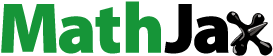
Abstract
One pot sequential reactions of silica gel with tetrachlorosilane/triethylamine mixture and after with the conical upper rim tetraphosphorylated tetrahydroxy(thia)calixarenes yielded porous organo-inorganic sorbents modified by spatially ordered phosphonate, phosphinate or phosphine oxide groups capable of co-operative binding of metal cations. The grafting improves efficacy of europium(III) sorption by the silica gels. DFT calculations of the calixarene-Eu3+ complexes predict a calixarene flattened cone conformation with the coordination of Eu3+ cation to three of four P = O oxygen atoms and one of the aromatic systems. Adding three water molecules excludes completely cation–π interactions.
GRAPHICAL ABSTRACT
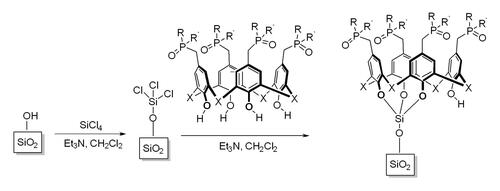
Introduction
The reprocessing and burial of radiotoxic nuclear power plant waste in deep mines represents a less-talked-about grand challenge confronting modern science and technology, in order to enable energy for humankind without the anthropogenic carbon dioxide emissions of fossil fuels. The main objective of this reprocessing is partitioning of short-lived cesium and strontium from long-lived radioactive metals, as well as, secondarily, separation of lanthanides from highly toxic actinides.[Citation1–3] While organophosphorus compounds such as tributylphosphate, 2-diethylhexylphosphonic acid, trialkylphosphine oxides, carbamoylphosphine oxides or their composites with porous organic or inorganic materials are widely used as ligands for radionuclide binding and decontamination,[Citation4] their separation selectivities are generally rather low.
A promising rational approach for improving selectivity of the organophosphorus ligands is linking of their P = O binding groups to a cup-shaped calixarene molecular platform. Such placement facilitates chelation of the metal cations by preorganized P = O groups.[Citation5] It has been shown that these cooperative interactions in phosphorylated calix[4] arenes increase the efficacy and selectivity of extraction of actinides and lanthanides, compared to commercial organophosphorus extractants.[Citation6,Citation7]
Calix[4] arenes modified with four bidentate carbamoylphosphine-oxide substituents efficiently extract both actinides and lanthanides.[Citation8,Citation9] On the other hand, in the pioneering work at the interface of supramolecular chemistry and materials, calixarene modified with carbamoylphosphine-oxide functional groups were covalently grafted to the silica gel surface, and demonstrate remarkable europium/americium selectivity.[Citation10]
In this work, a method for the covalent grafting of the upper-rim tetraphosphorylated tetrahydroxy(thia)calix[4] arenes to the silica gel surface was developed, and europium(III) sorption by the porous hybrid organic-inorganic materials was investigated.
Results and discussion
Grafting of tetraphosphorylated (thia)calix[4] arenes to the silica-gel surface
The covalent grafting of the lower rim tetraphosphorylated tetrahydroxy(tia)calix[4] arenes to the surface of silica gel 1 was performed in two stages according to literature examples[Citation10] (Scheme 1). In the first stage, silica gel 1 was treated with an excess of tetrachlorosilane and triethylamine in dichloromethane. After that, the modified silica gel 2 was heated with an excess of a mixture of phosphorylated tetrahydroxy(thia)calixarene 3a–e and triethylamine in toluene to give calixarene-grafted silica 4a–e.
Isopropoxyphosphonate and isopropoxyphosphinates 4a, b, d were converted into the corresponding dihydroxyphosphonic acids 5a, c or hydroxyphenylphosphinic acid 5b using the McKenna method.[Citation11] The reaction of 4a, b, d with an excess of trimethylbromosilane replaces isopropyl groups with trimethylsilyl groups, and leads to intermediate trimethylsilyl esters 6a, b, c. The subsequent treatment of 6a, b, c with an excess of wet methanol cleaves the P–O–Si bond and gives phosphonic or phosphinic acids 5a, b, c.
The content of the covalently grafted calixarenes on silica gels 4 and 5 was measured gravimetrically and ranged from 0.06 mmol/g (4e) to 0.18 mmol/g (4b) (). Such a significant calixarene concentration range on the silica gel surface can be explained by a different reactivity of OH groups at the lower rim and size of substituents at the upper rim of calixarenes 3a–e.[Citation12]
Table 1. Content of calixarenes grafted on silica gels 4, 5.
It should be noted that the treatment of isopropyl ethers 4a, b, d with trimethylbromosilane along with the substitution of isopropyl groups by trimethylsilyl groups causes some undesired side reaction of cleavage of Si–O bonds at the lower rim of the macrocycle, and slightly reduces the content of the grafted calixarenes ().
The calixarene-grafted silica gels 4 and 5 retain a fine-grained porous structure () according to scanning electron microscopy. We observed bands in the FTIR spectra of 4a, 4b, 5a and 5c near 1450 cm−1, which correspond to the C–C stretch of the aromatic rings.
Eu(III) sorption by silica gels
In order to evaluate the function of the synthesized grafted calixarenes on silica, sorption of europium(III) by silica gels 1, 4 and 5 from nitric acid solutions was investigated in the pH range of 1-7. The extraction of europium depends on the type of calixarene platform (X = CH2, S), the nature of the phosphorus-containing substituents, the calixarene surface concentration, and the acidity of the solution (). It should be noted that the grafting effect is most significant at pH 2–3, where matrix silica gel loses its ability to sorb Eu(III).
Table 2. pH Dependence of Eu(III) sorption by silica gels 1, 4 and 5 from nitric acid solutions.
For silica gels 4a, b and 5a, c, sorption isotherms were fitted with Langmuir and Freundlich isotherm models using non-linear regression. The Langmuir model characterizes a single-layer adsorption, and assumes no lateral interaction between adsorbates, whereas the Freundlich model characterizes adsorption on the binding sites with different affinities to the adsorbate. Fitted constants of the two models as well as correlation coefficients are presented in .
Table 3. Sorption parameters obtained according to Langmuir and Freundlich models.
Experimental data fitted with two isotherm models are shown in . The shape of the isotherm profiles is consistent with a complex structure of the sorbents, which possess a variety of binding sites.
Figure 2. Sorption isotherms of europium(III) by silica gels 4a, b and 5a, c: Langmuir model (a) and Freundlich model (b).
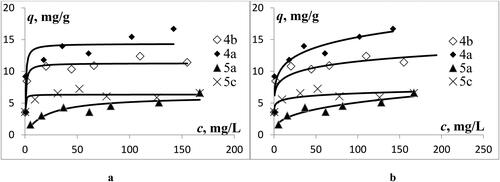
Such a behavior of the isotherms and close R2 values for the two models indicate energetic heterogeneity of the sorbent surfaces, and the realization of several binding mechanisms. Generally, it can be concluded that experimental data points are better fitted by the Langmuir isotherm equation. Sorption capacities were determined to be in the range of 6.4–14.4 mg/g (). The capacities are dependent on the electronic nature of the substituents at the phosphorus atoms, the size of the (thia)calixarene platform, and the content of the grafted (thia)calixarenes in the material.
Calculations
DFT modeling (RI-BP86/TZVP) was conducted to understand the nature of the Eu(III) complex formation. For this purpose, model calixarene tetrakis-dimethylphosphine oxide 7′ ( and ), which simulates the calixarenes grafted to silica gel (4), and complex 7′ with Eu(III) ( and ) were calculated. In model structure 7′, where three hydroxy-groups on the lower rim are connected by a silicon atom, the flattened cone conformation is fixed. Additional interactions stabilizing the conformation are a network of weak H-bonding between hydrogen atoms in the methyl groups and oxygen atoms of proximal P = O groups on the upper rim of the calixarene.
Figure 3. General structures of model calixarene 7' (a), and its Eu(III) complexes 7′Eu (b) and 7′Eu⋅3H2O (c).
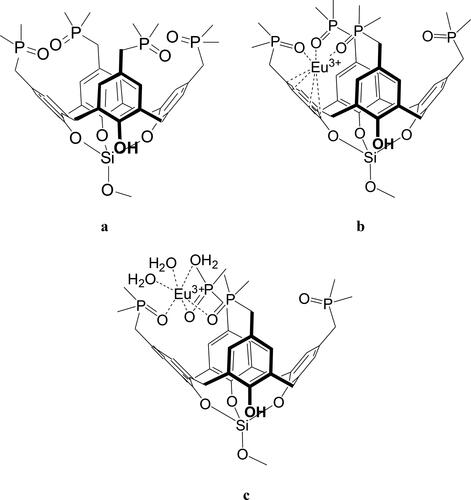
Figure 4. Optimized (RI-BP86/def-TZVP) structures of model calixarene 7′, and its Eu(III) complexes 7′Eu and 7′Eu⋅3H2O. Color coding: gray – C, red – O, beige – Si, light green – Eu. Hydrogen atoms are omitted for clarity (the structures are graphically presented with Jmol program[Citation12]).
![Figure 4. Optimized (RI-BP86/def-TZVP) structures of model calixarene 7′, and its Eu(III) complexes 7′Eu and 7′Eu⋅3H2O. Color coding: gray – C, red – O, beige – Si, light green – Eu. Hydrogen atoms are omitted for clarity (the structures are graphically presented with Jmol program[Citation12]).](/cms/asset/0662fc04-272f-417b-98af-f9cd2eacd540/gpss_a_2208705_f0004_c.jpg)
Recently, for ‘free’ (not grafted) calixarenes with similar substituents on the upper ring (-CH2-P(O)Ph2 and -CH2-P(O)(OMe)2), electronic-structure calculations predicted a participation of all P = O oxygen atoms in the coordination mode with a Eu3+ cation guest.[Citation13] In contrast, in the model complex 7'Eu () only three of the four oxygen atoms in the P = O moieties and one aromatic π-system of the calixarene coordinate to the metal cation (). The corresponding distances between Eu3+ and the four oxygen atoms of the P = O groups are 2.258, 2.260, 2.270 and 6.149 Å. The distances between Eu3+ and the six carbon atoms of the aromatic moiety are 3.17 to 3.68 Å, indicating much weaker cation–π interactions. The structure predicted for the gas phase led us to suggest that it is coordinatively unsaturated. The complex geometry will significantly change due to coordination of water molecules to Eu3+ as additional ligands (see as example of the complex 7'Eu with attached three water molecules 7'Eu⋅3H2O). The latter adduct includes a slightly distorted octahedral metal environment without any interaction with the aromatic π-systems of calixarene.
Experimental
Materials and instruments
Silica gel S60 (Zeocem, Bosnia and Herzegovina, particle size 40–60 µm, bulk density 481 g/l, pore volume 0.73 mL/g, specific surface area 486 m2/g, pore size 6.0 nm) was dried at 900 °C for 6 h before use. Dichloromethane, chloroform, and toluene were distilled over phosphorus pentoxide before use. All reactions were performed in a dry argon atmosphere using Schlenk-line procedures.
The pH was monitored using a pH meter STARTER 3100 (Ohaus, USA). The Eu(III) concentration in solutions before and after sorption was determined using inductively coupled plasma atomic emission spectrometry, using an iCAP 6300 Duo instrument (Thermo Scientific, USA).
Synthesis of tetraphosphorylated tetrahydroxycalix[4] arenes 3a–e
Calixarenes 3a–e were synthesized by the Arbuzov reaction of chlorometyl(thia)calix[4] arenes with isopropyl esters of corresponding PIII-acids according Ungaro’s[Citation14] and Shinkai’s[Citation15] method (see Scheme 1 in Supporting Information). Synthesis of compounds 3a,[Citation16] 3b[Citation17] and 3d[Citation18] has been described previously. Syntheses and characterization of compounds 3c and 3e are described in the Supporting Information.
Grafting of the silica gel surface with (thia)calix[4] arene phosphonates, phosphinates, and phosphine oxides
A solution of SiCl4 (1 M) and triethylamine (0.69 g, 6.8 mmol) in dichloromethane (20 mL) was added to a suspension of pre-dried silica gel (1.5 g) in dichloromethane (20 mL). The resulting mixture was stirred at room temperature for 12–14 h. The solvent was evaporated under reduced pressure (10–15 mm Hg) to yield a white powder, stored in a dry argon atmosphere. To the modified silica gel, a solution of (thia)calixarene 3a–e (0.46 mmol) and triethylamine (1.75 g, 17.3 mmol) in toluene (20 mL) was added. The suspension was stirred for 24 h at 110 °C under argon. After cooling, the grafted silica gels 4a–e was recovered via filtration, and washed with hot toluene (100-150 mL), methanol (20 mL), water (60 mL), and methanol again (60 mL), before being dried in vacuum (0.05 mm Hg) for 4 h at 40 °C. A white powder was obtained.
Synthesis of grafted (thia)calix[4] arene phosphonous or phosphinous acids on silica gel 5a–с
A solution of trimethylbromosilane (4.2 mL, 32 mmol) in dry chloroform (10 mL) was added to silica gels 4a, b, d (0.5 g). The suspension was stirred at room temperature for 48 h. The solid was (i) filtered off; (ii) washed with chloroform; (iii) stirred with methanol (5 mL) overnight at room temperature; (iv) filtered again; (v) washed with water, methanol and chloroform; (vi) dried under vacuum (0.05 mm Hg) for 4 h at room temperature. A white powder was obtained.
Eu(III) sorption by silica gels 1, 4, 5
The sorption was investigated in static mode. A suspension of the silica gel (0.025 g) in a nitric acid solution of Eu(III) (20 mL, concentration of Eu was 10 mg/L) was stirred at 300 rpm in a conical flask for 3 h at room temperature. The solution was filtered. The concentration of the Eu(III) solution before and after sorption was determined to calculate the amount that was adsorbed by difference.
Determination of the sorption parameters
Sorption capacity was calculated by the formula where
is initial concentration of Eu3+, mg/l;
is the concentration of Eu3+, mg/l in solution after sorption;
is volume of solution, l; and
is mass of sorbent, g.
The equation of the Langmuir isotherm has the following form where
is sorption capacity, mg/g;
is maximum sorption capacity, mg/g;
is Langmuir adsorption equilibrium constant, l/mg;
is equilibrium concentration of Eu3+, mg/l.
The Freundlich isotherm is described by the following equation where
is Freundlich’s constant, mg/g;
is equilibrium concentration of Eu3+, mg/l, and 1/n is indicates the intensity of the adsorption process and the distribution of active centers on the surface.
Details of calculations
All calculations were carried out using the TURBOMOLE program package (versions 6.4[Citation19] and 7.6[Citation20]).[Citation21] Geometry optimizations were performed without symmetry constrains using an unrestricted DFT (RI-BP86) level of theory.[Citation22,Citation23] Resolution of the Identity (RI)[Citation23–27] approximation was utilized in all cases to increase calculation speed and efficiency. For H, C, O and Si, the def-TZVP basis sets were used as implemented into the TURBOMOLE program set: the TZV triple-zeta basis sets[Citation28] were extended by adding polarization functions. This approximation level has previously proven to successfully reproduce conformational effects in calixarenes.[Citation29–31] For Eu, the basis set was used as implemented into def-TZVP basis sets: the pseudopotential for describing internal electronic shells[Citation32] and valence orbitals were represented as (14s13p10d8f1g)/[10s8p5d4f1g] with contraction (5111111111/61111111/61111/5111/1). The calculations were carried out for septet and nonet (six and eight unpaired electrons, respectively) ground-state structures. For the studied complexes, the septet electronic configuration was definitely more favored than that of nonet ground state, however the former one demonstrated slight spin contamination (<S*S > factor ∼12.6, app. 5%), due to the small derived HOMO–LUMO gap (∼0.021 eV). Vibration frequencies were derived analytically for the optimized structures at the RI-BP86/def-TZVP level of theory. All of the structures corresponded to local energy minima, and no imaginary frequencies were detected by vibration analyses. The complete calculation data set is given in the ESI.
Conclusions
Sequential treatment of silica gel with silicon tetrachloride and cup-shaped upper-rim phosphorylated tetrahydroxy(thia)calix[4] arenes in the presence of triethylamine leads to porous hybrid organic-inorganic adsorbents, the surface of which is modified by spatially organized phosphonate, phosphinate and phosphine oxide substituents, which are preorganized for metal cation complexation. Diisopropoxyphosphonate and isopropoxyphosphinate derivatives were converted into corresponding dihydroxyphosphonic acid or hydroxyphenylphosphinic acid derivatives by the treatment with trimethylbromosilane and subsequently with methanol. At the same time, trimethylbromosilane causes a side reaction of cleavage of Si–O bonds at the lower rim of the macrocycle, which slightly reduces the content of calixarene on the silica gel surface.
Silica-based sorbents containing 0.06–0.18 mmol/g of the covalently linked phosphorylated (thia)calixarenes were obtained by these methods. The covalent grafting of the calixarene on the silica gel surface significantly increases sorption of Eu(III) ions from dilute nitric acid solutions at pH 2–3 where the matrix silica gel loses its ability to sorb Eu(III). The sorption isotherms of Eu(III) are described by either Langmuir or Freundlich models, and demonstrate high sorption capacity exceeding up to 99% of europium from aqueous solution.
Electronic structure calculations of the calixarene-Eu(III) complexes predict a flattened cone conformation of the calixarene platform, and the coordination of a Eu(III) cation with three of the four P = O oxygen atoms and one of the aromatic functional groups of the grafted calixarene site. Adding three water molecules completely excludes the cation–π interactions.
Acknowledgements
A. B. Rozhenko thanks TURBOMOLE GmbH for kind providing the license for the newest version (v. 7.6) of the TURBOMOLE set of programs.
Disclosure Statement
The authors declare that they have no known competing financial interests or personal relationships that could have appeared to influence the work reported in this paper.
Additional information
Funding
References
- Jurate, V.; Mika, S. Distribution and Removal of Radionuclides from Contaminated Medium: A Review. Res. J. Chem. Environ. 2007, 11, 86– 103.
- Dietz, M. L.; Horwitz, E. P. Combining Solvent Extraction Processing for Actinide and Fission Product Separations. In Science and Technology for Disposal of Radioactive Tank Wastes, Schulz, W. W.; Lombardo, N. J. (Eds.); Plenum Press: New York, 1998.
- Runde, W. H.; Schulz, W. W. The Chemistry of the Actinide and Transactinide Elements. Morss, L. R.; Edelstein, N. M.; Fuger, J.; Katz, J. J. (Eds.), Springer, Dordrecht, 2006, 2, 1278– 1284
- Leoncini, A.; Huskens, J.; Verboom, W. Ligands for f-Element Extraction Used in the Nuclear Fuel Cycle. Chem. Soc. Rev. 2017, 46, 7229–7273. DOI: 10.1039/C7CS00574A.
- Karavan, M.; Arnaud-Neu, F.; Hubscher-Bruder, V.; Smirnov, I.; Kalchenko, V. Novel Phosphorylated Calixarenes for the Recognition of f-Elements. J. Incl. Phenom. Macrocycl. Chem. 2010, 66, 113–123. DOI: 10.1007/s10847-009-9660-4.
- Klimchuk, O.; Atamas, L.; Miroshnichenko, S.; Kalchenko, V.; Smirnov, I.; Babain, V.; Varnek, A.; Wipff, G. New Wide Rim Phosphomethylated Calix[4]arenes in Extraction of Americium and Europium. J. Incl. Phenom. Macrocycl. Chem 2004, 49, 47–56. DOI: 10.1023/B:JIPH.0000031112.16272.17.
- Kharchenko, S.; Drapailo, A.; Shishkina, S.; Shishkin, O.; Karavan, M.; Smirnov, I.; Ryabitskii, A.; Kalchenko, V. Dibutylphosphinoylmethyloxythiacalix[4]arenes. Synthesis, Structure, Americium, Europium and Technetium Extraction. Supramol. Chem. 2014, 26, 864–872. DOI: 10.1080/10610278.2014.890198.
- Atamas, L.; Klimchuk, O.; Rudzevich, V.; Pirozhenko, V.; Kalchenko, V.; Smirnov, I.; Babain, V.; Efremova, T.; Varnek, A.; Wipff, G.; et al. New Organophosphorus Calix[4]arene Ionophores for Trivalent Lanthanide and Actinide Cations. J. Supramol. Chem. 2002, 2, 421–427. DOI: 10.1016/S1472-7862(03)00052-2.
- Böhmer, V.; Dozol, J.-F.; Gruttner, C.; Liger, K.; Matthews, S. E.; Rudershausen, S.; Saadioui, M.; Wang, P. Separation of Lanthanides and Actinides Using Magnetic Silica Particles Bearing Covalently Attached tetra-CMPO-Calix[4]arenes. Org. Biomol. Chem. 2004, 2, 2327–2334. DOI: 10.1039/b405602g.
- May, E.; Solovyov, A.; Guo, Y.; Drapailo, A.; Matveev, Y.; Kalchenko, V.; Nitsche, H.; Katz, A. Unprecedented Increase in Affinity for Eu III over Am III through Silica Grafting of a Carbamoylmethylphosphine Oxide Calix[4]arene site. Eur. J. Inorg. Chem. 2016, 2016, 4542–4545. DOI: 10.1002/ejic.201600946.
- McKenna, C. E.; Higa, M. T.; Cheung, N. H.; McKenna, M.-C. The Facile Dealkylation of Phosphonic Acid Dialkyl Esters by Bromotrimethylsilane. Tetrahedron Lett. 1977, 18, 155–158. DOI: 10.1016/S0040-4039(01)92575-4.
- Katz, A.; Da Costa, P.; Lam, A.; Notestein, J. The First Single-Step Immobilization of a Calix[4]arene onto the Surface of Silica. Chem. Mater. 2002, 14, 3364–3368. DOI: 10.1021/cm020082l.
- Jmol: an open-source Java viewer for chemical structures in 3D. http://www.jmol.org/., version 13.0.12, 2013.
- Almi, M.; Arduini, A.; Casnati, A.; Pochini, A.; Ungaro, R. Chloromethylation of Calixarenes and Synthesis of New Water-Soluble Macrocyclic Hosts. Tetrahedron 1989, 45, 2177–2182. DOI: 10.1016/S0040-4020(01)80077-6.
- Arimura, T.; Nagasaki, T.; Shinkai, S.; Matsuda, T. Host-Guest Properties of New Water-Soluble Calixarenes Derived from p-(Chloromethyl) Calixarenes. J. Org. Chem. 1989, 54, 3766–3768. DOI: 10.1021/jo0027a003.
- Yesypenko, O. A.; Boiko, Y. S.; Belikov, K. N.; Bryleva, K. Y.; Bunina, Z. Y.; Rozhenko, A. B.; Shishkina, S. V.; Drapailo, A. B.; Rodik, R. V.; Atamas, L. I.; et al. Surface Modification of Aminopropylated Silica Gel with Tetraphosphorylated Bis-Methoxycarbonylmethoxycalix[4]arenes for Effective Europium(III) Sorption. Theor. Exp. Chem. 2020, 56, 252–260. DOI: 10.1007/s11237-020-09656-0.
- Buldenko, V. M.; Trush, V. V.; Kobzar, O. L.; Drapailo, A. B.; Kalchenko, V. I.; Vovk, A. I. Calixarene-Based Phosphinic Acids as Inhibitors of Protein Tyrosine Phosphatases. Bioorg. Med. Chem. Lett. 2019, 29, 797–801. DOI: 10.1016/j.bmcl.2019.01.026.
- Kasyan, O.; Swierczynski, D.; Drapailo, A.; Suwinska, K.; Lipkowski, J.; Kalchenko, V. Upper Rim Substituted Thiacalix[4]Arenes. Tetrahedron Lett. 2003, 44, 7167–7170. DOI: 10.1016/S0040-4039(03)01809-4.
- TURBOMOLE V6.4 2012, A Development of University of Karlsruhe and Forschungszentrum Karlsruhe GmbH, 1989-2007, TURBOMOLE GmbH, since 2007; available from http://www.turbomole.com.
- TURBOMOLE V7.6 2021, A Development of University of Karlsruhe and Forschungszentrum Karlsruhe GmbH, 1989-2007, TURBOMOLE GmbH, since 2007; available from http://www.turbomole.com.
- Furche, F.; Ahlrichs, R.; Hättig, C.; Klopper, W.; Sierka, M.; Weigend, F. Turbomole. Wiley Interdiscip. WIREs Comput. Mol. Sci. 2014, 4, 91–100. DOI: 10.1002/wcms.1162.
- Becke, A. D. Density-Functional Exchange-Energy Approximation with Correct Asymptotic Behavior. Phys. Rev. A Gen. Phys. 1988, 38, 3098–3100. DOI: 10.1103/PhysRevA.38.3098.
- Perdew, J. P. Density-Functional Approximation for the Correlation Energy of the Inhomogeneous Electron Gas. Phys. Rev. B Condens. Matter. 1986, 33, 8822–8824. DOI: 10.1103/PhysRevB.33.8822.
- Whitten, J. L. Coulombic Potential Energy Integrals and Approximations. J. Chem. Phys. 1973, 58, 4496–4501. DOI: 10.1063/1.1679012.
- Dunlap, B. I.; Connolly, J. W. D.; Sabin, J. R. On Some Approximations in Applications of Xα Theory. J. Chem. Phys. 1979, 71, 3396–3402. DOI: 10.1063/1.438728.
- Vahtras, O.; Almlöf, J.; Feyereisen, M. W. Integral Approximations for LCAO-SCF Calculations. Chem. Phys. Lett. 1993, 213, 514–518. DOI: 10.1016/0009-2614(93)89151-7.
- Eichkorn, K.; Treutler, O.; Öhm, H.; Häser, M.; Ahlrichs, R. Auxiliary Basis Sets to Approximate Coulomb Potentials. Chem. Phys. Lett. 1995, 240, 283–290. DOI: 10.1016/0009-2614(95)00621-A.
- Schäfer, A.; Huber, C.; Ahlrichs, R. Fully Optimized Contracted Gaussian Basis Sets of Triple Zeta Valence Quality for Atoms Li to Kr. J. Chem. Phys. 1994, 100, 5829–5835. DOI: 10.1063/1.467146.
- Stoll, I.; Mix, A.; Rozhenko, A. B.; Neumann, B.; Stammler, H.-G.; Mattay, J. Kemp’s Triacid Attached to octa-O-Methyl Resorc[4]arenes: Conformations in Solution and Comparative Binding Studies with Various 2-Amino Pyridines. Tetrahedron 2008, 64, 3813–3825. DOI: 10.1016/j.tet.2008.01.138.
- Letzel, M. C.; Schäfer, C.; Novara, F. R.; Speranza, M.; Rozhenko, A. B.; Schoeller, W. W.; Mattay, J. A. Kinetic Study of Guest Displacement Reactions on a Host-Guest Complex with a Photoswitchable Calixarene. J. Mass Spectrom. 2008, 43, 1553–1564. DOI: 10.1002/jms.1464.
- Fraschetti, C.; Letzel, M. C.; Paletta, M.; Mattay, J.; Speranza, M.; Filippi, A.; Aschi, M.; Rozhenko, A. B. Cyclochiral Resorcin[4]arenes Aseffective Enantioselectors in the Gas Phase. J. Mass Spectrom. 2012, 47, 72–78. DOI: 10.1002/jms.2028.
- Dolg, M.; Stoll, H.; Preuss, H. Energy‐Adjusted Ab Initio Pseudopotentials for the Rare Earth Elements. J. Chem. Phys. 1989, 90, 1730–1734. DOI: 10.1063/1.456066.