelial glycoprotein O-acylated sialic acids associated with the induction and healing phases. Histochem J. 16(5):555–564. doi: 10.1007/bf01041355.
- Anderson DMW, Eastwood MA, Brydon WG. 1986. The dietary effects of sodium carboxymethylcellulose in man. Food Hydrocolloids. 1(1):37–44. doi: 10.1016/S0268-005X(86)80005-4.
- Andreev-Andrievskiy AA, Zinovkin RA, Mashkin MA, Frolova OY, Kazaishvili YG, Scherbakova VS, Rudoy BA, Nesterenko VG. 2021. Gene expression pattern of Peyer’s patch lymphocytes exposed to kagocel suggests pattern-recognition receptors mediate its action. Front Pharmacol. 12:679511. doi: 10.3389/fphar.2021.679511.
- Anver MR, Cohen BJ. 1976. Animal model of human disease. Ulcerative colitis. Animal Model: ulcerative colitis induced in guinea pigs with degraded carrageenan. Am J Pathol. 84(2):431–434. https://www.ncbi.nlm.nih.gov/pmc/articles/PMC2032452/.
- Aoki K. 1978. A study of endotoxemia in ulcerative colitis and Crohn’s disease. II. Experimental study. Acta Med Okayama. 32(3):207–216. https://pubmed.ncbi.nlm.nih.gov/151486/.
- Ariffin SHZ, Yeen WW, Abidin IZZ, Wahab RMA, Ariffin ZZ, Senafi S. 2014. Cytotoxicity effect of degraded and undegraded kappa and iota carrageenan in human intestine and liver cell lines. BMC Complement Altern Med. 14(1):508. doi: 10.1186/1472-6882-14-508.
- Arksey H, O'Malley L. 2005. Scoping studies: towards a methodological framework. Int J Soc Res Methodol. 8(1):19–32. doi: 10.1080/1364557032000119616.
- Axelsson LG, Midtvedt T. 1997. Sulfation and MW-optimized carrageenan induces colon inflammation in germfree mice. Gastroenterology. 112(4):A925.
- Bancil AS, Sandall AM, Rossi M, Chassaing B, Lindsay JO, Whelan K. 2021. Food additive emulsifiers and their impact on gut microbiome, permeability, and inflammation: mechanistic insights in inflammatory bowel disease. J Crohns Colitis. 15(6):1068–1079. doi: 10.1093/ecco-jcc/jjaa254.
- Bär A, Van Ommen B, Timonen M. 1995. Metabolic disposition in rats of regular and enzymatically depolymerized sodium carboxymethylcellulose. Food Chem Toxicol. 33(11):901–907. doi: 10.1016/0278-6915(95)00068-d.
- Behr C, Cameron HJ, Strauss V, Walk T, van Ravenzwaay B. 2018. Baseline variability of the gut microbial community and related metabolic changes in plasma and feces. Naunyn-Schmiedeberg’s Arch Pharmacol. 391(Suppl. 1):S46–S47. Abstract no. P96. doi: 10.1007/s00210-018-1477-5.
- Benard C, Cultrone A, Michel C, Rosales C, Segain J-P, Lahaye M, Galmiche J-P, Cherbut C, Blottière HM. 2010. Degraded carrageenan causing colitis in rats induces TNF secretion and ICAM-1 upregulation in monocytes through NF-κB activation. PLoS One. 5(1):e8666. doi: 10.1371/journal.pone.0008666.
- Benitz K-F, Golberg L, Coulston F. 1973. Intestinal effects of carrageenans in the Rhesus monkey (Macaca mulatta). Food Cosmet Toxicol. 11(4):565–575. doi: 10.1016/s0015-6264(73)80327-x.
- Bhattacharyya S, Borthakur A, Dudeja PK, Tobacman JK. 2007. Carrageenan reduces bone morphogenetic protein-4 (BMP4) and activates the Wnt/β-catenin pathway in normal human colonocytes. Dig Dis Sci. 52(10):2766–2774. doi: 10.1007/s10620-006-9531-4.
- Bhattacharyya S, Borthakur A, Dudeja PK, Tobacman JK. 2008a. Carrageenan induces cell cycle arrest in human intestinal epithelial cells in vitro. J Nutr. 138(3):469–475. doi: 10.1093/jn/138.3.469.
- Bhattacharyya S, Borthakur A, Dudeja PK, Tobacman JK. 2010a. Prolonged activation of NFκB (RelA and RelB), BCL10, and IL-8 following exposure to common food additive carrageenan in human colonic epithelial cells may be attributable to NFκB binding site in BCL10 promoter. Cancer Res. 70(8_Supplement):5734–5734. doi: 10.1158/1538-7445.AM10-5734.
- Bhattacharyya S, Dudeja PK, Tobacman JK. 2008b. Carrageenan-induced NFκB activation depends on distinct pathways mediated by reactive oxygen species and Hsp27 or by Bcl10. Biochim Biophys Acta. 1780(7-8):973–982. doi: 10.1016/j.bbagen.2008.03.019.
- Bhattacharyya S, Dudeja PK, Tobacman JK. 2010b. Tumor necrosis factor α-induced inflammation is increased but apoptosis is inhibited by common food additive carrageenan. J Biol Chem. 285(50):39511–39522. doi: 10.1074/jbc.M110.159681.
- Bhattacharyya S, Feferman L, Tobacman JK. 2014a. Carrageenan increase in Wnt9a expression is mediated by arylsulfatase B (N-acetylgalactosamine-4-sulfatase), chondroitin-4-sulfate, galectin-3, and SP-1. Gastroenterology. 146(5)(Suppl 1):S-812. Abstract no. Tu1659. doi: 10.1016/s0016-5085(14)62935-8.
- Bhattacharyya S, Feferman L, Tobacman JK. 2014b. Increased expression of colonic Wnt9A through Sp1-mediated transcriptional effects involving arylsulfatase B, chondroitin 4-sulfate, and galectin-3. J Biol Chem. 289(25):17564–17575. doi: 10.1074/jbc.M114.561589.
- Bhattacharyya S, Gill R, Chen ML, Zhang F, Linhardt RJ, Dudeja PK, Tobacman JK. 2008c. Toll-like receptor 4 mediates induction of the Bcl10-NFκB-interleukin-8 inflammatory pathway by carrageenan in human intestinal epithelial cells. J Biol Chem. 283(16):10550–10558. doi: 10.1074/jbc.M708833200.
- Bhattacharyya S, Liu H, Zhang Z, Jam M, Dudeja PK, Michel G, Linhardt RJ, Tobacman JK. 2010c. Carrageenan-induced innate immune response is modified by enzymes that hydrolyze distinct galactosidic bonds. J Nutr Biochem. 21(10):906–913. doi: 10.1016/j.jnutbio.2009.07.002.
- Bhattacharyya S, Shumard T, Xie H, Dodda A, Varady KA, Feferman L, Halline AG, Goldstein JL, Hanauer SB, Tobacman JK. 2017. A randomized trial of the effects of the no-carrageenan diet on ulcerative colitis disease activity. Nutr Healthy Aging. 4(2):181–192. doi: 10.3233/NHA-170023.
- Bhattacharyya S, Tobacman JK. 2011. Common food additive carrageenan activates polyp formation pathways by reactive oxygen species, not by TLR4-BCL10 inflammatory cascade. Gastroenterology. 140(5)(Suppl 1):S-349. Abstract no. Sa1927. doi: 10.1016/s0016-5085(11)61420-0.
- Bhattacharyya S, Tobacman JK. 2012. Common food additive carrageenan activates wnt signaling pathway in mouse colon and human colonic epithelial cells. Cancer Res. 72(8_Supplement):163–163. doi: 10.1158/1538-7445.AM2012-163.
- Bhattacharyya S, Xue L, Devkota S, Chang E, Morris S, Tobacman JK. 2013. Carrageenan-induced colonic inflammation is reduced in Bcl10 null mice and increased in IL-10-deficient mice. Mediators Inflamm. 2013:397642. doi: 10.1155/2013/397642.
- Bixler HJ. 2017. The carrageenan controversy. J Appl Phycol. 29(5):2201–2207. doi: 10.1007/s10811-017-1132-4.
- Bliss DZ, Weimer PJ, Jung H-JG, Savik K. 2013. In vitro degradation and fermentation of three dietary fiber sources by human colonic bacteria. J Agric Food Chem. 61(19):4614–4621. doi: 10.1021/jf3054017.
- Bonfils S. 1970. Carrageenan and the human gut. Lancet. 296(7669):414–414. doi: 10.1016/s0140-6736(70)90022-x.
- Bordon Y. 2015. Mucosal immunology: food additives feed the fire. Nat Rev Immunol. 15(4):200. doi: 10.1038/nri3833.
- Borsani B, De Santis R, Perico V, Penagini F, Pendezza E, Dilillo D, Bosetti A, Zuccotti GV, D'Auria E. 2021. The role of carrageenan in inflammatory bowel diseases and allergic reactions: where do we stand? Nutrients. 13(10):3402. doi: 10.3390/nu13103402.
- Borthakur A, Bhattacharyya S, Anbazhagan AN, Kumar A, Dudeja PK, Tobacman JK. 2012. Prolongation of carrageenan-induced inflammation in human colonic epithelial cells by activation of an NFκB-BCL10 loop. Biochim Biophys Acta. 1822(8):1300–1307. doi: 10.1016/j.bbadis.2012.05.001.
- Borthakur A, Bhattacharyya S, Dudeja PK, Tobacman JK. 2007. Carrageenan induces interleukin-8 production through distinct Bcl10 pathway in normal human colonic epithelial cells. Am J Physiol Gastrointest Liver Physiol. 292(3):G829–G838. doi: 10.1152/ajpgi.00380.2006.
- Bourquin LD, Titgemeyer EC, Fahey GC Jr, Garleb KA. 1993. Fermentation of dietary fibre by human colonic bacteria: disappearance of, short-chain fatty acid production from, and potential water-holding capacity of, various substrates. Scand J Gastroenterol. 28(3):249–255. doi: 10.3109/00365529309096081.
- Bowen-Yacyshyn MB, Dieter L, Yacyshyn BR. 2005. Carboxy-methylcellulose (CMC) alters intestinal permeability, decreases inflammation and increases CEACAM1 isoform expression in the HLA-B27 transgenic rat. Gastroenterology. 128(4):A280. Abstract no. S1907. https://www.gastrojournal.org/action/showPdf?pii=S0016-5085%2805%2900640-2.
- Boxenbaum HG, Dairman W. 1977. Evaluation of an animal model for the screening of compounds potentially useful in human ulcerative colitis: effect of salicylazosulfapyridine and prednisolone on carrageenan-induced ulceration of the large intestine of the guinea pig. Drug Dev Ind Pharm. 3(2):121–130. doi: 10.3109/03639047709051919.
- Cani PD. 2015. Dietary emulsifiers - sweepers of the gut lining? Nat Rev Endocrinol. 11(6):319–320. doi: 10.1038/nrendo.2015.59.
- Carthew P. 2002. Safety of carrageenan in foods. Environ Health Perspect. 110(4):A176; author reply A176–A176; author reply A177. doi: 10.1289/ehp.110-a176a.
- Chassaing B, Compher C, Bonhomme B, Liu Q, Tian Y, Walters W, Nessel L, Delaroque C, Hao F, Gershuni V, et al. 2022a. Randomized controlled-feeding study of dietary emulsifier carboxymethylcellulose reveals detrimental impacts on the gut microbiota and metabolome. Gastroenterology. 162(3):743–756. doi: 10.1053/j.gastro.2021.11.006.
- Chassaing B, De Bodt J, Marzorati M, Van de Wiele T, Gewirtz AT. 2017a. Dietary emulsifiers directly alter human microbiota composition and gene expression ex vivo potentiating intestinal inflammation. Gut. 66(8):1414–1427. doi: 10.1136/gutjnl-2016-313099.
- Chassaing B, Gershuni V, McKeever L, Compher C, Bonhomme B, Harb AH, Nessel L, Wu GD, Gewirtz AT, Lewis JD. 2020. A randomized controlled-feeding study of the impact of the synthetic dietary emulsifier carboxymethylcellulose on human health and intestinal microbiota. Gastroenterology. 158(6)(Suppl 1):S–812. Abstract no. 1008. doi: 10.1016/s0016-5085(20)31184-7.
- Chassaing B, Gewirtz A. 2013. Food additives promote intestinal inflammation in susceptible hosts. Inflammatory Bowel Dis. 19(Suppl 1):S115–S116. Abstract no. P-231 YI. doi: 10.1097/01.MIB.0000439029.44015.b6.
- Chassaing B, Gewirtz AT. 2014. Food additives promote intestinal inflammation in susceptible hosts. Gastroenterology. 146(5)(Suppl 1):S-286. Abstract no. Sa1745. doi: 10.1016/s0016-5085(14)61016-7.
- Chassaing B, Gewirtz AT. 2017a. Dietary emulsifiers directly impact the transcriptome of a Crohn’s disease-associated Escherichia coli, increasing its ability to induce intestinal inflammation. United Eur Gastroenterol J. 5(5 Suppl):A29. Abstract no. OP067. doi: 10.1177/2050640617725668.
- Chassaing B, Gewirtz AT. 2017b. Pathobiont-free microbiota protects against gut inflammation induced by an innate immune deficiency or dietary perturbant of the microbiota. United Eur Gastroenterol J. 5(5 Suppl):A495–A496. Abstract no. P0956. doi: 10.1177/2050640617725676.
- Chassaing B, Gewirtz AT, Lewis JD. 2022b. Reply. J. Gastroenterol. 163(3):781–782. doi: 10.1053/j.gastro.2022.05.021.
- Chassaing B, Koren O, Goodrich JK, Poole AC, Srinivasan S, Ley RE, Gewirtz AT. 2015. Dietary emulsifiers impact the mouse gut microbiota promoting colitis and metabolic syndrome. Nature. 519(7541):92–96. https://www.nature.com/articles/nature14232. doi: 10.1038/nature14232.
- Chassaing B, Van De Wiele T, Gewirtz A. 2016. Dietary emulsifiers directly impact the human gut microbiota increasing its pro-inflammatory potential. United Eur Gastroenterol J. 4(5 Suppl 1):A93. Abstract no. OP234. doi: 10.1177/2050640616663688.
- Chassaing B, Van de Wiele T, Gewirtz A. 2017b. Dietary emulsifiers directly impact the human gut microbiota increasing its proinflammatory potential and ability to induce intestinal inflammation. Inflammatory Bowel Dis. 23(Suppl 1):S5. Abstract no. O-013. https://academic.oup.com/ibdjournal/article/23/suppl_1/S5/4561168.
- Chazelas E, Druesne-Pecollo N, Esseddik Y, Szabo de Edelenyi F, Agaesse C, De Sa A, Lutchia R, Rebouillat P, Srour B, Debras C, et al. 2021. Exposure to food additive mixtures in 106,000 French adults from the NutriNet-Santé cohort. Sci Rep. 11(1):19680. doi: 10.1038/s41598-021-98496-6.
- Chen H, Wang F, Mao H, Yan X. 2014. Degraded λ-carrageenan activates NF-κB and AP-1 pathways in macrophages and enhances LPS-induced TNF-α secretion through AP-1. Biochim Biophys Acta. 1840(7):2162–2170. doi: 10.1016/j.bbagen.2014.03.011.
- Chen M, Fan B, Liu S, Imam KMSU, Xie Y, Wen B, Xin F. 2020. The in vitro effect of fibers with different degrees of polymerization on human gut bacteria. Front Microbiol. 11:819. doi: 10.3389/fmicb.2020.00819.
- Choi HJ, Kim H-G, Kim J, Park S-H, Park J, Oh CG, Do KH, Lee SJ, Park YC, Ahn SC, et al. 2014. Pro-apoptotic action of macrophage inhibitory cytokine 1 and counteraction of activating transcription factor 3 in carrageenan-exposed enterocytes. Toxicol Lett. 231(1):1–8. doi: 10.1016/j.toxlet.2014.08.022.
- Choi HJ, Kim J, Park S-H, Do KH, Yang H, Moon Y. 2012. Pro-inflammatory NF-κB and early growth response gene 1 regulate epithelial barrier disruption by food additive carrageenan in human intestinal epithelial cells. Toxicol Lett. 211(3):289–295. doi: 10.1016/j.toxlet.2012.04.012.
- Cian RE, Drago SR, de Medina FS, Martinez-Augustin O. 2015. Proteins and carbohydrates from red seaweeds: evidence for beneficial effects on gut function and microbiota. Mar Drugs. 13(8):5358–5383. doi: 10.3390/md13085358.
- Cochrane Central Register of Controlled Trials. 2018. Functional research of emulsifiers in humans. NCT03440229, ID Number: CN-01523172. [accessed 2023 May 25]. https://clinicaltrials.gov/ct2/show/NCT03440229.
- Cochrane Central Register of Controlled Trials. 2021. Carrageenan in ulcerative colitis. ISRCTN90354393, 2021 (12), ID Number: CN-02351798. [accessed 2023 May 25]. https://trialsearch.who.int/Trial2.aspx?TrialID=ISRCTN90354393.
- Cohen SM, Ito N. 2002. A critical review of the toxicological effects of carrageenan and processed Eucheuma seaweed on the gastrointestinal tract. Crit Rev Toxicol. 32(5):413–444. doi: 10.1080/20024091064282.
- Commission Regulation (EU) No. 231/2012. 2012. Laying down specifications for food additives listed in Annexes II and III to Regulation (EC) No 1333/2008 of the European Parliament and of the Council. 9 March 2012, OJ L 83, 22.3.2012, p. 1–295. [accessed 2023 May 25]. https://eur-lex.europa.eu/legal-content/EN/TXT/?uri=celex:32012R0231.
- Cox S, Sandall A, Smith L, Rossi M, Whelan K. 2021. Food additive emulsifiers: a review of their role in foods, legislation and classifications, presence in food supply, dietary exposure, and safety assessment. Nutr Rev. 79(6):726–741. doi: 10.1093/nutrit/nuaa038.
- Cox SR, Sandall AM. 2021. Food-additive emulsifiers: the worst thing since sliced bread? Lancet Gastroenterol Hepatol. 6(7):532. https://www.thelancet.com/journals/langas/article/PIIS2468-1253(21)00189-8/fulltext. doi: 10.1016/S2468-1253(21)00189-8.
- Daudt HML, van Mossel C, Scott SJ. 2013. Enhancing the scoping study methodology: a large, inter-professional team’s experience with Arksey and O'Malley’s framework. BMC Med Res Methodol. 13(1):48. doi: 10.1186/1471-2288-13-48.
- David S, Fahoum L, Rozen G, Shaoul R, Shpigelman A, Meyron-Holtz EG, Lesmes U. 2019. Reply to the Comment on "Revisiting the carrageenan controversy: do we really understand the digestive fate and safety of carrageenan in our foods? by M. Weiner and J. McKim, Food Funct., 2019, 10. doi: 10.1039/C8FO01282B. Food Funct. 10(3):1763–1766. doi: 10.1039/C9FO00018F.
- David S, Levi CS, Fahoum L, Ungar Y, Meyron-Holtz EG, Shpigelman A, Lesmes U. 2018. Revisiting the carrageenan controversy: do we really understand the digestive fate and safety of carrageenan in our foods? Food Funct. 9(3):1344–1352. doi: 10.1039/c7fo01721a.
- Delahunty T, Recher L, Hollander D. 1987. Intestinal permeability changes in rodents: a possible mechanism for degraded carrageenan-induced colitis. Food Chem Toxicol. 25(2):113–118. doi: 10.1016/0278-6915(87)90143-8.
- De Siena M, Raoul P, Costantini L, Scarpellini E, Cintoni M, Gasbarrini A, Rinninella E, Mele MC. 2022. Food emulsifiers and metabolic syndrome: the role of the gut microbiota. Foods. 11(15):2205. doi: 10.3390/foods11152205.
- Dey P. 2019. Gut microbiota in phytopharmacology: a comprehensive overview of concepts, reciprocal interactions, biotransformations and mode of actions. Pharmacol Res. 147:104367. doi: 10.1016/j.phrs.2019.104367.
- Dräger G, Krause A, Möller L, Dumitriu S. 2011. Chapter 7, Carbohydrates. In: Lendlein A and Sisson A, editors. Handbook of biodegradable polymers: synthesis, characterization and applications. Wiley-VCH Verlag GmbH & Co. KGaA, Weinheim, Germany. p. 155–193. https://vdoc.pub/download/handbook-of-biodegradable-polymers-isolation-synthesis-characterization-and-applications-3mtpsl8717hg.
- du Preez R, Paul N, Mouatt P, Majzoub ME, Thomas T, Panchal SK, Brown L. 2020. Carrageenans from the red seaweed Sarconema filiforme attenuate symptoms of diet-induced metabolic syndrome in rats. Mar Drugs. 18(2):97. doi: 10.3390/md18020097.
- [EFSA] European Food Safety Authority FEEDAP Panel (EFSA Panel on Additives and Products or Substances used in Animal Feed), Bampidis V, Azimonti G, Bastos ML, Christensen H, Dusemund B, Kos Durjava M, Kouba M, López-Alonso M, López Puente S, Marcon F, et al. 2020. Scientific Opinion on the safety and efficacy of sodium carboxymethyl cellulose for all animal species. EFSA J. 18(7):6211, 13 p. [accessed 2023 May 25]. doi: 10.2903/j.efsa.2020.6211.
- [EFSA] European Food Safety Authority CEF Panel (EFSA Panel on Food Contact Materials, Enzymes, Flavourings and Processing Aids), Silano V, Bolognesi C, Chipman K, Cravedi J-P, Engel K-H, Fowler P, Franz R, Grob K, Gürtler R, Husøy T, et al. 2018c. Scientific Opinion on the safety assessment of the active substances carboxymethylcellulose, acetylated distarch phosphate, bentonite, boric acid and aluminium sulfate, for use in active food contact materials. EFSA J. 16(2):5121, 7 p. [accessed 2023 May 25]. doi: 10.2903/j.efsa.2018.5121.
- [EFSA] European Food Safety Authority ANS Panel (EFSA Panel on Food Additives and Nutrient Sources added to Food), Younes M, Aggett P, Aguilar F, Crebelli R, Di Domenico A, Dusemund B, Filipič M, Jose Frutos M, Galtier P, Gott D, et al. 2018b. Scientific Opinion on the re-evaluation of celluloses E 460(i), E 460(ii), E 461, E 462, E 463, E 464, E 465, E 466, E 468 and E 469 as food additives. EFSA J. 16(1):5047. 104 p. [accessed 2023 May 25]. doi: 10.2903/j.efsa.2018.5047.
- [EFSA] European Food Safety Authority ANS Panel (EFSA Panel on Food Additives and Nutrient Sources added to Food), Younes M, Aggett P, Aguilar F, Crebelli R, Filipič M, Frutos MJ, Galtier P, Gott D, Gundert-Remy U, Kuhnle GG, et al. 2018a. Scientific Opinion on the re-evaluation of carrageenan (E 407) and processed Eucheuma seaweed (E 407a) as food additives. EFSA J. 16(4):5238, 112 p. [accessed 2023 May 25]. doi: 10.2903/j.efsa.2018.5238.
- [EFSA] European Food Safety Authority CEF Panel (EFSA Panel on food contact materials, enzymes, flavourings and processing aids). 2012. Scientific Opinion on the safety evaluation of the active substances sodium carboxy methyl cellulose, bentonite, aluminium potassium sulphate for use in active food contact materials. EFSA J. 10(10):2904, 9 p. doi: 10.2903/j.efsa.2012.2904. [accessed 2023 May 25].
- [EFSA] European Food Safety Authority FAF Panel (EFSA Panel on Food Additives Flavourings), Younes M, Aquilina G, Castle L, Degen G, Engel K-H, Fowler PJ, Frutos Fernandez MJ, Fürst P, Gürtler R, Husøy T, et al. 2022. Scientific Opinion on the re-evaluation of sodium carboxy methyl cellulose (E 466) as a food additive in foods for infants below 16 weeks of age and follow-up of its re-evaluation as food additive for uses in foods for all population groups. EFSA J. 20(12):7665, 32 p. [accessed 2023 May 25]. doi: 10.2903/j.efsa.2022.7665.
- Elsenhans B, Caspary WF. 1989. Differential changes in the urinary excretion of two orally administered polyethylene glycol markers (PEG 900 and PEG 4000) in rats after feeding various carbohydrate gelling agents. J Nutr. 119(3):380–387. doi: 10.1093/jn/119.3.380.
- Elson CO, Sartor RB, Tennyson GS, Riddell RH. 1995. Experimental models of inflammatory bowel disease. Gastroenterology. 109(4):1344–1367. doi: 10.1016/0016-5085(95)90599-5.
- Evans PRC, Nowell S, Thomas IAP. 1965. Blind trial of degraded carrageenin and aluminium hydroxide gel in the treatment of peptic ulceration. Postgrad Med J. 41(471):48–52. doi: 10.1136/pgmj.41.471.48.
- Fahoum L, Lesmes U, Meyron EG. 2015. Physiologically digested carrageenan affects intestinal barrier function. Inflamm Res. 64(Suppl 2):S155–S156. doi: 10.1007/s00011-015-0839-4.
- Fahoum L, Moscovici A, David S, Shaoul R, Rozen G, Meyron-Holtz EG, Lesmes U. 2017. Digestive fate of dietary carrageenan: evidence of interference with digestive proteolysis and disruption of gut epithelial function. Mol Nutr Food Res. 61(3):1600545. doi: 10.1002/mnfr.201600545.
- Farag A, Zahran NM, Salem AS, Awney HA. 2018. Long-term feeding effect of mono and divalent cation kappa carrageenan on some antioxidant, biochemical and histological parameters of growing male rats. Proc Nutr Soc. 77(OCE2): E44. doi: 10.1017/S0029665118000381.
- Fath RB Jr, Deschner EE, Winawer SJ, Dworkin BM. 1984. Degraded carrageenan-induced colitis in CF1 mice. A clinical, histopathological and kinetic analysis. Digestion. 29(4):197–203. doi: 10.1159/000199033.
- Fujita T, Sakurai K. 1995. Efficacy of glutamine-enriched enteral nutrition in an experimental model of mucosal ulcerative colitis. Br J Surg. 82(6):749–751. doi: 10.1002/bjs.1800820611.
- Gao Y, Wu A, Li Y, Chang Y, Xue C, Tang Q. 2022. The risk of carrageenan-induced colitis is exacerbated under high-sucrose/high-salt diet. Int J Biol Macromol. 210:475–482. doi: 10.1016/j.ijbiomac.2022.04.158.
- Gerasimidis K, Bryden K, Chen X, Papachristou E, Verney A, Roig M, Hansen R, Nichols B, Papadopoulou R, Parrett A. 2020. The impact of food additives, artificial sweeteners and domestic hygiene products on the human gut microbiome and its fibre fermentation capacity. Eur J Nutr. 59(7):3213–3230. doi: 10.1007/s00394-019-02161-8.
- Gibson GR, Cummings JH, Macfarlane GT. 1991. Growth and activities of sulphate-reducing bacteria in gut contents of healthy subjects and patients with ulcerative colitis. FEMS Microbiol Ecol. 86(2):103–111. doi: 10.1111/j.1574-6968.1991.tb04799.x.
- Gkikas K, Gerasimidis K, Milling S, Ijaz UZ, Hansen R, Russell RK. 2020. Dietary strategies for maintenance of clinical remission in inflammatory bowel diseases: are we there yet? Nutrients. 12(7):2018. doi: 10.3390/nu12072018.
- Glade MJ, Meguid MM. 2016. A glance at … dietary emulsifiers, the human intestinal mucus and microbiome, and dietary fiber. Nutrition. 32(5):609–614. doi: 10.1016/j.nut.2015.12.036.
- Goens D, Micic D. 2020. Role of diet in the development and management of Crohn’s disease. Curr Gastroenterol Rep. 22(4):19. doi: 10.1007/s11894-020-0755-9.
- Gotteland M, Riveros K, Gasaly N, Carcamo C, Magne F, Liabeuf G, Beattie A, Rosenfeld S. 2020. The pros and cons of using algal polysaccharides as prebiotics. Front Nutr. 7:163. doi: 10.3389/fnut.2020.00163.
- Goyal N, Rana A, Ahlawat A, Bijjem KRV, Kumar P. 2014. Animal models of inflammatory bowel disease: a review. Inflammopharmacol. 22(4):219–233. doi: 10.1007/s10787-014-0207-y.
- Grasso P, Gangolli SD, Butterworth KR, Wright MG. 1975. Studies of degraded carrageenan in rats and guinea-pigs. Food Cosmet Toxicol. 13(2):195–201. doi: 10.1016/S0015-6264(75)80004-6.
- Grasso P, Sharratt M, Carpanini FMB, Gangolli SD. 1973. Studies on carrageenan and large-bowel ulceration in mammals. Food Cosmet Toxicol. 11(4):555–564. doi: 10.1016/s0015-6264(73)80326-8.
- Gubina-Vakyulyk GI, Gorbach TV, Tkachenko AS, Tkachenko MO. 2015. Damage and regeneration of small intestinal enterocytes under the influence of carrageenan induces chronic enteritis. Comp Clin Pathol. 24(6):1473–1477. doi: 10.1007/s00580-015-2102-3.
- Halmos EP, Mack A, Gibson PR. 2019. Review article: emulsifiers in the food supply and implications for gastrointestinal disease. Aliment Pharmacol Ther. 49(1):41–50. doi: 10.1111/apt.15045.
- Han Z-L, Yang M, Fu X-D, Chen M, Su Q, Zhao Y-H, Mou H-J. 2019. Evaluation of prebiotic potential of three marine algae oligosaccharides from enzymatic hydrolysis. Mar Drugs. 17(3):173. doi: 10.3390/md17030173.
- Harmuth-Hoene A-E, Schwerdtfeger E. 1979. Effect of indigestible polysaccharides on protein digestibility and nitrogen retention in growing rats. Nutr Metab. 23(5):399–407. doi: 10.1159/000176285.
- Hata K, Tanaka T, Kohno H, Suzuki R, Qiang SH, Kuno T, Hirose Y, Hara A, Mori H. 2006. Lack of enhancing effects of degraded λ-carrageenan on the development of β-catenin-accumulated crypts in male DBA/2J mice initiated with azoxymethane. Cancer Lett. 238(1):69–75. doi: 10.1016/j.canlet.2005.06.016.
- He X, Sun C, Fang J, Wu C, Zhang Y, Zhang X, Fang Y. 2022. In vitro colonic fermentation profiles and microbial responses of cellulose derivatives with different colloidal states. J Agric Food Chem. 70(30):9509–9519. doi: 10.1021/acs.jafc.2c01721.
- Heindel JJ, Howard S, Agay-Shay K, Arrebola JP, Audouze K, Babin PJ, Barouki R, Bansal A, Blanc E, Cave MC, et al. 2022. Obesity II: establishing causal links between chemical exposures and obesity. Biochem Pharmacol. 199:115015. doi: 10.1016/j.bcp.2022.115015.
- Heineken TS. 1961. Carrageenan in the management of peptic ulcer. A preliminary report. Am J Gatroenterol. 35:619–621. https://pubmed.ncbi.nlm.nih.gov/13712917/.
- Hibi T, Ogata H, Sakuraba A. 2002. Animal models of inflammatory bowel disease. J Gastroenterol. 37(6):409–417. doi: 10.1007/s005350200060.
- Hirono I, Sumi Y, Kuhara K, Miyakawa M. 1981. Effect of degraded carrageenan on the intestine in germfree rats. Toxicol Lett. 8(4-5):207–212. doi: 10.1016/0378-4274(81)90102-8.
- Ho K, Suskind D, Wahbeh G, Zheng H, Singh N, Nuding M, Lee D. 2021. Evaluating the role of food additives exposures in pediatric Crohn’s disease and ulcerative colitis. J Pediatr Gastroenterol Nutr. 73(Suppl 1):S233–S235.
- Holder MK, Peters NV, Whylings J, Fields CT, Gewirtz AT, Chassaing B, de Vries GJ. 2019. Dietary emulsifiers consumption alters anxiety-like and social-related behaviors in mice in a sex-dependent manner. Sci Rep. 9(1):172. doi: 10.1038/s41598-018-36890-3.
- [IARC] International Agency for Research on Cancer. 1987. IARC Monographs on the evaluation of the carcinogenic risks to humans. Overall evaluations of carcinogenicity: an updating of IARC monographs. Volumes 1 to 42. Supplement (7). [accessed 2023 May 25]. https://publications.iarc.fr/Book-And-Report-Series/Iarc-Monographs-Supplements/Overall-Evaluations-Of-Carcinogenicity-An-Updating-Of-IARC-Monographs-Volumes-1%E2%80%9342-1987.
- Ishioka T, Kuwabara N, Oohashi Y, Wakabayashi K. 1987. Induction of colorectal tumors in rats by sulfated polysaccharides. Crit Rev Toxicol. 17(3):215–244. doi: 10.3109/10408448709071209.
- Jensen BH, Andersen JO, Poulsen SS, Skov Olsen P, Nørby Rasmussen S, Hansen SH, Hvidberg EF. 1984. The prophylactic effect of 5-aminosalicylic acid and salazosulphapyridine on degraded-carrageenan-induced colitis in guinea pigs. Scand J Gastroenterol. 19(3):299–303. doi: 10.1080/00365521.1984.12005725.
- Jiang HY, Wang F, Chen H-M, Yan X-J. 2013. κ-carrageenan induces the disruption of intestinal epithelial Caco-2 monolayers by promoting the interaction between intestinal epithelial cells and immune cells. Mol Med Rep. 8(6):1635–1642. doi: 10.3892/mmr.2013.1726.
- Jimenez Loayza JJ, Berendsen EM, Teh J-J, Hoedt EC, Zhang J, Liu Q, Hamilton AL, Wilson-O'Brien A, Trakman GL, Lin W, et al. 2019. The common food additives sodium sulfite and polysorbate 80 have a profound inhibitory effect on the commensal, anti-inflammatory bacterium Faecalibacterium prausnitzii: the ENIGMA study. J Crohns Colitis. 13(Supplement_1):S542–S543. Abstract no. P837. doi: 10.1093/ecco-jcc/jjy222.961.
- Juśkiewicz J, Zduńczyk Z. 2004. Effects of cellulose, carboxymethylcellulose and inulin fed to rats as single supplements or in combinations on their caecal parameters. Comp Biochem Physiol A Mol Integr Physiol. 139(4):513–519. doi: 10.1016/j.cbpb.2004.10.015.
- Kang S, de Klerk A, Noon E, Teh JJ, Zhang J, Zhang F, Liu Q, Hamilton AL, Wilson-O'Brien A, Trakman GL, et al. 2021. Food additives enhance pro-inflammatory bacterial growth in Crohn’s disease mucosa-associated microbiota. The Enigma Study. Gastroenterology. 160(6 Suppl):S-119. Abstract no. 604. doi: 10.1016/S0016-5085(21)01036-2.
- Kang S, Teh JJ, Schooth L, Zhang J, Hu J, Hamilton AL, Wilson-O'Brien A, Ching JYL, Lin W, Trakman GL, et al. 2022. The food additive carrageenan has differential effects on the growth of the mucosa-associated microbiota from Asian and Western people with Crohn’s disease and healthy controls: the ENIGMA study. J Gastroenterol Hepatol. 37(Suppl 1):110. Abstract no. 299. doi: 10.1111/jgh.15952.
- Kanneganti M, Mino-Kenudson M, Mizoguchi E. 2011. Animal models of colitis-associated carcinogenesis. J Biomed Biotechnol. 2011:342637. doi: 10.1155/2011/342637.
- Kikut J, Konecka N, Ziętek M, Szczuko M. 2018. Inflammatory bowel disease etiology: current knowledge. Pteridines. 29(1):206–214. doi: 10.1515/pteridines-2018-0020.
- Kim H-S, Berstad A. 1992. Experimental colitis in animal models. Scand J Gastroenterol. 27(7):529–537. doi: 10.3109/00365529209000116.
- Kirsch PP. 2002. Carrageenan: a safe additive. Environ Health Perspect. 110(6):A288; author reply A288. doi: 10.1289/ehp.110-a288a.
- Kitano A, Matsumoto T, Hiki M, Hashimura H, Yoshiyasu K, Okawa K, Kuwajima S, Kobayashi K. 1986. Epithelial dysplasia of the rabbit colon induced by degraded carrageenan. Cancer Res. 46(3):1374–1376. https://europepmc.org/article/med/3943102.
- Kitano A, Matsumoto T, Oshitani N, Nakagawa M, Yasuda K, Watanabe Y, Tomobuchi M, Obayashi M, Tabata A, Fukushima R, et al. 1996. Distribution and anti-inflammatory effect of mesalazine on carrageenan-induced colitis in the rabbit. Clin Exp Pharmacol Physiol. 23(4):305–309. doi: 10.1111/j.1440-1681.1996.tb02828.x.
- Kitsukawa Y, Saito H, Suzuki Y, Kasanuki J, Tamura Y, Yoshida S. 1992. Effect of ingestion of eicosapentaenoic acid ethyl ester on carrageenan-induced colitis in guinea pigs. Gastroenterology. 102(6):1859–1866. doi: 10.1016/0016-5085(92)90306-j.
- Kuang R, O'Keefe SJD, del Aguila de Rivers CR, Koutroumpakis F, Binion DG. 2023. Is salt at fault? Dietary salt consumption and inflammatory bowel disease. Inflamm Bowel Dis. 29(1):140–150. doi: 10.1093/ibd/izac058.
- Lallès J-P, Boudry G, Favier C, Sève B. 2006. High-viscosity carboxymethylcellulose reduces carbachol-stimulated intestinal chloride secretion in weaned piglets fed a diet based on skimmed milk powder and maltodextrin. Br J Nutr. 95(3):488–495. doi: 10.1079/bjn20051673.
- Langman JM, Rowland R, Vernon-Roberts B. 1985. Carrageenan colitis in the guinea pig: pathological changes and the importance of ascorbic acid deficiency in disease induction. Aust J Exp Biol Med Sci. 63(5):545–553. doi: 10.1038/icb.1985.58.
- Laster J, Bonnes SL, Rocha J. 2019. Increased use of emulsifiers in processed foods and the links to obesity. Curr Gastroenterol Rep. 21(11):61. doi: 10.1007/s11894-019-0723-4.
- Laudisi F, Stolfi C, Monteleone G. 2019. Impact of food additives on gut homeostasis. Nutrients. 11(10):2334. doi: 10.3390/nu11102334.
- Lee D, Swan CK, Suskind D, Wahbeh G, Vanamala J, Baldassano RN, Leonard MB, Lampe JW. 2018. Children with Crohn’s disease frequently consume select food additives. Dig Dis Sci. 63(10):2722–2728. doi: 10.1007/s10620-018-5145-x.
- Levac D, Colquhoun H, O'Brien KK. 2010. Scoping studies: advancing the methodology. Implementation Sci. 5:69. doi: 10.1186/1748-5908-5-69.
- Levine A, Rhodes JM, Lindsay JO, Abreu MT, Kamm MA, Gibson PR, Gasche C, Silverberg MS, Mahadevan U, Boneh RS, et al. 2020. Dietary guidance from the International Organization for the Study of Inflammatory Bowel Diseases. Clin Gastroenterol Hepatol. 18(6):1381–1392. doi: 10.1016/j.cgh.2020.01.046.
- Levine AR, Picoraro JA, Dorfzaun S, Leleiko NS. 2022. Emulsifiers and intestinal health: an introduction. J Pediatr Gastroenterol Nutr. 74(3):314–319. doi: 10.1097/mpg.0000000000003361.
- Li M, Shang Q, Li G, Wang X, Yu G. 2017. Degradation of marine algae-derived carbohydrates by Bacteroidetes isolated from human gut microbiota. Mar Drugs. 15(4):92. doi: 10.3390/md15040092.
- Li S, Hu J, Yao H, Geng F, Nie S. 2023. Interaction between four galactans with different structural characteristics and gut microbiota. Crit Rev Food Sci Nutr. 63(19):3653–3663. doi: 10.1080/10408398.2021.1992605.
- Liao Y-C, Chang C-C, Nagarajan D, Chen C-Y, Chang J-S. 2021. Algae-derived hydrocolloids in foods: applications and health-related issues. Bioengineered. 12(1):3787–3801. doi: 10.1080/21655979.2021.1946359.
- Limketkai BN, Iheozor-Ejiofor Z, Gjuladin-Hellon T, Parian A, Matarese LE, Bracewell K, MacDonald JK, Gordon M, Mullin GE. 2019. Dietary interventions for induction and maintenance of remission in inflammatory bowel disease. Cochrane Database Syst Rev. 2(2): CD012839. doi: 10.1002/14651858.CD012839.pub2.
- Ling K-Y, Bhalla D, Hollander D. 1988. Mechanisms of carrageenan injury of IEC18 small intestinal epithelial cell monolayers. Gastroenterology. 95(6):1487–1495. doi: 10.1016/s0016-5085(88)80067-2.
- Liu C, Zhan S, Tian Z, Li N, Li T, Wu D, Zeng Z, Zhuang X. 2022. Food additives associated with gut microbiota alterations in inflammatory bowel disease: friends or enemies? Nutrients. 14(15):3049. doi: 10.3390/nu14153049.
- Liu F, Hou P, Zhang H, Tang Q, Xue C, Li RW. 2021. Food-grade carrageenans and their implications in health and disease. Compr Rev Food Sci Food Saf. 20(4):3918–3936. doi: 10.1111/1541-4337.12790.
- Liu J, Zhan X, Wan J, Wang Y, Wang C. 2015. Review for carrageenan-based pharmaceutical biomaterials: favourable physical features versus adverse biological effects. Carbohydr Polym. 121:27–36. doi: 10.1016/j.carbpol.2014.11.063.
- Lock JY, Carlson TL, Wang C-M, Chen A, Carrier RL. 2018. Acute exposure to commonly ingested emulsifiers alters intestinal mucus structure and transport properties. Sci Rep. 8(1):10008. doi: 10.1038/s41598-018-27957-2.
- Logan M, Gkikas K, Svolos V, Nichols B, Milling S, Gaya DR, Seenan JP, Macdonald J, Hansen R, Ijaz UZ, et al. 2020. Analysis of 61 exclusive enteral nutrition formulas used in the management of active Crohn’s disease – new insights into dietary disease triggers. Aliment Pharmacol Ther. 51(10):935–947. doi: 10.1111/apt.15695.
- MacPherson B, Pfeiffer CJ. 1976. Experimental colitis. Digestion. 14(5-6):424–452. doi: 10.1159/000197966.
- Madhère SM, Reichner J, Finkelstein S, Pricolo VE. 1994. Histologic and immunologic changes in lambda-carrageenan induced inflammatory bowel disease. Gastroenterology. 106(4):A1036.
- Mallett AK, Rowland IR, Bearne CA, Nicklin S. 1985. Influence of dietary carrageenans on microbial biotransformation activities in the cecum of rodents and on gastrointestinal immune status in the rat. Toxicol Appl Pharmacol. 78(3):377–385. doi: 10.1016/0041-008x(85)90243-1.
- Mankes R, Abraham R. 1975. Lysosomal dysfunction in colonic submucosal macrophages of Rhesus monkeys caused by degraded iota carrageenan. Proc Soc Exp Biol Med. 150(1):166–170. doi: 10.3181/00379727-150-38996.
- Marburger A. 2003. Alginate und Carrageenane - Eigenschaften, Gewinnung und Anwendungen in Schule und Hochschule. [Alginates and carrageenans – properties, extraction and applications in schools and universities]. [dissertation]. German: Philipps-Universität Marburg. https://archiv.ub.uni-marburg.de/ubfind/Record/urn:nbn:de:hebis:04-z2004-0110/Description#tabnav.
- Marcus AJ, Marcus SN, Marcus R, Watt J. 1989. Rapid production of ulcerative disease of the colon in newly-weaned guinea-pigs by degraded carrageenan. J Pharm Pharmacol. 41(6):423–426. doi: 10.1111/j.2042-7158.1989.tb06493.x.
- Marcus R, Watt J. 1969. Seaweeds and ulcerative colitis in laboratory animals. Lancet. 294(7618):489–490. doi: 10.1016/s0140-6736(69)90187-1.
- Marcus R, Watt J. 1970. Carrageenan and the human gut. Lancet. 296(7675):726. doi: 10.1016/S0140-6736(70)92000-3.
- Marcus SN, Marcus AJ, Marcus R, Ewen SWB, Watt J. 1992. The pre-ulcerative phase of carrageenan-induced colonic ulceration in the guinea-pig. Int J Exp Path. 73(4):515–526. https://pubmed.ncbi.nlm.nih.gov/1356411/.
- Marion-Letellier R, Amamou A, Savoye G, Ghosh S. 2019. Inflammatory bowel diseases and food additives: to add fuel on the flames!. Nutrients. 11(5):1111. doi: 10.3390/nu11051111.
- Martino JV, Van Limbergen J, Cahill LE. 2017. The role of carrageenan and carboxymethylcellulose in the development of intestinal inflammation. Front Pediatr. 5:96. doi: 10.3389/fped.2017.00096.
- Matsumoto T, Kitano A, Oshitani N, Obata A, Hiki M, Hashimura H, Okawa K, Nagura H, Kobayashi K. 1988. Immunoglobulin-containing cells in the colonic mucosa of rabbits with carrageenan-induced colitis. Dis Colon Rectum. 31(9):723–729. doi: 10.1007/bf02552594.
- McElligott TF, Hurst EW. 1968. Long-term feeding studies of methyl ethyl cellulose ('Edifas’ A) and sodium carboxymethyl cellulose ('Edifas’ B) in rats and mice. Food Cosmet Toxicol. 6(4):449–460. doi: 10.1016/0015-6264(68)90135-1.
- McKim JM. 2014. Food additive carrageenan: part I: a critical review of carrageenan in vitro studies, potential pitfalls, and implications for human health and safety. Crit Rev Toxicol. 44(3):211–243. doi: 10.3109/10408444.2013.861797.
- McKim JM Jr, Baas H, Rice GP, Willoughby JA Sr, Weiner ML, Blakemore W. 2016. Effects of carrageenan on cell permeability, cytotoxicity, and cytokine gene expression in human intestinal and hepatic cell lines. Food Chem Toxicol. 96:1–10. doi: 10.1016/j.fct.2016.07.006.
- McKim JM Jr, Wilga PC, Pregenzer JF, Blakemore WR. 2015. The common food additive carrageenan is not a ligand for Toll-Like-Receptor 4 (TLR4) in an HEK293-TLR4 reporter cell-line model. Food Chem Toxicol. 78:153–158. doi: 10.1016/j.fct.2015.01.003.
- McKim JM, Willoughby JA Sr, Blakemore WR, Weiner ML. 2019. Clarifying the confusion between poligeenan, degraded carrageenan, and carrageenan: a review of the chemistry, nomenclature, and in vivo toxicology by the oral route. Crit Rev Food Sci Nutr. 59(19):3054–3073. doi: 10.1080/10408398.2018.1481822.
- Metzler-Zebeli BU, Hooda S, Pieper R, Zijlstra RT, van Kessel AG, Mosenthin R, Gänzle MG. 2010. Nonstarch polysaccharides modulate bacterial microbiota, pathways for butyrate production, and abundance of pathogenic Escherichia coli in the pig gastrointestinal tract. Appl Environ Microbiol. 76(11):3692–3701. doi: 10.1128/AEM.00257-10.
- Mi Y, Chin YX, Cao WX, Chang YG, Lim PE, Xue CH, Tang QJ. 2020. Native κ-carrageenan induced-colitis is related to host intestinal microecology. Int J Biol Macromol. 147:284–294. doi: 10.1016/j.ijbiomac.2020.01.072.
- Michel C, Macfarlane GT. 1996. Digestive fates of soluble polysaccharides from marine macroalgae: involvement of the colonic microflora and physiological consequences for the host. J Appl Bacteriol. 80(4):349–369. doi: 10.1111/j.1365-2672.1996.tb03230.x.
- Miclotte L, De Paepe K, Rymenans L, Callewaert C, Raes J, Rajkovic A, Van Camp J, Van de Wiele T. 2020. Dietary emulsifiers alter composition and activity of the human gut microbiota in vitro, irrespective of chemical or natural emulsifier origin. Front Microbiol. 11:577474. doi: 10.3389/fmicb.2020.577474.
- Milard M, Laugerette F, Bugeat S, Plaisancié P, Létisse M, Meugnier E, Loizon E, Durand A, Buisson C, Géloën A, et al. 2018. Metabolic effects in mice of cream formulation: addition of both thickener and emulsifier does not alter lipid metabolism but modulates mucus cells and intestinal endoplasmic reticulum stress. J Dairy Sci. 101(12):10649–10663. doi: 10.3168/jds.2018-14783.
- Mottet NK. 1972. On animal models for inflammatory bowel disease. Gastroenterology. 62(6):1269–1271. doi: 10.1016/S0016-5085(72)80095-7.
- Moyana T, Lalonde J-MA. 1991. Carrageenan-induced intestinal injury: possible role of oxygen free radicals. Ann Clin Lab Sci. 21(4):258–263. https://pubmed.ncbi.nlm.nih.gov/1859164/.
- Moyana TN, Lalonde J-MA. 1990. Carrageenan-induced intestinal injury in the rat - a model for inflammatory bowel disease. Ann Clin Lab Sci. 20(6):420–426. https://pubmed.ncbi.nlm.nih.gov/2073092/.
- Moyana TN, Xiang J, Qi Y, Kalra J. 1994. Development of the early mucosal lesions in experimental inflammatory bowel disease - implications for pathogenesis. Exp Mol Pathol. 60(2):119–129. doi: 10.1006/exmp.1994.1011.
- Munn Z, Peters MDJ, Stern C, Tufanaru C, McArthur A, Aromataris E. 2018. Systematic review or scoping review? Guidance for authors when choosing between a systematic or scoping review approach. BMC Med Res Methodol. 18(1):143. doi: 10.1186/s12874-018-0611-x.
- Munyaka PM, Sepehri S, Ghia J-E, Khafipour E. 2016. Carrageenan gum and adherent invasive Escherichia coli in a piglet model of inflammatory bowel disease: impact on intestinal mucosa-associated microbiota. Front Microbiol. 7:462. doi: 10.3389/fmicb.2016.00462.
- Nabarawi NA. 2014. Effect of atorvastatin (HMG-CoA reductase inhibitor) on experimentally induced colitis in mice. Int J Pharm Pharm Sci. 6(1):649–653. https://innovareacademics.in/journal/ijpps/Vol6Issue1/8308.pdf.
- Naimi S, Viennois E, Gewirtz AT, Chassaing B. 2021. Direct impact of commonly used dietary emulsifiers on human gut microbiota. Microbiome. 9(1):66. doi: 10.1186/s40168-020-00996-6.
- Neurath MF. 2012. Animal models of inflammatory bowel diseases: illuminating the pathogenesis of colitis, ileitis and cancer. Dig Dis. 30(Suppl 1):91–94. doi: 10.1159/000341131.
- Nicklin S, Miller K. 1989. Intestinal uptake and immunological effects of carrageenan - current concepts. Food Addit Contam. 6(4):425–436. doi: 10.1080/02652038909373801.
- Nie Q, Hu J, Gao H, Li M, Sun Y, Chen H, Zuo S, Fang Q, Huang X, Yin J, et al. 2021. Bioactive dietary fibers selectively promote gut microbiota to exert antidiabetic effects. J Agric Food Chem. 69(25):7000–7015. doi: 10.1021/acs.jafc.1c01465.
- Niewiadomski O. 2018. Update on the epidemiology of Australian inflammatory bowel disease from the Geelong cohort: does diet matter after all? J Gastroenterol Hepatol. 33(Suppl 3):20–21. doi: 10.1111/jgh.14426.
- Norris AA, Lewis AJ, Zeitlin IJ. 1981. Inability of degraded carrageenan fractions to induce inflammatory bowel ulceration in the guinea-pig. J Pharm Pharmacol. 33(1):612–613. doi: 10.1111/j.2042-7158.1981.tb13881.x.
- Ohnishi A, Hashimoto K, Ozono E, Sasaki M, Sakamoto A, Tashiro K, Moriuchi H. 2019. Anaphylaxis to carboxymethylcellulose: add food additives to the list of elicitors. Pediatrics. 143(3):e20181180. doi: 10.1542/peds.2018-1180.
- Olsen PS, Poulsen SS. 1980. Stereomicroscopic and histologic changes in the colon of guinea pigs fed degraded carrageenan. Acta Pathol Microbiol Scand A. 88A(1-6):135–141. doi: 10.1111/j.1699-0463.1980.tb02478.x.
- Onderdonk AB. 1985a. Experimental models for ulcerative colitis. Dig Dis Sci. 30(12 Suppl):40S–44S. doi: 10.1007/BF01296973.
- Onderdonk AB. 1985b. The carrageenan model for experimental ulcerative colitis. Prog Clin Biol Res. 186:237–245. https://pubmed.ncbi.nlm.nih.gov/4034603/.
- Onderdonk AB, Franklin ML, Cisneros RL. 1981. Production of experimental ulcerative colitis in gnotobiotic guinea pigs with simplified microflora. Infect Immun. 32(1):225–231. doi: 10.1128/iai.32.1.225-231.1981.
- Onderdonk AB, Hermos JA, Bartlett JG. 1977. The role of the intestinal microflora in experimental colitis. Am J Clin Nutr. 30(11):1819–1825. doi: 10.1093/ajcn/30.11.1819.
- Onderdonk AB, Steeves RM, Cisneros RL, Bronson RT. 1984. Adoptive transfer of immune enhancement of experimental ulcerative colitis. Infect Immun. 46(1):64–67. doi: 10.1128/iai.46.1.64-67.1984.
- Onishchenko A, Gubina-Vakulyck G, Knigavko O, Sharashydze K, Pionova O, Butov D, Polikarpova H, Tkachenko A. 2022. Intake of semirefined carrageenan causes low-grade colonic inflammation and alters expression of epithelial-mesenchymal transition markers. Malays J Biochem Mol Biol. 1:8–17. http://repo.knmu.edu.ua/handle/123456789/31197.
- Oscarsson E, Lindberg T, Zeller KS, Lindstedt M, Agardh D, Håkansson Å, Östbring K. 2020. Changes in intestinal permeability ex vivo and immune cell activation by three commonly used emulsifiers. Molecules. 25(24):5943. doi: 10.3390/molecules25245943.
- Page MJ, McKenzie JE, Bossuyt PM, Boutron I, Hoffmann TC, Mulrow CD, Shamseer L, Tetzlaff JM, Akl EA, Brennan SE, et al. 2021a. The PRISMA 2020 statement: an updated guideline for reporting systematic reviews. BMJ. 372:n71. doi: 10.1136/bmj.n71.
- Page MJ, Moher D, Bossuyt PM, Boutron I, Hoffmann TC, Mulrow CD, Shamseer L, Tetzlaff JM, Akl EA, Brennan SE, et al. 2021b. PRISMA 2020 explanation and elaboration: updated guidance and exemplars for reporting systematic reviews. BMJ. 372:n160. doi: 10.1136/bmj.n160.
- Park S, Kang Y, Koh H, Kim S. 2020. Increasing incidence of inflammatory bowel disease in children and adolescents: significance of environmental factors. Clin Exp Pediatr. 63(9):337–344. doi: 10.3345/cep.2019.00500.
- Partridge D, Lloyd KA, Rhodes JM, Walker AW, Johnstone AM, Campbell BJ. 2019. Food additives: assessing the impact of exposure to permitted emulsifiers on bowel and metabolic health – introducing the FADiets study. Nutr Bull. 44(4):329–349. doi: 10.1111/nbu.12408.
- Paula Neto HA, Ausina P, Gomez LS, Leandro JGB, Zancan P, Sola-Penna M. 2017. Effects of food additives on immune cells as contributors to body weight gain and immune-mediated metabolic dysregulation. Front Immunol. 8:1478. doi: 10.3389/fimmu.2017.01478.
- Peters MDJ, Marnie C, Colquhoun H, Garritty CM, Hempel S, Horsley T, Langlois EV, Lillie E, O’Brien KK, Tunçalp Ӧ, et al. 2021a. Scoping reviews: reinforcing and advancing the methodology and application. Syst Rev. 10(1):263. doi: 10.1186/s13643-021-01821-3.
- Peters MDJ, Marnie C, Tricco AC, Pollock D, Munn Z, Alexander L, McInerney P, Godfrey CM, Khalil H. 2021b. Updated methodological guidance for the conduct of scoping reviews. JBI Evid Implement. 19(1):3–10. doi: 10.1097/XEB.0000000000000277.
- Pintauro SJ, Gilbert SW. 1990. The effects of carrageenan on drug-metabolizing enzyme system activities in the guinea-pig. Food Chem Toxicol. 28(12):807–811. doi: 10.1016/0278-6915(90)90053-P.
- Pogozhykh D, Posokhov Y, Myasoedov V, Gubina-Vakulyck G, Chumachenko T, Knigavko O, Polikarpova H, Kalashnyk-Vakulenko Y, Sharashydze K, Nakonechna O, et al. 2021. Experimental evaluation of food-grade semi-refined carrageenan toxicity. Int J Mol Sci. 22(20):11178. doi: 10.3390/ijms222011178.
- Pollock D, Tricco AC, Peters MDJ, Mclnerney PA, Khalil H, Godfrey CM, Alexander LA, Munn Z. 2022. Methodological quality, guidance, and tools in scoping reviews: a scoping review protocol. JBI Evid Synth. 20(4):1098–1105. doi: 10.11124/JBIES-20-00570.
- Poulsen E. 1973. Short-term peroral toxicity of undegraded carrageenan in pigs. Food Cosmet Toxicol. 11(2):219–227. doi: 10.1016/S0015-6264(73)80488-2.
- Pricolo VE, Madhere SM, Finkelstein SD, Reichner JS. 1996. Effects of lambda-carrageenan induced experimental enterocolitis on splenocyte function and nitric oxide production. J Surg Res. 66(1):6–11. doi: 10.1006/jsre.1996.0364.
- Regulation (EC) No 1333/2008. 2008a. EU database of food additives, based on the Union list of food additives in Annex II of Regulation (EC) No 1333/2008. [accessed 2023 May 25]. https://ec.europa.eu/food/food-feed-portal/screen/food-additives/search.
- Regulation (EC) No 1333/2008. 2008b. Regulation (EC) No 1333/2008 of the European Parliament and of the Council of 16 December 2008 on food additives. OJ L 354, 31.12.2008, p. 16–33. [accessed 2023 May 25]. https://eur-lex.europa.eu/legal-content/EN/TXT/?uri=celex%3A32008R1333.
- Reveley H, Frank L, Javadpour A, Samocha-Bonet D, Hold G, Lambert K. 2022. Emulsifier intake in a cohort of Australians with inflammatory bowel disease. J Gastroenterol Hepatol. 37(Suppl. 1):219. Abstract no. 169. doi: 10.1111/jgh.15957?af=R.
- Rinninella E, Cintoni M, Raoul P, Gasbarrini A, Mele MC. 2020. Food additives, gut microbiota, and irritable bowel syndrome: a hidden track. Int J Environ Res Public Health. 17(23):8816. doi: 10.3390/ijerph17238816.
- Rousta E, Oka A, Liu B, Herzog J, Bhatt AP, Wang J, Habibi Najafi MB, Sartor RB. 2021. The emulsifier carboxymethylcellulose induces more aggressive colitis in humanized mice with inflammatory bowel disease microbiota than polysorbate-80. Nutrients. 13(10):3565. doi: 10.3390/nu13103565.
- Rowland IR, Mallett AK, Wise A, Bailey E. 1983. Effect of dietary carrageenan and pectin on the reduction of nitro-compounds by the rat caecal microflora. Xenobiotica. 13(4):251–256. doi: 10.3109/00498258309052261.
- Rustia M, Shubik P, Patil K. 1980. Lifespan carcinogenicity tests with native carrageenan in rats and hamsters. Cancer Lett. 11(1):1–10. doi: 10.1016/0304-3835(80)90122-6.
- Sabljic N, Dahleh S, Bird RP. 2002. Risk of developing abberant crypt foci in the carrageenan model of inflammatory bowel disease: an in vivo study on the effect of dietary lipids on colonic HMG-CoA reductase protein expression. Cancer Epidemiol Biomarkers Prev. 11 (Suppl 10):1190S–1191S.
- Sandall AM, Cox SR, Lindsay JO, Gewirtz AT, Chassaing B, Rossi M, Whelan K. 2020. Emulsifiers impact colonic length in mice and emulsifier restriction is feasible in people with Crohn’s disease. Nutrients. 12(9):2827. doi: 10.3390/nu12092827.
- Sasson AN, Ananthakrishnan AN, Raman M. 2021. Diet in treatment of inflammatory bowel diseases. Clin Gastroenterol Hepatol. 19(3):425–435. doi: 10.1016/j.cgh.2019.11.054.
- Schooth LF, Loayza JJJ, Teh JJ, Zhang J, Zhang F, Liu Q, Hamilton AL, Wilson-O'Brien A, Trakman GL, Lin WY, et al. 2020. Crohn’s disease pathobiont Enterobacteriaceae growth is modulated by food additives and oxygen availability: factors in pathophysiology and recurrence. The Enigma Study. Gastroenterology. 158(6)(Suppl 1):S-2. Abstract no. 4. doi: 10.1016/s0016-5085(20)30685-5.
- Shah R, Kolanos R, DiNovi MJ, Mattia A, Kaneko KJ. 2017. Dietary exposures for the safety assessment of seven emulsifiers commonly added to foods in the United States and implications for safety. Food Addit Contam Part A Chem Anal Control Expo Risk Assess. 34(6):905–917. doi: 10.1080/19440049.2017.1311420.
- Shang Q, Jiang H, Cai C, Hao J, Li G, Yu G. 2018. Gut microbiota fermentation of marine polysaccharides and its effects on intestinal ecology: an overview. Carbohydr Polym. 179:173–185. doi: 10.1016/j.carbpol.2017.09.059.
- Shang Q, Sun W, Shan X, Jiang H, Cai C, Hao J, Li G, Yu G. 2017. Carrageenan-induced colitis is associated with decreased population of anti-inflammatory bacterium, Akkermansia muciniphila, in the gut microbiota of C57BL/6J mice. Toxicol Lett. 279:87–95. doi: 10.1016/j.toxlet.2017.07.904.
- Shiau S-Y, Chang GW. 1984. Degraded carrageenan increases permeability of phenol red in the rat intestine. Fed Proc. 43(4):1065. Abstract no. 4562. http://www.shiau.ntou.edu.tw/e4.htm#Conference_paper_(International_conference_only).
- Shiau S-Y, Chang GW. 1986. Effects of certain dietary fibers on apparent permeability of the rat intestine. J Nutr. 116(2):223–232. doi: 10.1093/jn/116.2.223.
- Strober W. 1985. Animal models of inflammatory bowel disease—an overview. Dig Dis Sci. 30(12 Suppl):3S–10S. doi: 10.1007/BF01296964.
- Sun Y, Cui X, Duan M, Ai C, Song S, Chen X. 2019. In vitro fermentation of κ-carrageenan oligosaccharides by human gut microbiota and its inflammatory effect on HT29 cells. J Funct Foods. 59:80–91. doi: 10.1016/j.jff.2019.05.036.
- Swidsinski A, Ung V, Sydora BC, Loening-Baucke V, Doerffel Y, Verstraelen H, Fedorak RN. 2009. Bacterial overgrowth and inflammation of small intestine after carboxymethylcellulose ingestion in genetically susceptible mice. Inflamm Bowel Dis. 15(3):359–364. doi: 10.1002/ibd.20763.
- Taché S, Peiffer G, Millet A-S, Corpet DE. 2000. Carrageenan gel and aberrant crypt foci in the colon of conventional and human flora-associated rats. Nutr Cancer. 37(2):193–198. doi: 10.1207/S15327914NC372_12.
- Tan H, Nie S. 2021. Functional hydrocolloids, gut microbiota and health: picking food additives for personalized nutrition. FEMS Microbiol Rev. 45(4):1–18. doi: 10.1093/femsre/fuaa065.
- Tkachenko A, Marakushyn D, Kalashnyk I, Korniyenko Y, Onishchenko A, Gorbach T, Nakonechna O, Posokhov Y, Tsygankov A. 2018a. A study of enterocyte membranes during activation of apoptotic processes in chronic carrageenan-induced gastroenterocolitis. Med Glas (Zenica). 15(2):87–92. https://repo.knmu.edu.ua/handle/123456789/21050.
- Tkachenko A, Myasoedov V, Gubina-Vakulyck G, Onishchenko A, Tkachenko V, Nakonechna O. 2021. Assessment of a common food additive E407a toxicity. FEBS Open Bio. 11(Suppl 1):85. Abstract no. SpT-03-04. doi: 10.1002/2211-5463.13206.
- Tkachenko AS, Gubina-Vakulyck GI, Klochkov VK, Kavok NS, Onishchenko AI, Gorbach TV, Nakonechna OA. 2020. Experimental evaluation of the impact of gadolinium orthovanadate GdVO4:Eu3+ nanoparticles on the carrageenan-induced intestinal inflammation. Acta Medica (Hradec Králové). 63(1):18–24. doi: 10.14712/18059694.2020.11.
- Tkachenko AS, Onishchenko AI, Gorbach TV, Gubina-Vakulyсk GI. 2018b. O-6-methylguanine-DNA methyltransferase (MGMT) overexpression in small intestinal mucosa in experimental carrageenan-induced enteritis. Malays J Biochem Mol Biol. 21(3):77–80. https://elibrary.ru/item.asp?id=41666737.
- Tobacman JK. 2001. Review of harmful gastrointestinal effects of carrageenan in animal experiments. Environ Health Perspect. 109(10):983–994. doi: 10.1289/ehp.01109983.
- Tobacman JK. 2002a. Carrageenan in foods: response. Environ Health Perspect. 110(4):A176–A177. doi: 10.1289/ehp.110-a176b.
- Tobacman JK. 2002b. Carrageenan: response. Environ Health Perspect. 110(6):A288. doi: 10.1289/ehp.110-a288b.
- Tobacman JK. 2003. Toxic considerations related to ingestion of carrageenan. In Preedy WR and Watson RR eds. Reviews in Food and Nutrition Toxicity, 1st ed., 26 pages. Taylor & Francis, CRC Press, London and New York. doi: 10.1201/9780203634523-13/toxic-considerations-related-ingestion-carrageenan-joanne-tobacman.
- Toyoda K, Nishikawa A, Furukawa F, Kawanishi T, Hayashi Y, Takahashi M. 1994. Cell proliferation induced by laxatives and related compounds in the rat intestine. Cancer Lett. 83(1-2):43–49. doi: 10.1016/0304-3835(94)90297-6.
- Tricco AC, Lillie E, Zarin W, O'Brien KK, Colquhoun H, Levac D, Moher D, Peters MDJ, Horsley T, Weeks L, et al. 2018. PRISMA extension for scoping reviews (PRISMA-ScR): checklist and explanation. Ann Intern Med. 169(7):467–473. doi: 10.7326/M18-0850.
- Ung V, Swidsinski A, Foshaug R, Doyle J, Sydora B, Fedorak R. 2009. Carboxymethyl cellulose ingestion leads to bacterial overgrowth and inflammation in the small intestine of IL-10 gene-deficient mice. Can J Gastroenterol. 23(Suppl A):64A–65A. Abstract no13.
- Ung VYL, Foshaug RR, MacFarlane SM, Churchill TA, Doyle JSG, Sydora BC, Fedorak RN. 2010. Oral administration of curcumin emulsified in carboxymethyl cellulose has a potent anti-inflammatory effect in the IL-10 gene-deficient mouse model of IBD. Dig Dis Sci. 55(5):1272–1277. doi: 10.1007/s10620-009-0843-z.
- [US FDA] United States Food and Drug Administration (USA). 2023a. CFR – Code of Federal Regulations, Title 21, Chapter I, Part 172, Sec. 172.620 Carrageenan and Sec. 172.626 Salts of carrageenan. [accessed 2023 May 25]. https://www.ecfr.gov/.
- [US FDA] United States Food and Drug Administration (USA). 2023b. CFR – Code of Federal Regulations, Title 21, Chapter I, Part 182, Sec. 182.1745 and Part 582, Sec. 582.1745 Sodium carboxymethylcellulose. [accessed 2023 May 25]. https://www.ecfr.gov/.
- Valizadeh H, Fahimfar H, Ghanbarzadeh S, Islambulchilar Z, Zakeri-Milani P. 2015. Effect of anionic macromolecules on intestinal permeability of furosemide. Drug Dev Ind Pharm. 41(2):190–193. doi: 10.3109/03639045.2013.851210.
- van der Waaij D, Cohen BJ, Anver MR. 1974. Mitigation of experimental inflammatory bowel disease in guinea pigs by selective elimination of the aerobic Gram-negative intestinal microflora. Gastroenterology. 67(3):460–472. doi: 10.1016/S0016-5085(19)32848-3.
- Viennois E, Bretin A, Dubé PE, Maue AC, Dauriat CJG, Barnich N, Gewirtz AT, Chassaing B. 2020. Dietary emulsifiers directly impact adherent-invasive E. coli gene expression to drive chronic intestinal inflammation. Cell Rep. 33(1):108229. doi: 10.1016/j.celrep.2020.108229.
- Viennois E, Chassaing B. 2018. First victim, later aggressor: how the intestinal microbiota drives the pro-inflammatory effects of dietary emulsifiers? Gut Microbes. 9(3):288–291. doi: 10.1080/19490976.2017.1421885.
- Viennois E, Chassaing B. 2021. Consumption of select dietary emulsifiers exacerbates the development of spontaneous intestinal adenoma. Int J Mol Sci. 22(5):2602. doi: 10.3390/ijms22052602.
- Viennois E, Merlin D, Gewirtz AT, Chassaing B. 2017. Dietary emulsifier-induced low-grade inflammation promotes colon carcinogenesis. Cancer Res. 77(1):27–40. doi: 10.1158/0008-5472.can-16-1359.
- Vo TD, Lynch BS, Roberts A. 2019. Dietary exposures to common emulsifiers and their impact on the gut microbiota: is there a cause for concern? Compr Rev Food Sci Food Saf. 18(1):31–47. doi: 10.1111/1541-4337.12410.
- Wagner R, Buettner J, Heni M, Fritsche L, Kullmann S, Wagmüller M, Peter A, Machann J, Pape U-F, Van Hall G, et al. 2018a. The common food additive carrageenan increases intestinal permeability without affecting whole-body insulin sensitivity in humans. Diabetologia. 61(Suppl 1):S333. Abstract no. 686. doi: 10.1007/s00125-018-4693-0.
- Wagner R, Büttner J, Heni M, Fritsche L, Kullmann S, Wagmüller M, Peter A, Preissl H, Machann J, Pape U-F, et al. 2018b. The common food additive carrageenan increases intestinal permeability without affecting whole-body insulin sensitivity in humans – Results from a randomized, double-blind crossover study. Diabetes. 67(Supplement_1):A78–A79. doi: 10.2337/db18-295-OR.
- Wang Q, Zhang L, He Y, Zeng L, He J, Yang Y, Zhang T. 2021. Effect of κ-carrageenan on glucolipid metabolism and gut microbiota in high-fat diet-fed mice. J Funct Foods. 86:104707. doi: 10.1016/j.jff.2021.104707.
- Wang X, Fang Y, Liang W, Liang M, Xu L, Yu J. 2022. Dietary additive carrageenan metabolized by human gut microbiota and promoting colorectal cancer. Gut. 71(Suppl 2):A66. Abstract no. IDDF2022-ABS-0253. doi: 10.1136/gutjnl-2022-IDDF.79.
- Washington K, Zemper AED. 2019. Apc-related models of intestinal neoplasia: a brief review for pathologists. Surg Exp Pathol. 2:11. doi: 10.1186/s42047-019-0036-9.
- Watanabe K, Reddy BS, Wong CQ, Weisburger JH. 1978. Effect of dietary undegraded carrageenan on colon carcinogenesis in F344 rats treated with azoxymethane or methylnitrosourea. Cancer Res. 38(12):4427–4430. https://pubmed.ncbi.nlm.nih.gov/719628/.
- Watt J, Marcus R. 1969. Ulcerative colitis in the guinea‐pig caused by seaweed extract. J Pharm Pharmacol. 21(Supplement_1):187S–188S. doi: 10.1111/j.2042-7158.1969.tb08372.x.
- Watt J, Marcus R. 1971. Carrageenan-induced ulceration of the large intestine in the guinea pig. Gut. 12(2):164–171. doi: 10.1136/gut.12.2.164.
- Watt J, Marcus R. 1973. Experimental ulcerative disease of the colon in animals. Gut. 14(6):506–510. doi: 10.1136/gut.14.6.506.
- Watt J, Marcus R. 1975. Experimental ulcerative disease of the colon. Meth Achiev Exp Pathol. 7:56–71. https://pubmed.ncbi.nlm.nih.gov/1202321/.
- Watt J, Marcus R. 1981. Harmful effects of carrageenan fed to animals. Cancer Detect Prev. 4(1-4):129–134. https://pubmed.ncbi.nlm.nih.gov/7349769/.
- Weiner ML. 1991. Toxicological properties of carrageenan. Agents Actions. 32(1-2):46–51. doi: 10.1007/BF01983307.
- Weiner ML. 2014. Food additive carrageenan: part II: a critical review of carrageenan in vivo safety studies. Crit Rev Toxicol. 44(3):244–269. doi: 10.3109/10408444.2013.861798.
- Weiner ML, McKim JM Jr. 2019. Comment on “Revisiting the carrageenan controversy: do we really understand the digestive fate and safety of carrageenan in our foods? by S. David, C. S. Levi, L. Fahoum, Y. Ungar, E. G. Meyron-Holtz, A. Shpigelman and U. Lesmes, Food Funct., 2018, 9, 1344–1352. Food Funct. 10(3):1760–1762. doi: 10.1039/C8FO01282B.
- Wellens J, Vermeire S, Sabino J. 2022. The role of carboxymethylcellulose in health and disease: is the plot thickening? Gastroenterology. 163(3):780–781. doi: 10.1053/j.gastro.2022.01.007.
- Wells ML, Potin P, Craigie JS, Raven JA, Merchant SS, Helliwell KE, Smith AG, Camire ME, Brawley SH. 2017. Algae as nutritional and functional food sources: revisiting our understanding. J Appl Phycol. 29(2):949–982. doi: 10.1007/s10811-016-0974-5.
- Whittaker JS, Freeman HJ. 1988. Fibre and carrageenan in inflammatory bowel disease. Can J Gastroenterol. 2(Suppl A):39A–45A.
- Wirtz S, Neurath MF. 2000. Animal models of intestinal inflammation: new insights into the molecular pathogenesis and immunotherapy of inflammatory bowel disease. Int J Colorectal Dis. 15(3):144–160. doi: 10.1007/s003840000227.
- Wu B, Bhatnagar R, Indukuri VV, Chopra S, March K, Cordero N, Chopra S, Reddivari L. 2020. Intestinal mucosal barrier function restoration in mice by maize diet containing enriched flavan-4-ols. Nutrients. 12(4):896. doi: 10.3390/nu12040896.
- Wu W, Wang F, Gao X, Niu T, Zhen Z, Chen H, Yan X. 2016a. Enhanced effect of κ-carrageenan on TNBS-induced inflammation in mice. Int Immunopharmacol. 39:218–228. doi: 10.1016/j.intimp.2016.07.031.
- Wu W, Wang F, Gao X, Niu T, Zhu X, Yan X, Chen H. 2016b. Synergistic effect of κ-carrageenan on oxazolone-induced inflammation in BALB/c mice. BMC Gastroenterol. 16(1):41. doi: 10.1186/s12876-016-0459-7.
- Wu W, Zhen Z, Niu T, Zhu X, Gao Y, Yan J, Chen Y, Yan X, Chen H. 2017. κ-Carrageenan enhances lipopolysaccharide-induced interleukin-8 secretion by stimulating the Bcl10-NF-κB pathway in HT-29 cells and aggravates C. freundii-induced inflammation in mice. Mediators Inflamm. 2017:8634865. doi: 10.1155/2017/8634865.
- Wu W, Zhou D, Xuan R, Zhou J, Liu J, Chen J, Han H, Niu T, Li X, Chen H, et al. 2021. λ-carrageenan exacerbates Citrobacter rodentium-induced infectious colitis in mice by targeting gut microbiota and intestinal barrier integrity. Pharmacol Res. 174:105940. doi: 10.1016/j.phrs.2021.105940.
- Wu W, Zhou J, Xuan R, Chen J, Han H, Liu J, Niu T, Chen H, Wang F. 2022. Dietary κ-carrageenan facilitates gut microbiota-mediated intestinal inflammation. Carbohydr Polym. 277:118830. doi: 10.1016/j.carbpol.2021.118830.
- Wyatt GM, Horn N, Gee JM, Johnson IT. 1988. Intestinal microflora and gastrointestinal adaptation in the rat in response to non-digestible dietary polysaccharides. Br J Nutr. 60(2):197–207. doi: 10.1079/BJN19880091.
- Xiong W-J, Qiu Q-Y, Qiu D-K. 2005. Protective effect of Jiechangning decoction in treating experimental ulcerative colitis in guinea pigs. Chin J Integr Med. 11(1):49–53. doi: 10.1007/bf02835750.
- Xu D, Wu X, Gillilland M, Zheng W, Hu X, Martens E, Owyang C. 2019. Altered gut microbiota induced by a dietary emulsifier degrades the colonic mucus barrier and promotes visceral hypersensitivity. Gastroenterology. 156(6 Suppl 1):S-685. Abstract no. Su1996. doi: 10.1016/s0016-5085(19)38630-5.
- Xu D, Zhang Y, Wu J, Hill D, Ausland C, Yin Y, Martens E, Owyang C. 2020. Role of dietary and non-digestible carbohydrates in gut microecosystem and intestinal mucus degradation. Gastroenterology. 158(6 Suppl 1):S-1216. Abstract no. Tu1913. doi: 10.1016/S0016-5085(20)33700-8.
- Yacyshyn MB, Case JW, Jin H, Yacyshyn BR. 2011. Evaluation of goblet cell populations in HLA B27 rats before and after treatment with the soluble fiber carboxymethylcellulose. Gastroenterology. 140(5 Suppl 1):S-850. Abstract no. Tu1858. doi: 10.1016/s0016-5085(11)63527-0.
- Yang B, Bhattacharyya S, Linhardt R, Tobacman J. 2012. Exposure to common food additive carrageenan leads to reduced sulfatase activity and increase in sulfated glycosaminoglycans in human epithelial cells. Biochimie. 94(6):1309–1316. doi: 10.1016/j.biochi.2012.02.031.
- Yin Y, Li M, Gu W, Zeng B, Liu W, Zhu L, Pi X, Primerano DA, Yu HD, Wei H, et al. 2021. Carrageenan oligosaccharides and associated carrageenan-degrading bacteria induce intestinal inflammation in germ-free mice. J Genet Genomics. 48(9):815–824. doi: 10.1016/j.jgg.2021.08.001.
- Yoshida Y, Murata Y. 1990. Inflammatory bowel disease in Japan: studies of epidemiology and etiopathogenesis. Med Clin North Am. 74(1):67–90. doi: 10.1016/S0025-7125(16)30587-9.
- Zangara M, Sangwan N, McDonald C. 2021. Common food additives accelerate onset of inflammatory bowel disease in mice by altering microbiome composition and host-microbe interaction. Inflammatory Bowel Dis. 27(Supplement_1):S39. doi: 10.1093/ibd/izaa347.096.
- Zhang H, Cao W, Liu F, Gao Y, Chang Y, Xue C, Tang Q. 2021. The mechanism exploration of the non-colonic toxicity and obesity inhibition of food-grade κ-carrageenan by transcriptome. Food Sci Nutr. 9(11):6232–6244. doi: 10.1002/fsn3.2581.
- Zhang J, Sun Q, Teh JJ, Kang S, Hu J, Lin W, Trakman G, Hamilton A, Wilson-O'Brien A, Ching JYL, et al. 2022a. Food additive nanoparticles are associated with gut microbiome dysbiosis and targeted bacterial changes in Crohn’s disease: The ENIGMA study. J Gastroenterol Hepatol. 37(Suppl 1):113–114. Abstract no. 363. doi: 10.1111/jgh.15952.
- Zhang J, Sun Q, Teh JJ, Kang S, Hu J, Lin W, Trakman G, Hamilton A, Wilson O'Brien A, Ching JYL, et al. 2022b. Food additive nanoparticles is associated with specific pro-inflammatory bacterial changes in Crohn’s disease. The ENIGMA Study. United Eur Gastroenterol J. 10(Suppl 8):618–619. Abstract no. P0240. doi: 10.1002/ueg2.12290.
- Zhang J, Sun Q, Teh JJ, Kang S, Hu J, Lin WY, Trakman GL, Hamilton AL, Wilson-O’Brien A, Ching JY, et al. 2022c. Food additives intake is associated with microbiome dysbiosis and specific pro-inflammatory bacterial changes in Crohn’s disease. The ENIGMA Study. Gastroenterology. 162(Suppl 7):S-85. Abstract no. 397. doi: 10.1016/s0016-5085(22)60218-x.
- Zheng W, Xu D, Li J-Y, Wu X, Hu X, Gillilland M, Zhou S-Y, Owyang C. 2019. Dietary emulsifiers reduce thermogenesis in brown adipose tissue, leading to obesity and metabolic syndrome. Gastroenterology. 156(6 Suppl 1):S-50-S-51. Abstract no. 264. doi: 10.1016/s0016-5085(19)36908-2.
- Zheng W, Xu D, Wu X, Hu X, Li J-Y, Gillilland M, Zhou S-Y, Owyang C. 2020. Impaired thermogenesis in brown adipose tissue induced by dietary emulsifiers carboxymethyl cellulose is mediated by mucolytic bacteria and LPS endotoxemia. Gastroenterology. 156(6 Suppl 1):S-201. Abstract no. 1011. doi: 10.1016/s0016-5085(20)31187-2.
- Zijlstra FJ, Garrelds IM, van Dijk APM, Wilson JHP. 1992. Experimental colitis in mice: effects of olsalazine on eicosanoid production in colonic tissue. Agents Actions. Spec No: 36(Suppl 1):C76–C78. doi: 10.1007/BF01991028.
- Zinöcker MK. 2019. Tilsetningsstoffer og tarmhelse. Referat fra NSEs høstseminar: Tarmhelse, av Bøhler L og Pedersen IM. [Food additives and intestinal health. Minutes from Norsk Selskap for Ernæring (NSE)s [Norwegian Association for Nutrition]’s autumn seminar; Intestinal health, by Bøhler L and Pedersen IM]. Norsk Tidsskrift for Ernæring (NTFE). 17(4):1–5. Norwegian. doi: 10.18261/ntfe.17.4.17.
- Zinöcker MK, Lindseth IA. 2019. Tilsetningsstoffer – er vi føre var? [Food additives - are we taking precautions?] Tidsskr Nor Laegeforen. 139(12). Norwegian. doi: 10.4045/tidsskr.19.0474.
Appendices
Appendix A.
PRISMA-ScR checklist filled in for this scoping review
Preferred Reporting Items for Systematic reviews and Meta-Analyses extension for Scoping Reviews (PRISMA-ScR) Checklist
From: Tricco AC, Lillie E, Zarin W, O'Brien KK, Colquhoun H, Levac D, et al. PRISMA Extension for Scoping Reviews (PRISMAScR): Checklist and Explanation. Ann Intern Med. 2018;169:467–473. doi: 10.7326/M18-0850.
Appendix B.
First literature search
Scoping review on food additives and adverse effects on the intestines
Database: Ovid MEDLINE(R) and Epub Ahead of Print, In-Process, In-Data-Review & Other Non-Indexed Citations, Daily and Versions(R) <1946 to January 28, 2022>
Date: 31.01.22
Number of hits: 377
Database: Embase <1974 to 2022 January 28>
Date: 31.01.22
Number of hits: 527
Database: Scopus
Date: 31.01.22
Number of hits: 817
Database: Web of Science Core Collection (1987-present):
Science Citation Index Expanded (SCI-EXPANDED)--1987-present
Social Sciences Citation Index (SSCI)--1987-present
Arts & Humanities Citation Index (AHCI)--1987-present
Emerging Sources Citation Index (ESCI)--2017-present
Date: 31.01.22
Number of hits: 509
Database: Cochrane Database of Systematic Reviews Issue 1 of 12, January 2022
Cochrane Central Register of Controlled Trials Issue 12 of 12, December 2021
Date: 28.01.22
Number of hits: 22 (1 Cochrane review, 21 Trials)
Database: Epistemonikos
Comment: Simplified search strategy due to the limited search functionality
Date: 31.01.22
Number of hits: 66
(carrageen* or carragen* or carrhagen* or carragheen* or carrogeen* or genugel or killeen or satiagum or seakem or viscarin or burtonite or "processed Eucheuma seaweed" or "processed Eucheuma seaweeds" or "carboxylmethyl cellulose" or "carboxy methyl cellulose" or "carboxymethyl cellulose" or carboxymethylcellulose or aquacel or aquaplast or carmellose or "croscarmellose sodium" or cellolax or cethylose or polycell or polycel or ruspol or "cellulose gum" or CMC or almelose or apergel or blandlax or "bu lax" or carbethox or "carbose d" or carmellose or carmethose or "cel o brandt" or cellofas or eskalose or gelaxin or glycocellon or moventon or natulose or regucellulose or "sodium cellulose glycolate" or thylose or "tylose mga" or "tylose sodium" or "xylo mucine" or xylomucin) AND (intestin* or bowel* or gut or colon or rectum or "anal canal" or "anal canals")
Appendix C.
Repeated literature search
Scoping review on food additives and adverse effects on the intestines – repeated search
Database: Ovid MEDLINE(R) and Epub Ahead of Print, In-Process, In-Data-Review & Other Non-Indexed Citations, Daily and Versions <1946 to December 06, 2022>
Date: 07.12.22
Number of hits: 41
Database: Embase <1974 to 2022 December 06>
Date: 07.12.22
Number of hits: 73
Database: Scopus
Date: 07.12.22
Number of hits: 71
Database: Web of Science Core Collection (1987-present):
Science Citation Index Expanded (SCI-EXPANDED)--1987-present
Social Sciences Citation Index (SSCI)--1987-present
Arts & Humanities Citation Index (AHCI)--1987-present
Emerging Sources Citation Index (ESCI)--2017-present
Date: 08.12.22
Number of hits: 52
Database: Cochrane Central Register of Controlled Trials
Issue 11 of 12, November 2022
Cochrane Database of Systematic Reviews
Issue 12 of 12, December 2022
Date: 08.12.22
Number of hits: 2 (0 Cochrane review, 2 Trials)
Database: Epistemonikos
Comment: Simplified search strategy due to the limited search functionality
Date: 08.12.22
Number of hits: 15
(carrageen* or carragen* or carrhagen* or carragheen* or carrogeen* or genugel or killeen or satiagum or seakem or viscarin or burtonite or "processed Eucheuma seaweed" or "processed Eucheuma seaweeds" or "carboxylmethyl cellulose" or "carboxy methyl cellulose" or "carboxymethyl cellulose" or carboxymethylcellulose or aquacel or aquaplast or carmellose or "croscarmellose sodium" or cellolax or cethylose or polycell or polycel or ruspol or "cellulose gum" or CMC or almelose or apergel or blandlax or "bu lax" or carbethox or "carbose d" or carmellose or carmethose or "cel o brandt" or cellofas or eskalose or gelaxin or glycocellon or moventon or natulose or regucellulose or "sodium cellulose glycolate" or thylose or "tylose mga" or "tylose sodium" or "xylo mucine" or xylomucin) AND (intestin* or bowel* or gut or colon or rectum or "anal canal" or "anal canals")
Custom date range:
From: 01-02-22 To: 08-12-22
Appendix D.
Publications excluded at full-text level with reasons
Appendix E.
Eppi-reviewer Code book
Eppi-Reviewer Code book for scoping review on adverse effects on the intestines of CGN (E 407)/processed Eucheuma seaweed (E 407a) and CMC (E 466)