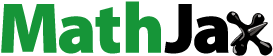
Abstract
Here we study the roughening behavior, scaling properties and the wetting nature of the Silicon surfaces that have been irradiated with 3 keV Ar ions. These surfaces illustrate the formation of nanostructures and ripples, which evolve at higher fluences. The scaling studies have been applied to understand the evolution of the surfaces. Height- Height correlation method has been utilized to extract important scaling parameters, and the derived Hurst exponents (H) indicate these surfaces to be of self-affine type. The derived correlation lengths (ξ) reflect long ranged correlations on the surface and evolution of the nanostructures. The nano-patterned surfaces show near hydrophobic behavior, which gets further enhanced with increasing irradiation.
1. Introduction
Silicon-based nanomaterials demonstrate numerous technological benefits with wide range of applications in the fields of energy, environment, biology and biomedicine (Citation1, Citation2). Advantages of excellent electronic and mechanical properties added to well-developed silicon technology have assisted these nanostructures in becoming excellent candidates for the development of high performance sensors (Citation3, Citation4). Nanostructured silicon-based sensors are extensively utilized in the detection of biological targets like DNA (Citation5), proteins (Citation6), virus (Citation7) as well as for analyte–receptor binding (Citation8). The adhesive properties of Si devices are guided by the roughness and surface chemistry which play crucial role in many disciplines of biological cell integration and development of biomimetic materials for tissue engineering, etc. (Citation9). For these applications, monitoring and understanding the properties of the surfaces, like its roughness characteristics and wetting behavior are essential. Wetting phenomenon is ubiquitous to nature with widespread technological implications and the importance of Si surfaces stems from their omnipresence in most silicon-based wetting explorations.
Real surfaces are always characterized through roughness which depends on the fabrication process and existence of adsorbed species. Many surfaces in nature, e.g. surfaces prepared by ion irradiation, vapor deposition of metal films, fractures (crack propagation), corrosion or wear of surfaces, and many other natural surfaces are described by roughness with self-affine properties (Citation10). Under the theory of kinetic roughening, a flat surface essentially grows and roughens with time, evolving under varying scenarios (Citation11, Citation12). The dynamic scaling hypothesis by Family-Vicsek shows that root mean square (rms) roughness, σ, of such growing surfaces demonstrate scaling behavior (Citation13, Citation14) and the exponents describe the evolution process. Understanding surface evolution after ion bombardment is a complex process due to the involvement of several components like ion beam parameters, surface properties, etc. (Citation15, Citation16). In this respect, scaling parameters like roughness exponents or Hurst exponents H, obtained via height–height correlation method provide essential salient characteristics of the surface evolution, as well an understanding on the self-affine nature of the surface (Citation14).
Rough surfaces exhibit fluctuations in height h() at position
, relative to a smooth mean height value, and are characterized by a root mean square roughness σ. For an isotropic rough surface, the height–height correlation (HHC) function, G
, is represented as
, where the ensemble average is taken over all pairs of points on the surface, which are separated by an in-plane length r. For a self-affine surface, this correlation function displays an asymptotic behavior, saturating at 2
, beyond the in-plane correlation length ξ, with HHC displaying scaling relation (Citation14):
(1)
(1) Here with
,
for
and
for
. Then,
for
and
for
. H is referred to as the roughness exponent or Hurst parameter
. It describes the scaling and fractal properties of the surface and characterizes the degree of surface irregularity. Small values of
) correspond to extremely jagged or irregular surface, whereas large values
are related to smooth topography. The fractal dimension (
) of the surface is further related to the Hurst exponent,
(Citation17). The correlation length ξ represents the separation among the correlated structures on the surface.
Wetting on a smooth flat solid substrate can be expressed by Young's relation (Citation18), , where the contact angle
between a surface and a liquid droplet depends on the interfacial free energies of the solid/vapor
, solid/liquid
and liquid/vapor
interfaces. Surfaces are hydrophilic at low values of
, with complete wetting at
, and hydrophobic for
. With the introduction of roughness on the surface, the above relation does not remain valid and wetting is usually described approximately by two classical thermodynamic models of Wenzel (Citation19) and Cassie–Baxter (Citation20). The Wenzel model is based on complete contact at the solid–liquid interface whereas the Cassie–Baxter model is applicable for interfaces that are only partially wetted (Citation21). Some Molecular Dynamics simulations have discussed the influence of correlated roughness on the wetting of self affine (
) surfaces (Citation22). With correlations, distant events can affect each other through interactive fluctuations on a rough surface, and may produce disorder in wetting.
This paper discusses the roughening behavior, scaling properties and the wetting nature of the silicon surfaces irradiated by low energy ion beams. At low ion fluences, Si surfaces show the development of nanostructures and ripples, which grow and evolve at higher fluences. The surface roughness increases after low ion fluences but then systematically decreases with increasing fluence. Height–height correlation technique has been utilized here to obtain the roughness exponent or the Hurst exponent (H) which is a crucial scaling parameter and provides important insight into the growth processes that occur at any surface, growing under non-equilibrium conditions. Here the obtained H values also illustrate that the surfaces are of self affine nature. An increase in the correlation length, ξ, with ion fluence illustrates the evolution of the nanostructures on the ion irradiated surfaces. The nano-patterned surfaces show near hydrophobic behavior, which gets further enhanced with increasing irradiation.
2. Experiment
Commercially available epi-polished p-Si(100) single crystals, procured from MTI corp. USA, have been used here. Off normal ion irradiations (with ions incident at an angle of from the normal) were performed on the Si substrates at room temperature under UHV conditions using 3 keV Ar
at the fluences of
,
and
. Si(100) surface shows the formation of rich variety of nanostructures at these ion incident angles which are also important in applications (Citation23).
The morphology of the surfaces has been investigated by Atomic Force Microscope (Nanoscope V Multimode) from Bruker. All images reported here have been acquired in tapping mode under ambient conditions. The presented HHC data is derived from an analysis of nearly 300 AFM images with varying scan sizes. The contact angle (CA) measurements were performed with water droplets in static mode using a commercial CA goniometer (OCA15EC) from Dataphysics. Contact angles were measured from right- and left-side views of the image with an accuracy of . An average of both angles for 8 images is reported here.
3. Results and discussion
Figure shows SPM images from pristine (un-irradiated) silicon surface as well as after ion irradiation at various fluences. The pristine surface is smooth with an rms roughness of 0.072 nm. After irradiation at low fluences of and
, development of weak ripple patterns and nanostructures is observed with an overall surface rms roughness of 0.192 and 0.187 nm, respectively. A well-defined ripple pattern is observed after the fluence of
. The rms roughness at this stage is 0.139 nm. The ripple patterns become more distinct at the highest fluence with their wave vector
oriented parallel to the ion beam direction. Spontaneous formation of self-organized nanoscale patterns on surfaces, during ion irradiation, is controlled by the competition between the erosion on the surface and smoothening by diffusive processes (Citation24).
Figure 1. AFM images for (a) unirradiated surface as well as after irradiation with fluences of (b)
, (c)
and (d)
. Ion beam direction is shown by an arrow.
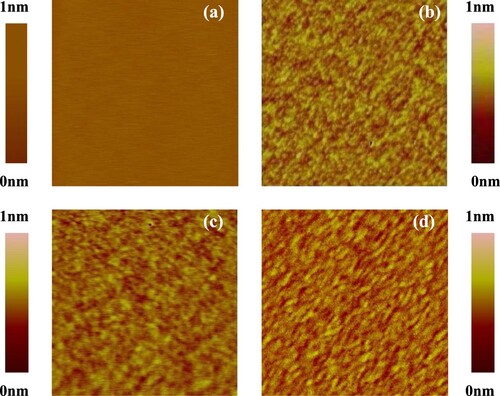
For computing HHC function, the data for height for a given distance ( r) is extracted from AFM image. With
, it is computed by first taking the average height across all data points and subsequently subtracting this average height from each data point with. HHC is shown in Figure for pristine (using Figure a) and surfaces irradiated with fluences of
(using Figure b),
(using Figure c) and
(using Figure d). In addition, for calculating HHC, many more (
) images of various scan sizes were used. With
when
and
when
, a phenomenological scaling function of form
(2)
(2) has been utilized (Citation25) to obtain the values of σ, ξ and H (Table ) from the fit. The rms roughness σ is 0.1 nm for the un-irradiated surface but increases to 0.21 nm after irradiation of
. For higher fluences, this rms roughness decreases. However, the decrease is small. σ, and its trends, obtained here using HHC are similar to the behavior seen via AFM images (in Figure ). The height fluctuations or the patterning on a surface occurs via a combination of several factors like sputter induced surface erosion, as well as bulk and surface diffusion during the irradiation. The decrease in the surface roughness can be contributed by enhanced diffusive processes as well as changes in the nano patterns. For the un-irradiated surface a high ξ is observed, which decreases on the surface irradiated at
. With further irradiation, a systematic increase in ξ indicates enlarging nanostructures as well as the evolution of ripples (Figure d). This also suggests an increased long-ranged roughness. Hurst parameter H, on the other hand, is related to the short wavelength roughness (Citation17). With 0.5<H<1, all these surfaces can be characterized as self affine (Citation14). Self-affine surfaces have the property that under suitable scale transformations, the statistical properties of the surfaces remain invariant (Citation14). Highest H is observed here for the surfaces irradiated with
and decreases systematically for higher fluences. Qualitatively, decrease in H (or increase in fractal dimension
) suggests an increase in the short-ranged roughness (Citation17). Moreover, with
and
, these surfaces have weak roughness which can cause liquid drop to wet the crevices on the surface upon contact (Citation26, Citation27).
Figure 2. HHC for the un-irradiated surface as well as after irradiation with fluences and values of fit.
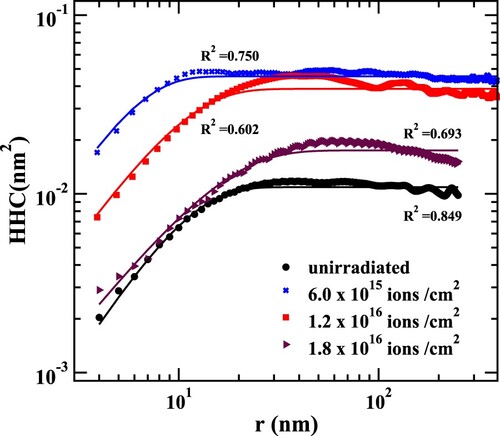
Table 1. HHC fitting parameters (σ, ξ, H, ) and contact angle (CA) of surfaces.
The contact angle measurements on all the silicon surfaces are presented in Figure . For the un-irradiated surface, a contact angle of is obtained (Figure a). Similar value of CA has been earlier observed on clean silicon (Citation28). After irradiation, contact angle systematically increases (Table ). The ion sputtered surfaces, thus, tend to wet less than the un-irradiated surface. With the fractal dimension of the surface, D
, increasing mildly with fluence (Table ), contact angle and wetting behavior demonstrate dependence on the scaling properties of the surface. Increasing D
increases the short wavelength roughness, and this surface jaggedness along with other morphological parameters, σ and ξ play role in determining the wetting properties of the silicon surface (Citation27).
4. Conclusion
This paper discusses the roughening behavior, scaling properties and the wetting nature of Si(100) surfaces that have been modified via low energy ion beams. The surfaces display the development of nanostructures and ripple patterns upon irradiation. The scaling properties have been investigated and the height–height correlation technique has been applied to understand the scaling parameters. The Hurst (H) exponent illustrates that the surfaces are of self-affine nature. The nano-patterned surfaces exhibit a less hydrophilic nature, which gets further reduced with increasing irradiation.
Acknowledgments
We would like to thanks Prof. A. P. Pathak for long-term association.
Disclosure statement
No potential conflict of interest was reported by the author(s).
References
- Soo Choi, H.; Liu, W.; Misra, P.; Tanaka, E.; Zimmer, J.P.; Itty Ipe, B.; Bawendi, M.G.; Frangioni, J.V. Nat. Biotechnol. 2007, 25, 1165–1170.
- O'Farrell, N.; Houlton, A.; Horrocks, B.R. Int. J. Nanomed. 2006, 4, 451–472.
- Tanaka, T.; Mangala, L.S.; Vivas-Mejia, P.E.; Nieves-Alicea R, R.; Mann, A.P.; Mora, E.; Han, H.D.; Shahzad, M.M.; Liu, X.; Bhavane, R.; Gu, J.; Fakhoury, J.R.; Chiappini, C.; Lu, C.; Matsuo, K.; Godin, B.; Stone, R.L.; Nick, A.M.; Lopez-Berestein, G.; Sood, A.K.; Ferrari, M. Cancer Res. 2010, 70, 3687.
- Xu, R.; Huang, Y.; Mai, J.; Zhang, G.; Guo, X.; Xia, X.; Koay, E.J.; Qin, G.; Erm, D.R.; Li, Q.; Liu, X.; Ferrari, M.; Shen, H. Small 2013, 9, 1799–1808.
- Zhang, Y.F.; Tang, Y.H.; Wang, N.; Yu, D.P.; Lee, C.S.; Bello, I.; Lee, S.T. Appl. Phys. Lett. 1998, 72, 1835–1837.
- Wang, Y.; Wang, T.; Da, P.; Xu, M.; Wu, H.; Zheng, G. Adv. Mater. 2013, 25, 5177–5195.
- Liu, Z.; Sun, X.; Nakayama-Ratchford, N.; Dai, H. ACS Nano. 2007, 1, 50–56.
- Gu, L.; Ruff, L.E.; Qin, Z.; Corr, M.; Hedrick, S.M.; Sailor, M.J. Adv. Mater. 2012, 24, 3981–3987.
- Cole, D. Surface Chemistry and Adhesive Properties of Oxidised Si Surfaces, Thesis Queens College, University of Cambridge, 2007.
- Mandelbrodt, B.B. The Fractal Geometry of Nature; Freeman: New York, 1982.
- Ramasco, J.J.; Lopez, J.M.; Rodriguez, M.A. Phys. Rev. Lett. 2000, 84, 2199–2202.
- Palasantzas, G.; Krim, J. Phys. Rev. B. 1993, 48, 2873–2877.
- Family, F.; Vicsek, T. Dynamics of Fractal Surface; World Scientific: Singapore, 1991.
- Barabasi, A.L.; Stanley, H.E. Fractal Concepts in Surface Growth; Cambridge University Press: Cambridge, 1995.
- Solanki, V.; Majumder, S.; Mishra, I.; Dash, P.; Singh, C.; Kanjilal, D.; Varma, S. J. Appl. Phys. 2014, 115, 124306.
- Majumder, S.; Paramanik, D.; Solanki, V.; Bag, B.P.; Varma, S. Appl. Phys. Lett. 2011, 98, 053105.
- Yang, C.; Tartaglino, U.; Persson, B.N.J. Phys. Rev. Lett. 2006, 97, 116103.
- Young, T. Philos. Trans. R. Soc. London. 1805, 95, 65–87.
- Wenzel, N. Ind. Eng. Chem. 1936, 38, 988–994.
- Cassie, B.D.; Baxter, S. Trans. Faraday Soc. 1994, 40, 546.
- Zhou, X.B.; De Hosson, J.T.M. J. Mater. Res. 1995, 10, 1984–1992.
- Chow, T.S. J. Phys. Condens. Matter. 1998, 10, L445–L451.
- Mishra, I.; Majumder, S.; Joshi, S.R.; Subudhi, U.; Varma, S. Chem. Phys. 2022, 562, 111665.
- Keller, A.; Facsko, S. Materials 2010, 3, 4811–4841.
- Kesarwani, R.; Dey, P.P.; Khare, A. RSC Adv. 2019, 9, 7967–7974.
- Sarkar, S.; Patra, S.; Gayathri, N.; Banerjee, S. Appl. Phys. Lett. 2010, 96, 063112.
- Palasantzas, G.; De Hosson, J.T.M. Acta Mater. 2001, 49, 3533–3538.
- Han, S.; Yang, R.; Li, C.; Yang, L. Appl. Sci. 2019, 9, 566.