Abstract
We studied the expression of programed death 1 (PD-1) receptor and its ligands (PD-L1/-L2) by immunohistochemistry and its association with clinicopathological features in 81 posttransplant lymphoproliferative disorders (PTLDs) following solid organ transplantation. Overall, 67% (54/81) of the PTLDs were positive in any of the three immunostainings. PD-1 was detected on tumor-infiltrating cells in 41% (33/81) of the PTLDs. PD-L1 was expressed on ≥5% of the tumor cells in 50% (40/80) and PD-L2 in 32% (23/72) of the PTLDs. All Burkitt lymphomas were PD-L1 negative. Expression of PD-L1 tended to be associated with non-germinal center-type of diffuse large B-cell lymphoma (63% vs. 33% in GC-type, p = .14) and latent membrane protein-1+ PTLD (76% vs. 44% in LPM1-, p = .09). Heart recipients had more frequent PTLDs with PD-1+ microenvironment (p = .01). The frequent expression of PD-1 or -L1/-L2 in PTLD warrants further clinical evaluation of the efficacy and safety of PD-(L)1 inhibitors for refractory PTLD.
Introduction
Posttransplant lymphoproliferative disorder (PTLD) is a potentially fatal complication, which affects ∼1% of recipients of solid organ transplants [Citation1]. Although some patients respond to reduction of immunosuppression alone, most patients need treatment with rituximab and/or chemotherapy [Citation2–4]. The prognosis for those who relapse after chemotherapy is dismal and new therapeutic options are needed. Adoptive immunotherapy with Epstein–Barr virus (EBV)-specific cytotoxic T cells has shown good response rates in patients with EBV-positive (EBV+) PTLD who failed conventional therapy but is not readily available [Citation5]. Furthermore, the growing proportion of late-onset EBV– PTLD [Citation6,Citation7] is of course not treatable with such adoptive cell therapy.
In the non-transplant setting, therapy with programed death 1 (PD-1) pathway inhibitors have been exceptionally effective in relapsed or refractory Hodgkin lymphoma [Citation8–10] and partially also in relapsed or refractory diffuse large B-cell lymphoma (DLBCL) and T-cell lymphoma [Citation11,Citation12]. Currently available drugs target either PD-1 or its ligand PD-L1. PD-1 is expressed mainly by activated T cells, but also by natural killer cells, monocytes, and B cells and it interacts with its ligands PD-L1 and -L2, which are expressed on tumor cells and macrophages in the tumor microenvironment. This interaction suppresses the function of effector T cells and is a mechanism for tumors to evade the immune response. Expression of PD-L1 on tumor cells is considered the most robust biomarker for treatment response to PD-(L)1 inhibitors but also PD-1-positive tumor-infiltrating immune cells and PD-L2-positive tumor cells have been associated with the response [Citation13].
Therapy with PD-(L)1 inhibitors could potentially become a treatment option also for refractory or relapsed PTLD. However, this requires knowledge of the expression of PD-1 and its ligands in PTLD. Approximately 60–100% of PTLDs have been reported to be positive for PD-L1 in previous small case series [Citation14–17]. Correlations between expression of PD-1 and its ligands and PTLD subtypes and clinical features have so far been little studied. Therefore, we investigated the expression of PD-1, -L1, and -L2 in 81 PTLDs retrieved from a larger population-based case series and correlated the expression of these biomarkers with clinicopathological features.
Materials and methods
Identification of cases
All cases of PTLD with sufficient material for further analyses (n = 81) from a previously reported population-based case series of PTLDs following solid organ transplantation (n = 135) were included in this study [Citation6]. In brief, 10,010 recipients of solid organ transplants in Sweden between 1970 and 2006 were identified in the Swedish National Inpatient Register. Through linkage to the Swedish Cancer Register individuals who developed lymphoma posttransplant between 1980 and 2006 were identified. The only two cases that were reported between 1970 and 1980 were not included in this study. Polymorphic PTLD, monomorphic B- and T-cell PTLD, and Hodgkin-type PTLD were included, whereas indolent lymphomas and plasma cell tumors were not. The study was approved by the Regional Ethical Review Board in Uppsala, Sweden and was conducted in accordance with the Declaration of Helsinki and the Swedish Ethical Review Act.
Clinical data
The medical records at all transplantation centers in Sweden and from all the hospitals throughout the country where the patients had been followed posttransplant were retrospectively reviewed by one physician (AK) to obtain clinical information. We retrospectively defined the stage of the lymphoma according to Ann Arbor, the performance status according to the Eastern Cooperative Oncology Group, and the age-adjusted International Prognostic Index of the patients if not originally documented in the medical records.
Reevaluation of PTLDs
The tumor biopsies have been reevaluated according to the 2008 revision of the WHO classification of lymphoid neoplasms by an experienced hematopathologist (CS) and the diagnoses are consistent with the revision of 2016 [Citation18]. Cell-of-origin classification of DLBCL into the germinal center (GC) or non-GC subtype was based on immunohistochemistry (IHC) and the Hans algorithm [Citation19]. EBV status in PTLD tissue was analyzed by EBV-encoded RNA (EBER) in situ hybridization (ISH). Expression of latent membrane protein 1 (LMP1) was determined by IHC in routine diagnostics. The presence of intratumoral regulatory T cells (Tregs) was analyzed by IHC for forkhead box protein 3 (FoxP3) as previously reported [Citation20].
Immunohistochemistry for PD-1, PD-L1, and PD-L2
IHC and slide scanning were performed at the Swedish Science for Life Laboratory facilities, Uppsala University with protocols described elsewhere [Citation21]. In brief, 4-μm thick formalin-fixed paraffin-embedded (FFPE) tissue sections of biopsies of PTLD were deparaffinized and re-hydrated, blocked for endogenous peroxidase, and subjected to heat-induced antigen retrieval. Automated IHC was performed using a LabVision Autostainer 480S (Thermo Fisher Scientific, Runcorn, UK). Primary antibodies, PD-1 (dilution 1:35, clone NAT105; Abcam, Cambridge, UK), PD-L1 (dilution 1:75, clone E1L3N; Cell Signaling Technology, Danvers, MA), and PD-L2 (dilution 1:75, rabbit polyclonal, clone HPA013411; Atlas Antibodies, Stockholm, Sweden) were applied to the slides for 30 min, followed by incubation of secondary reagent (anti-rabbit/mouse HRP-conjugated, UltraVision; Thermo Fisher Scientific) and developed with 3,3′-diaminobenzidine as the substrate. Tissue sections were counterstained in Mayer’s hematoxylin and mounted. The slides were scanned using the automated scanning system Aperio XT (Aperio Technologies, Vista, CA).
Analysis of immunostainings and definitions
Evaluation of the immunostainings was done manually by an experienced hematopathologist (CS). At least 10 high power fields were evaluated in areas dominated by lymphoma cells. PD-L1 and PD-L2 positive cases of PTLD were defined as ≥5% of the tumor cells showing moderate or strong staining of PD-L1 or PD-L2 and graded into ≥5–<30% or ≥30% positive tumor cells. Among PD-L1 negative PTLD, PD-L1 in the total tissue cellularity was assessed and a PD-L1+ microenvironment was defined as ≥20% of the total cells showing moderate or strong staining of PD-L1. These thresholds for PD-L1 positivity in tumor cells and microenvironment are comparable with the ones used in previous case series of PTLD and DLBCL [Citation16,Citation22]. The proportion of PD-1+ tumor-infiltrating cells was presented as the number of positive cells per high power field (×200) in accordance with previous studies [Citation23].
Statistical analyses
Comparisons of clinicopathological features were performed using the Chi-square test or the Fisher’s exact test for categorical variables and the Mann–Whitney U-test for continuous variables. End of follow-up for survival was 25 October 2012. Overall survival was defined as the time from PTLD diagnosis to death. Differences in survival were calculated with the log-rank test. p Value less than .05 was considered statistically significant. Statistica Software (version 13; Stat Soft Inc., Tulsa, OK) was used in all analyses.
Results
Clinicopathological characteristics of the study population
The study population consisted of nine children and 72 adults who received kidney (59%), heart (15%), liver (14%), or lung (12%) transplants (). Most patients (75%) had late-onset PTLD, that is, development >1-year posttransplant. A majority (77%) of the patients presented with extranodal disease, most commonly in the gastrointestinal tract (26%). The PTLD involved the allograft in 14%.
Table 1. Associations between the expression of PD-1, PD-L1, and PD-L2 in PTLD tissue, PTLD subtype, and clinical features.
Most PTLDs were monomorphic B cell lymphoma (70%), followed by monomorphic T cell lymphoma (19%), polymorphic PTLD (6%), and Hodgkin lymphoma-type PTLD (2%). The most common subtype was DLBCL (58%), which usually was of the non-GC type (80%). Of the 81 PTLDs, 54% were EBV + and 46% were EBV–.
Expression of PD-1, PD-L1, and PD-L2 in PTLD
Overall, 67% (54/81) of the PTLDs were positive in at least one of the immunostainings for PD-1 on tumor-infiltrating cells or PD-L1 or -L2 on tumor cells. PD-1 was expressed on tumor-infiltrating cells in 41% (33/81) of the PTLDs, whereas no case had tumor cells expressing PD-1 (). PD-L1 was detected in ≥5% of the tumor cells in 50% (40/80) and PD-L2 in 32% (23/72) of the PTLDs. Of the 51 positive cases with results from all three immunostainings, 9 cases (18%) were PD-1+/PD-L1+/PD-L2+, 11 cases (22%) were PD-1+/PD-L1+/PD-L2–, 6 cases (12%) were PD-1+/PD-L1–/PD-L2+, 5 cases (10%) were PD-1–/PD-L1+/PD-L2+, 5 cases (10%) were only PD-1+, 12 cases (24%) were only PD-L1+, and 3 cases (6%) were only PD-L2+ ().
Figure 1. Proportion of PTLDs positive for PD-1 in tumor-infiltrating cells (A) and for PD-L1 and PD-L2 in tumor cells (B).
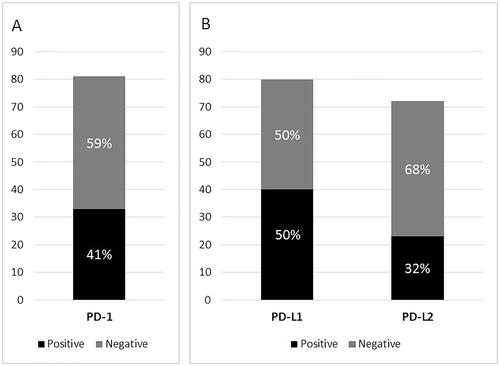
Figure 2. Expression of PD-1, -L1, and -L2 by subtype and EBV-status of PTLD.
PTLD: posttransplant lymphoproliferative disorder; EBER: Epstein–Barr virus-encoded RNA; LMP1: latent membrane protein 1; PD-1: programed death protein 1; PD-L1: programed death ligand 1; PD-L2: programed death ligand 2; H: heart; K: kidney; LU: lung; P: pancreas; LI: liver; DLBCL: diffuse large B-cell lymphoma; NGC: non-germinal center; GC: germinal center; Aggressive B: aggressive B-cell lymphoma, unclassifiable; Aggressive T: aggressive T-cell lymphoma, unclassifiable; ALCL: anaplastic large cell lymphoma; EATL: enteropathy-associated T-cell lymphoma; HSTL: hepatosplenic T-cell lymphoma; LBTL: lymphoblastic lymphoma; PTCL-NOS: peripheral T-cell lymphoma, unspecified; L-UNC: lymphoma, unclassifiable; +: positive; –: negative; MD: missing data.
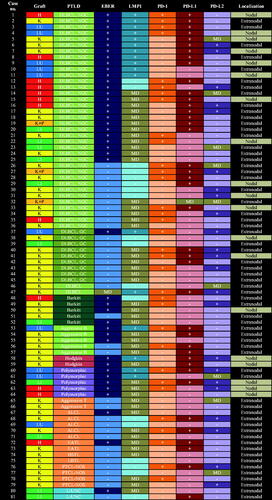
Regarding the degree of positivity, 73% (29/40) of PD-L1+ and 78% (18/23) of PD-L2+ PTLDs showed expression in >30% of the tumor cells ( and ). In PD-L1 negative cases, the microenvironment of the tumor was assessed for an expression of PD-L1 exceeding 20% of the cells as done in previous studies, but this was not observed in any of the 37 evaluable cases.
Figure 3. Immunohistochemical stainings of PD-1, PD-L1, and PD-L2 in PTLD. First row: Anaplastic large cell lymphoma (ALCL) positive in all three stainings, with a membranous staining pattern for PD-1(A) and PD-L1 (B), and a cytoplasmic and nuclear pattern for PD-L2 (C); Second row: Diffuse large B-cell lymphoma (DLBCL) of non-germinal center (non-GC) type with a membranous staining pattern for PD-1 (D) and PD-L1 (E), but negative for PD-L2 (F); Third row: DLBCL of GC type positive for PD-1 in a membranous pattern (G), with <5% of the tumor cells positive for PD-L1, therefore regarded as PD-L1 negative (H), but PD-L2 positive in a cytoplasmic and nuclear pattern (I); Fourth row: DLBCL of non-GC type negative for PD-1 (J), but positive for PD-L1 in a membranous (K) and PD-L2 in a cytoplasmic pattern (L).
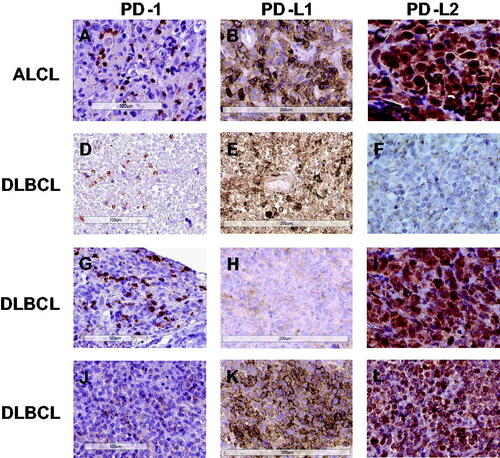
Table 2. Expression of PD-1, PD-L1, and PD-L2 in PTLD tissue.
Table 3. Combinations of expression of PD-1, PD-L1, and PD-L2 by subtype and EBV-status of PTLD.
The expression of PD-1, -L1, and -L2 by subtype and EBV-status of PTLD is presented in , , and . Both Hodgkin lymphomas were PD-L1+ and PD-1–. All five Burkitt lymphomas were PD-L1–, which seemed more frequent than other PTLD subtypes (100% vs. 47%, p = .05). There was a trend that non-GC DLBCL, compared with GC DLBCL, was associated with expression of PD-L1 (63% vs. 33%, p = .14). LMP1+ PTLD tended more frequently to be PD-L1+ than LMP1– cases (76% vs. 44%, p = .09), whereas there was no difference in the expression of PD-1 or PD-L2 between LMP1+ and LMP1– PTLD. Expression of EBER or intratumoral Tregs was not significantly associated with the detection of PD-1 or its ligands. No other PTLD subtype differed in their expression of PD-1, -L1, or -L2.
PTLDs with a PD-1+ microenvironment were more frequent in recipients of hearts compared with recipients of other transplants (75% vs. 35%, p = .01). PD-L1+ PTLD was more rarely found in the blood or bone marrow (3% vs. 28%, p < .01) and was associated with a lower presentation stage (71% vs. 37% stage I–III, p < .01) compared with PD-L1– cases. No other significant associations between the expression of PD-1, -L1, and -L2 and clinical features or overall survival were found.
Discussion
In this large nationwide study of PTLD following solid organ transplantation, we confirm that about two-thirds of PTLDs express either PD-1 or its ligands as previously indicated by small case series. PD-L1 was expressed in 50% of our 81 PTLDs, which is in line with the frequency found in three previous case series of PTLD comprising 8 (frequency not shown), 26 (73%), and 17 (60%) cases, respectively [Citation14–16]. In a study by de Jong et al. [Citation17], all 19 PTLDs were reported to be PD-L1+. Generally, the choice of the antibody may affect the result. However, in this study we have used the same anti PD-L1 antibody (clone E1L3N; Cell Signaling Technology) as the study by de Jong et al. that had the most divergent result [Citation17], whereas the other three studies have used four other anti PD-L1 antibodies (clone SP263, Roche, clone 29E.2A3, BioLegend, clone 15, Sino Biological, and clone 339.7G11; Dana-Farber Cancer Institute) [Citation14–16]. We have used clone E1L3N in other studies and are confident with its performance [Citation24]. The anti PD-1 antibody (clone NAT105; Abcam) in our present study has been used in studies of DLBCL and Hodgkin lymphoma in immunocompetent individuals [Citation22,Citation24]. NAT 105 has also been used in the study of PTLD by Morscio et al. [Citation14]; PD-1+ cells were rare but no data were shown. None of the other above mentioned studies of PTLD has investigated the expression of PD-1 and only de Jong et al. studied PD-L2. They found a slightly lower percentage of PD-L2+ PTLDs than we did (2/9 vs. 23/72) but with the use of another antibody (clone D7U8C; Cell Signaling Technology) [Citation17]. The anti PD-L2 antibody (clone HPA 013411; Atlas Antibodies) that we used has been used to demonstrate the prognostic impact of PD-L2 expression in lung cancer [Citation25].
Another factor that may influence the result is the composition of PTLD subtypes in the study. EBV has been shown to induce PD-L1 in a subset of Hodgkin lymphomas, PTLDs, and EBV-transformed lymphoblastoid cell lines [Citation15]. The mechanism for this is that LMP1, which is the major oncogene of EBV, promotes Activator Protein 1 (AP1) and Janus kinase/Signal Transducer and Activator of Transcription (JAK/STAT) signaling leading to increased PD-L1 promoter activity. In our case series, PD-L1+ PTLD tended more often to be LMP1+, which fits well with the mechanism described above. However, expression of LMP1 was not associated with detection of PD-1 or PD-L2. Neither was EBV-status of PTLD when analyzed by EBER ISH, which is the golden standard for detection of latent EBV infection, associated with expression of PD-1, -L1, or -L2 in accordance with previously published small case series on PD-L1 and -L2 expression in EBV + and EBV– PTLD [Citation16,Citation17]. Burkitt lymphomas express, in contrast to most PTLDs, a minimum of EBV gene products and are thus LMP1 negative. All five Burkitt PTLDs in our study were PD-L1–, which adds evidence to the previously reported eight cases of EBV + Burkitt lymphomas that Burkitt PTLDs are consistently PD-L1 negative [Citation16].
In Hodgkin lymphomas, genetic aberrations within the 9p24.1 locus leading to overexpression of PD-L1 and PD-L2 are frequently observed [Citation26]. Genetic alternations of 9p24.1 may also be a common feature in posttransplant DLBCL according to recent case series [Citation17,Citation27]. Ferreiro et al. found that two of five EBV + PTLDs had amplification of 9p24.1 [Citation27]. de Jong et al. observed that seven of eight evaluated posttransplant DLBCL showed either polysomy, copy gain, amplification, or chromosomic rearrangements at the PD-L1/PD-L2 locus in both EBV + and EBV– cases [Citation17]. This may be a shared pathogenetic mechanism for DLBCL associated with immunodeficiency, which is shown to be PD-L1+ to a much higher extent than DLBCL in immunocompetent individuals; ∼60–100% vs. 11% [Citation15–17,Citation22]. In DLBCL in immunocompetent individuals, the PD-L1 expression is associated with non-GC type [Citation22] and likewise in our case series of PTLD, non-GC DLBCL, compared with GC DLBCL, tended more frequently to be PD-L1+.
Infiltration of Tregs in the microenvironment of tumors is another identified immune escape mechanism besides the upregulation of PD-L1. We have previously reported that Tregs were rare in PTLD tissue and did not influence survival [Citation20]. In this case series, there was no association between expression of intratumoral Tregs and PD-1 or its ligands.
An unexpected finding was that PTLDs with a PD-1+ microenvironment were overrepresented in recipients of heart transplants. One would rather expect that heart recipients who generally receive heavier immunosuppression compared with kidney recipients would have less numerous activated infiltrating immune cells. We can only speculate that the distribution of PTLD subtypes may in part explain this difference, for example, the large proportion of non-GC DLBCL (67%) in this subgroup. Another theory is that different allografts may have different immunogenicity [Citation28].
Given the promising results with PD-1 checkpoint inhibitors in Hodgkin lymphoma [Citation8–10] and to a lesser degree also in DLBCL and T-cell lymphoma in immunocompetent individuals [Citation11,Citation12] one would consider its use in refractory or relapsed PTLD bearing in mind the high frequency of PD-1, -L1, and -L2 expression. However, there is limited experience, if any, of treatment of PTLD with PD-1 checkpoint inhibitors in solid organ recipients. In contrast, there is a growing number of case reports of the use of PD-1 inhibitors for skin cancers, mainly metastatic melanoma, in solid organ recipients, which appears to be effective but associated with a high frequency of acute allograft rejection [Citation28–31]. Four of eight reported cases of solid organ recipients who received PD-1 inhibitors experienced graft rejection that did not respond to immunosuppressive therapy and thus resulted in graft loss [Citation28]. In addition, after allogeneic hematopoietic stem cell transplantation, the use of PD-1 inhibitors is hampered by a high risk of complications in the form of severe GVHD [Citation32,Citation33]. Further studies of the safety of PD-(L)1 inhibitors in transplant recipients are warranted. However, in circumstances when an allograft loss would be acceptable, for example in a kidney recipient that may return to dialysis, and when the therapy is assumed to be life-saving there may be a therapeutic place for PD-(L)1 inhibitors in PTLD.
Some limitations should be noted when interpreting this work. First, immunostainings for PD-L2 were difficult to interpret due to either weak staining or unspecific staining of the background. Thus, some of the cases reported as PD-L2+ may be false positive and some cases judged as unspecific staining may be false negative. Second, although this is a nationwide case series gathering PTLDs from three decades, the sample size may be too small to detect differences between groups, such as LMP1+ vs. LMP1– PTLD and GC vs. non-GC DLBCL. Nevertheless, this is the largest study of the expression of PD-1 and its ligands in PTLD to date, with well-characterized PTLDs and detailed clinical data.
In conclusion, following solid organ transplantation two-thirds of PTLDs were positive for either PD-1 or its ligands. LMP1+ PTLD and non-GC DLBCL-type of PTLD tended to be PD-L1 positive more frequently. PD-L1 negativity, on the other hand, was associated with Burkitt-type of PTLD and localization in the bone marrow. Heart recipients had more frequently PTLDs with a PD-1+ microenvironment for unknown reasons. Although the high frequency of PD-1/-L1 expression in PTLD in solid organ recipients may indicate a potential for response to PD-(L)1 blockade, extreme caution should be taken regarding the use in this patient group considering the putative high risk of allograft rejection.
Potential conflict of interest
Disclosure forms provided by the authors are available with the full text of this article online at https://doi.org/10.1080/10428194.2018.1480767.
Acknowledgements
This work was supported by the Lion’s Cancer Research Foundation, the Uppsala-Örebro Regional Research Council, and the Swedish Cancer Society.
References
- Parker A, Bowles K, Bradley JA, et al. Diagnosis of post-transplant lymphoproliferative disorder in solid organ transplant recipients - BCSH and BTS Guidelines. Br J Haematol. 2010;149:675–692.
- Reshef R, Vardhanabhuti S, Luskin MR, et al. Reduction of immunosuppression as initial therapy for posttransplantation lymphoproliferative disorder. Am J Transplant. 2011;11:336–347.
- Evens AM, David KA, Helenowski I, et al. Multicenter analysis of 80 solid organ transplantation recipients with post-transplantation lymphoproliferative disease: outcomes and prognostic factors in the modern era. J Clin Oncol. 2010;28:1038–1046.
- Trappe R, Oertel S, Leblond V, et al. Sequential treatment with rituximab followed by CHOP chemotherapy in adult B-cell post-transplant lymphoproliferative disorder (PTLD): the prospective international multicentre phase 2 PTLD-1 trial. Lancet Oncol. 2012;13:196–206.
- Haque T, Wilkie GM, Jones MM, et al. Allogeneic cytotoxic T-cell therapy for EBV-positive posttransplantation lymphoproliferative disease: results of a phase 2 multicenter clinical trial. Blood. 2007;110:1123–1131.
- Kinch A, Baecklund E, Backlin C, et al. A population-based study of 135 lymphomas after solid organ transplantation: the role of Epstein-Barr virus, hepatitis C and diffuse large B-cell lymphoma subtype in clinical presentation and survival. Acta Oncol. 2014;53:669–679.
- Luskin MR, Heil DS, Tan KS, et al. The impact of EBV status on characteristics and outcomes of posttransplantation lymphoproliferative disorder. Am J Transplant. 2015;15:2665–2673.
- Ansell SM, Lesokhin AM, Borrello I, et al. PD-1 blockade with nivolumab in relapsed or refractory Hodgkin’s lymphoma. N Engl J Med. 2015;372:311–319.
- Armand P, Shipp MA, Ribrag V, et al. Programmed death-1 blockade with pembrolizumab in patients with classical Hodgkin lymphoma after brentuximab vedotin failure. J Clin Oncol. 2016;34:3733–3739.
- Chen R, Zinzani PL, Fanale MA, et al. Phase II Study of the efficacy and safety of pembrolizumab for relapsed/refractory classic Hodgkin lymphoma. J Clin Oncol. 2017;35:2125–2132.
- Armand P, Nagler A, Weller EA, et al. Disabling immune tolerance by programmed death-1 blockade with pidilizumab after autologous hematopoietic stem-cell transplantation for diffuse large B-cell lymphoma: results of an international phase II trial. J Clin Oncol. 2013;31:4199–4206.
- Lesokhin AM, Ansell SM, Armand P, et al. Nivolumab in patients with relapsed or refractory hematologic malignancy: preliminary results of a Phase Ib study. J Clin Oncol. 2016;34:2698–2704.
- Mahoney KM, Rennert PD, Freeman GJ. Combination cancer immunotherapy and new immunomodulatory targets. Nat Rev Drug Discov. 2015;14:561–584.
- Morscio J, Finalet Ferreiro J, Vander Borght S, et al. Identification of distinct subgroups of EBV-positive post-transplant diffuse large B-cell lymphoma. Mod Pathol. 2017;30:370–381.
- Green MR, Rodig S, Juszczynski P, et al. Constitutive AP-1 activity and EBV infection induce PD-L1 in Hodgkin lymphomas and posttransplant lymphoproliferative disorders: implications for targeted therapy. Clin Cancer Res. 2012;18:1611–1618.
- Chen BJ, Chapuy B, Ouyang J, et al. PD-L1 expression is characteristic of a subset of aggressive B-cell lymphomas and virus-associated malignancies. Clin Cancer Res. 2013;19:3462–3473.
- de Jong D, Roemer MG, Chan JK, et al. B-cell and classical Hodgkin lymphomas associated with immunodeficiency: 2015 SH/EAHP Workshop Report-Part 2. Am J Clin Pathol. 2017;147:153–170.
- Swerdlow SH, Campo E, Pileri SA, et al. The 2016 revision of the World Health Organization classification of lymphoid neoplasms. Blood. 2016;127:2375–2390.
- Hans CP, Weisenburger DD, Greiner TC, et al. Confirmation of the molecular classification of diffuse large B-cell lymphoma by immunohistochemistry using a tissue microarray. Blood. 2004;103:275–282.
- Berglund D, Kinch A, Edman E, et al. Expression of intratumoral forkhead box protein 3 in posttransplant lymphoproliferative disorders: clinical features and survival outcomes. Transplantation. 2015;99:1036–1042.
- Kampf C, Olsson I, Ryberg U, et al. Production of tissue microarrays, immunohistochemistry staining and digitalization within the human protein atlas. J Vis Exp. 2012;(63):3620. doi: 10.3791/3620.
- Kiyasu J, Miyoshi H, Hirata A, et al. Expression of programmed cell death ligand 1 is associated with poor overall survival in patients with diffuse large B-cell lymphoma. Blood. 2015;126:2193–2201.
- Kwon D, Kim S, Kim PJ, et al. Clinicopathological analysis of programmed cell death 1 and programmed cell death ligand 1 expression in the tumour microenvironments of diffuse large B cell lymphomas. Histopathology. 2016;68:1079–1089.
- Hollander P, Kamper P, Smedby KE, et al. High proportions of PD-1(+) and PD-L1(+) leukocytes in classical Hodgkin lymphoma microenvironment are associated with inferior outcome. Blood Adv. 2017;1:1427–1439.
- Zhang Y, Wang L, Li Y, et al. Protein expression of programmed death 1 ligand 1 and ligand 2 independently predict poor prognosis in surgically resected lung adenocarcinoma. Onco Targets Ther. 2014;7:567–573.
- Green MR, Monti S, Rodig SJ, et al. Integrative analysis reveals selective 9p24.1 amplification, increased PD-1 ligand expression, and further induction via JAK2 in nodular sclerosing Hodgkin lymphoma and primary mediastinal large B-cell lymphoma. Blood. 2010;116:3268–3277.
- Ferreiro JF, Morscio J, Dierickx D, et al. EBV-positive and EBV-negative posttransplant diffuse large B cell lymphomas have distinct genomic and transcriptomic features. Am J Transplant. 2016;16:414–425.
- Kittai AS, Oldham H, Cetnar J, et al. Immune checkpoint inhibitors in organ transplant patients. J Immunother. 2017;40:1–281.
- Ong M, Ibrahim AM, Bourassa-Blanchette S, et al. Antitumor activity of nivolumab on hemodialysis after renal allograft rejection. J Immunotherapy Cancer. 2016;4:64.
- Lipson EJ, Bagnasco SM, Moore J, Jr, et al. Tumor regression and allograft rejection after administration of anti-PD-1. N Engl J Med. 2016;374:896–898.
- Alhamad T, Venkatachalam K, Linette GP, et al. Checkpoint inhibitors in kidney transplant recipients and the potential risk of rejection. Am J Transplant. 2016;16:1332–1333.
- Haverkos BM, Abbott D, Hamadani M, et al. PD-1 blockade for relapsed lymphoma post-allogeneic hematopoietic cell transplant: high response rate but frequent GVHD. Blood. 2017;130:221–228.
- Herbaux C, Gauthier J, Brice P, et al. Efficacy and tolerability of nivolumab after allogeneic transplantation for relapsed Hodgkin lymphoma. Blood. 2017;129:2471–2478.