Abstract
Elastin is an important protein of the extracellular matrix of higher vertebrates, which confers elasticity and resilience to various tissues and organs including lungs, skin, large blood vessels and ligaments. Owing to its unique structure, extensive cross-linking and durability, it does not undergo significant turnover in healthy tissues and has a half-life of more than 70 years. Elastin is not only a structural protein, influencing the architecture and biomechanical properties of the extracellular matrix, but also plays a vital role in various physiological processes. Bioactive elastin peptides termed elastokines – in particular those of the GXXPG motif – occur as a result of proteolytic degradation of elastin and its non-cross-linked precursor tropoelastin and display several biological activities. For instance, they promote angiogenesis or stimulate cell adhesion, chemotaxis, proliferation, protease activation and apoptosis. Elastin-degrading enzymes such as matrix metalloproteinases, serine proteases and cysteine proteases slowly damage elastin over the lifetime of an organism. The destruction of elastin and the biological processes triggered by elastokines favor the development and progression of various pathological conditions including emphysema, chronic obstructive pulmonary disease, atherosclerosis, metabolic syndrome and cancer. This review gives an overview on types of human elastases and their action on human elastin, including the formation, structure and biological activities of elastokines and their role in common biological processes and severe pathological conditions.
Introduction
Elastin is an essential extracellular matrix (ECM) protein of vertebrates and the core protein of elastic fibers. It provides elasticity and resilience to many vertebrate tissues such as major blood vessels, lungs, skin, elastic ligaments, and cartilage and is, thus, critical for their long-term function. It possesses exceptional properties including elasticity, tensile strength and extreme durability, and has a unique chemical composition, containing large amounts of the four hydrophobic amino acids Gly, Ala, Val, and Pro. Elastin is secreted in the form of its monomeric precursor tropoelastin (TE) that occurs in different isoforms due to alternative splicing of its pre-mRNA. TE consists of alternating highly hydrophobic domains and more hydrophilic Lys-containing KA/KP domains, of which the hydrophobic regions are responsible for self-aggregation and tensile properties, while the latter are involved in cross-linking. Cross-linking transforms monomeric TE into polymeric elastin (Vrhovski and Weiss Citation1998; Akagawa and Suyama Citation2000; Mithieux and Weiss Citation2005) ().
Figure 1. Structure of elastic fibers with a microfibrillar mantle and an elastin core composed of cross-linked tropoelastin molecules. The color version of this figure is available online.
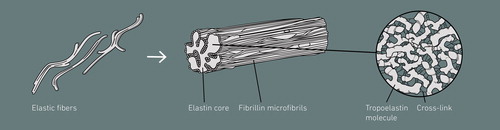
Elastic fibers are composed of an elastin core and a microfibrillar mantle (). Elastogenesis, the process of elastic fiber formation, is initiated by the assembly of fibrillin microfibrils, which serve as a scaffold for elastin deposition (Thomson et al. Citation2019). Translation of TE mRNA occurs on the surface of the rough endoplasmic reticulum. TE is hydroxylated at prolyl residues by prolyl 4-hydroxylase and is then transported through the Golgi apparatus to the cell surface. Before secretion, TE binds with its chaperon, the elastin-binding protein (EBP), and the EBP-TE complex is secreted (Hinek and Rabinovitch Citation1994; Davis and Mecham Citation1998; Vrhovski and Weiss Citation1998). In the extracellular space, TE is released from EBP and undergoes self-association in an endothermic, entropically driven liquid–liquid phase separation termed coacervation (Muiznieks et al. Citation2018). The alignment of TE and the subsequent cross-linking is promoted by fibulin-4, which mediates the association between TE and lysyl oxidase (LOX) (McLaughlin et al. Citation2006; Yanagisawa and Davis Citation2010; Nakamura Citation2018). Initially, allysine (α-aminoadipic acid-δ-semialdehyde) is produced through oxidative deamination of an ε-amino group of a Lys residue by LOX or LOX-like proteins. Intra- and inter-molecular cross-links are subsequently formed by non-enzymatic condensation, producing allysine aldol or dehydrolysinonorleucine, which further condense to form the stable and non-reducible cross-links desmosine and isodesmosine, unique to elastin (Eyre et al. Citation1984; Vrhovski and Weiss Citation1998; Akagawa and Suyama Citation2000; Mithieux and Weiss Citation2005). In most mammalian tissues, the major part of elastogenesis occurs in a small developmental window, reaching its maximum during early neonatal periods. In mature organs and tissues, elastin synthesis is repressed by posttranscriptional factors (Parks et al. Citation1993). Once formed, elastin shows virtually no turnover in healthy tissues, exhibiting a half-life greater than 70 years (Shapiro et al. Citation1991; Powell et al. Citation1992) due to its cross-linked nature, which is associated with a high resistance and durability. However, elastin accumulates damage over the lifetime of an organism as a result of aging processes induced by innate tissue degeneration, enzymatic activity and environmental influences, which may result in a loss of function of elastin or pathological conditions.
Elastin is not only a structure protein, but also plays an active role in various physiological processes (Debelle and Tamburro Citation1999). Some elastin-derived peptides (EDPs), which are liberated upon proteolytic degradation of elastin mainly from the hydrophobic domains (), promote angiogenesis (Robinet et al. Citation2005) and are associated with the regulation of various cell activities, including cell adhesion, chemotaxis, migration, proliferation, protease activation, and apoptosis () (Jung et al. Citation1998; Maquart et al. Citation2004; Moroy et al. Citation2005; Antonicelli et al. Citation2007). EDPs are also referred to as matrikines, that is, peptides with biological activities that are released through degradation of an ECM protein, or more specifically elastokines, that is, peptides with biological activities released by the action of enzymes from elastin (Maquart et al. Citation1999; Duca et al. Citation2004; Maquart et al. Citation2004, Citation2005). Both words, matrikine and elastokine, refer to the cytokine-like properties of the fragments of matrix proteins (Antonicelli et al. Citation2007). Studies suggest that EDPs containing the (X)GXXPG motif are biologically active, since these are able to interact with EBP (Mecham et al. Citation1989; Brassart et al. Citation2001). Proteolytic damage of elastic fibers upon aberrant expression of elastases, together with further biological processes triggered by elastokines, may contribute to the development and progression of severe pathological conditions including cardiovascular diseases such as atherosclerosis (Castiglione Morelli et al. Citation1997; Maurice et al. Citation2013) or lung emphysema (Houghton et al. Citation2006) (). This review aims to give a comprehensive overview on the formation of elastokines by elastases, the nature, biological role and structure-function relationship of elastokines, and their connection to pathological conditions.
Figure 2. Generation and effects of human elastokines. (A) Elastases derived from the classes of metalloendopeptidases, serine proteases, cysteine proteases and aspartic proteases cleave (B) human tropoelastin and elastic fibers, which leads to (C) the release of bioactive elastin peptides from the hydrophobic elastin domains. (D) The bioactive elastin peptides interact with different types of elastin receptors and (E) induce a variety of biological activities, which may eventually lead to (F) the development and/or progression of pathological conditions. Human tropoelastin (C) is show as isoform 2 (SwissProt accession number P15502-2). The color version of this figure is available online.
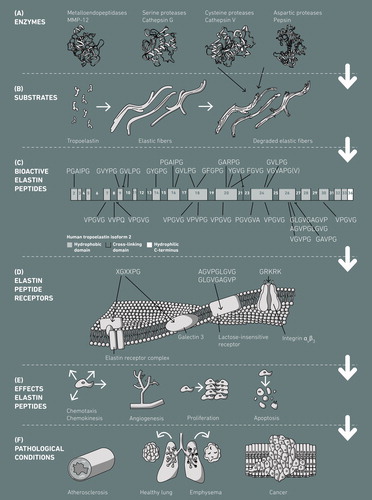
Release of elastokines through human elastases
Intact elastin is completely insoluble and extremely resistant toward intrinsic and extrinsic influences. However, during the lifetime of an organism, elastin is repetitively exposed to a variety of enzymatic, chemical and biophysical influences. Enzymatic degradation of elastin occurs during constitutive expression of proteases in healthy tissues and as a result of up-regulation of elastase expression during inflammatory conditions. Continuous exposure to elastases and concomitant release of elastokines may lead to serious and irreversible damage of elastic fibers, loss of elastic fiber function, chronic inflammation and an increase in morbidity and mortality through the development and progression of various cardiovascular diseases () (Antonicelli et al. Citation2007). Elastases that degrade elastin belong to the classes of matrix metalloproteinases (MMPs), aspartic proteases, serine proteases and cysteine proteases (), and their cleavage behavior with respect to elastin is summarized in . Werb et al. defined elastases as proteinases that are “able to solubilize and degrade insoluble elastin fibers alone” (Werb et al. Citation1982), and Bieth described an elastase as a protease capable of degrading mature cross-linked elastin (Bieth Citation1986). Both definitions do not include the fact that elastin cleavage by certain enzymes requires pre-damage of elastin, occurring as a result of aging processes, as has for instance been described for the serine protease human leukocyte elastase or the metalloendopeptidase neprilysin (Boudier et al. Citation1981; Schmelzer et al. Citation2012; Mora Huertas et al. Citation2018).
Table 1. Elastases and their cleavage specificities with respect to tropoelastin and elastin.
Digestive proteases
The aspartic protease pepsin (PEP; EC 3.4.23.1) degrades proteins in the stomach with a broad specificity (). It is secreted by the chief cells in the stomach in the form of its inactive precursor pepsinogen, which is activated by H+ in the stomach lumen, resulting in a conformational change and exposure of the catalytically active site. The unfolded active form of pepsinogen cleaves itself in an autocatalytic fashion, thereby generating PEP (Bhutia and Ganapathy Citation2018). When digests of skin elastin with PEP were analyzed, it was found that the protease frequently cleaves C-terminal to Gly, Val, Ala, Leu and Phe residues (Schmelzer et al. Citation2005). Overall, PEP has been reported to preferably cleave at hydrophobic and aromatic residues (Konigsberg et al. Citation1963; Magee Citation1974; Schmelzer et al. Citation2007).
Pancreatic secretions of the human gastrointestinal tract contain several serine proteases such as trypsin (TR; EC 3.4.21.4), chymotrypsin (CTR; EC 3.4.21.1) and pancreatic elastase (PE; EC 3.4.21.71), also known as chymotrypsin-like elastase 2A (Cela2A), which are all secreted as inactive precursors (Bhutia and Ganapathy Citation2018). Limited proteolysis of trypsinogen through intestinal enteropeptidases generates the active enzyme TR, which then releases CTR and PE from their precursors. CTR can completely degrade elastin, however has a narrower cleavage specificity than PE as it cleaves at Tyr, Trp, Phe and Leu residues, which occur in elastin only in small amounts (Loeven Citation1970; Bauer et al. Citation1976; Collins et al. Citation1985). Mass spectrometric analysis of elastin digests resulting from incubation of skin elastin with CTR further revealed that CTR produces larger and fewer peptides from elastin than PEP, which has a broader cleavage specificity (Getie, Schmelzer, Weiss, et al. Citation2005). Skin elastin is cleaved by CTR predominantly C-terminal to Tyr, Phe and Leu (Getie, Schmelzer, Weiss, et al. Citation2005; Gupta et al. Citation2010). PE is an aggressive elastase with a broad cleavage specificity and cleaves elastin C-terminal to Gly, Val, Leu, Ala and Ile and to lesser extent Phe, Pro, Glu and Arg (Powers et al. Citation1977; McRae et al. Citation1980; Mecham et al. Citation1997; Getie, Schmelzer, and Neubert Citation2005; Heinz et al. Citation2014). TR only cleaves C-terminal of Lys and Arg (Loeven Citation1970; Collins et al. Citation1985), which is difficult with 88% of elastin’s Lys residues being modified and involved in cross-links (Kozel et al. Citation2003). While TR does not cleave mature elastin well, it cleaves elastin’s non-cross-linked precursor TE with accessible Lys residues (Heinz et al. Citation2014).
Overall, it is unclear whether bioactive elastin peptides from animal food products released by digestive proteases are absorbed into the systemic circulation. While di- and tripeptides have been shown to be transported intact through the intestinal epithelium by H+-dependent peptide transporter 1 (PepT1), oligopeptides are not substrates for PepT1 (Fei et al. Citation1994; Vig et al. Citation2006). The paracellular pathway modulated by tight junctions and the transcellular pathway, however, have been shown to be involved in transepithelial absorption of peptides (Pauletti et al. Citation1997; Satake et al. Citation2002). Nevertheless, it is unlikely that the activity of such food-derived elastin peptides plays a significant role in the human body.
Metalloendopeptidases
MMPs form a large family of multidomain zinc- and calcium-dependent endopeptidases known to cleave various components of the ECM. MMPs play a central role in connective tissue remodeling and regulation of cell matrix composition through their effects on cell migration, cell differentiation, cell growth, wound healing, inflammation, angiogenesis, and apoptosis. The disruption of the physiological balance between MMP activation and deactivation is connected with severe diseases such as atherosclerosis, arthritis, pulmonary emphysema, myocardial infarction, as well as tumor growth and metastasis (Nagase and Woessner Citation1999; Sternlicht and Werb Citation2001; Visse and Nagase Citation2003; Sbardella et al. Citation2012). Five MMPs are known to cleave elastin: MMP-2 (EC 3.4.24.24), MMP-7 (EC 3.4.24.23), MMP-9 (EC 3.4.24.35), MMP-12 (EC 3.4.24.65) and MT1-MMP (MMP-14) (EC 3.4.24.80) (Sbardella et al. Citation2012; Van Doren Citation2015). MMP-2 (gelatinase A) plays an important role in ECM degradation during cell migration and tissue remodeling. It readily digests denatured different collagens, elastin, fibronectin and laminin (Gehrmann et al. Citation2002). MMP-7 (matrilysin 1) is mainly expressed by epithelial cells and processes various proteins, including various collagens, laminin, pro-tumor necrosis factor-α and α2-macroglobulin (Visse and Nagase Citation2003). MMP-9 (gelatinase B) is secreted by neutrophils and macrophages and has also been found in various malignant cells. It has, for instance, been shown to cleave different collagens, laminin, and plasminogen (Visse and Nagase Citation2003). MMP-12 (macrophage elastase) is expressed mainly by macrophages and cleaves a variety of substrates, including different collagens, laminin, pro-tumor necrosis factor-α, and plasminogen (Visse and Nagase Citation2003). MMP-14, the first membrane-type MMP identified on the surface of invasive tumor cells (Sato et al. Citation1994), digests ECM proteins such as fibronectin, laminin, various collagens and elastin (Imai et al. Citation1996; Will et al. Citation1996; Ohuchi et al. Citation1997; Koshikawa et al. Citation2000). With respect to the cleavage site specificities in elastin, it was found that MMP-7, -9 and -12 similarly tolerate hydrophobic and/or aliphatic amino acids, including Pro, Gly, Ile, and Val, at P1′ (). MMP-7 shows a strong preference for Leu at P1′, as do MMP-9 and MMP-12. Of all three MMPs, MMP-12 best tolerates bulky charged and aromatic amino acids at P1′. All three MMPs showed a clear preference for Pro at P3 (Netzel-Arnett et al. Citation1993; Nagase and Fields Citation1996; Gronski et al. Citation1997; Mecham et al. Citation1997; Heinz et al. Citation2010, Citation2011). The MMP-2 subsite preferences in P1′ are Leu > Ile/Phe > Val > Ala (Nagase and Fields Citation1996), while MMP-14 cleaves mainly N-terminal to Gly, Ala, Val and Leu in TE and elastin (Miekus et al. Citation2019).
Neprilysin (NEP; EC 3.4.24.11) is a type-II integral membrane glycoprotein and member of the M13 subgroup of zinc-dependent endopeptidases (Sexton et al. Citation2012). It is also known as skin fibroblast-derived elastase (Morisaki et al. Citation2010) and is widely expressed throughout mammalian tissues, including lungs, male genital tract, various epithelia and neural synapses (Sexton et al. Citation2012). It is mainly involved in extracellular catabolism of bioactive peptides such as enkephalins and atrial natriuretic peptide (Oefner et al. Citation2000), but also degrades components of the elastic fiber system (Szendroi et al. Citation1984; Morisaki et al. Citation2010). A recent study indicates that NEP may play a role in the elastolysis of elastin from old subjects (75 years), which has already undergone aging induced by intrinsic and extrinsic factors (described in more detail in section Aging), but not of elastin from young individuals (10 years). Through the release of elastokines and the overall enhanced activity of NEP in UV-induced extrinsic skin aging (Nakajima et al. Citation2012), the enzyme may contribute to ECM aging and break down in particular of elastic fibers that are pre-damaged through aging processes (Mora Huertas et al. Citation2018). NEP shows a strong preference for Gly at P1, while Gly, Ala and Val, but also Phe and Tyr were well accepted at P1′ during cleavage of TE and skin elastin (Mora Huertas et al. Citation2018). The cleavage site preferences are consistent with previous reports (Tiraboschi et al. Citation1999; Sexton et al. Citation2012).
Serine proteases
The neutrophil serine proteases human leukocyte elastase (HLE; EC 3.4.21.37), proteinase 3 (PR3; EC 3.4.21.76) and cathepsin G (CG; EC 3.4.21.20) are members of the chymotrypsin family, which is characterized by the presence of a catalytic triad consisting of His57, Asp102 and Ser195 (chymotrypsin numbering). They play a fundamental role in a variety of physiological processes including defense against infections, degradation of ECM components during inflammation processes, tissue remodeling and wound healing (Korkmaz et al. Citation2008). HLE, PR3 and CG are secreted by human polymorphonuclear neutrophils and are stored in their active forms in azurophilic granules, from which they are released in response to inflammation processes. HLE, also referred to as neutrophil elastase, catalyzes the degradation of elastin, different collagens, fibronectin and laminins, in addition to plasma proteins such as immunoglobulins (Owen and Campbell Citation1999). CG shows 37% sequence homology with HLE and cleaves a variety of substrates, including elastin, type IV collagen, fibronectin, laminins and plasma components such as immunoglobulins (Owen and Campbell Citation1999). PR3, also referred to as myeloblastin, shows 54% sequence homology with HLE and degrades elastin, type IV collagen, fibronectin and laminins (Owen and Campbell Citation1999). HLE and PR3 similarly cleave C-terminal to hydrophobic and/or aliphatic residues such as Ala, Gly and Val in elastin and TE () (Mecham et al. Citation1997; Heinz et al. Citation2012), which was also found in studies on model substrates (McRae et al. Citation1980; Rao et al. Citation1991; Brubaker et al. Citation1992; Koehl et al. Citation2003). CG has a broader cleavage specificity () and additionally cleaves C-terminal to bulky aliphatic and aromatic residues such as Leu, Phe and Tyr in elastin (Heinz et al. Citation2012) as well as Lys/Phe > Arg/Leu > Met > Ala > Asp in model substrates (Polanowska et al. Citation1998).
There are several studies that underline the importance of concerted action of enzymes during degradation of elastin. Schmelzer et al. showed that HLE does not degrade intact elastin fibers from young individuals, but hydrolyzes elastin derived from the skin of old individuals. CG, however, cleaves elastin fibers from both young and old individuals (Schmelzer et al. Citation2012). The results indicate that HLE is not the major driving force for elastolysis, but may still promote elastic fiber breakdown in concerted action with other enzymes such as CG. This has also been described by Boudier et al., who found that pre-digestion of human lung elastin by CG significantly enhances the elastolytic activity of HLE in a subsequent incubation (Boudier et al. Citation1981).
Chymotrypsin-like elastase 1 (Cela1; EC 3.4.21.36), also known as elastase 1, is a protease that is expressed in lung epithelial cells and macrophages during development and post-pneumonectomy lung regeneration. It has also been referred to as pancreatic elastase 1, however, it is not expressed in the pancreas. Recently, it has been shown that Cela1 plays a role in physiologic and pathologic stretch-dependent remodeling processes in the postnatal lung, in particular with respect to elastin fiber organization and function. In TE and elastin, Cela1 cleaves C-terminal to hydrophobic residues such as Gly > Ala > Val/Leu (Joshi et al. Citation2018).
Cysteine proteases
Cysteine proteases are members of the C1 family of papain-like enzymes, the best characterized family of cysteine peptidases. There are six cysteine proteases that are known to degrade elastin with differing elastolytic activity, namely cathepsin B, F, K, L, V and S (Turk et al. Citation2012) (). Cathepsin V was found to be the most potent elastase followed by cathepsins K, S, F, L and B (Bromme et al. Citation1996; Yasuda et al. Citation2004). Cathepsin K (CK; EC 3.4.22.38), also referred to as cathepsin O2, is highly expressed in osteoclasts, multinucleated giant cells, most epithelial cells, macrophages and in the synovial fibroblasts of rheumatoid arthritis joints (Punturieri et al. Citation2000; Alves et al. Citation2003). It plays an essential role in bone resorption, which has been shown in mice and humans (Asagiri and Takayanagi Citation2007) and degrades different collagens as well as elastin (Lecaille et al. Citation2008). Cathepsin B (CB; EC 3.4.22.1) is distributed all throughout the human body and is normally stored within lysosomes, acting on proteins that have entered the lysosomal system through endo- or phagocytosis (Mort and Buttle Citation1997). It is, however, also secreted as a proenzyme extracellularly and cleaves various other proteins such as different types of collagens and fibronectin. Its elastolytic activity is very low (Yasuda et al. Citation2004). Cathepsin F (CF; EC 3.4.22.41) is ubiquitously expressed in human tissues, which indicates a housekeeping function in cells (Wang et al. Citation1998), however only shows low elastolytic properties (Yasuda et al. Citation2004). Cathepsin L (CL; EC 3.4.22.15) is a ubiquitously expressed lysosomal protease responsible for intracellular protein breakdown. Extracellularly, it degrades different collagens, fibronectin and elastin (Mason et al. Citation1986; Lecaille et al. Citation2007; Novinec et al. Citation2007; Yang et al. Citation2007). Cathepsin V (CV; EC 3.4.22.43), also known as cathepsin L2, is mainly expressed in thymus, testis and corneal epithelium and may play a critical role in thymic self-antigen presentation (Bromme et al. Citation1999; Tolosa et al. Citation2003). It shows the strongest elastolytic activity of all mammalian cysteine proteases (Yasuda et al. Citation2004). Cathepsin S (CS; EC 3.4.22.27) is primarily expressed in lymphatic tissue such as spleen (Kirschke and Wiederanders Citation1994). It cleaves type I collagen, elastin and laminin when released into the ECM (Wang et al. Citation2006). With respect to the cleavage specificities in TE and elastin, it was found that CK, CS and CV prefer cleaving C-terminal to Gly ≫ Ala/Val. At P2, the three enzymes prefer Gly, Val, Ala and Pro (Panwar et al. Citation2020). For CK, it has been reported earlier in studies with model peptides and human bone collagen that Lys, Arg, Phe, Tyr, His and Try are preferred at P1, but Ala and Gly are also tolerated. CK accommodates hydrophobic residues such as Leu, Val, Ile and Pro at P2 (Atley et al. Citation2000; Alves et al. Citation2003). Experiments with CB, CL and CS on a proteomic peptide library revealed that all three proteases prefer Gly and Ala at P1. CL and CS show an additional preference for Gln at P1, while His and Pro are preferred at P2′and P3′. At P2, CB accommodates aromatic and aliphatic residues such as Try, Val, Ala and Gly, while CL prefers Phe, Tyr, Ile, Leu, Val and Trp (Biniossek et al. Citation2011). CS accommodates Phe, Leu, Ile as well as smaller residues such as Gly, Ala and Val at P2 (Bromme et al. Citation1989; Biniossek et al. Citation2011). CF was found to tolerate Phe and Leu at P2 (Wang et al. Citation1998).
Structure, receptors and biological effects of elastokines
TE consists of alternating hydrophobic and more hydrophilic domains, with the hydrophilic domains being involved in cross-linking. All known bioactive peptides are derived from the non-cross-linked hydrophobic domains (), whose building blocks are GX, PX, GGX and PGX, with X = G, A, V, L or I (). The hydrophobic domains are responsible for self-aggregation and tensile properties of elastin (Vrhovski and Weiss Citation1998). In particular domains 17–27, which contain the four biggest hydrophobic domains encrypting various elastokines, have been described to be key regions for coacervation of TE molecules during elastic fiber formation (Jensen et al. Citation2000; Dyksterhuis et al. Citation2007). These domains are solvent-exposed and it is, hence, likely that they are cleaved by elastases during ECM turnover, tissue aging and elastic fiber diseases, resulting in the release of elastokines.
Table 2. Bioactive elastin peptides from human elastin.
Structure of elastokines
In the 1980s, Senior et al. described chemotactic activity of peptides from HLE digests of bovine elastin (Senior et al. Citation1980), of TE (Senior et al. Citation1982) and of the sequence VGVAPG, a repeating peptide in TE (Senior et al. Citation1984). Structural studies on VGVAPG using nuclear magnetic resonance and circular dichroism spectroscopies suggested that the peptide occurs in folded conformations in organic solvents (Bisaccia et al. Citation1994; Castiglione Morelli et al. Citation1997). In later studies, it was found that the GXXPG sequence adopts a type VIII β-turn conformation, which is important with regards to binding of elastokines to EBP, a part of the elastin-receptor complex (ERC) that mediates the action of certain elastokines (Brassart et al. Citation2001; Fuchs et al. Citation2001). A few years later, molecular dynamics simulations on VGVAPG, (VGVAPG)2 and (VGVAPG)3 showed that VGVAPG exists in an equilibrium between different conformations including extended, polyproline II (PPII) and folded conformations such as the type VIII β-turn (Duca et al. Citation2004; Floquet et al. Citation2004). The repetition of VGVAPG leads to the formation of additional structures very close to a type VIII β-turn, and the authors hypothesized that the repeated VGVAPG sequence in domain 24 may favor elastin–cell interactions as VGVAPG is more likely to bind to EBP if it occurs in the TE sequence multiple times (Duca et al. Citation2004). With respect to other elastokines, it has been described that GXXP sequences, in which X is not Gly, are well able to adopt the type VIII β-turn conformation (Brassart et al. Citation2001; Fuchs et al. Citation2001; Moroy et al. Citation2005). Pro following a Gly (GPXPG) has been reported to stabilize the type VIII β-turn owing to its unique heterocyclic pyrrolidine ring structure (Moroy et al. Citation2005).
Elastin receptors
At the end of the 1980s, Hinek et al. and Mecham et al. showed that elastin binds to a peripheral membrane protein, termed EBP, via the VGVAPG repeat (Mecham et al. Citation1989). The authors further proposed that EBP is part of the ERC, constituting three proteins, two membrane-bound components (55 and 61 kDa) and the peripheral 67 kDa EBP (). They found that EBP has a biologically significant galactoside-binding site, which plays a role in elastic fiber assembly (Hinek et al. Citation1988; Mecham et al. Citation1989). A few years later, Hinek at al. identified strong homology between the EBP and an inactive, alternatively spliced version of β-galactosidase (β-Gal; EC 3.2.1.23) (Hinek et al. Citation1993), and it was later confirmed by Privitera et al. that both are identical (Privitera et al. Citation1998). It was further found that EBP protects TE from self-aggregation and proteolytic degradation during elastogenesis (Hinek and Rabinovitch Citation1994; Hinek Citation1995). EBP also possesses galactolectin properties, that is, binds β-galactosugars such as galactose or lactose, and it was suggested that elastin-EBP interactions may be allosterically regulated by β-galactosugars bound to EBP (Hinek et al. Citation1988; Mecham et al. Citation1989). With respect to the other two sub-units of ERC, Hinek et al. proposed and later confirmed that these correspond to the proteins known to interact with β-Gal in the lysosomes, that is, neuraminidase (Neu-1; EC 3.2.1.18) and cathepsin A/protective protein (PPCA; EC 3.4.16.1) (Hinek Citation1996; Hinek et al. Citation2006). The function of PPCA in lysosomes is to protect β-Gal and Neu-1 from intralysosomal digestion by forming a complex with the two other enzymes (Callahan Citation1999), while the sialidase Neu-1 catalyzes the hydrolysis of terminal sialic acid residues of oligosaccharides, glycoproteins and glycolipids (Achyuthan and Achyuthan Citation2001). In addition to its important role in elastin assembly (Hinek et al. Citation2006), the ERC transduces intracellular signals and mediates the action of elastokines (Duca et al. Citation2004). Duca et al. have shown that EDPs stimulate the production of pro-MMP-1 in human skin fibroblasts through activation of the extracellular signal-regulated kinase (ERK) 1/2 pathway, and the Neu-1 sub-unit is responsible for signal transduction, presumably through sialic acid generation from undetermined substrates (Duca et al. Citation2002, Citation2005, Citation2007). Neu-1 catalyzes the local conversion of the monosialodihexosylganglioside into lactosylceramide, a critical second messenger of ERC signaling pathways (Rusciani et al. Citation2010). Recently, two segments in human Neu-1 (139–159 and 316–333) have been identified as transmembrane domains, controlling dimerization and sialidase activity of Neu-1 (Maurice et al. Citation2016). The biological effects of EDPs are not only mediated through binding to the cell surface receptor ERC, but also through a galectin-3 receptor (Pocza et al. Citation2008), integrins αvβ3 and αvβ5 (Rodgers and Weiss Citation2005; Bax et al. Citation2009; Lee et al. Citation2014) and a lactose-insensitive elastin receptor (Maeda et al. Citation2007) (). Galectin-3 is strongly expressed in neoplastic cells and is involved in tumor metastasis (Bresalier et al. Citation1996). Integrins αvβ3 and αvβ5 bind ECM proteins such as fibrinogen, vitronectin and fibronectin mainly through the typical RGD integrin binding motif. In the case of TE, binding has been shown to occur via the GRKRK C-terminus with integrin αvβ3 (Bax et al. Citation2009) and via the central part (domains 17 and 18) of the TE molecule with integrin αvβ5 (Lee et al. Citation2014). Both interactions lead to cell adhesion to TE.
Overall, there are 17 GXXPG sequences in elastin, 9 of which have been shown to display biological activities (, ), while 8 have not been investigated on potential bioactivities except for GGVPG, which has shown to not be chemotactic to monocytes (Castiglione Morelli et al. Citation1997). In addition to GXXPG-based elastokines, further peptides have been described to display biological activities, including VVPQ (Spezzacatena et al. Citation2005), VPGVG (Wachi et al. Citation1995), VGVPG (Castiglione Morelli et al. Citation1997), PGVGVA (Hauck et al. Citation1995) and the nonapeptides GLGVGAGVP (Long et al. Citation1989) and AGVPGLGVG (Maeda et al. Citation2007).
Chemokinetic and chemotactic activity
Cells may respond to a migration-enhancing factor either by directed movement in the process chemotaxis or by random, multidirectional movement in the process of chemokinesis (). Senior et al. described the chemotactic properties of VGVAPG, which induces fibroblast and monocyte migration (Senior et al. Citation1984). Long et al. later showed that GLGVGAGVP and VGVAPG are chemoattractants for bovine aortic endothelial cells (Long et al. Citation1989). In 1993, Grosso et al. confirmed that VGVAPG, but also PGAIPG and GAIPG induce fetal bovine nuchal ligament fibroblast cell migration, while VGVPG, a highly repeated pentapeptide in TE, did not result in cell migration. PGAIPG was found to be chemotactic, and GAIPG was chemokinetic (Grosso and Scott Citation1993a). The presence of lactose inhibited the fibroblast chemotaxis induced by both PGAIPG and VGVAPG, however, did not affect cell migration induced by GAIPG. These findings lead the authors to the conclusion that not only VGVAPG (Mecham et al. Citation1989), but also PGAIPG is a ligand of EBP, while GAIPG does not seem to display its biological activity through interaction with EBP (Grosso and Scott Citation1993a). In another study, Grosso et al. showed that PGAIPG is chemotactic to neutrophils and Lewis lung carcinoma tumor cells, which was also found to be mediated by EBP (Grosso and Scott Citation1993b). Chemokinetic activity and migration of monocytes induced by GLVPG and YGVG has been shown by Bisaccia et al., who also demonstrated chemotactic activity of FGVG, GVAPG and VGVAPG (Bisaccia et al. Citation1994). Castiglione Morelli et al. confirmed that VGVAPG and GVAPG as well as VGVPG are chemotactic to monocytes, with the action of VGVAPG and VGVPG being mediated by EBP, while the action of GVAPG seemed to be mediated by another receptor (Castiglione Morelli et al. Citation1997). Monocyte chemotaxis of VGVAPG in response to VGVAPG was also confirmed by Hance et al. (Hance et al. Citation2002). It was also shown by Uemura et al. that an EDP from oxalic acid degradation of bovine elastin displays chemotactic activity toward monocytes (Uemura and Okamoto Citation1997). Robinet et al. described enhanced human endothelial cell migration in response to the action of VGVAPG (Robinet et al. Citation2005). Maeda et al. demonstrated that AGVPGLGVG is chemotactic to macrophages, which is mediated by EBP, but also by another yet unknown receptor (Maeda et al. Citation2007). In 2008, VGVAPG and VAPG were found to be chemotactic to melanoma cells, which is mediated by galectin-3 and integrin ανβ3 receptors (Pocza et al. Citation2008). Coquerel et al. described migration of glioblastoma cells through the healthy brain parenchyma during brain tumor development induced by (VGVAPG)3 and κ-elastin (Coquerel et al. Citation2009), a mixture of EDPs derived from treatment of elastin with potassium hydroxide (Jacob and Hornebeck Citation1985). Bovine and porcine EDPs were further found to stimulate the migration of fibroblasts (Shiratsuchi et al. Citation2010). Recently, Desforges et al. showed that VGVAPG induces extravillous trophoblast migration during pregnancy, aiding the process of uterine spiral artery remodeling (Desforges et al. Citation2015).
Stimulation of protein expression
The release of elastokines, which induce the expression of proteases, may accelerate the progression of pathological conditions, inducing chronic inflammation (Antonicelli et al. Citation2007). Brassart et al. showed that VGVAPG, GVAPGV and PGAIPG stimulate pro-MMP-1 expression, and VGVAPG further causes up-regulation of pro-MMP-3 in human skin fibroblast cell culture. This was inhibited by lactose, indicating that the biological effect of the peptides is mediated through binding to EBP (Brassart et al. Citation2001). VGVAPG, PGAIPG and κ-elastin were also shown to enhance EBP-mediated MMP-2 and MMP-3 production (Huet et al. Citation2001), and it was further found that EDPs from κ-elastin up-regulate the pro-MT1-MMP and pro-MMP-2 expression (Robinet et al. Citation2005). Pro-MT1-MMP up-regulation through elastokines is EBP-mediated and involves activation of the MEK1/2/Erk1/2 pathway via PI3-kinase/Akt- and NO-dependent signaling (Fahem et al. Citation2008). In contrast, enhanced expression of MMP-2 and MMP-3 through the action of VGVAPG and VAPG is mediated by galectin-3 and EBP receptors (Pocza et al. Citation2008). In vitro tests have revealed that GVYPG, GVLPG and GFGPG stimulate the pro-MMP-1 secretion (Heinz et al. Citation2012), while GYGPG and GARPG induce the pro-MMP-2 secretion (Heinz et al. Citation2010). (VGVAPG)3 increases MMP-2 and MMP-9 secretion and MMP-12 synthesis as well as expression of the TE gene (Coquerel et al. Citation2009). Szychowski et al. recently described that VGVAPG enhanced mRNA expression of tissue inhibitor of MMP-2 (Timp-2) and Timp-3 genes in an EBP-dependent manner. The authors further found that mRNA expression of MMP-2, MMP-9 and Timp-4 were only partially EBP-dependent and the decrease in mRNA expression of Timp-1 was EBP-independent (Szychowski, Wójtowicz, et al. Citation2019).
EDPs do not only induce the up-regulation of elastases, but also of other proteins, which play a role during cardiovascular diseases and cancer progression. VGVAPG and VAPG induce the expression of C-X-C chemokine receptor type 4 (CXCR-4), C-X-C motif chemokine ligand 12 (CXCL-12) and vascular epidermal growth factor C (VEGF-C), which is mediated by interactions with the galectin-3 and integrin ανβ3 receptors and is associated with melanoma progression (Pocza et al. Citation2008). EDPs from κ-elastin and VGVAPG were found to up-regulate EBP on mRNA and protein level, which was associated with cytokine production on human peripheral blood lymphocytes and CD4+ T cell subpopulations, suggesting a role of EDPs in Th-1 differentiation and cytokine regulation in atherosclerosis, where a Th-1 immune response predominates (Debret et al. Citation2005).
Proliferation
Wachi et al. demonstrated that VPGVG and (VPGVG)n induce proliferation of smooth muscle cells and reduce elastin synthesis and its mRNA levels (Wachi et al. Citation1995), and Kamoun et al. described a proliferative effect of VGVAPG on human skin fibroblasts (Kamoun et al. Citation1995), which was confirmed by Tajima et al., who additionally demonstrated a reduction of elastin mRNA levels for VGVAPG (Tajima et al. Citation1997). Mochizuki et al. demonstrated that cultured smooth muscle cells from porcine coronary arteries up-regulate their proliferation after exposure to κ-elastin or VGVAPG in an EBP-mediated manner. In vascular diseases, the local accumulation of TE and EDPs may lead to the activation of medial smooth muscle cells into a proliferative and migratory phenotype, which participates in the formation of the occlusive arterial lesions (Mochizuki et al. Citation2002). In 2010, Shiratsuchi et al. described that EDPs obtained by treating elastin with oxalic acid enhance the proliferation of fibroblasts (Shiratsuchi et al. Citation2010). Human lymphocytes also express EBP and show increased proliferation and increased production of a serine protease in the presence of EDPs. T-lymphocytes are present in atherosclerotic plaques, where elastin degradation occurs, and may contribute to the chronicity of atherosclerotic lesions (Peterszegi et al. Citation1996). Recently, Szychowski described an antiproliferative effect as a result of an increase in the reactive oxygen species levels by VGVAPG in neuroblastoma cells with a yet unknown mechanism of action (Szychowski, Rombel-Bryzek, et al. Citation2019).
Angiogenesis
Nackman et al. found that the administration of VGVAPG or elastase in a rat aneurysm model leads to induced adventitial angiogenesis (Nackman et al. Citation1997). Robinet et al. showed that the occupancy of EBP by EDPs from κ-elastin and VGVAPG on vascular endothelial cells triggered neoangiogenesis, which was associated with pro-MT1-MMP and pro-MMP-2 up-regulation and may play role in the progression of atherosclerotic abdominal aortic aneurysm (Robinet et al. Citation2005). Fahem et al. confirmed angiogenic effects of EDPs using (VGVAPG)3, which induced MT1-MMP expression through NO production mediated by EBP (Fahem et al. Citation2008). MT1-MMP has been shown to play a major role in angiogenesis (Seiki and Yana Citation2003). VGVAPG and VAPG increase the angiogenic potential of melanoma cells by enhancing the expression of VEGF-C, which is mediated by the binding of EDPs to the galectin-3 receptor (Pocza et al. Citation2008). Gunda et al. confirmed angiogenic activity of κ-elastin and VGVAPG in choroidal endothelial cells, which is inhibited by a type IV collagen fragment, showing potential for the treatment of diseases involving choroidal neovascularization (Gunda et al. Citation2013).
Apoptosis
Aging is associated with accumulation of ceramides in tissues, which eventually induce apoptosis () (Cutler and Mattson Citation2001). Cantarelli et al. showed that EDPs antagonize ceramide-induced apoptosis in fibroblast cell culture, which is mediated by EBP (Cantarelli et al. Citation2009). Péterszegi et al. described the opposite effect for EDPs from κ-elastin with regards to EBP-mediated apoptosis. In low concentrations (l–10 µg mL−1) of κ-elastin, a stimulation of lymphocyte proliferation and elastase up-regulation were observed, while at higher concentrations (100 µg mL−1), lymphocyte proliferation and elastase production decreased and apoptotic cell death increased (Peterszegi and Robert Citation1998; Peterszegi et al. Citation1999).
Adhesion to cells
Interactions between ECM macromolecules and tumor cells play a major role in cancer progression, in particular metastasis formation. Blood et al. demonstrated that VGVAPG binds to lung carcinoma cells (Blood et al. Citation1988). Timar et al. found that native elastin and κ-elastin adhere to cancer cell lines, namely lung carcinoma cells and amelanotic melanoma cells, which may be associated to intra- and extravasation of invasive tumor cells (Timar et al. Citation1991). Svitkina and Parsons also showed that fibrous elastin and EDPs bind to lung carcinoma, breast carcinoma, melanoma and prostate cancer cells (Svitkina and Parsons Citation1993), and Jung et al. demonstrated that κ-elastin binds to astrocytoma cell lines (Jung et al. Citation1999). VGVAPG and VAPG were shown to bind melanoma cells (Pocza et al. Citation2008). Binding of purified elastin fibers to human skin fibroblasts and porcine aortic smooth muscle cells (Hornebeck et al. Citation1986), and EBP-mediated binding of κ-elastin to fibroblasts have also been demonstrated (Groult et al. Citation1991). EBP-mediated binding of α-elastin, soluble elastin obtained after treatment with 0.25 M oxalic acid at 100 °C (Partridge et al. Citation1955), to cardinal ligament fibroblasts has also been described (Yamamoto et al. Citation2002). Bax et al. revealed that cell adhesion to human TE is mediated via the C-terminal GRKRK motif in TE and the integrin αvβ3 receptor on the fibroblast surface. The interaction was found to be independent of EBP (Bax et al. Citation2009).
Other biological activities
Faury et al. described effects of TE and EDPs on vascular tone. TE, κ-elastin, VGV, PGV and VGVAPG induced an EDP-mediated endothelium- and dose-dependent vasorelaxation and increased endothelial Ca2+ concentrations (Faury et al. Citation1995, Citation1998). Spezzacatena et al. described mitogenic activity of VVPQ in human dermal fibroblasts (Spezzacatena et al. Citation2005), and Simionescu et al. reported that EDPs induce an EDP-mediated osteogenic response in vascular smooth muscle cells, which exhibited an increased expression of bone proteins including core binding factor α-1, osteocalcin and alkaline phosphatase. This may contribute to vascular calcification (Simionescu et al. Citation2005). Karnik et al. described that TE activates myofibrillar organization of vascular smooth muscle cells. VGVAPG was found to induce myofibrillogenesis in a time- and dose-dependent fashion, and both VGVAPG and TE stimulate actin polymerization. The authors suggested that unidentified cell surface receptor different from EBP mediates the described effects (Karnik et al. Citation2003).
Elastases, elastokines and their roles in aging and pathological conditions
Aging
Aging processes are characterized by progressive deterioration of the integrity of organs and tissues induced by intrinsic and extrinsic factors such as innate tissue degeneration and environmental influences including UV radiation, smoking and air pollution (Braverman and Fonferko Citation1982; Naylor et al. Citation2011; Vierkötter and Krutmann Citation2012). With respect to ECM proteins, it has recently been shown that there is a direct causal link between the exposure to UV radiation, reactive oxygen species and structural damage to fibrillin microfibrils and fibronectin (Hibbert et al. Citation2019) and that collagen VI alpha-3 and fibrillin-1 or become more proteolytically susceptible after having been exposed to UV radiation (Eckersley et al. Citation2020). Another study investigated the influence of extrinsic and extrinsic aging on the morphology and susceptibility of elastin toward enzymatic degradation. Morphological differences in the elastin fibers were found between samples from young and old individuals, and marker peptides were identified, which revealed clear differences in the enzymatic susceptibility between samples from sun-exposed regions or non-sun exposed regions of the body of young and old individuals (Mora Huertas et al. Citation2016). In fact, elastin accumulates damage during aging over the lifespan of an organism due to its low turnover and effects including enzymatic degradation (Antonicelli et al. Citation2007), oxidative damage (Watanabe et al. Citation1996), formation of advanced glycation endproducts (Paul and Bailey Citation1996), calcification (Proudfoot and Shanahan Citation2001), aspartic acid racemization (Powell et al. Citation1992; Sivan et al. Citation2012), lipid accumulation (Robert et al. Citation2008), carbamylation (Gorisse et al. Citation2016) and mechanical fatigue (O’Rourke Citation2007). Aging of elastin involves fragmentation and thinning of elastin structures and may result in impaired elastic fiber function, reduced tissue elasticity, chronic inflammation or eventually loss of function of tissues and organs, considerably increasing morbidity and mortality (Green et al. Citation2014). Overall, all the mentioned factors further enhance elastin’s susceptibility to proteolysis by specific elastases, triggering processes induced by elastokines, which often contribute to the development and progression of various cardiovascular diseases or cancer () (Baud et al. Citation2013; Duca et al. Citation2016).
Cardiovascular diseases
Atherosclerosis, ascending thoracic aortic aneurysm (ATAA), abdominal aortic aneurysm (AAA), heart insufficiency or heart arrest often occur as a result of aging-related damage to elastin in blood vessels (Antonicelli et al. Citation2007; Robert et al. Citation2008). AAA and ATAA involve structural deterioration, enlargement and eventual rupture of the aorta. Both pathologies are triggered by factors including inflammation in the arterial wall, the overproduction of proteolytic enzymes such as MMP-1, -2, -3, -8, -9, -12, -13, -14 and -19 and a decrease of some of their inhibitors (Loftus and Thompson Citation2002; Choudhury et al. Citation2009; Rabkin Citation2017). Those MMPs degrade components of the ECM that normally maintain the arterial structure such as different collagens, elastin, laminin, proteoglycans and fibronectin (Rabkin Citation2017), which in the case of elastin leads to a release of elastokines. In AAA tissue, EDPs attract mononuclear phagocytes through interactions with EBP, thereby enhancing the inflammatory response that accompanies aneurysmal degeneration (Hance et al. Citation2002). It has also been shown that infusion of VGVAPG into rats induces aneurysms by stimulating adventitial angiogenesis (Nackman et al. Citation1997). Atherosclerosis is a progressive inflammatory disease characterized by arterial wall thickening due to the formation of atherosclerotic plaques. Plaque growth and subsequent narrowing of the arterial lumen are induced by cytokines and growth factors and further deposition as well as degradation of ECM components. Several elastinolytic and collagenolytic MMPs, including MMP-1, -2, -3, -7, -9, -12, -13 and -14, are involved in the degradation of the fibrous plaques, leading to plaque rupture and ischemic events (Loftus and Thompson Citation2002; Katsuda and Kaji Citation2003). Alongside elastin degradation, dysfunctional elastogenesis has been described to occur during atheroma formation, leading to the formation of TE plaques which fail to cross-link into functional elastic fibers (Phinikaridou et al. Citation2018). These TE plaques may be degraded easily, releasing elastokines. EDPs have been shown to accelerate the progression of atherosclerosis in a ERC-mediated manner by further triggering the production of elastinolytic enzymes (Brassart et al. Citation2001; Huet et al. Citation2001; Petersen et al. Citation2002; Robinet et al. Citation2005), resulting in a vicious circle between EDP release and elastase up-regulation. Elastokines have further been described to enhance lipid oxidation and calcification of the vascular wall (Maurice et al. Citation2013). In addition, elastokines display further effects that may enhance atherogenesis such as monocyte chemotaxis (Castiglione Morelli et al. Citation1997), free radical release, LDL oxidation and endogenous cholesterol production (Fulop et al. Citation1998). Chronic injections of EDPs or VGVAPG in mice revealed an increase in atherosclerotic plaque size in these mice (Gayral et al. Citation2014). A more detailed review on the involvement of EDPs in the development and progression of cardiovascular diseases can be found elsewhere (Wahart et al. Citation2019).
Lung emphysema and chronic obstructive lung disease
Chronic obstructive lung disease (COPD) is characterized by chronic inflammation, parenchymal destruction and respiratory symptoms such as shortness of breath and airflow limitations. The disease is caused by exposure to noxious particles and gases such as tobacco smoke or air pollution (Perng and Chen Citation2017). Emphysema, a major symptom of COPD, is associated with constant enlargement of the peripheral airspaces of lung bronchioles as a result of the destruction of alveolar wall matrix structures (Houghton Citation2015). It is caused by up-regulation of proteases such as MMPs, serine proteases and cysteine proteases including MMP-1, -2, -8, -9, -12, -13 and -14, HLE, as well as cathepsins K, L and S, which all display elastinolytic and collagenolytic properties (Tetley Citation2002; Elkington and Friedland Citation2006; Lagente et al. Citation2009; Pandey et al. Citation2017). EDPs have been identified as a driving force in the progression of the disease through recruitment of monocytes, release of MMP-12 and degradation of elastin, which releases further elastokines leading to the formation of a vicious circle (Houghton et al. Citation2006). It has also been proposed that the continuous release of EDPs as a result of cleavage by serine proteases and MMPs may be associated with the activation of elastin-specific B and and Th1 cells, which trigger the secretion of MMP-12 and HLE, leading to inflammation and massive elastolysis (Lee et al. Citation2007).
Diabetes
Insulin signaling primarily regulates glucose homeostasis, while insulin signaling impairment leads to insulin resistance, which is connected to type II diabetes, obesity and further cardiovascular disorders (Martin et al. Citation1992). Glucose homeostasis has been shown to be influenced by modulation of sialic acid levels in adipocyte culture. Since sialidase activity increases after binding of EDPs to ERC, it has been hypothesized that EDPs may be involved in the development of insulin resistance in mice. It was indeed recently shown that acute or chronic intravenous injections of EDPs in mice induced hyperglycemic effects associated with glucose uptake reduction and insulin resistance in skeletal muscle, liver and adipose tissue. The proposed mechanism of action includes interaction of the Neu-1 subunit of ERC with the insulin receptor triggered by binding of EDPs to ERC (Blaise et al. Citation2013). EDP release may be stimulated by HLE, whose action has been described to induce insulin resistance in mice (Talukdar et al. Citation2012).
Nonalcoholic fatty liver disease
Nonalcoholic fatty liver disease (NAFLD) is characterized by various symptoms from steatosis, hepatocyte injury and inflammation leading to the development of nonalcoholic steatohepatitis (NASH), which is associated with fibrosis and cirrhosis. The exact cause of NAFLD is not yet know although predisposing factors such as obesity, insulin resistance, metabolic syndrome and aging including lifestyle and Western diet have been described (Fontana et al. Citation2013). In a recent study, Romier et al. describe that the accumulation of EDPs as a result of enzymatic cleavage of elastin, for instance by HLE, induces hepatic lipogenesis, inflammation and fibrosis through enhanced collagen and elastin expression in mice. These effects were described for treatment of mice with κ-elastin and involve inhibition of the LKB1-AMPK pathway as a result of ERC activation, and it was found that EDPs promote the transition from NAFLD to NASH. EDP effects were reduced by inhibition of HLE, ERC inhibitors and EDP inhibitors (Romier et al. Citation2018).
Cancer
Elastinolytic activity of tumors has been described in various studies (Hornebeck et al. Citation1978; Jones and DeClerck Citation1980; Werb et al. Citation1982; Caenazzo et al. Citation1990). It has further been shown that the metastatic potential of various cancer types including breast, colon, head and neck cancers or melanoma is associated with MMP expression and matrix degradation (Duffy Citation1996). Melanoma progression is a multifactorial process that involves chronic inflammation and cell invasion through the ECM after degradation of ECM components, in particular by MMP-1, -2, -13 and -14 (Hofmann et al. Citation2000). Fragmentation of elastin was found to mainly occur at the invasive front of melanoma cells, and EDPs enhanced MMP-2 and -14 production by melanoma cells in an ERC-mediated manner, which in turn allowed further melanoma cell invasion through the ECM (Ntayi et al. Citation2004). EDPs were also found to lead to IL-1β mRNA and protein up-regulation after interaction with EBP. IL-1β is a pleiotropic cytokine that exacerbates invasiveness, adhesion, angiogenesis, and spreading of metastases during melanoma progression (Debret et al. Citation2006). VGVAPG and VAPG bind directly to galectin-3, integrin αvβ3 and EBP and contribute to melanoma progression as they are chemotactic for melanoma cells, increase the expression of CXCR-4 and CXCL-12 chemokines, enhance the expression of ECM-degrading MMP-2 and -3, increase the attachment of melanoma cells and the expression of different adhesion molecules (Pocza et al. Citation2008). EDPs, TE or XGVAPG peptides have also been found to trigger pro-MMP-1 overproduction by HT-1080 fibrosarcoma cells at the protein and enzyme levels (Huet et al. Citation2002). EDPs further increase MMP-2 production by human dermal fibroblasts, stimulate fibroblast chemotaxis, lead to expression of both MMP-1 and -3 and accelerate tumor angiogenesis (Kamoun et al. Citation1995; Nackman et al. Citation1996; Brassart et al. Citation2001), which all play a role in melanoma progression. κ-elastin and (VGVAPG)3 have also been shown to increase the invasiveness of glioma brain tumor cells alongside with an enhanced MMP-2 secretion and MMP-12 synthesis in an EBP-mediated manner (Coquerel et al. Citation2009). VGVAPG is chemotactic for cells able to colonize the lung (Blood et al. Citation1988; Blood and Zetter Citation1989). PGAIPG was also demonstrated to be chemotactic for rodent tumor cells (Grosso and Scott Citation1993b). There is some controversy in the literature concerning the question whether elastin can influence proliferation of tumor cells (mitogenic signaling). EDPs have also been described to induce cell cycle entry of human glioma cells (Jung et al. Citation1998; Hinek et al. Citation1999). More recently, it has been shown that κ-elastin increases the invasive capacities of lung tumor cells by stimulating MMP-2 and urokinase plasminogen activator release mediated by a lactose-insensitive receptor (Toupance et al. Citation2012). Moreover, κ-elastin and VGVAPG have been described to stimulate heat-shock protein 90 up-regulation as well as MMP-2 and urokinase plasminogen activator accumulation, thereby strongly potentiating fibrosarcoma cell migration and matrix invasion capacities (Donet et al. Citation2014).
Conclusions and future perspectives
Due to its hydrophobicity and extensive cross-linking, the important extracellular matrix protein elastin is insoluble and highly resistant to proteolytic degradation, exhibiting a half-life of more than 70 years. However, in connection with its slow turnover, elastin accumulates aging- and disease-related damage, which may lead to loss of function of elastin and, hence, failure of elastin-rich organs and tissues. In addition to extrinsic factors such as UV radiation, smoking and air pollution, intrinsic influences including the action of elastases accelerate aging of elastin. Over the last decades until quite recently, 20 human elastases have been identified derived from the enzyme classes of aspartic, metallo, serine and cysteine endopeptidases. The action of these elastases on elastin not only directly contributes to intrinsic aging processes, which are associated to structural damage of elastin, but also leads to the release of bioactive peptides, so-called elastokines. Elastokines promote angiogenesis and are connected with the regulation of various cell activities, including cell adhesion, chemotaxis, migration, proliferation, protease activation, and apoptosis. An aberrant expression of elastases together with the biological processes triggered by elastokines may support the development and progression of various pathological conditions including atherosclerosis, emphysema and chronic obstructive pulmonary disease.
Since the pioneering work of Senior et al. in the early 1980s, there has been an increasing research activity around elastokines and their biological activities as well as their connection with pathological conditions. While the signaling pathways of elastokine effects mediated by the elastin receptor complex have been and are being investigated, not much is known about the interactions and signaling of elastokines with the other elastin receptors, such as galectin-3 as well as integrins αvβ3 and αvβ5, and there is still an unknown lactose-insensitive elastin receptor. Future research focusing on these receptors would help to better understand the action of elastokines and potentially allow developing strategies to inhibit their biological activities that are associated with cardiovascular diseases. In addition, there are still a lot of unanswered questions regarding the connection between elastokines and the development of diseases as well as the roles of different elastases and their interplay with elastokines. A lot of research is, therefore, currently being carried out in the field of vascular aging, metabolic syndrome and cancer development, and more and more knowledge will be gained in the next couple of years and may help finding options to slow down the progression of severe cardiovascular diseases. Another interesting research focus could be directed toward beneficial effects of elastokines, such as angiogenesis or immune cell migration, to investigate their effects on matrix remodeling during tissue regeneration and wound healing.
To summarize, even after 40 years of research on elastokines, it is still of great importance to understand and characterize elastin-degrading processes, including the cleavage behavior of elastinolytic enzymes as well as the action of the peptides released upon degradation. This approach may aid in the development of directed therapies to treat pathologies related to elastin degradation, the overexpression of elastases and the subsequent release of elastokines. Such therapies may include for instance selective enzyme inhibitors or inhibitors that hinder the interaction of elastokines with their signaling receptors. In the case of elastokines with beneficial effects, drug delivery systems containing elastokines may be a useful for example to treat wounds.
Abbreviations | ||
β-Gal | = | β-galactosidase |
CB | = | cathepsin B |
Cela1 | = | chymotrypsin-like elastase 1 |
Cela2A | = | chymotrypsin-like elastase 2A |
CF | = | cathepsin F |
CG | = | cathepsin G |
cGMP | = | cyclic guanosine monophosphate |
CL | = | cathepsin L |
COPD | = | chronic obstructive pulmonary disease |
CS | = | cathepsin S |
CTR | = | chymotrypsin |
CV | = | cathepsin V |
CXCL-12 | = | C-X-C motif chemokine ligand 12 |
CXCR-4 | = | C-X-C chemokine receptor type 4 |
EBP | = | elastin binding protein |
ECM | = | extracellular matrix |
EDP | = | elastin-derived peptide |
ERC | = | elastin receptor complex |
ERK | = | extracellular signal-regulated kinase |
HLE | = | human leukocyte elastase |
LOX | = | lysyl oxidase |
MMP | = | matrix metalloproteinase |
mRNA | = | messenger ribonucleic acid |
NAFLD | = | nonalcoholic fatty liver disease |
NASH | = | nonalcoholic steatohepatitis |
NEP | = | neprilysin |
Neu-1 | = | neuraminidase-1 |
PE | = | pancreatic elastase |
PEP | = | pepsin |
PepT1 | = | peptide transporter 1 |
PPCA | = | cathepsin A/protective protein |
PR3 | = | proteinase 3 |
TE | = | tropoelastin |
Th-1 | = | type 1 T helper cell |
Timp | = | tissue inhibitor of matrix metalloproteinases |
TR | = | trypsin |
UV | = | ultraviolet |
VEGF | = | vascular epidermal growth factor |
Disclosure statement
No potential conflict of interest was reported by the author(s).
Additional information
Funding
References
- Achyuthan KE, Achyuthan AM. 2001. Comparative enzymology, biochemistry and pathophysiology of human exo-alpha-sialidases (neuraminidases). Comp Biochem Physiol B, Biochem Mol Biol. 129(1):29–64.
- Akagawa M, Suyama K. 2000. Mechanism of formation of elastin crosslinks. Connect Tissue Res. 41(2):131–141.
- Alves MF, Puzer L, Cotrin SS, Juliano MA, Juliano L, Bromme D, Carmona AK. 2003. S3 to S3' subsite specificity of recombinant human cathepsin K and development of selective internally quenched fluorescent substrates. Biochem J. 373(3):981–986.
- Antonicelli F, Bellon G, Debelle L, Hornebeck W. 2007. Elastin-elastases and inflamm-aging. Curr Top Dev Biol. 79:99–155.
- Asagiri M, Takayanagi H. 2007. The molecular understanding of osteoclast differentiation. Bone. 40(2):251–264.
- Atley LM, Mort JS, Lalumiere M, Eyre DR. 2000. Proteolysis of human bone collagen by cathepsin K: characterization of the cleavage sites generating by cross-linked N-telopeptide neoepitope. Bone. 26(3):241–247.
- Baud S, Duca L, Bochicchio B, Brassart B, Belloy N, Pepe A, Dauchez M, Martiny L, Debelle L. 2013. Elastin peptides in aging and pathological conditions. Biomol Concepts. 4(1):65–76.
- Bauer CA, Thompson RC, Blout ER. 1976. The active centers of Streptomyces griseus protease 3, alpha-chymotrypsin, and elastase: enzyme-substrate interactions close to the scissile bond. Biochemistry. 15(6):1296–1299.
- Bax DV, Rodgers UR, Bilek MM, Weiss AS. 2009. Cell adhesion to tropoelastin is mediated via the C-terminal GRKRK motif and integrin alphaVbeta3. J Biol Chem. 284(42):28616–28623.
- Bhutia YD, Ganapathy V. 2018. Protein digestion and absorption. In: Said HM, editor. Physiology of the gastrointestinal tract. Cambridge, Massachusetts, United States: Academic Press; p. 1063–1086.
- Bieth JG. 1986. Elastases: catalytic and biological properties. In: Mecham RP, editor. Regulation of matrix accumulation. Orlando, FL: Academic Press, Inc; p. 217–320.
- Biniossek ML, Nagler DK, Becker-Pauly C, Schilling O. 2011. Proteomic identification of protease cleavage sites characterizes prime and non-prime specificity of cysteine cathepsins B, L, and S. J Proteome Res. 10(12):5363–5373.
- Bisaccia F, Morelli MA, De Biasi M, Traniello S, Spisani S, Tamburro AM. 1994. Migration of monocytes in the presence of elastolytic fragments of elastin and in synthetic derivates. Structure-activity relationships. Int J Pept Protein Res. 44(4):332–341.
- Blaise S, Romier B, Kawecki C, Ghirardi M, Rabenoelina F, Baud S, Duca L, Maurice P, Heinz A, Schmelzer CE, et al. 2013. Elastin-derived peptides are new regulators of insulin resistance development in mice. Diabetes. 62(11):3807–3816.
- Blood CH, Sasse J, Brodt P, Zetter BR. 1988. Identification of a tumor cell receptor for VGVAPG, an elastin-derived chemotactic peptide. J Cell Biol. 107(5):1987–1993.
- Blood CH, Zetter BR. 1989. Membrane-bound protein kinase C modulates receptor affinity and chemotactic responsiveness of Lewis lung carcinoma sublines to an elastin-derived peptide. J Biol Chem. 264(18):10614–10620.
- Boudier C, Holle C, Bieth JG. 1981. Stimulation of the elastolytic activity of leukocyte elastase by leukocyte cathepsin G. J Biol Chem. 256(20):10256–10258.
- Brassart B, Fuchs P, Huet E, Alix AJ, Wallach J, Tamburro AM, Delacoux F, Haye B, Emonard H, Hornebeck W, et al. 2001. Conformational dependence of collagenase (matrix metalloproteinase-1) up-regulation by elastin peptides in cultured fibroblasts. J Biol Chem. 276(7):5222–5227.
- Braverman IM, Fonferko E. 1982. Studies in cutaneous aging: I. The elastic fiber network. J Invest Dermatol. 78(5):434–443.
- Bresalier RS, Byrd JC, Wang L, Raz A. 1996. Colon cancer mucin: a new ligand for the beta-galactoside-binding protein galectin-3. Cancer Res. 56(19):4354–4357.
- Bromme D, Li Z, Barnes M, Mehler E. 1999. Human cathepsin V functional expression, tissue distribution, electrostatic surface potential, enzymatic characterization, and chromosomal localization. Biochemistry. 38(8):2377–2385.
- Bromme D, Okamoto K, Wang BB, Biroc S. 1996. Human cathepsin O2, a matrix protein-degrading cysteine protease expressed in osteoclasts. Functional expression of human cathepsin O2 in Spodoptera frugiperda and characterization of the enzyme. J Biol Chem. 271(4):2126–2132.
- Bromme D, Steinert A, Friebe S, Fittkau S, Wiederanders B, Kirschke H. 1989. The specificity of bovine spleen cathepsin S. A comparison with rat liver cathepsins L and B. Biochem J. 264(2):475–481.
- Brubaker MJ, Groutas WC, Hoidal JR, Rao NV. 1992. Human neutrophil proteinase 3: mapping of the substrate binding site using peptidyl thiobenzyl esters. Biochem Biophys Res Commun. 188(3):1318–1324.
- Caenazzo C, Spina M, Onisto M, Fastelli G, Gotte L, Agostini C, Semenzato G, Garbisa S. 1990. Degradation of immobilized soluble elastin by tumor cells in culture: quantitation by ELISA. Int J Cancer. 46(3):552–558.
- Callahan JW. 1999. Molecular basis of GM1 gangliosidosis and Morquio disease, type B. Structure-function studies of lysosomal beta-galactosidase and the non-lysosomal beta-galactosidase-like protein. Biochim Biophys Acta. 1455(2–3):85–103.
- Cantarelli B, Duca L, Blanchevoye C, Poitevin S, Martiny L, Debelle L. 2009. Elastin peptides antagonize ceramide-induced apoptosis. FEBS Lett. 583(14):2385–2391.
- Castiglione Morelli MA, Bisaccia F, Spisani S, De Biasi M, Traniello S, Tamburro AM. 1997. Structure-activity relationships for some elastin-derived peptide chemoattractants. J Pept Res. 49(6):492–499.
- Choudhury N, Bouchot O, Rouleau L, Tremblay D, Cartier R, Butany J, Mongrain R, Leask RL. 2009. Local mechanical and structural properties of healthy and diseased human ascending aorta tissue. Cardiovasc Pathol. 18(2):83–91.
- Collins JF, Thoman PJ, Shaw SL, Fine R. 1985. Studies on the elastolytic activity of chymotrypsin. Connect Tissue Res. 13(4):291–298.
- Coquerel B, Poyer F, Torossian F, Dulong V, Bellon G, Dubus I, Reber A, Vannier JP. 2009. Elastin-derived peptides: matrikines critical for glioblastoma cell aggressiveness in a 3-D system. Glia. 57(16):1716–1726.
- Cutler RG, Mattson MP. 2001. Sphingomyelin and ceramide as regulators of development and lifespan. Mech Ageing Dev. 122(9):895–908.
- Davis EC, Mecham RP. 1998. Intracellular trafficking of tropoelastin. Matrix Biol. 17(4):245–254.
- Debelle L, Tamburro AM. 1999. Elastin: molecular description and function. Int J Biochem Cell Biol. 31(2):261–272.
- Debret R, Antonicelli F, Theill A, Hornebeck W, Bernard P, Guenounou M, Le Naour R. 2005. Elastin-derived peptides induce a T-helper type 1 polarization of human blood lymphocytes. Arterioscler Thromb Vasc Biol. 25(7):1353–1358.
- Debret R, Le Naour RR, Sallenave JM, Deshorgue A, Hornebeck WG, Guenounou M, Bernard P, Antonicelli FD. 2006. Elastin fragments induce IL-1beta upregulation via NF-kappaB pathway in melanoma cells. J Invest Dermatol. 126(8):1860–1868.
- Desforges M, Harris LK, Aplin JD. 2015. Elastin-derived peptides stimulate trophoblast migration and invasion: a positive feedback loop to enhance spiral artery remodelling. Mol Hum Reprod. 21(1):95–104.
- Donet M, Brassart-Pasco S, Salesse S, Maquart FX, Brassart B. 2014. Elastin peptides regulate HT-1080 fibrosarcoma cell migration and invasion through an Hsp90-dependent mechanism. Br J Cancer. 111(1):139–148.
- Duca L, Blaise S, Romier B, Laffargue M, Gayral S, El Btaouri H, Kawecki C, Guillot A, Martiny L, Debelle L, et al. 2016. Matrix ageing and vascular impacts: focus on elastin fragmentation. Cardiovasc Res. 110(3):298–308.
- Duca L, Blanchevoye C, Cantarelli B, Ghoneim C, Dedieu S, Delacoux F, Hornebeck W, Hinek A, Martiny L, Debelle L. 2007. The elastin receptor complex transduces signals through the catalytic activity of its Neu-1 subunit. J Biol Chem. 282(17):12484–12491.
- Duca L, Debelle L, Debret R, Antonicelli F, Hornebeck W, Haye B. 2002. The elastin peptides-mediated induction of pro-collagenase-1 production by human fibroblasts involves activation of MEK/ERK pathway via PKA- and PI(3)K-dependent signaling. FEBS Lett. 524(1–3):193–198.
- Duca L, Floquet N, Alix AJ, Haye B, Debelle L. 2004. Elastin as a matrikine. Crit Rev Oncol Hematol. 49(3):235–244.
- Duca L, Lambert E, Debret R, Rothhut B, Blanchevoye C, Delacoux F, Hornebeck W, Martiny L, Debelle L. 2005. Elastin peptides activate extracellular signal-regulated kinase 1/2 via a Ras-independent mechanism requiring both p110gamma/Raf-1 and protein kinase A/B-Raf signaling in human skin fibroblasts. Mol Pharmacol. 67(4):1315–1324.
- Duffy MJ. 1996. Proteases as prognostic markers in cancer. Clin Cancer Res. 2(4):613–618.
- Dyksterhuis LB, Baldock C, Lammie D, Wess TJ, Weiss AS. 2007. Domains 17-27 of tropoelastin contain key regions of contact for coacervation and contain an unusual turn-containing crosslinking domain. Matrix Biol. 26(2):125–135.
- Eckersley A, Ozols M, O’Cualain R, Keevill E-J, Foster A, Pilkington S, Knight D, Griffiths CEM, Watson REB, Sherratt MJ. 2020. Proteomic fingerprints of damage in extracellular matrix assemblies. Matrix Biol Plus. 5:100027.
- Elkington PT, Friedland JS. 2006. Matrix metalloproteinases in destructive pulmonary pathology. Thorax. 61(3):259–266.
- Eyre DR, Paz MA, Gallop PM. 1984. Cross-linking in collagen and elastin. Annu Rev Biochem. 53:717–748.
- Fahem A, Robinet A, Cauchard JH, Duca L, Soula-Rothhut M, Rothhut B, Soria C, Guenounou M, Hornebeck W, Bellon G. 2008. Elastokine-mediated up-regulation of MT1-MMP is triggered by nitric oxide in endothelial cells. Int J Biochem Cell Biol. 40(8):1581–1596.
- Faury G, Garnier S, Weiss AS, Wallach J, Fülöp T, Jacob M-P, Mecham RP, Robert L, Verdetti J. 1998. Action of tropoelastin and synthetic elastin sequences on vascular tone and on free Ca2+ level in human vascular endothelial cells. Circ Res. 82(3):328–226.
- Faury G, Ristori MT, Verdetti J, Jacob MP, Robert L. 1995. Effect of elastin peptides on vascular tone. J Vasc Res. 32(2):112–119.
- Fei YJ, Kanai Y, Nussberger S, Ganapathy V, Leibach FH, Romero MF, Singh SK, Boron WF, Hediger MA. 1994. Expression cloning of a mammalian proton-coupled oligopeptide transporter. Nature. 368(6471):563–566.
- Floquet N, Hery-Huynh S, Dauchez M, Derreumaux P, Tamburro AM, Alix AJ. 2004. Structural characterization of VGVAPG, an elastin-derived peptide. Biopolymers. 76(3):266–280.
- Fontana L, Zhao E, Amir M, Dong H, Tanaka K, Czaja MJ. 2013. Aging promotes the development of diet-induced murine steatohepatitis but not steatosis. Hepatology. 57(3):995–1004.
- Fuchs P, Debelle L, Alix A. 2001. Structural study of some specific elastin hexapeptides activating MMP1. J Mol Struct. 565–566:335–339.
- Fulop T, Jr., Jacob MP, Khalil A, Wallach J, Robert L. 1998. Biological effects of elastin peptides. Pathol Biol. 46(7):497–506.
- Gayral S, Garnotel R, Castaing-Berthou A, Blaise S, Fougerat A, Berge E, Montheil A, Malet N, Wymann MP, Maurice P, et al. 2014. Elastin-derived peptides potentiate atherosclerosis through the immune Neu1-PI3Kγ pathway. Cardiovasc Res. 102(1):118–127.
- Gehrmann M, Briknarova K, Banyai L, Patthy L, Llinas M. 2002. The col-1 module of human matrix metalloproteinase-2 (MMP-2): structural/functional relatedness between gelatin-binding fibronectin type II modules and lysine-binding kringle domains. Biol Chem. 383(1):137–148.
- Getie M, Schmelzer CEH, Neubert R. 2005b. Characterization of peptides resulting from digestion of human skin elastin with elastase. Proteins. 61(3):649–657.
- Getie M, Schmelzer CEH, Weiss AS, Neubert R. 2005. Complementary mass spectrometric techniques to achieve complete sequence coverage of recombinant human tropoelastin. Rapid Commun Mass Spectrom. 19(20):2989–2993.
- Gorisse L, Pietrement C, Vuiblet V, Schmelzer CE, Kohler M, Duca L, Debelle L, Fornes P, Jaisson S, Gillery P. 2016. Protein carbamylation is a hallmark of aging. Proc Natl Acad Sci USA. 113(5):1191–1196.
- Green EM, Mansfield JC, Bell JS, Winlove CP. 2014. The structure and micromechanics of elastic tissue. Interface Focus. 4(2):20130058.
- Gronski TJ, Jr., Martin RL, Kobayashi DK, Walsh BC, Holman MC, Huber M, Van Wart HE, Shapiro SD. 1997. Hydrolysis of a broad spectrum of extracellular matrix proteins by human macrophage elastase. J Biol Chem. 272(18):12189–12194.
- Grosso LE, Scott M. 1993a. PGAIPG, a repeated hexapeptide of bovine tropoelastin, is a ligand for the 67-kDa bovine elastin receptor. Matrix. 13(2):157–164.
- Grosso LE, Scott M. 1993b. PGAIPG, a repeated hexapeptide of bovine and human tropoelastin, is chemotactic for neutrophils and Lewis lung carcinoma cells. Arch Biochem Biophys. 305(2):401–404.
- Groult V, Hornebeck W, Ferrari P, Tixier JM, Robert L, Jacob MP. 1991. Mechanisms of interaction between human skin fibroblasts and elastin: differences between elastin fibres and derived peptides. Cell Biochem Funct. 9(3):171–182.
- Gunda V, Verma RK, Sudhakar YA. 2013. Inhibition of elastin peptide-mediated angiogenic signaling mechanism(s) in choroidal endothelial cells by the α6(IV)NC1 collagen fragment. Invest Ophthalmol Vis Sci. 54(13):7828–7835.
- Gupta N, Hixson KK, Culley DE, Smith RD, Pevzner PA. 2010. Analyzing protease specificity and detecting in vivo proteolytic events using tandem mass spectrometry. Proteomics. 10(15):2833–2844.
- Hance KA, Tataria M, Ziporin SJ, Lee JK, Thompson RW. 2002. Monocyte chemotactic activity in human abdominal aortic aneurysms: role of elastin degradation peptides and the 67-kD cell surface elastin receptor. J Vasc Surg. 35(2):254–261.
- Hauck M, Seres I, Kiss I, Saulnier J, Mohacsi A, Wallach J, Fulop T. Jr. 1995. Effects of synthesized elastin peptides on human leukocytes. Biochem Mol Biol Int. 37(1):45–55.
- Heinz A, Jung MC, Duca L, Sippl W, Taddese S, Ihling C, Rusciani A, Jahreis G, Weiss AS, Neubert RHH, et al. 2010. Degradation of tropoelastin by matrix metalloproteinases - cleavage site specificities and release of matrikines. Febs J. 277(8):1939–1956.
- Heinz A, Jung MC, Jahreis G, Rusciani A, Duca L, Debelle L, Weiss AS, Neubert RHH, Schmelzer C. 2012. The action of neutrophil serine proteases on elastin and its precursor. Biochimie. 94(1):192–202.
- Heinz A, Schräder CU, Baud S, Keeley FW, Mithieux SM, Weiss AS, Neubert RHH, Schmelzer C. 2014. Molecular-level characterization of elastin-like constructs and human aortic elastin. Matrix Biol. 38:12–21.
- Heinz A, Taddese S, Sippl W, Neubert RHH, Schmelzer C. 2011. Insights into the degradation of human elastin by matrilysin-1. Biochimie. 93(2):187–194.
- Hibbert SA, Watson REB, Griffiths CEM, Gibbs NK, Sherratt MJ. 2019. Selective proteolysis by matrix metalloproteinases of photo-oxidised dermal extracellular matrix proteins. Cell Signal. 54:191–199.
- Hinek A, Jung S, Rutka JT. 1999. Cell surface aggregation of elastin receptor molecules caused by suramin amplified signals leading to proliferation of human glioma cells. Acta Neuropathol. 97(4):399–407.
- Hinek A, Pshezhetsky AV, von Itzstein M, Starcher B. 2006. Lysosomal sialidase (neuraminidase-1) is targeted to the cell surface in a multiprotein complex that facilitates elastic fiber assembly. J Biol Chem. 281(6):3698–3710.
- Hinek A. 1995. The 67 kDa spliced variant of beta-galactosidase serves as a reusable protective chaperone for tropoelastin. Ciba Found Symp. 192:185–196.
- Hinek A. 1996. Biological roles of the non-integrin elastin/laminin receptor. Biol Chem. 377(7–8):471–480.
- Hinek A, Rabinovitch M. 1994. 67-kD elastin-binding protein is a protective “companion” of extracellular insoluble elastin and intracellular tropoelastin. J Cell Biol. 126(2):563–574.
- Hinek A, Rabinovitch M, Keeley F, Okamura-Oho Y, Callahan J. 1993. The 67-kD elastin/laminin-binding protein is related to an enzymatically inactive, alternatively spliced form of beta-galactosidase. J Clin Invest. 91(3):1198–1205.
- Hinek A, Wrenn DS, Mecham RP, Barondes SH. 1988. The elastin receptor: a galactoside-binding protein. Science. 239(4847):1539–1541.
- Hofmann UB, Westphal JR, Van Muijen GN, Ruiter DJ. 2000. Matrix metalloproteinases in human melanoma. J Invest Dermatol. 115(3):337–344.
- Hornebeck W, Adnet JJ, Robert L. 1978. Age dependent variation of elastin and elastase in aorta and human breast cancers. Exp Gerontol. 13(5):293–298.
- Hornebeck W, Tixier JM, Robert L. 1986. Inducible adhesion of mesenchymal cells to elastic fibers: elastonectin. Proc Natl Acad Sci USA. 83(15):5517–5520.
- Houghton AM. 2015. Matrix metalloproteinases in destructive lung disease. Matrix Biol 44. 46:167–174.
- Houghton AM, Quintero PA, Perkins DL, Kobayashi DK, Kelley DG, Marconcini LA, Mecham RP, Senior RM, Shapiro SD. 2006. Elastin fragments drive disease progression in a murine model of emphysema. J Clin Invest. 116(3):753–759.
- Huet E, Brassart B, Cauchard JH, Debelle L, Birembaut P, Wallach J, Emonard H, Polette M, Hornebeck W. 2002. Cumulative influence of elastin peptides and plasminogen on matrix metalloproteinase activation and type I collagen invasion by HT-1080 fibrosarcoma cells. Clin Exp Metastasis. 19(2):107–117.
- Huet E, Brassart B, Wallach J, Debelle L, Haye B, Emonard H, Hornebeck W. 2001. [Effect of elastin peptides on the production of matrix metalloproteinase 2 by human skin fibroblasts in culture]. J Soc Biol. 195(2):165–172. French.
- Imai K, Ohuchi E, Aoki T, Nomura H, Fujii Y, Sato H, Seiki M, Okada Y. 1996. Membrane-type matrix metalloproteinase 1 is a gelatinolytic enzyme and is secreted in a complex with tissue inhibitor of metalloproteinases 2. Cancer Res. 56(12):2707–2710.
- Jacob MP, Hornebeck W. 1985. Isolation and characterization of insoluble and kappa − elastins. Front Matrix Biol. 10:92–129.
- Jensen SA, Vrhovski B, Weiss AS. 2000. Domain 26 of tropoelastin plays a dominant role in association by coacervation. J Biol Chem. 275(37):28449–28454.
- Jones PA, DeClerck YA. 1980. Destruction of extracellular matrices containing glycoproteins, elastin, and collagen by metastatic human tumor cells. Cancer Res. 40(9):3222–3227.
- Joshi R, Heinz A, Fan Q, Guo S, Monia B, Schmelzer CEH, Weiss AS, Batie M, Parameshwaran H, Varisco BM. 2018. Role for cela1 in postnatal lung remodeling and alpha-1 antitrypsin-deficient emphysema. Am J Respir Cell Mol Biol. 59(2):167–178.
- Jung S, Hinek A, Tsugu A, Hubbard SL, Ackerley C, Becker LE, Rutka JT. 1999. Astrocytoma cell interaction with elastin substrates: implications for astrocytoma invasive potential. Glia. 25(2):179–189.
- Jung S, Rutka JT, Hinek A. 1998. Tropoelastin and elastin degradation products promote proliferation of human astrocytoma cell lines. J Neuropathol Exp Neurol. 57(5):439–448.
- Kamoun A, Landeau JM, Godeau G, Wallach J, Duchesnay A, Pellat B, Hornebeck W. 1995. Growth stimulation of human skin fibroblasts by elastin-derived peptides. Cell Adhes Commun. 3(4):273–281.
- Karnik SK, Wythe JD, Sorensen L, Brooke BS, Urness LD, Li DY. 2003. Elastin induces myofibrillogenesis via a specific domain. VGVAPG Matrix Biol. 22(5):409–425.
- Katsuda S, Kaji T. 2003. Atherosclerosis and extracellular matrix. J Atheroscler Thromb. 10(5):267–274.
- Kirschke H, Wiederanders B. 1994. Cathepsin S and related lysosomal endopeptidases. Meth Enzymol. 244:500–511.
- Koehl C, Knight CG, Bieth JG. 2003. Compared Action of Neutrophil Proteinase 3 and Elastase on Model Substrates. Favorable effect of S’-P’ interactions on proteinase 3 catalysis. J Biol Chem. 278(15):12609–12612.
- Konigsberg W, Goldstein J, Hill RJ. 1963. The structure of human hemoglobin. VII. The digestion of the beta chain of human hemoglobin with pepsin. J Biol Chem. 238:2028–2033.
- Korkmaz B, Moreau T, Gauthier F. 2008. Neutrophil elastase, proteinase 3 and cathepsin G: physicochemical properties, activity and physiopathological functions. Biochimie. 90(2):227–242.
- Koshikawa N, Giannelli G, Cirulli V, Miyazaki K, Quaranta V. 2000. Role of cell surface metalloprotease MT1-MMP in epithelial cell migration over laminin-5. J Cell Biol. 148(3):615–624.
- Kozel BA, Wachi H, Davis EC, Mecham RP. 2003. Domains in tropoelastin that mediate elastin deposition in vitro and in vivo. J Biol Chem. 278(20):18491–18498.
- Lagente V, Le Quement C, Boichot E. 2009. Macrophage metalloelastase (MMP-12) as a target for inflammatory respiratory diseases. Expert Opin Ther Targets. 13(3):287–295.
- Lecaille F, Bromme D, Lalmanach G. 2008. Biochemical properties and regulation of cathepsin K activity. Biochimie. 90(2):208–226.
- Lecaille F, Chowdhury S, Purisima E, Bromme D, Lalmanach G. 2007. The S2 subsites of cathepsins K and L and their contribution to collagen degradation. Protein Sci. 16(4):662–670.
- Lee P, Bax DV, Bilek MM, Weiss AS. 2014. A novel cell adhesion region in tropoelastin mediates attachment to integrin αVβ5. J Biol Chem. 289(3):1467–1477.
- Lee SH, Goswami S, Grudo A, Song LZ, Bandi V, Goodnight-White S, Green L, Hacken-Bitar J, Huh J, Bakaeen F, et al. 2007. Antielastin autoimmunity in tobacco smoking-induced emphysema. Nat Med. 13(5):567–569.
- Loeven WA. 1970. Susceptibility of various kinds of elastin to elastolytic enzymes, trypsin and chymotrypsin. Clin Chim Acta. 27(3):521–533.
- Loftus IM, Thompson MM. 2002. The role of matrix metalloproteinases in vascular disease. Vasc Med. 7(2):117–133.
- Long MM, King VJ, Prasad KU, Freeman BA, Urry DW. 1989. Elastin repeat peptides as chemoattractants for bovine aortic endothelial cells. J Cell Physiol. 140(3):512–518.
- Maeda I, Mizoiri N, Briones MP, Okamoto K. 2007. Induction of macrophage migration through lactose-insensitive receptor by elastin-derived nonapeptides and their analog. J Pept Sci. 13(4):263–268.
- Magee DF. 1974. Pepsin. Med Clin North Am. 58(6):1277–1287.
- Maquart FX, Bellon G, Pasco S, Monboisse JC. 2005. Matrikines in the regulation of extracellular matrix degradation. Biochimie. 87(3–4):353–360.
- Maquart FX, Pasco S, Ramont L, Hornebeck W, Monboisse JC. 2004. An introduction to matrikines: extracellular matrix-derived peptides which regulate cell activity. Implication in tumor invasion. Crit Rev Oncol Hematol. 49(3):199–202.
- Maquart FX, Simeon A, Pasco S, Monboisse JC. 1999. Regulation of cell activity by the extracellular matrix: the concept of matrikines. J Soc Biol. 193(4–5):423–428.
- Martin BC, Warram JH, Krolewski AS, Soeldner JS, Kahn CR, Martin BC, Bergman RN. 1992. Role of glucose and insulin resistance in development of type 2 diabetes mellitus: results of a 25-year follow-up study. Lancet. 340(8825):925–929.
- Mason RW, Johnson DA, Barrett AJ, Chapman HA. 1986. Elastinolytic activity of human cathepsin L. Biochem J. 233(3):925–927.
- Maurice P, Baud S, Bocharova OV, Bocharov EV, Kuznetsov AS, Kawecki C, Bocquet O, Romier B, Gorisse L, Ghirardi M, et al. 2016. New insights into molecular organization of human neuraminidase-1: transmembrane topology and dimerization ability. Sci Rep. 6:38363.
- Maurice P, Blaise S, Gayral S, Debelle L, Laffargue M, Hornebeck W, Duca L. 2013. Elastin fragmentation and atherosclerosis progression: the elastokine concept. Trends Cardiovasc Med. 23(6):211–221.
- McLaughlin PJ, Chen Q, Horiguchi M, Starcher BC, Stanton JB, Broekelmann TJ, Marmorstein AD, McKay B, Mecham R, Nakamura T, et al. 2006. Targeted disruption of fibulin-4 abolishes elastogenesis and causes perinatal lethality in mice. Mol Cell Biol. 26(5):1700–1709.
- McRae B, Nakajima K, Travis J, Powers JC. 1980. Studies on reactivity of human leukocyte elastase, cathepsin G, and porcine pancreatic elastase toward peptides including sequences related to the reactive site of alpha 1-protease inhibitor (alpha 1-antitrypsin). Biochemistry. 19(17):3973–3978.
- Mecham RP, Broekelmann TJ, Fliszar CJ, Shapiro SD, Welgus HG, Senior RM. 1997. Elastin degradation by matrix metalloproteinases. Cleavage site specificity and mechanisms of elastolysis. J Biol Chem. 272(29):18071–18076.
- Mecham RP, Hinek A, Entwistle R, Wrenn DS, Griffin GL, Senior RM. 1989. Elastin binds to a multifunctional 67-kilodalton peripheral membrane protein. Biochemistry. 28(9):3716–3722.
- Miekus N, Luise C, Sippl W, Baczek T, Schmelzer CEH, Heinz A. 2019. MMP-14 degrades tropoelastin and elastin. Biochimie. 165:32–39.
- Mithieux SM, Weiss AS. 2005. Elastin. Adv Protein Chem. 70:437–461.
- Mochizuki S, Brassart B, Hinek A. 2002. Signaling pathways transduced through the elastin receptor facilitate proliferation of arterial smooth muscle cells. J Biol Chem. 277(47):44854–44863.
- Mora Huertas AC, Schmelzer CEH, Hoehenwarter W, Heyroth F, Heinz A. 2016. Molecular-level insights into aging processes of skin elastin. Biochimie. 128–129:163–173.
- Mora Huertas AC, Schmelzer CEH, Luise C, Sippl W, Pietzsch M, Hoehenwarter W, Heinz A. 2018. Degradation of tropoelastin and skin elastin by neprilysin. Biochimie. 146:73–78.
- Morisaki N, Moriwaki S, Sugiyama-Nakagiri Y, Haketa K, Takema Y, Imokawa G. 2010. Neprilysin is identical to skin fibroblast elastase: its role in skin aging and UV responses. J Biol Chem. 285(51):39819–39827.
- Moroy G, Alix AJP, Hery-Huynh S. 2005. Structural characterization of human elastin derived peptides containing the GXXP sequence. Biopolymers. 78(4):206–220.
- Mort JS, Buttle DJ. 1997. Cathepsin B. Int J Biochem Cell Biol. 29(5):715–720.
- Muiznieks LD, Sharpe S, Pomes R, Keeley FW. 2018. Role of liquid-liquid phase separation in assembly of elastin and other extracellular matrix proteins. J Mol Biol. 430(23):4741–4753.
- Nackman GB, Karkowski FJ, Halpern VJ, Gaetz HP, Tilson MD. 1996. Elastin degradation products induce adventitial angiogenesis in the Anidjar/Dobrin rat aneurysm model. Ann N Y Acad Sci. 800:260–262.
- Nackman GB, Karkowski FJ, Halpern VJ, Gaetz HP, Tilson MD. 1997. Elastin degradation products induce adventitial angiogenesis in the Anidjar/Dobrin rat aneurysm model. Surgery. 122(1):39–44.
- Nagase H, Fields GB. 1996. Human matrix metalloproteinase specificity studies using collagen sequence-based synthetic peptides. Biopolymers. 40(4):399–416.
- Nagase H, Woessner JF. Jr. 1999. Matrix metalloproteinases. J Biol Chem. 274(31):21491–21494.
- Nakajima H, Ezaki Y, Nagai T, Yoshioka R, Imokawa G. 2012. Epithelial-mesenchymal interaction during UVB-induced up-regulation of neutral endopeptidase. Biochem J. 443(1):297–305.
- Nakamura T. 2018. Roles of short fibulins, a family of matricellular proteins, in lung matrix assembly and disease. Matrix Biol. 73:21–33.
- Naylor EC, Watson RE, Sherratt MJ. 2011. Molecular aspects of skin ageing. Maturitas. 69(3):249–256.
- Netzel-Arnett S, Sang QX, Moore WG, Navre M, Birkedal-Hansen H, Van Wart HE. 1993. Comparative sequence specificities of human 72- and 92-kDa gelatinases (type IV collagenases) and PUMP (matrilysin). Biochemistry. 32(25):6427–6432.
- Novinec M, Grass RN, Stark WJ, Turk V, Baici A, Lenarcic B. 2007. Interaction between human cathepsins K, L, and S and elastins: mechanism of elastinolysis and inhibition by macromolecular inhibitors. J Biol Chem. 282(11):7893–7902.
- Ntayi C, Labrousse AL, Debret R, Birembaut P, Bellon G, Antonicelli F, Hornebeck W, Bernard P. 2004. Elastin-derived peptides upregulate matrix metalloproteinase-2-mediated melanoma cell invasion through elastin-binding protein. J Invest Dermatol. 122(2):256–265.
- O’Rourke MF. 2007. Arterial aging: pathophysiological principles. Vasc Med. 12(4):329–341.
- Oefner C, D’Arcy A, Hennig M, Winkler FK, Dale GE. 2000. Structure of human neutral endopeptidase (neprilysin) complexed with phosphoramidon. J Mol Biol. 296(2):341–349.
- Ohuchi E, Imai K, Fujii Y, Sato H, Seiki M, Okada Y. 1997. Membrane type 1 matrix metalloproteinase digests interstitial collagens and other extracellular matrix macromolecules. J Biol Chem. 272(4):2446–2451.
- Owen CA, Campbell EJ. 1999. The cell biology of leukocyte-mediated proteolysis. J Leukoc Biol. 65(2):137–150.
- Pandey KC, De S, Mishra PK. 2017. Role of proteases in chronic obstructive pulmonary disease. Front Pharmacol. 8:512.
- Panwar P, Hedtke T, Heinz A, Andrault PM, Hoehenwarter W, Granville DJ, Schmelzer CEH, Bromme D. 2020. Expression of elastolytic cathepsins in human skin and their involvement in age-dependent elastin degradation. Biochim Biophys Acta Gen Subj. 1864(5):129544.
- Parks WC, Pierce RA, Lee KA, Mecham RP. 1993. Elastin. In: Kleinman HK, editor. Advances in Molecular and Cell Biology. Greenwich, CT: JAI Press. p. 133–182.
- Partridge SM, Davis HF, Adair GS. 1955. The chemistry of connective tissues. 2. Soluble proteins derived from partial hydrolysis of f elastin. Biochem J. 61(1):11–21.
- Paul RG, Bailey AJ. 1996. Glycation of collagen: the basis of its central role in the late complications of ageing and diabetes. Int J Biochem Cell Biol. 28(12):1297–1310.
- Pauletti GM, Okumu FW, Borchardt RT. 1997. Effect of size and charge on the passive diffusion of peptides across Caco-2 cell monolayers via the paracellular pathway. Pharm Res. 14(2):164–168.
- Perng DW, Chen PK. 2017. The relationship between airway inflammation and exacerbation in chronic obstructive pulmonary disease. Tuberc Respir Dis. 80(4):325–335.
- Petersen E, Wagberg F, Angquist KA. 2002. Serum concentrations of elastin-derived peptides in patients with specific manifestations of atherosclerotic disease. Eur J Vasc Endovasc Surg. 24(5):440–444.
- Peterszegi G, Robert AM, Robert L. 1996. Presence of the elastin-laminin receptor on human activated lymphocytes. C R Acad Sci III. 319(9):799–803.
- Peterszegi G, Robert L. 1998. Cell death induced in lymphocytes expressing the elastin-laminin receptor by excess agonists: necrosis and apoptosis. Biomed Pharmacother. 52(9):369–377.
- Peterszegi G, Texier S, Robert L. 1999. Cell death by overload of the elastin-laminin receptor on human activated lymphocytes: protection by lactose and melibiose. Eur J Clin Invest. 29(2):166–172.
- Phinikaridou A, Lacerda S, Lavin B, Andia ME, Smith A, Saha P, Botnar RM. 2018. Tropoelastin: a novel marker for plaque progression and instability. Circ Cardiovasc Imaging. 11(8):e007303.
- Pocza P, Süli-Vargha H, Darvas Z, Falus A. 2008. Locally generated VGVAPG and VAPG elastin-derived peptides amplify melanoma invasion via the galectin-3 receptor. Int J Cancer. 122(9):1972–1980.
- Polanowska J, Krokoszynska I, Czapinska H, Watorek W, Dadlez M, Otlewski J. 1998. Specificity of human cathepsin G. Biochim Biophys Acta. 1386(1):189–198.
- Powell JT, Vine N, Crossman M. 1992. On the accumulation of D-aspartate in elastin and other proteins of the ageing aorta. Atherosclerosis. 97(2–3):201–208.
- Powers JC, Gupton BF, Harley AD, Nishino N, Whitley RJ. 1977. Specificity of porcine pancreatic elastase, human leukocyte elastase and cathepsin G. Inhibition with peptide chloromethyl ketones. Biochim Biophys Acta. 485(1):156–166.
- Privitera S, Prody CA, Callahan JW, Hinek A. 1998. The 67-kDa enzymatically inactive alternatively spliced variant of beta-galactosidase is identical to the elastin/laminin-binding protein. J Biol Chem. 273(11):6319–6326.
- Proudfoot D, Shanahan CM. 2001. Biology of calcification in vascular cells: intima versus media. Herz. 26(4):245–251.
- Punturieri A, Filippov S, Allen E, Caras I, Murray R, Reddy V, Weiss SJ. 2000. Regulation of elastinolytic cysteine proteinase activity in normal and cathepsin K-deficient human macrophages. J Exp Med. 192(6):789–799.
- Rabkin SW. 2017. The role matrix metalloproteinases in the production of aortic aneurysm. Prog Mol Biol Transl Sci. 147:239–265.
- Rao NV, Wehner NG, Marshall BC, Gray WR, Gray BH, Hoidal JR. 1991. Characterization of proteinase-3 (PR-3), a neutrophil serine proteinase. Structural and functional properties. J Biol Chem. 266(15):9540–9548.
- Robert L, Robert AM, Fulop T. 2008. Rapid increase in human life expectancy: will it soon be limited by the aging of elastin? Biogerontology. 9(2):119–133.
- Robinet A, Fahem A, Cauchard JH, Huet E, Vincent L, Lorimier S, Antonicelli F, Soria C, Crepin M, Hornebeck W, et al. 2005. Elastin-derived peptides enhance angiogenesis by promoting endothelial cell migration and tubulogenesis through upregulation of MT1-MMP. J Cell Sci. 118(2):343–356.
- Rodgers UR, Weiss AS. 2005. Cellular interactions with elastin. Pathol Biol. 53(7):390–398.
- Romier B, Ivaldi C, Sartelet H, Heinz A, Schmelzer CEH, Garnotel R, Guillot A, Jonquet J, Bertin E, Gueant JL, et al. 2018. Production of elastin-derived peptides contributes to the development of nonalcoholic steatohepatitis. Diabetes. 67(8):1604–1615.
- Rusciani A, Duca L, Sartelet H, Chatron-Colliet A, Bobichon H, Ploton D, Le Naour R, Blaise S, Martiny L, Debelle L. 2010. Elastin peptides signaling relies on neuraminidase-1-dependent lactosylceramide generation. PloS One. 5(11):e14010.
- Satake M, Enjoh M, Nakamura Y, Takano T, Kawamura Y, Arai S, Shimizu M. 2002. Transepithelial transport of the bioactive tripeptide, val-pro-pro, in human intestinal Caco-2 cell monolayers. Biosci Biotechnol Biochem. 66(2):378–384.
- Sato H, Takino T, Okada Y, Cao J, Shinagawa A, Yamamoto E, Seiki M. 1994. A matrix metalloproteinase expressed on the surface of invasive tumour cells. Nature. 370(6484):61–65.
- Sbardella D, Fasciglione GF, Gioia M, Ciaccio C, Tundo GR, Marini S, Coletta M. 2012. Human matrix metalloproteinases: an ubiquitarian class of enzymes involved in several pathological processes. Mol Aspects Med. 33(2):119–208.
- Schmelzer CEH, Getie M, Neubert R. 2005. Mass spectrometric characterization of human skin elastin peptides produced by proteolytic digestion with pepsin and thermitase. J Chromatogr A. 1083(1–2):120–126.
- Schmelzer CEH, Jung MC, Wohlrab J, Neubert RHH, Heinz A. 2012. Does human leukocyte elastase degrade intact skin elastin? Febs J. 279(22):4191–4200.
- Schmelzer CEH, Schops R, Reynell L, Ulbrich-Hofmann R, Neubert RHH, Raith K. 2007. Peptic digestion of beta-casein. Time course and fate of possible bioactive peptides. J Chromatogr A. 1166(1–2):108–115.
- Seiki M, Yana I. 2003. Roles of pericellular proteolysis by membrane type-1 matrix metalloproteinase in cancer invasion and angiogenesis. Cancer Sci. 94(7):569–574.
- Senior RM, Griffin GL, Mecham RP. 1980. Chemotactic activity of elastin-derived peptides. J Clin Invest. 66(4):859–862.
- Senior RM, Griffin GL, Mecham RP. 1982. Chemotactic responses of fibroblasts to tropoelastin and elastin-derived peptides. J Clin Invest. 70(3):614–618.
- Senior RM, Griffin GL, Mecham RP, Wrenn DS, Prasad KU, Urry DW. 1984. Val-Gly-Val-Ala-Pro-Gly, a repeating peptide in elastin, is chemotactic for fibroblasts and monocytes. J Cell Biol. 99(3):870–874.
- Sexton T, Hitchcook LJ, Rodgers DW, Bradley LH, Hersh LB. 2012. Active site mutations change the cleavage specificity of neprilysin. PloS One. 7(2):e32343.
- Shapiro SD, Endicott SK, Province MA, Pierce JA, Campbell EJ. 1991. Marked longevity of human lung parenchymal elastic fibers deduced from prevalence of D-aspartate and nuclear weapons-related radiocarbon. J Clin Invest. 87(5):1828–1834.
- Shiratsuchi E, Ura M, Nakaba M, Maeda I, Okamoto K. 2010. Elastin peptides prepared from piscine and mammalian elastic tissues inhibit collagen-induced platelet aggregation and stimulate migration and proliferation of human skin fibroblasts. J Pept Sci. 16(11):652–658.
- Simionescu A, Philips K, Vyavahare N. 2005. Elastin-derived peptides and TGF-beta1 induce osteogenic responses in smooth muscle cells. Biochem Biophys Res Commun. 334(2):524–532.
- Sivan SS, Van El B, Merkher Y, Schmelzer CEH, Zuurmond AM, Heinz A, Wachtel E, Varga PP, Lazary A, Brayda-Bruno M, et al. 2012. Longevity of elastin in human intervertebral disc as probed by the racemization of aspartic acid. Biochim Biophys Acta. 1820(10):1671–1677.
- Spezzacatena C, Pepe A, Green LM, Sandberg LB, Bochicchio B, Tamburro AM. 2005. Synthesis, solution structure and biological activity of Val-Val-Pro-Gln, a bioactive elastin peptide. Eur J Org Chem. 2005(8):1644–1651.
- Sternlicht MD, Werb Z. 2001. How matrix metalloproteinases regulate cell behavior. Annu Rev Cell Dev Biol. 17:463–516.
- Svitkina TM, Parsons DF. 1993. Binding of some metastatic tumor cell lines to fibrous elastin and elastin peptides. Int J Cancer. 53(5):824–828.
- Szendroi M, Meimon G, Bakala H, Frances C, Robert L, Godeau G, Hornebeck W. 1984. On the presence of a metalloprotease in human skin fibroblasts that degrades the human skin elastic fiber system. J Invest Dermatol. 83(3):224–229.
- Szychowski KA, Rombel-Bryzek A, Dołhańczuk-Śródka A, Gmiński J. 2019. Antiproliferative effect of elastin-derived peptide VGVAPG on SH-SY5Y neuroblastoma cells. Neurotox Res. 36(3):503–514.
- Szychowski KA, Wójtowicz AK, Gmiński J. 2019. Impact of elastin-derived peptide VGVAPG on matrix metalloprotease-2 and -9 and the tissue inhibitor of metalloproteinase-1, -2, -3 and -4 mRNA expression in mouse cortical glial cells in vitro. Neurotox Res. 35(1):100–110.
- Taddese S, Weiss AS, Neubert RHH, Schmelzer CEH. 2008. Mapping of macrophage elastase cleavage sites in insoluble human skin elastin. Matrix Biol. 27(5):420–428.
- Taddese S, Weiss AS, Jahreis G, Neubert RH, Schmelzer CEH. 2009. In vitro degradation of human tropoelastin by MMP-12 and the generation of matrikines from domain 24. Matrix Biol. 28(2):84–91.
- Tajima S, Wachi H, Uemura Y, Okamoto K. 1997. Modulation by elastin peptide VGVAPG of cell proliferation and elastin expression in human skin fibroblasts. Arch Dermatol Res. 289(8):489–492.
- Talukdar S, Oh DY, Bandyopadhyay G, Li D, Xu J, McNelis J, Lu M, Li P, Yan Q, Zhu Y, et al. 2012. Neutrophils mediate insulin resistance in mice fed a high-fat diet through secreted elastase. Nat Med. 18(9):1407–1412.
- Tetley TD. 2002. Macrophages and the pathogenesis of COPD. Chest. 121(5):156S–159S.
- Thomson J, Singh M, Eckersley A, Cain SA, Sherratt MJ, Baldock C. 2019. Fibrillin microfibrils and elastic fibre proteins: functional interactions and extracellular regulation of growth factors. Semin Cell Dev Biol. 89:109–117.
- Timar J, Lapis K, Fulop T, Varga ZS, Tixier JM, Robert L, Hornebeck W. 1991. Interaction between elastin and tumor cell lines with different metastatic potential; in vitro and in vivo studies. J Cancer Res Clin Oncol. 117(3):232–238.
- Tiraboschi G, Jullian N, Thery V, Antonczak S, Fournie-Zaluski MC, Roques BP. 1999. A three-dimensional construction of the active site (region 507-749) of human neutral endopeptidase (EC.3.4.24.11). Protein Eng. 12(2):141–149.
- Tolosa E, Li W, Yasuda Y, Wienhold W, Denzin LK, Lautwein A, Driessen C, Schnorrer P, Weber E, Stevanovic S, et al. 2003. Cathepsin V is involved in the degradation of invariant chain in human thymus and is overexpressed in myasthenia gravis. J Clin Invest. 112(4):517–526.
- Toupance S, Brassart B, Rabenoelina F, Ghoneim C, Vallar L, Polette M, Debelle L, Birembaut P. 2012. Elastin-derived peptides increase invasive capacities of lung cancer cells by post-transcriptional regulation of MMP-2 and uPA. Clin Exp Metastasis. 29(5):511–522.
- Turk V, Stoka V, Vasiljeva O, Renko M, Sun T, Turk B, Turk D. 2012. Cysteine cathepsins: from structure, function and regulation to new frontiers. Biochim Biophys Acta. 1824(1):68–88.
- Uemura Y, Okamoto K. 1997. Elastin-derived peptide induces monocyte chemotaxis by increasing intracellular cyclic GMP level and activating cyclic GMP dependent protein kinase. Biochem Mol Biol Int. 41(1):57–64.
- Van Doren SR. 2015. Matrix metalloproteinase interactions with collagen and elastin. Matrix Biol. 44-46:224–231.
- Vierkötter A, Krutmann J. 2012. Environmental influences on skin aging and ethnic-specific manifestations. Dermatoendocrinol. 4(3):227–231.
- Vig BS, Stouch TR, Timoszyk JK, Quan Y, Wall DA, Smith RL, Faria TN. 2006. Human PEPT1 pharmacophore distinguishes between dipeptide transport and binding. J Med Chem. 49(12):3636–3644.
- Visse R, Nagase H. 2003. Matrix metalloproteinases and tissue inhibitors of metalloproteinases: structure, function, and biochemistry. Circ Res. 92(8):827–839.
- Vrhovski B, Weiss AS. 1998. Biochemistry of tropoelastin. Eur J Biochem. 258(1):1–18.
- Wachi H, Seyama Y, Yamashita S, Suganami H, Uemura Y, Okamoto K, Yamada H, Tajima S. 1995. Stimulation of cell proliferation and autoregulation of elastin expression by elastin peptide VPGVG in cultured chick vascular smooth muscle cells. FEBS Lett. 368(2):215–219.
- Wahart A, Hocine T, Albrecht C, Henry A, Sarazin T, Martiny L, El Btaouri H, Maurice P, Bennasroune A, Romier-Crouzet B, et al. 2019. Role of elastin peptides and elastin receptor complex in metabolic and cardiovascular diseases. Febs J. 286(15):2980–2993.
- Wang B, Shi GP, Yao PM, Li Z, Chapman HA, Bromme D. 1998. Human cathepsin F. Molecular cloning, functional expression, tissue localization, and enzymatic characterization. J Biol Chem. 273(48):32000–32008.
- Wang B, Sun J, Kitamoto S, Yang M, Grubb A, Chapman HA, Kalluri R, Shi GP. 2006. Cathepsin S controls angiogenesis and tumor growth via matrix-derived angiogenic factors. J Biol Chem. 281(9):6020–6029.
- Watanabe M, Sawai T, Nagura H, Suyama K. 1996. Age-related alteration of cross-linking amino acids of elastin in human aorta. Tohoku J Exp Med. 180(2):115–130.
- Werb Z, Banda MJ, McKerrow JH, Sandhaus RA. 1982. Elastases and elastin degradation. J Invest Dermatol. 79(1):154s–159s.
- Will H, Atkinson SJ, Butler GS, Smith B, Murphy G. 1996. The soluble catalytic domain of membrane type 1 matrix metalloproteinase cleaves the propeptide of progelatinase A and initiates autoproteolytic activation. Regulation by TIMP-2 and TIMP-3. J Biol Chem. 271(29):17119–17123.
- Yamamoto M, Akazawa K, Aoyagi M, Yamamoto N, Yamamoto K. 2002. Changes in elastin-binding protein in fibroblasts derived from cardinal ligaments of patients with prolapsus uteri. Cell Biol Int. 26(5):441–449.
- Yanagisawa H, Davis EC. 2010. Unraveling the mechanism of elastic fiber assembly: the roles of short fibulins. Int J Biochem Cell Biol. 42(7):1084–1093.
- Yang M, Zhang Y, Pan J, Sun J, Liu J, Libby P, Sukhova GK, Doria A, Katunuma N, Peroni OD, et al. 2007. Cathepsin L activity controls adipogenesis and glucose tolerance. Nat Cell Biol. 9(8):970–977.
- Yasuda Y, Li Z, Greenbaum D, Bogyo M, Weber E, Bromme D. 2004. Cathepsin V, a novel and potent elastolytic activity expressed in activated macrophages. J Biol Chem. 279(35):36761–36770.