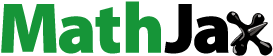
ABSTRACT
A combined volatile oil (VO) was extracted from Rosmarinus officinalis, Lavandula angustifolia and Citrus aurantium by simultaneous hydro- and steam-distillation, and encapsulated in β-cyclodextrin by co-precipitation. Response surface methodology (RSM) was used to optimize microencapsulation conditions. The variable recovered powder, VO retention degree, and inclusion efficiency were investigated based on two factors: solid-to-liquid and liquid-to-liquid (ethanol/water) ratios. The responses were influenced by the concentration of ethanol in the reaction mixture and the amount of VO used. VO release from the inclusion complexes was investigated in 10% ethanol and in 3% acetic acid. The obtained data were fitted to the first-order Korsmeyer-Peppas, Higuchi, and Peppas-Sahlin models. The Higuchi model gave the fittest approach for the VO release profile in both cases, showing that the release mechanism was controlled by Fickian diffusion.
1. Introduction
Oxidation processes and microorganism contamination play an important role in food quality loss during production, transportation, and storage, leading to spoilage and food poisoning, which have a negative worldwide impact in health and economy (Citation1). To overcome these issues, there is a pressing need for safe preservatives with high antioxidant and antimicrobial abilities. Synthetic additives were widely used to inhibit microbial growth and oxidative degradation of macromolecules such as lipids or proteins. However, these chemicals have shown several side effects, toxicity, and interaction with other additives during food processing (Citation2,Citation3); hence, there is a need for alternatives. Essential oils (EOs) and their components gained a huge interest in different fields, from pharmaceutical to agri-food industries, due to their antioxidant and antimicrobial activities (Citation4) and may constitute a response to this demand.
Rosemary (Rosmarinus officinalis L., Lamiaceae), lavender (Lavandula angustifolia L., Lamiaceae) and citrus (Citrus aurantium L., Rutaceae) EOs have been widely applied in traditional medicine and food products because of their biological properties (Citation5–7). Notwithstanding, despite their biological attributes, EOs are generally sensible to oxygen and light, being also volatile, which have limited their use in food processing (Citation8). To minimize these disadvantages, the encapsulation technology has been applied with the aim of providing the bioactive substances with a shell against external factors, such as extreme pH, light, moisture, oxygen and heat, thus promoting their stability, while forming a sustained-release material that enhances bioavailability. Moreover, the transformation of a greasy volatile oil (VO) into a microcrystalline system makes processing easier, while increasing water solubility and improving flavor and aroma (Citation9).
For food applications, the encapsulation material must be ‘generally recognized as safe’ (GRAS) (Citation10,Citation11), and several materials have been exploited for that purpose such as alginate (Citation12), chitosan (Citation13), β-cyclodextrin (β-CD) and derivatives (Citation14,Citation15), or a combination of different materials (Citation16). β-CD is a cyclic oligosaccharide composed by seven d-glucose units linked by α-d-(1→4) linkages, forming a toroidal, hollow, truncated cone structure with a hydrophobic cavity of 0.6–1.0 nm in diameter and an outer hydrophilic surface. This structure can host hydrophobic bioactive compounds by their inclusion in the centre through hydrophobic or Van der Waals interactions. These inclusion complexes (ICs) improve the hydro-solubility of insoluble guests, such as EOs (Citation9). There are several methods for encapsulating EOs into β-CD, being co-precipitation and kneading the most popular (Citation17).
Following our previous work on encapsulation of R. officinalis EO (Citation18), this study aimed to incorporate a combined (1/3:1/3:1/3 weight ratio) R. officinalis, L. angustifolia and C. aurantium volatile oil (RLC-VO), extracted by simultaneous hydro- and steam-distillation (SHSD) (Citation19), into β-CD, by co-precipitation. This method was optimized using response surface methodology (RSM), focusing on the independent parameters, solvent (ethanol/water) volume ratio and β-CD/RLC-VO mass ratio, and using recovered powder, VO retention degree and inclusion efficacy as dependent responses. Finally, determination of the chemical composition of the RLC-VO was carried out before and after encapsulation, as well as the physicochemical characterization of the ICs powders. The in vitro VO release under different conditions was also investigated, and data were fitted to four kinetic equations, to determine an appropriate model to predict the releasing mechanism of the RLC-VO from the ICs.
2. Material and methods
2.1. Plant material and simultaneous hydro- and steam-distillation (SHD)
R. officinalis, C. aurantium and L. angustifolia were harvested from the Gersif area (forest area Ait Serghouchen, Morocco), the Botanical Garden of the Faculty of Science and Technology (Fes, Morocco) and the region of Oulmas – Morocco, respectively, in flowering phase (May and June 2018). The volatile oil (VO) extraction was carried out according to the protocol described by El Kharraf et al. (Citation19), using a modified distillation apparatus. A total of one hundred gram of plants were used, combined at a ratio of 1/3:1/3:1/3 (w/w) of R. officinalis: L. angustifolia: C. aurantium. In the extractor, R. officinalis was on the perforate plate and L. angustifolia above whereas C. aurantium leaves were submerged into 1000 mL of water in the round bottom flask. The extraction lasted 3 h. The RLC-VO was stored at 4°C until further use.
2.2. Production and characterization of the inclusion complexes of RLC-VO in β-CD
The ICs of RLC-VO in β-CD were produced by co-precipitation and further characterized, as described in El-Kharraf et al. (Citation18).
X-ray powder diffraction was used to assess the changes occurring in the crystalline ordering of β-CD after the inclusion procedure. The patterns, in the range 5–40 degrees (2θ), were recorded with a Panalytical X’Pert Pro diffractometer, operating at 45 kV and 30 mA. The Cu Kα radiation was filtered by Ni and the patterns were acquired using a step size of 0.02 s and 200 s per step.
Fourier Transform Infrared (FTIR) spectra of β-CD, ICs, and the RLC-VO were recorded in a Bruker Tensor 27 spectrophotometer (Bruker Tensor 27, Billerica, MA, USA). The powders were dried in an oven at 65°C overnight, blended with KBr and pressed into pellets. FTIR spectra were acquired in the range 4000–400 cm−1. As for the VO, it was spread out between two sodium chloride windows and scanned in the range 4000–600 cm−1. In both the cases, the resolution was 4 cm−1, and 25 scans were performed.
2.3. Experimental design using response surface methodology (RSM)
The optimization of VO encapsulation parameters was performed by response surface methodology using a face-centred central composite design (FCCCD) with two independent variables: the solid to liquid (β-CD/RLC-VO) and liquid to liquid (ethanol/water) ratios and aimed to enhance the dependent variables: recovered powder, oil retention degree and inclusion efficacy, as previously reported (Citation20). The FCCD was selected among the different CCDs called the rotatable CCDs, due to its simplicity where it requires only three levels of each independent variable, and the region of operability described by variable ranges includes the full region of interest in this study. Also, it generates reduced prediction error allowing better estimation of quadratic effects in compared with the rotatable CCDs (Citation21).
The experiments were conducted in a random order according to the face-centred experimental matrix of a second-degree polynomial (Equation 1). The independent variables, real values and codes are shown in .
Table 1. Central composite design with the yield of recovered powder, inclusion efficiency, and oil retention degree.
Y: Predicted responses, β0: Constant coefficient, βi, βii, βij: Coefficients of linear, quadratic, and interaction effects, Xi, Xj: Independent variables, E: Error.
A global desirability function (D) was used to estimate the optimal conditions for the dependent variables, according to Equation 2.
: Partial desirability function of reaching the optimal response.
2.4. Release of VO from microcapsules
Two different media were used, ethanol 10% and acetic acid 3%, to estimate the ICs release profile in vitro. The selection of media was according to the EU commission regulation 10/2011 EU (Citation22,Citation23). To describe the release kinetic profile of VO in the media, four mathematical model approaches were studied: first order, Korsmeyer-Peppas, Higuchi and Peppas-Sahlin. The in vitro assay was carried out according to Costamagna et al. (Citation24) with slight modifications. An amount of 4 mg of powder was dissolved in 50 mL of 10% ethanol or 3% acetic acid solutions under continuous magnetic stirring, for 3 days. At regular time intervals, 5 mL of each medium was picked up to quantify the amount of RLC-VO released and it was immediately replaced with a fresh solution, to maintain the initial volume. The RLC-VO in each aliquot of 5 mL was extracted with 5 mL of hexane, then evaporated at 25°C and the VO was weighted. Three independent assays were performed.
The empirical model for the first-order release of the loaded compounds from water-soluble porous matrices is as shown in Equation 3 (Citation25).
C0: Initial concentration of RLC-VO, C: Amount of released RLC-VO, k: First order rate constant, t: Time.
To describe the release rate from an ointment (planar system), the following model (Equation 4) was developed by Higuchi, relating to particles dispersed in homogeneous matrices submitted to diffusing according to Fick’s law with n = 0.5 (Citation26).
Mt/M∞: Fraction of RLC-VO released.
Korsmeyer-Peppas proposed a model (Equation 5), where n plays an important role to determine the release mechanism. When the value of n is × 0.45 a Fickian diffusion mechanism operates, whilst 0.45 < n < 0.89 indicates non-Fickian transport, and n > 0.89 represents an erosion mechanism (Citation27).
The Peppas – Sahlin model was also used, using Equation 6 (Citation28).
k1: Diffusion rate kinetic constant (Fickian), k2: Erosion rate constant, m: Fickian diffusion exponent
2.5. Determination of RLC-VO from β-CD
The RLC-VO from β-CD complexes was extracted after suspending about 0.5 g of ICs in 10 ml of distilled water. The mixture was vortexed for 3 min at room temperature and the RLC-VO was obtained by a liquid–liquid extraction, using in-lab distilled n-pentane, in a ratio of 5 mL of n-pentane per volume of mixture (x 3). Pentane extracts were concentrated, at room temperature under reduced pressure on a rotary evaporator (Rotary Evaporator RE-51). Each extract was then collected in a vial and concentrated to a minimum volume (100 µL), at room temperature, under nitrogen flux, using a blow-down evaporator system. The extracted essential oils were stored at −20°C, until analysis.
2.6. Gas chromatography (GC) and Gas chromatography-mass spectrometry (GC-MS) analysis
The volatile oils were analysed by GCMS for component identification, and by GC for component quantification according to Machado et al. (Citation29) and ISO 7609 (Citation30).
2.7. Statistical analysis
The trials were performed in triplicate and the data collected from FCCCD were carried out through ANOVA for the dependent variable in triplicate to determine the model significance and suitability. Significant and high significant levels were fixed at p < 0.05, p < 0.01 and p < 0.001, the construct of the FCCD and data analysis were processed by software Nemrodw®
3. Results and discussion
3.1. Optimization of RLC-VO encapsulation in β-CD
To determine the optimal parameters for preparing RCL-VO/β-CD IC maximizing the recovered powder, inclusion efficiency, and VO retention degree, two independent variables and a factorial central composite design containing ten experimental runs were used (). The assays were run in random order to minimize the effect of unexplained variability that can be observed in the dependent variables.
The inclusion efficiency and VO retention degree capacity during co-precipitation are dependent on the size and chemical properties of guest molecules (Citation31), in this case the combined rosemary, citrus and lavender VOs. In addition, ethanol was used as co-solvent to better solubilize the VOs, since they are not water-soluble. Nevertheless, ethanol must be used in a limited concentration because high concentrations are associated to low inclusion efficiencies (Citation18). For this reason, the design variables studied were wall/core ratio (X1: β-CD/RLC-VO w/w) and solvent composition (X2: ethanol/water v/v) and their levels were selected based on literature data (Citation18,Citation20,Citation32).
Ethanol concentration as a co-solvent was fixed in three levels, the highest being 1:1, which allowed a better ICs without decreasing the inclusion efficiency, as pointed out by Goran et al. (Citation20) and Ghofran et al. (Citation33). The β-CD/RLC-VO ratio was fixed under 20% to avoid dissipation during the including process.
The central composite design used to optimize the encapsulation parameters and the subsequent responses allied to it are depicted in . The least-square method was used to estimate the regression coefficients models, linear, quadratic, and interaction terms (). According to the t-student test, both linear coefficients, X1 and X2, significantly affected powder recovery (p < 0.05) and inclusion efficiency (p < 0.001), whilst the VO retention degree was only influenced by solvent composition (p < 0.01).
Table 2. Estimated regression coefficients for the second-order model and analyses of variance (ANOVA) for the experimental data.
Indeed, reducing the ethanol amount in the reactional mixture from 50% (1:1) to 33% (1:2) had a positive effect on inclusion efficiency (), since ethanol helps to dissolve the hydrophobic terpenes. Ghofran et al. (Citation33) reported that the inclusion efficiency of thymol in β-CD could reach 96.2 ± 1.0% (w/w) at 0.5% (v/v) ethanol, revealing a strong correlation between the medium polarity and the hydrophobic interactions necessary for encapsulation. Likewise, El Kharraf et al. (Citation18) reported that an increase in ethanol concentration from 50% to 75% is reversely correlated with inclusion efficiency, with this response reaching only 19.4 mg/g at 75% versus 85.4 mg/g at 50%. Several authors reported that a high ethanol amount, ranging between 75% and 100%, might disrupt the non-covalent bonds necessary for the guest molecules to stabilize in the core/wall of cyclodextrin (Citation34), although Coleman et al. (Citation35) reported that β-CD is not soluble in 100% ethanol. López and Pascual Villalobos (Citation36) found that the inclusion efficiency of geraniol and linalool in β-CD was 34% and 31%, respectively, using water/ethanol (1:2) whilst in the same co-solvent ratio only 6% of curcumin was incorporated into β-CD (Citation37).
Table 3. Fitting response model.
Moreover, the linear parameter X2 had a positive effect on the recovered powder and the inclusion efficiency. Simultaneously, this factor reversely affected the VO retention degree at a highly significant level (p < 0.001), resulting in a decline in the amount of RLC-VO included at the cavity of β-CD, which might be due to the larger wall/core ratio when higher β-CD mass is used (Citation38). Besides, the difference between the weight of used and encapsulated RLC-VO might also result from VO evaporation during co-precipitation or just its retention in the solvent (Citation20,Citation39).
The quadratic term X12 was slightly significant on powder recovery and inclusion efficiency (p < 0.05), but moderately significant on the oil retention degree (p < 0.01), whereas X22 was moderately significant on inclusion efficiency and highly significant (p < 0.001) on oil retention degree (). Apart from powder recovery (p < 0.01), the interaction terms were highly significant, with a negative influence on the former and on VO retention degree, and a positive effect on inclusion efficiency.
Experimental data regrouped from the different combinations of independent variables adequately fitted the second-order quadratic model, according to ANOVA analysis results (), with p-values <0.001, meaning that the models are representative. The coefficients of determination, R2, were 0.983, 0.999 and 0.995 for Y1: recovered powder, Y2: VO retention degree, and Y3: inclusion efficiency, respectively, meaning that 99% of the response variations were engendered by the variation in the factors, and only 1% of total variations could not be explained by the model. The statistical parameters also suggest a good correlation between experimental and predicted Y1, Y2 and Y3 values. Moreover, the low coefficient of variation values (CV < 10%) clearly imply that those response surface models were reproducible and reliable (). The lack-of-fit was not significant compared to pure error and indicated the suitability of the models to predict the variations.
Using equations in it is possible to draw response surface plots which, being 3D representations, provide a better understanding of the interactions between the factors.
shows the interactions between ethanol concentration (v/v) and β-CD/RLC-VO (w/w) ratio, and the variables Y1: recovered powder, Y2: VO retention degree, and Y3: inclusion efficiency. shows that the recovered powder and inclusion efficiency can reach values >0.999 g and 80 mg/g, respectively, in the range between 33% and 50% for ethanol/water and [15:85] to [20:80] for β-CD/RLC-VO ratios. As can be noticed in (c,c’), the plot is elliptic, indicating that the simultaneous increase in both factors decreased the VO retention degree, reaching a minimum at 44% ethanol/water and [15:85] β-CD/RLC-VO ratios. Similar results were reported by other authors (Citation20,Citation33,Citation38–40).
Figure 1. Response surface 2D and 3D showing the interaction effects of X1 and X2 supsub1 Ethanol/water ratio (mL/mL)), supsub2 β-CD/RLC-VO ratio (g/g), respectively) on recovered powder a and a’, Inclusion Efficiency b and b’, and oil retention degree c and c’.
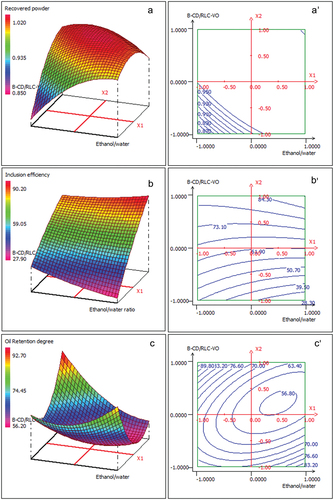
Optimization of the encapsulation process aims to enhance the quality of ICs by increasing the recovered powder, the VO retention degree, and, specially, the inclusion efficiency, by preventing any loss of VO and minimizing the un-encapsulated amount at the surface. Derringer and Suich (Citation41) methodology was used to determine the optimum composition of the independent variables, based on the experimental data collected and the results estimated by model building (). From EquationEquation 4(4)
(4) , the optimal encapsulation parameters coding values were determined to be −0.986 and −0.375 for the factors ethanol/water and β-CD/RLC-VO ratios, respectively. At those composite conditions, the desirable response values of Y1, Y2, and Y3 reached 0.93 g, 68.28% and 59.26 mg/g, respectively, within a D-optimal of 99.71%.
3.2. Morphology and particles size analysis
The morphological characteristics of β-CD/RLC-VO ICs was confirmed by SEM analysis (). The ICs were amorphous, with irregular crystals in different sizes (), which highly suggests a strong aggregation as reported by Fauziah et al. (Citation42). This may result from particles self-assembly because of lack in net charge, which agrees with many reports (Citation43,Citation44). The particles diameter ranged from 2 to 5 µm (). Similar results were previously reported for encapsulated trans-cinnamaldehyde and β-CD ICs of oregano EO (Citation45). Notwithstanding, the average diameter of aggregates formed under the experimental conditions [1:1]; [15:85] and [1:1]; [20:80] had similar diameter values (3.3 ± 1.0 µm and 3.6 ± 1.1 µm, respectively), yet they were significantly higher when compared to the ICs recovered from [1:1]; [10:90], with 2.1 ± 0.9 µm. In contrast, El Kharraf et al. (Citation18) reported that the sizes of rosemary EO ICs from the experimental condition [1:1]; [10:90] were significantly larger (2.7 ± 0.2 µm) than those estimated for ICs from the experimental condition [1:1]; [15:85], and [1:1]; [20:80] (2.1 ± 0.8 and 2.1 ± 0.9 µm). The authors suggested that those results were due to the poor binding of rosemary EO at the surface. In fact, the dissimilarity to those previous results may be explained by the richness of RLC-VO and its equal distribution between the inner and the surface of β-CD.
3.3. Fourier Transform Infrared Spectroscopy (FTIR)
RLC-VO inclusion into β-CD was confirmed by FTIR spectroscopy. The FTIR spectra of RLC-VO, β-CD and the ICs recovered from [1:1], [10:90]; [1:1], [15:85]; and [1:1], [20:80] formulations are depicted in . The spectrum of β-CD () presents a characteristic band at 3337 cm−1, corresponding to the stretching vibrations of –OH groups and a band at 2920 cm−1 due to C-H bonds stretching. The band at 1638 cm−1 is the result of O-H bending vibration of adsorbed water molecules, and the broad one at 1412 cm−1 results from the overlap of the CH2 scissoring and wagging with the O-H in plan bending bands. The intense broad band at 1028 cm−1 and the one at 1155 cm−1 might be attributed to the stretching vibrations of C-O, C-C, and C–O–C bonds. The RLC-VO spectrum () shows the characteristic broad band at 3460 cm−1 relative to O-H bond stretching, and those of CH3 and CH2 asymmetric and symmetric stretching at 2964 cm−1 and 2867 cm−1. The sharp band at 1743 cm−1 is attributed to the stretching vibrations of C=O groups, while those at 1450 cm−1 and 1375 cm−1 are associated with CH3, CH2 and O-H bending, respectively. The bands at 1236 cm−1, 1171 cm−1 and 985 cm−1 are attributed to the stretching of C-C and C-O bonds, and to the out-of-plane C-H and O-H bending, respectively. These observations are consistent with the presence of 1,8-cineole, camphor, linalool and linalyl acetate, major components of RLC-VO (). The FTIR spectra of the ICs recovered from [1:1], [10:90]; [1:1], [15:85]; and [1:1], [20:80] formulations () do not significantly differ from each other, being essentially a combination of the two previous ones, thus indicating the successful association of RLC-VO and β-CD.
Figure 3. FTIR Spectra of the Inclusion Complexes Powders Recovered from Experiments [1:1]; [20:80] a), [1:1]; [15:85] b) and [1:1]; [10:90] c), β-CD d) and RLC-VO e).
![Figure 3. FTIR Spectra of the Inclusion Complexes Powders Recovered from Experiments [1:1]; [20:80] a), [1:1]; [15:85] b) and [1:1]; [10:90] c), β-CD d) and RLC-VO e).](/cms/asset/686aa352-b209-4ecf-a820-6aba69fee9d1/tjeo_a_2185309_f0003_oc.jpg)
Table 4. Kinetics constant and correlation coefficients of the RLC-VO release profile in different the hydroalcoholic and acidic systems.
Table 5. Chemical composition of Rosmarinus officinalis, Lavandula angustifolia, and Citrus Aurantium VO and their relative contents before and after microencapsulation.
3.4. XRD analysis
Powder X-ray diffraction (XRD) is widely recognised as a useful technique to investigate the formation of inclusion complexes with cyclodextrin. Changes in the diffraction pattern of the parent cyclodextrin and, in particular, the disappearance of diffraction peaks associated to the cage-type packing structure and the appearance of peaks that are assigned to the channel-type packing have been accepted as an indication of the formation of inclusion complexes (Citation46,Citation47).
displays a XRD pattern that is typical of crystalline β-CD adopting the cage-type packing (Citation45,Citation47), with peaks at 9.12º, 10.75º, 12.57º and 22.81º among others. shows a representative XRD pattern of the samples. The sample was clearly crystalline, but the pattern indicates some significant changes when compared with the pristine β-CD. Several peaks associated to the cage-type packing vanish and new peaks are now clearly visible. Among these peaks, those at 12.03º and 17.60º have been associated to the channel-type packing of β-CD (Citation45), which is strong evidence of the formation of inclusion complexes.
3.5. In vitro release of RLC-VO from the inclusion complexes
RLC-VO release profiles from microcapsules with β-CD/RLC-VO ratios [10:90], [15:85] and [20:80] obtained in 1:1 ethanol/water that showed high inclusion efficiency and VO retention degree are depicted in . For 3 days, the released percentage in the two selected media, 10% ethanol and 3% acetic acid, was measured and exhibited two characteristic phases: a rapid release phase (burst effect) that ranged from 0 h to 8 h for both used media, during which less than 30% of the RLC-VO was released in the acidic medium and under 70% was diffused in the hydroalcoholic one, for the three ICs. After this phase, a slower and sustained release phase was noticed, with release rates increasing from 30% to 99.99% and from 70% to 99.99% for acidic and hydroalcoholic media, respectively, at the time interval between 8 h and 72 h.
Figure 5. The Release Profile of the RLC-VO in Different Media from ICs: The Inclusion Complexes Recovered Powder from Experimental (a) [10:90], (b) [15:85], and (c) [20:80].
![Figure 5. The Release Profile of the RLC-VO in Different Media from ICs: The Inclusion Complexes Recovered Powder from Experimental (a) [10:90], (b) [15:85], and (c) [20:80].](/cms/asset/1093bfa8-6049-488b-8cd5-d32efd3f10c5/tjeo_a_2185309_f0005_oc.jpg)
Interestingly, the release profiles of RLC-VO from all three ICs were similar in both media. Nevertheless, the release rate was higher in 10% ethanol, which could be explained by the fact that the ICs are more soluble in the hydroalcoholic solution than in the acidic medium. The release rate differences among the three ICs could be attributed to the inclusion efficiency, which was higher for the [20:80] sample (inclusion efficiency <80 mg/g) compared with ICs of [10:90] and [15:85], with inclusion efficiency values inferior to 50 mg/g. In addition, it was noticed a release stability between 8 and 24 h in 10% ethanol curve of the [20:80] sample, and after that new increase. Although still not fully understood, this may partially be explained by medium saturation, due to high ICs solubility and high VO release rate, that slow the dissociation rate of VO from the β-CD.
Release data were fitted to four empirical models: first order, Korsmeyer-Peppas, Higuchi, and Peppas-Sahlin. The statistical and kinetic parameters calculated are grouped in . R2adj correlation coefficient values demonstrated that Higuchi model showed the best mathematical fitting for the release data collected (0.91 < R2adj<0.99) thus indicating that Fickian diffusion is the dominant mechanism of release of RLC-VO in both media. In conclusion, the release is due to a swelling process, which begins by a washing phase of the VO from the surface, followed by powder swelling by the fluid media, which drives the VO to diffuse out during the process, without β-CD structure disruption. Similar observations have been reported by several authors (Citation45,Citation47–49).
3.6. Chemical composition of RLC-VO and β-CD-RLC-VO ICs
The percentages of volatile compounds in RLC-VO and its ICs are depicted in . The major components of RLC-VO were 1,8-cineole, camphor, linalool and linalyl acetate. β-CD selectivity mainly depends on the physicochemical properties of the guest molecules, such as size, charge, and polarity, besides external factors, as the encapsulation process, and co-solvent ratio and nature. As shown in , β-CD had a higher affinity for the oxygenated monoterpenes (81.6% in RLC-VO vs 89.2% encapsulated) than for monoterpenes (14.9% in RLC-VO vs 8.4% encapsulated). The higher relative percentages of linalool, borneol and camphor in the encapsulated VO can be explained by their high affinity to cyclodextrins, as previously reported by some authors (Citation23,Citation50). Furthermore, Donze et al. (Citation51) reported inclusion selectivity to be extremely dependent on co-solvent, and in an ethanol/water ratio of 1:1, the relative selectivity of β-CD towards terpenes was in the following order: borneol > camphor > linalool = cineole >limonene > αpinene > myrcene.
4. Conclusions
ICs of RCL-VO in β-CD were successfully produced by co-precipitation, affording a powder composed of micrometre-sized aggregates. The use of RSM allowed to optimize three important production factors, recovered powder, VO retention degree and inclusion efficiency, by adjusting ethanol/water and β-CD/RLC-VO ratios. The chemical composition of RLC-VO was preserved during the encapsulation process. The developed RCL-VO loaded microcapsules thus present a potential to be used in the pharmaceutical and food industries as an antimicrobial or antioxidant agent.
Author contribution
SEK, SEG, JPL, AMRC, AMM, CST: investigation, methodology; AF, EMEH, ACF, MGM: investigation, conceptualization, SEK: writing the original draft; JPL, AMRC, ACF, MGM: writing the final manuscript. All authors have read and approved the final manuscript.
Acknowledgments
The authors acknowledge the financial support by the Fundação para a Ciência e a Tecnologia I.P. (FCT), under projects UIDB/05183/2020; UIDB/00100/2020 and CESAM UIDP/50017/2020+UIDB/50017/2020+LA/P/0094/2020.
Disclosure statement
No potential conflict of interest was reported by the authors.
Additional information
Funding
References
- WHO/OECD, Foodborne Disease in OECD Countries: Present State and Economic Costs. OECD Publishing, Paris (2003). doi: 10.1787/9789264105386-en
- M. Viuda-Martos, M.A. Mohamady, J. Fernández-López, K.A. Abd ElRazik, E.A. Omer, J.A. Pérez-Alvarez and E. Sendra, In vitro antioxidant and antibacterial activities of essentials oils obtained from Egyptian aromatic plants. Food Control, 22(11), 1715–1722 (2011). doi: 10.1016/j.foodcont.2011.04.003.
- N. El Abed, B. Kaabi, M.I. Smaali, M. Chabbouh, K. Habibi, M. Mejri, M.N. Marzouki and S.B.H. Ahmed, Chemical composition, antioxidant and antimicrobial activities of Thymus capitata essential oil with its preservative effect against Listeria monocytogenes inoculated in minced beef meat. Evidence-Based Complementary and Alternative Medicine, 2014, 152487 (2014). doi: 10.1155/2014/152487.
- P. Tongnuanchan and S. Benjakul, Essential oils: extraction, bioactivities, and their uses for food preservation: a review. Journal of Food Science, 79(7), R1231–1249 (2014). doi: 10.1111/1750-3841.12492.
- E. Sarrou, P. Chazopoulou, K. Dimassi-Theriou and I. Therios, Volatile constituents and antioxidant activity of peel, flowers and leaf oils of Citrus aurantium L. growing in Greece. Molecules, 18(9), 10639–10647 (2013). doi: 10.3390/molecules180910639.
- A. Carrasco, V. Tomas, J. Tudela and M.G. Miguel, Comparative study of GC-MS characterization, antioxidant activity and hyaluronidase inhibition of different species of Lavandula and Thymus essential oils. Flavour and Fragrance Journal, 31(1), 57–69 (2016). doi: 10.1002/ffj.3283.
- M.V. Alvarez, L.A. Ortega-Ramirez, B.A. Silva-Espinoza, G.A. Gonzalez-Aguilar and J.F. Ayala-Zavala, Antimicrobial, antioxidant, and sensorial impacts of oregano and rosemary essential oils over broccoli florets. Journal of Food Processing and Preservation, 43(3), e13889 (2019). doi: 10.1111/jfpp.13889.
- F. Tao, L.E. Hill, Y. Peng and C.L. Gomes, Synthesis and characterization of β-cyclodextrin inclusion complexes of thymol and thyme oil for antimicrobial delivery applications. LWT - Food Science and Technology, 59(1), 247–255 (2014). doi: 10.1016/j.lwt.2014.05.037.
- C.H.M. Marques, A review on cyclodextrin encapsulation of essential oils and volatiles. Flavour and Fragrance Journal, 25(5), 313–326 (2010). doi: 10.1002/ffj.2019.
- M.R.I. Shishir, L. Xie, C. Sun, X. Zheng and W. Chen, Advances in micro and nano-encapsulation of bioactive compounds using biopolymer and lipid-based transporters. Trends in Food Science & Technology, 78, 34–60 (2018). doi: 10.1016/j.tifs.2018.05.018.
- M. Saifullah, M.R.I. Shishir, R. Ferdowsi, M.R.T. Rahman and Q. van Vuong, Micro and nano encapsulation, retention and controlled release of flavor and aroma compounds: a critical review. Trends in Food Science & Technology, 86, 230–251 (2019). doi: 10.1016/j.tifs.2019.02.030.
- M.J. Paris, N. Ramirez-Corona, E. Palou and A. Lapez-Malo, Modelling release mechanisms of cinnamon (Cinnamomum zeylanicum) essential oil encapsulated in alginate beads during vapor-phase application. Journal of Food Engineering, 282, 110024 (2020). doi: 10.1016/j.jfoodeng.2020.110024.
- M. Al-Moghazy, H. El-Sayed, H.H. Salama and A.A. Nada, Edible packaging coating of encapsulated thyme essential oil in liposomal chitosan emulsions to improve the shelf life of Karish cheese. Food Bioscience, 43, 101230 (2021). doi: 10.1016/j.fbio.2021.101230.
- C. Bai, P. Xu, W. Qi, Q. Kong, J. Wang, T. Liao and G. Xiong, Preparation and characterization of a sustained-release bio-preservative based on β-cyclodextrin encapsulated eugenol. Food Bioscience Journal, 42, 2212–4292 (2021). doi: 10.1016/j.fbio.2021.101192.
- M.D. Köse, B.N. Tekin and O. Bayraktar, Simultaneous isolation and selective encapsulation of volatile compounds from essential oil during electrospraying of β-cyclodextrin. Carbohydrate Polymers, 258, 117673 (2021). doi: 10.1016/j.carbpol.2021.117673.
- M. Atefi, K. Nayebzadeh, A. Mohammadi and A.M. Mortazavian, Using ß-cyclodextrin and Arabic Gum as Wall Materials for Encapsulation of Saffron Essential Oil. Iranian Journal of Pharmaceutical Research, 16(1), 93–102 (2017).
- G. Wadhwa, S. Kumar, L. Chhabra, S. Mahant and R. Rao, Essential oil–cyclodextrin complexes: an updated review. Journal of Inclusion Phenomena and Macrocyclic Chemistry, 89(1–2), 39–58 (2017). doi: 10.1007/s10847-017-0744-2.
- S. El-Kharraf, H. Farah, E.M. El-Hadrami, S. El-Guendouz, J.P. Lourenço, A.M.R. Costa and M.G. Miguel, Encapsulation of Rosmarinus officinalis essential oil in β-cyclodextrins. Journal of Food Processing and Preservation, 45(10), e15806 (2021). doi: 10.1111/jfpp.15806.
- S. El Kharraf, M.L. Faleiro, F. Abdellah, S. El-Guendouz, E.M. El Hadrami and M.G. Miguel, Simultaneous hydrodistillation-steam distillation of Rosmarinus officinalis, Lavandula angustifolia and Citrus aurantium from Morocco, major terpenes: impact on biological activities. Molecules, 26(18), 5452 (2021). doi: 10.3390/molecules26185452.
- M.P. Goran, S.S. Gordana and S.N. Radulović, Encapsulation of cinnamon oil in β-cyclodextrin. Journal of Medicinal Plants Research, 14(4), 1382–1390 (2010). doi: 10.5897/JMPR10.146.
- S. Beg and Z. Rahman, Central Composite Designs and Their Applications in Pharmaceutical Product Development: Beg, S. (eds). Vol. V1, pp. 64–74, Springer (2021). doi: 10.1007/978-981-33-4717-5_6
- Commission Regulation (EU) No 10/2011, Regulations: on plastic materials and articles intended to come into contact with food. Official Journal of the European Union, L12, 74–75 (2011). https://eur-lex.europa.eu/LexUriServ/LexUriServ.do?uri=OJ:L:2011:012:0001:0089:en:PDF
- C. Dima, M. Cotârleţ, B. Tiberius, G. Bahrim, P. Alexe and Ş. Dima, Encapsulation of coriander essential oil in β-cyclodextrin: antioxidant and antimicrobial properties evaluation. Romanian Biotechnological Letters, 19(2), 9128–9140 (2014).
- M.S. Costamagna, L.G. Gómez-Mascaraque, I.C. Zampini, M.R. Alberto, J. Pérez, A. López-Rubio and M.I. Isla, Microencapsulated chañar phenolics: a potential ingredient for functional foods development. Journal of Functional Foods, 37, 523–530 (2017). doi: 10.1016/j.jff.2017.08.018.
- P. Costa and J.M.S. Lobo, Modeling and comparison of dissolution profiles. European Journal of Pharmaceutical Sciences, 13(2), 123–133 (2001). doi: 10.1016/S0928-0987(01)00095-1.
- G. Singhvi and M. Singh, In vitro drug release characterization models: a Review. International Journal of Pharmaceutical Studies and Research, 2, 77–84 (2011).
- S. Dash, P.N. Murthy, L. Nath and P. Chowdhury, Kinetic modeling on drug release from controlled drug delivery systems. Acta Poloniae Pharmaceutica - Drug Research, 67, 217–223 (2010).
- J.M. Unagolla and A.C. Jayasuriya, Drug transport mechanisms and in vitro release kinetics of vancomycin encapsulated chitosan-alginate polyelectrolyte microparticles as a controlled drug delivery system. European Journal of Pharmaceutical Sciences, 114, 199–209 (2018). doi: 10.1016/j.ejps.2017.12.012.
- A.M. Machado, M. Antunes, M.G. Miguel, M. Vilas-Boas and A.C. Figueiredo, Volatile profile of Portuguese monofloral honeys: significance in botanical origin determination. Molecules, 26(16), 4970 (2021). doi: 10.3390/molecules26164970.
- ISO 7609, Essential oils - analysis by gas chromatography on capillary columns - general method.
- J. Hogenbom, A. Jones, H.V. Wang, L.J. Pickett and N. Faraone, Synthesis and characterization of β-cyclodextrin-essential oil inclusion complexes for tick repellent development. Polymers, 13(11), 1892 (2021). doi: 10.3390/polym13111892.
- B.R. Bhandari, B.R. D’arc and L.L. Thi Bich, Lemon oil to β-cyclodextrin ratio effect on the inclusion efficiency of β-cyclodextrin and the retention of oil volatiles in the complex. Journal of Agricultural and Food Chemistry, 46(4), 1494–1499 (1998). doi: 10.1021/jf970605n.
- A.N. Ghofran, M.J. Cran, A.J. Smallridge and S.W. Bigger, Optimisation of β-cyclodextrin inclusion complexes with natural antimicrobial agents: thymol, carvacrol and linalool. Journal of Microencapsulation, 35(1), 26–35 (2017). doi: 10.1080/02652048.2017.1413147.
- P. Kalathenos and N.J. Russell, Ethanol as a Food Preservative. Food Preservatives. Springer, Boston (2003). doi: 10.1007/978-0-387-30042-9_10
- A.W. Coleman, M. Munoz, A.K. Chatjigakis and P. Cardot, Classification of the solubility behaviour of β-cyclodextrin in aqueous–co-solvent mixtures. Journal of Physical Organic Chemistry, 6(12), 651–659 (1993). doi: 10.1002/poc.610061203.
- M.D. López and M.J. Pascual-Villalobos, Analysis of monoterpenoids in inclusion complexes with β -cyclodextrin and study on ratio effect in these microcapsules. Julius-Kühn-Archiv, 425, 705–709 (2010).
- E.I. Paramera, S.J. Konteles and V.T. Karathanos, Stability and release properties of curcumin encapsulated in Saccharomyces cerevisiae, β-cyclodextrin and modified starch. Food Chemistry, 125(3), 913–922 (2011). doi: 10.1016/j.foodchem.2010.09.071.
- J. Ning and S. Yue, Optimization of preparation conditions of Eucalyptus essential oil microcapsules by response surface methodology. Journal of Food Processing and Preservation, 43(11), e14188 (2019). doi: 10.1111/jfpp.14188_2019.
- Y. Wang, C. Yin, X. Cheng, G. Li, Y. Shan and X. Zhu, β-Cyclodextrin inclusion complex containing Litsea cubeba essential oil: preparation, optimization, physicochemical, and antifungal characterization. Coatings, 10(9), 850 (2020). doi: 10.3390/coatings10090850.
- S. Ma, Z. Zhao and P. Liu, Optimization of preparation process of β-cyclodextrin inclusion compound of clove essential oil and evaluation of heat stability and antioxidant activities in vitro. Journal of Food Measurement and Characterization, 12(3), 2057–2067 (2018). doi: 10.1007/s11694-018-9820-6.
- G. Derringer and R. Suich, Simultaneous optimization of several response variables. Journal of Quality Technology, 12(4), 214–219 (1980). doi: 10.1080/00224065.1980.11980968.
- C.I. Fauziah, A.H. Zaibunnisa, H. Osman and W.A.W. Mustapha, Thermal analysis and surface morphology study of cholesterol: β-cyclodextrin inclusion complex. Advanced Materials Research, 812, 221–225 (2013). doi: 10.4028/scientific.net/AMR.812.221.
- E.-J. Seo, S.-G. Min and M.J. Choi, Release characteristics of freeze-dried eugenol encapsulated with β-cyclodextrin by molecular inclusion method. Journal of Microencapsulation, 27(6), 496–505 (2010). doi: 10.3109/02652041003681398.
- W. Zhang, X. Li, T. Yu, L. Yuan, G. Rao, D. Li and C. Mu, Preparation, physicochemical characterization, and release behavior of the inclusion complex of trans-anethole and β-cyclodextrin. Food Research International, 74, 55–62 (2015). doi: 10.1016/j.foodres.2015.04.029.
- F. Kayaci, H. Sen, H. Durgun and T. Uyar, Functional electrospun polymeric nanofibers incorporating geraniol–cyclodextrin inclusion complexes: high thermal stability and enhanced durability of geraniol. Food Research International, 62, 424–431 (2014). doi: 10.1016/j.foodres.2014.03.033.
- A. Harada, M. Okada, J. Li and M. Kamachi, Preparation and characterization of inclusion complexes of poly(propylene glycol)with cyclodextrins. Macromolecules, 28(24), 8406–8411 (1995). doi: 10.1021/ma00128a060.
- P. Surathi and V.M. Karbhari, Hygrothermal Effects on Durability and Moisture Kinetics of Fiber-Reinforced, 1st edn. University of California, San Diego, CA, USA (2006).
- T. Higuchi, Mechanism of sustained-action medication theoretical analysis of rate of release of solid drugs dispersed in solid matrices. Journal of Pharmaceutical Sciences, 52(12), 1145–1149 (1963). doi: 10.1002/jps.2600521210.
- M. Lis, Ó. García Carmona, C. García Carmona and F. Maestá Bezerra, Inclusion complexes of citronella oil with β-cyclodextrin for controlled release in biofunctional textiles. Polymers, 10(12), 1324 (2018). doi: 10.3390/polym10121324.
- R.L. Abarca, J. Rodríguez Francisco, A. Guarda, J. Galotto María and E. Bruna Julio, Characterization of β-cyclodextrin inclusion complexes containing an essential oil component. Food Chemistry, 196, 968–975 (2016). doi: 10.1016/j.foodchem.2015.10.023.
- C. Donze and A.W. Coleman, β-CD inclusion complexes: relative selectivity of terpene and aromatic guest molecules studied by competitive inclusion experiments. Journal of Inclusion Phenomena and Macrocyclic Chemistry, 16(1), 1–15 (1993). doi: 10.1007/bf00708758.