Abstract
Most myelodysplastic syndromes (MDS)-patients receive multiple red blood cell transfusions (RBCT). Transfusions may cause iron-related toxicity and mortality, influencing outcome after allogeneic HSCT. This prospective non-interventional study evaluated 222 MDS and CMML patients undergoing HSCT. Overall survival (OS), relapse-free survival (RFS), non-relapse mortality (NRM), and relapse incidence (RI) at 36 months were 52%, 44%, 25%, and 31%, respectively. Age, percentage of marrow blasts and severe comorbidities impacted OS. RFS was significantly associated with RBCT burden prior to HSCT (HR: 1.7; p = .02). High ferritin levels had a significant negative impact on OS and RI, but no impact on NRM. Administration of iron chelation therapy prior to HSCT did not influence the outcome, but early iron reduction after HSCT (started before 6 months) improved RFS significantly after transplantation (56% in the control group vs. 90% in the treated group, respectively; p = .04). This study illustrates the impact of RBCT and related parameters on HSCT-outcome. Patients with an expected prolonged survival after transplantation may benefit from early iron reduction therapy after transplantation.
Introduction
Allogeneic hematopoietic stem cell transplantation (HSCT) is the most potent curative therapeutic option in patients with myelodysplastic syndromes (MDS) [Citation1]. Due to high treatment-related morbidity and mortality, combined with a high relapse risk, the current long-term overall survival rate is around 35% [Citation2–4]. Non-relapse mortality (NRM) has decreased after the introduction of reduced intensity regimens (RIC) for the elderly and more frail patients with MDS [Citation5,Citation6]. Many parameters such as age, comorbidity, advanced disease stage, and cytogenetic risk category according to the (revised)-International Prognostic Scoring system (IPSS-R) have been reported to impact treatment outcome [Citation4,Citation7–10]. Around 80% of patients with MDS present with anemia and many of these patients receive multiple red blood cell transfusions (RBCT) during the course of their disease [Citation11]. RBCT and associated iron overload may cause toxicity and mortality, and influence outcome after HSCT [Citation12–15]. Most studies are retrospective and selection bias and lack of detailed data about interventions, outcome, and potentially confounding risk factors might have influenced conclusions.
The Chronic Malignancies Working Party (CMWP) of the EBMT performed a large prospective, non-interventional study to evaluate prognostic pre-transplant factors in MDS and chronic myelomonocytic leukemia (CMML). The primary objective of this study was to evaluate the relation between iron toxicity and treatment-related mortality after allogeneic HSCT in adult MDS and CMML patients. We excluded patients treated with intensive chemotherapy from this study, because previous intensive chemotherapy is expected to have an impact on important pre-HSCT co-variables, including advanced stages of disease (relapsed or refractory), more co-morbid conditions and poorer general condition. This approach is expected to be associated with a reduced pretreatment related morbidity, and a reduction of the interval between diagnosis and HSCT. Secondary objectives were (i) description of treatment outcomes defined by NRM, OS, relapse incidence (RI) and relapse-free survival (RFS); (ii) impact of RBCT and surrogate iron markers that may reflect iron burden on treatment outcome. The final objective was to evaluate the impact of iron reduction therapy prior and post HSCT on the outcome after HSCT.
Patients and methods
Two hundred twenty-two adult (age ≥18 years at HSCT) patients with de-novo MDS, AML-MDS with 20–30% marrow blasts (formerly RAEBt) or patients with CMML who received upfront HSCT after bone marrow ablative or reduced intensity regimens were included prospectively between January 2009 and January 2014. Patients had an ECOG performance status between 0 and 2. Exclusion criteria included previous intensive anti-leukemic chemotherapy, patients with juvenile CMML, patients with therapy-related MDS, AML, or CMML after treatment with immunosuppressive or cytotoxic treatment for a non-myeloid malignancy, patients who had received auto-HSCT, candidates for cord blood HSCT or syngeneic HSCT, inadequate renal function (ECC <60 ml/min and/or creatinine >2.5 times upper limit of normal value), inadequate hepatic function (transaminases >2.5 times upper limit of normal value), history of seizures, pregnancy, and women of child-bearing potential and not using adequate contraceptives, uncontrolled hypertension. The research was conducted according to the Declaration of Helsinki and written informed consent was obtained from each participating patient. Trial registered at Clinicaltrial.gov Identifier: 842205547.
Retrospective data were collected by survey at diagnosis, and prospectively at transplantation and 6 weeks, 100 days, 6 months, 1 year, and annually thereafter. Missing data were collected by follow-up surveys. Based on the study objectives the following variables were collected: age, gender, comorbidities, WHO classification, cytogenetic characteristics according to IPSS-R, number of transfusions, ferritin levels, hemoglobin levels, C-reactive protein (CRP), donor type, female-donor/male recipient vs. other combinations, interval diagnosis, and HSCT, intensity of HSCT conditioning, acute and chronic graft vs. host disease (GvHD), and data concerning treatment with iron chelation or phlebotomy.
Primary end-points were overall survival (OS), relapse-free survival (RFS), relapse incidence (RI), and non-relapse mortality (NRM), evaluated at 36 months after transplant. The median follow-up was estimated using the reverse Kaplan–Meier method. Additionally, acute GvHD grade I–II and III–IV, and limited and extensive chronic GvHD were evaluated at 100 days and 36 months after transplant, respectively. OS and RFS were estimated using the Kaplan–Meier product limit estimation method, and differences in subgroups were assessed by the Log-Rank test. Cumulative incidences of relapse and NRM, were analyzed in a competing risks framework. The cumulative incidences of acute GvHD grade I–II and III–IV were estimated as competing risks, considering as competing for event death before aGvHD. The cumulative incidences of limited and extensive cGvHD were estimated equivalently. Subgroup differences were assessed by Gray’s test. Cox proportional hazards regression was used to assess the impact of potential risk factors in univariable and multivariable analyses. Multivariable analyses of RI and NRM were performed using Cox cause-specific hazards models. The impact of post-transplant iron reduction therapy was investigated using landmark analyses at 6, 12 and 24 months. The landmark population was defined as patients alive (event = death) and event-free (event = death and/or relapse) at the respective landmark time points. All p values were two-sided and p < .05 was considered significant.
Results
Demographic and transplantation data
The study included 181 patients with MDS, 16 patients with AML-MDS, and 25 patients with CMML from 29 European transplant centers (for details, see ). The median age was 59 years (range 19–76 years). WHO-classification at time of transplantation resulted in 20 patients with RA/RARS, 36 with RCMD/RCMD-RS, 1 with 5q-, 50 with RAEB-1, 74 with RAEB-2, 25 with CMML, and 16 with AML-MDS. In total, 70% of the patients had received RBCT prior to HSCT. Median number of RBC units transfused before transplantation: 12 units (range: 1–146 units); median ferritin levels: 700 ng/ml (range 8–9033 ng/ml). Median interval between diagnosis and HSCT was 10 months (range 1–128 months). Time between diagnosis and HSCT was <6 months for 56 patients and ≥6 months for 166 patients. A total of 99 patients received standard conditioning (MAC) and 123 received a reduced intensity (RIC) regime. After conditioning, 70 patients received stem cells from a sibling, 4 from a mismatched relative, and 148 patients from an unrelated donor. The incidence of acute GvHD grade I–II and grade III–IV at 100 days was 1% (25–37%) and 14% (9–18%), respectively. The incidence of limited or extensive chronic GvHD at 36 months was 47% (40–54%) and 20% (15–26%), respectively.
Table 1. Demographic data and estimates of survival outcomes and cumulative incidences.
Univariable analysis of relevant factors on primary end points
The median follow-up of the 222 registered patients after HSCT was 39 months with 95% confidence intervals (CI) ranging from 36 to 44 months. OS, RFS, NRM, and RI at 36 months were 52% (95% CI: 45–59%), 44% (95% CI: 37%–51%), 25% (95% CI: 19%–32%) and 31% (95% CI: 24%–37%), respectively. Age had a significant impact on OS when analyzed as continuous variable per decade (HR: 1.4, 95%CI: 1.1–1.8; p = .002), and on RFS (HR: 1.4, 1.1–1.8; p = .002), but a borderline impact on NRM (HR: 1.4, 1.0–1.8; p = .05) and on relapse incidence (HR: 1.3, 1.0–1.7; p = .07). To evaluate the impact of WHO-classification on treatment outcome, patients were divided in 4 subgroups: patients with 5% marrow blasts or less at time of HSCT (RA, RARS, RCMD, RCMD-RS, 5q-), patients with 6–19% marrow blasts (RAEB1 and RAEB2), patients with 20–29% marrow blasts (AML-MDS) and patients with CMML. The WHO classification influenced significantly OS at 3 years (p = .002) (, ). Cytogenetics according to IPSS-R categories had no significant impact on treatment outcome. However, the group with very poor-risk cytogenetics could not be analyzed separately due to its small size (n = 9) (). Only 11 patients were transplanted with bone marrow as stem cell source, precluding an analysis of the impact of stem cell source. Transfusion burden (≤20 RBCT vs. >20 RBCT) did not have a significant impact on any of the outcome parameters. Ferritin levels (>1000 ng/ml) had a non-significant negative impact on NRM and OS after HSCT, but elevated CRP levels (>10 mg/l) influenced OS significantly (p = .02).
Correlations of potentially confounding factors for the multivariable analyses
Patients with more than 5% bone marrow blasts received a low number of red blood cell units (0–20 RBCT) before HSCT more frequently than patients with less than 5% bone marrow blasts (p = .01), mainly explained by a shorter duration of the interval between diagnosis and HSCT in patients with more advanced MDS according to WHO-classification (data not shown). The number of RBCT before HSCT was not associated with the cytogenetic risk category according to IPSS-R (p = .7) nor with the interval between diagnosis and actual HSCT of the whole cohort, including patients with less than 5% marrow blasts (<6 vs. >6 months) (p = .2). As expected, ferritin levels (>1000 ng/ml vs. <1000 ng/ml) were influenced by a number of administered RBCT prior to HSCT (p < .001). Ferritin levels, measured as units of 1000 mg/L were associated with elevated CRP levels (p = .03) in this study. Co-morbidities and intensity conditioning (MAC vs. RIC) were significantly associated (p < .001).
Multivariable cox models
The basic model was restricted to the traditional variables indicating a potential prognostic impact, according to the literature and the results from the univariable analyses (). Therefore, only WHO-classification, age at HSCT, donor type, sex-match, and intensity of conditioning regimen were included in the model. We added one by one the other variables of specific interest in the current study: RBC transfusions, CRP levels, ferritin levels (continuous in units of 1000 ng/ml) and comorbidities. This approach was necessary due to the highly correlated nature of these variables. The transfusion burden prior to HSCT influenced RFS significantly (HR: 1.7; p = .02). The impact of pre-HSCT ferritin levels was minor but significant on OS (HR: 1.2; p = .05) and RI (HR: 1.3; p = .04). Presence of severe comorbidities influenced OS after HSCT (HR: 1.8; p = .04). Elevated CRP-levels (>10 mg/L) had a borderline negative impact on survival (HR: 1.6; p = .06).
Table 2. Multivariate Cox models for assessment of the impact of relevant factors for all major outcomes.
Impact of iron chelation before HSCT on outcome
Thirty-one (14%) patients received iron chelation prior to HSCT and ferritin levels at HSCT were available in 28 patients of them. The median duration of chelation prior to HSCT was 4 months (range: 0–40 months). The median ferritin levels at HSCT was 1598 ng/ml, 9 chelated patients had ferritin levels <1000 ng/ml at the time of HSCT. Sixteen chelated patients had received >20 units of RBCT prior to HSCT. The outcome of the patients chelated prior to transplant conditioning was compared with a non-chelated control group of 70 patients who had ferritin levels >1000 ng/ml at HSCT or who had received >20 units of RBCT prior to HCT conditioning. The overall 3-year survival in the chelated group was 44% (21–67%) similar to the survival in the control group, 45% (33–57%, p = .6; ). The RFS, RI, and NRM were also similar in both groups: 33% (13–54%) in the chelated patients vs. 38% (26–50%) in the non-chelated patients, 34% (15–53%) vs. 33% (22–44%), and 32% (13–53%) vs. 29% (18–40%), respectively ().
Figure 2. Overall survival (A) and relapse-free survival (RFS) according to iron reduction therapy prior to Transplantation (B). Kaplan–Meier plot for OS (A) and RFS (B) in patients who either received chelation therapy or could have received chelation therapy based on their increased ferritin levels or received transfusions, prior to transplant. The corresponding 95% confidence intervals are indicated by the gray regions.
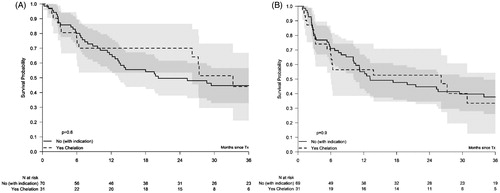
Impact of phlebotomies or iron chelation after HSCT on outcome
Twenty patients have been treated with phlebotomies after HSCT. Most patients (80%) started phlebotomies during the first year after HSCT. The median ferritin levels before starting phlebotomies were: 3134 ng/ml (range: 937–9985 ng/ml). The number of phlebotomies was available in 16 patients: 9 patients underwent <10 phlebotomies and 7 patients 10 or more phlebotomies. The ferritin levels decreased to levels <1000 ng/ml in 10 out of 19 patients at the time of completion of phlebotomies or at 24 months after HSCT and decreased more than 50% in five additional patients. In the remaining 5 patients, the effect was not evaluable mainly due to insufficient follow-up after starting phlebotomies.
Sixteen patients have received iron chelation (deferasirox only) after HSCT. Nine patients started chelators during the first 6 months after HSCT and 13 patients during the first year after HSCT. The median interval between HSCT and starting chelation was 5 months (range: 1–18). The median ferritin level before starting chelation was: 3122 ng/ml (range: 69–9040 ng/ml). The median duration of iron chelation was 4 months (range: 0.5–40 months). The ferritin levels decreased to levels <1000 ng/ml in 3 out of 16 patients during iron chelation and decreased more than 50% in four additional patients. In the remaining patients, the effect was not evaluable due to missing ferritin levels (5 patients) or too short duration of chelation (7 patients). The median duration of iron chelation in the 5 responding patients was 4 months (range: 1.5–7 months).
We combined the data of both interventions, because the number of patients who were treated with either chelation or phlebotomies was relatively small, and both interventions are aiming at reducing the iron overload. Fourteen patients started treatment with either phlebotomies or chelation during the first 6 months after HSCT, and 27 patients started these interventions within the first 12 months after HSCT. The outcome of the patients treated with iron reductive interventions was compared with the untreated control groups with ferritin levels >1000 ng/ml alive and relapse-free at the landmarks of 6, 12 and 24 months, respectively ( and ). Only patients who received iron reductive therapy within 6 months after HSCT had a significantly improved 3-year RFS compared to the non-treated patient population (90% (71–100%) vs. 56% (46–67%); p = .04 , and ). The survival at this landmark analysis was reduced compared to the control group with a 3-year OS of 90% (71–100%) vs. 65% (54–75%) in the control group ( and ). The outcomes at later landmark analyses did not indicate relevant differences with the exception of a moderate difference of the NRM at both landmarks ( and ).
Figure 3. Overall survival and relapse-free survival of patients alive and relapse-free at 6 months after transplantation, stratified in 2 groups according to iron reduction therapy given during the first 6 months after transplantation or not. Landmarked Kaplan–Meier plot for OS and RFS in patients who either received iron reduction therapy or could have received iron reduction therapy based on their increased ferritin levels or received transfusions. Patients were selected based on ferritin levels >1000 ng/ml. The landmark time-point is indicated by the vertical dashed line. Only patients alive and relapse-free at 6 months are included. The corresponding 95% confidence intervals are indicated by the gray regions.
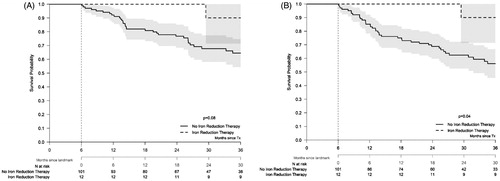
Table 3. Outcome of patients with iron reduction treatment after HSCT.
Table 4. Landmark population: alive and relapse free.
Discussion
Allogeneic hematopoietic stem cell transplantation (HSCT) is considered the most potent curative option in myelodysplastic syndromes (MDS). Many retrospective analyses evaluated relevant prognostic parameters, to enable adequate patient selection for treatment [Citation16,Citation17] and reviewed in [Citation18]. This large prospective observational study collected detailed information on several relevant prognostic factors, including relevant patient characteristics, disease modalities, transfusion data, and iron parameters in patients with MDS undergoing HSCT. The overall outcome of this study, and especially the NRM of 25% (95% CI: 19%–32%) at 36 months, compares favorably with other recently published studies [Citation4,Citation6,Citation10] despite the high mean age of 59 years in this study. Age, BM blast percentage, and comorbidity at time of HSCT had a significant influence on outcome after HSCT. The overall outcome of this study, and especially the NRM at 36 months, compares favorably with other recently published studies reflecting the data of this study showed that intensity of conditioning regimen had no impact on overall survival, confirming several other retrospective studies [Citation5,Citation6,Citation19,Citation20].
The primary objective of this study was to study the impact of iron overload and iron toxicity on outcome after HSCT. As expected [Citation21], the data showed a significant association between transfusion burden and ferritin levels (p < .001). A high RBCT burden, higher than 20 units prior to transplantation, was significantly associated with a decreased relapse-free survival (HR: 1.7; p = .02). Although it is difficult to separate the impact of transfusion load as an independent prognostic marker from the progression of bone marrow failure and increased tumor burden in nontransplanted MDS patients, several studies showed a significant impact of transfusion burden on outcome after HSCT [Citation17,Citation22,Citation23], including our own retrospective study [Citation24]. In this study, the impact of high transfusion burden on overall survival was less explicit (HR: 1.5; p = .09), in contrast to the significantly reduced RFS, which can be explained by the smoldering nature of less advanced MDS patients in this cohort (only 16 patients had AML-MDS at time of transplantation). This selection may be associated with a prolonged survival after relapse.
Ferritin is an acute-phase protein, and therefore also elevated in case of infection, inflammation or high tumor burden [Citation25]. To correct for this confounding factor we analyzed CRP levels, another acute phase protein, which was significantly correlated with ferritin levels. Both ferritin levels and elevated CRP levels had an impact on overall survival, but only ferritin levels had a significant impact on relapse incidence reflecting its association with more advanced MDS stages. Therefore, the impact of elevated CRP levels on NRM was more pronounced compared to the impact of elevated ferritin levels, confirming data from a large HSCT study in AML and MDS [Citation26]. Ferritin levels are sometimes used as a marker of transfusion burden [Citation27], but in the multivariable analysis of that study, only co-morbidities and percentage of marrow blasts at time of HSCT predicted OS [Citation27]. Therefore, it has been postulated that transferrin saturation, NTBI, and LPI are better parameters to monitor and to study iron toxicity during conditioning for HSCT and the first two weeks after HSCT [Citation28]. When transferrin saturation exceeds 85%, species of non-transferrin-bound iron (NTBI), and its redox active component labile plasma iron (LPI), become detectable in the plasma [Citation29,Citation30]. Transferrin becomes saturated immediately during transplant conditioning and remains elevated until engraftment [Citation30]. These redox active components lead in various steps to associated tissue damage, mostly cardiac, endocrine and liver tissue, and may also affect clonal evolution in MDS [Citation31]. Reduction of iron stores, may alleviate long-term effects of iron toxicity in transfusion-dependent patients with MDS [Citation32]. Consensus statements advice to administer iron chelation therapy in those patients with an expected long-term survival [Citation33,Citation34]. In high-risk MDS, patients may not live long enough after HSCT to be exposed to the prolonged toxic effects of transfusional therapy. Studies the impact of iron chelation in a transplant setting is ongoing [Citation35].
In the current study, we were able to investigate the impact of iron reduction therapy on outcome after HSCT. It is important to realize that both high ferritin levels and high transfusion burden were associated with an inferior outcome in this study. Both the chelated and its control group were selected based on these two poor prognostic factors. Iron overload in the transplant setting may be reduced both by iron chelation therapy [Citation36,Citation37] and by phlebotomies [Citation38]. Administration of iron chelation therapy prior to HSCT had no influence on treatment outcome in this study. However, the ferritin levels of the chelated patients at HSCT were still elevated with a median level of 1772 ng/ml, and only 8 out the 28 chelated patients with available ferritin levels had ferritin levels <1000 ng/ml at HSCT. This indicates that the duration of iron chelation was insufficient in the majority of cases, probably reflecting the short period of iron chelation prior to HSCT in this study. More efficient iron chelation prior to HSCT may be achieved by earlier iron reduction prior to the transplant procedure and/or by more vigorous iron chelation using higher dosages of chelators in the immediate pre-transplant setting [Citation33,Citation35].
Patients with MDS may also receive iron reduction interventions to reduce iron overload after HSCT [Citation39]. We were able to evaluate the influence of iron reduction therapy after HSCT in a subgroup of 35 patients, consisting of iron chelation in 16 patients and/or phlebotomies in 20 patients (one patient received bot phlebotomies and iron chelation). Iron reduction by phlebotomies started within one year after HSCT in 80% and by iron chelation in 81% of the patients receiving the respective therapies. The efficacy of reducing ferritin levels by phlebotomies was excellent with more than 50% reduction in 74% of the patients and 28% after chelation, probably reflecting the short period of chelation (median 4 months). Most events (relapse or death by any cause) occurred within the 6 months after transplantation in our study. Therefore, iron reduction therapy is expected to have more impact on the outcome, if initiated within 6 months after HSCT, as shown in our study. The patient population who received iron chelation therapy or phlebotomies within 6 months after HSCT had a significantly improved RFS compared to the non-chelated patient population with ferritin levels >1000 ng/ml or having received transfusions before HSCT (p = .04), while the OS at this landmark was moderately (p = .08) improved. Selection of the patients for either of the interventions cannot be ruled out, but the data in the supplementary table do not show remarkable differences in both groups.
In conclusion, analysis of this non-interventional prospective data from MDS patients, not pretreated with intensive chemotherapy before undergoing allogeneic HSCT, demonstrated that transfusion burden prior to HSCT influenced progression-free survival, without a significant effect on survival. Relapse-free survival may be predicted by serum ferritin levels, in contrast to CRP levels, but this effect may reflect the association with tumor burden rather than iron overload. Administration of iron reduction prior to HSCT had no impact on the primary outcome, but iron reduction therapy after HSCT increased relapse-free survival, if initiated within 6–12 months after HSCT. The predictive value of the transfusion burden prior to HSCT may be less relevant in patients with more advanced disease stages due to dominant effect of the disease stage on outcome after HSCT.
Potential conflict of interest
Disclosure forms provided by the authors are available with the full text of this article online at https://doi.org/10.1080/10428194.2019.1594215.
Supplemental Material
Download MS Word (26.8 KB)Acknowledgments
We would like to thank all centers who have participated in this study.
Additional information
Funding
References
- Gangat N, Patnaik MM, Tefferi A. Myelodysplastic syndromes: contemporary review and how we treat. Am J Hematol. 2016;91:76–89.
- Kroger N. Allogeneic stem cell transplantation for elderly patients with myelodysplastic syndrome. Blood. 2012;119:5632–5639.
- Damaj G, Mohty M, Robin M, et al. Upfront allogeneic stem cell transplantation after reduced-intensity/nonmyeloablative conditioning for patients with myelodysplastic syndrome: a study by the Societe Francaise de Greffe de Moelle et de Therapie Cellulaire. Biol Blood Marrow Transplant. 2014;20:1349–1355.
- Koenecke C, Gohring G, de Wreede LC, et al. Impact of the revised International Prognostic Scoring System, cytogenetics and monosomal karyotype on outcome after allogeneic stem cell transplantation for myelodysplastic syndromes and secondary acute myeloid leukemia evolving from myelodysplastic syndromes: a retrospective multicenter study of the European Society of Blood and Marrow Transplantation. Haematologica. 2015;100:400–408.
- Martino R, Iacobelli S, Brand R, et al. Retrospective comparison of reduced-intensity conditioning and conventional high-dose conditioning for allogeneic hematopoietic stem cell transplantation using HLA-identical sibling donors in myelodysplastic syndromes. Blood. 2006;108:836–846.
- Martino R, Henseler A, van Lint M, et al. Long-term follow-up of a retrospective comparison of reduced-intensity conditioning and conventional high-dose conditioning for allogeneic transplantation from matched related donors in myelodysplastic syndromes. Bone Marrow Transplant. 2017;52:1107–1112.
- Sorror ML, Martin PJ, Storb RF, et al. Pretransplant comorbidities predict severity of acute graft-versus-host disease and subsequent mortality. Blood. 2014;124:287–295.
- Greenberg PL, Tuechler H, Schanz J, et al. Revised international prognostic scoring system for myelodysplastic syndromes. Blood. 2012;120:2454–2465.
- Schanz J, Tuchler H, Sole F, et al. New comprehensive cytogenetic scoring system for primary myelodysplastic syndromes (MDS) and oligoblastic acute myeloid leukemia after MDS derived from an international database merge. JCO. 2012;30:820–829.
- Scheid C, de Wreede L, van Biezen A, et al. Validation of the revised IPSS at transplant in patients with myelodysplastic syndrome/transformed acute myelogenous leukemia receiving allogeneic stem cell transplantation: a retrospective analysis of the EBMT chronic malignancies working party. Bone Marrow Transplant. 2017;52:1519–1525.
- Arber DA, Orazi A, Hasserjian R, et al. The 2016 revision to the World Health Organization classification of myeloid neoplasms and acute leukemia. Blood. 2016;127:2391–2405.
- Waszczuk-Gajda A, Madry K, Machowicz R, et al. Red blood cell transfusion dependency and hyperferritinemia are associated with impaired survival in patients diagnosed with myelodysplastic syndromes: results from the first polish MDS-PALG registry. Adv Clin Exp Med. 2016;25:633–641.
- Alessandrino EP, Porta MGD, Bacigalupo A, et al. Prognostic impact of pre-transplantation transfusion history and secondary iron overload in patients with myelodysplastic syndrome undergoing allogeneic stem cell transplantation: a GITMO study. Haematologica. 2010;95:476–484.
- Armand P, Sainvil MM, Kim HT, et al. Pre-transplantation iron chelation in patients with MDS or acute leukemia and iron overload undergoing myeloablative allo-SCT. Bone Marrow Transplant. 2013;48:146–147.
- Bazuaye GN, Buser A, Gerull S, et al. Prognostic impact of iron parameters in patients undergoing allo-SCT. Bone Marrow Transplant. 2012;47:60–64.
- Santini V, Girelli D, Sanna A, et al. Hepcidin levels and their determinants in different types of myelodysplastic syndromes. PLoS One. 2011;6:e23109.
- Alessandrino EP, la Porta MG, Bacigalupo A, et al. WHO classification and WPSS predict posttransplantation outcome in patients with myelodysplastic syndrome: a study from the Gruppo Italiano Trapianto di Midollo Osseo (GITMO). Blood. 2008;112:895–902.
- de Witte T, Bowen D, Robin M, et al. Allogeneic hematopoietic stem cell transplantation for MDS and CMML: recommendations from an international expert panel. Blood. 2017;129:1753–1762.
- Lim Z, Brand R, Martino R, et al. Allogeneic hematopoietic stem-cell transplantation for patients 50 years or older with myelodysplastic syndromes or secondary acute myeloid leukemia. JCO. 2010;28:405–411.
- Festuccia M, Deeg HJ, Gooley TA, et al. Minimal identifiable disease and the role of conditioning intensity in hematopoietic cell transplantation for myelodysplastic syndrome and acute myelogenous leukemia evolving from myelodysplastic syndrome. Biol Blood Marrow Transplant. 2016;22:1227–1233.
- Sahlstedt L, Ebeling F, von Bonsdorff L, et al. Non-transferrin-bound iron during allogeneic stem cell transplantation. Br J Haematol. 2001;113:836–838.
- Pereira A, Nomdedeu M, Aguilar JL, et al. Transfusion intensity, not the cumulative red blood cell transfusion burden, determines the prognosis of patients with myelodysplastic syndrome on chronic transfusion support. Am J Hematol. 2011;86:245–250.
- Malcovati L, Germing U, Kuendgen A, et al. Time-dependent prognostic scoring system for predicting survival and leukemic evolution in myelodysplastic syndromes. JCO. 2007;25:3503–3510.
- Cremers EM, van Biezen A, de Wreede LC, et al. Prognostic pre-transplant factors in myelodysplastic syndromes primarily treated by high dose allogeneic hematopoietic stem cell transplantation: a retrospective study of the MDS subcommittee of the CMWP of the EBMT. Ann Hematol. 2016;95:1971–1978.
- Kanda J, Mizumoto C, Ichinohe T, et al. Pretransplant serum ferritin and C-reactive protein as predictive factors for early bacterial infection after allogeneic hematopoietic cell transplantation. Bone Marrow Transplant. 2011;46:208–216.
- Artz AS, Logan B, Zhu X, et al. The prognostic value of serum C-reactive protein, ferritin, and albumin prior to allogeneic transplantation for acute myeloid leukemia and myelodysplastic syndromes. Haematologica. 2016;101:1426–1433.
- Platzbecker U, Bornhauser M, Germing U, et al. Red blood cell transfusion dependence and outcome after allogeneic peripheral blood stem cell transplantation in patients with de novo myelodysplastic syndrome (MDS). Biol Blood Marrow Transplant. 2008;14:1217–1225.
- Hilken A, Langebrake C, Wolschke C, et al. Impact of non-transferrin-bound iron (NTBI) in comparison to serum ferritin on outcome after allogeneic stem cell transplantation (ASCT). Ann Hematol. 2017;96:1379–1388.
- de Swart L, Reiniers C, Bagguley T, et al. Labile plasma iron levels predict survival in patients with lower-risk myelodysplastic syndromes. Haematologica. 2018;103:69–79.
- Pullarkat V. Iron toxicity in hematopoietic stem cell transplantation: strike while the iron is labile. Acta Haematol. 2014;131:220–221.
- Hod EA, Zhang N, Sokol SA, et al. Transfusion of red blood cells after prolonged storage produces harmful effects that are mediated by iron and inflammation. Blood. 2010;115:4284–4292.
- Gattermann N, Finelli C, Della Porta M, et al. Hematologic responses to deferasirox therapy in transfusion-dependent patients with myelodysplastic syndromes. Haematologica. 2012;97:1364–1371.
- Porter JB, de Witte T, Cappellini MD, et al. New insights into transfusion-related iron toxicity: implications for the oncologist. Crit Rev Oncol Hematol. 2016;99:261–271.
- Malcovati L, Hellstrom-Lindberg E, Bowen D, et al. Diagnosis and treatment of primary myelodysplastic syndromes in adults: recommendations from the European LeukemiaNet. Blood. 2013;122:2943–2964.
- Sivgin S, Eser B. The management of iron overload in allogeneic hematopoietic stem cell transplant (alloHSCT) recipients: where do we stand? Ann Hematol. 2013;92:577–586.
- Lee JW, Kang HJ, Kim EK, et al. Effect of iron overload and iron-chelating therapy on allogeneic hematopoietic SCT in children. Bone Marrow Transplant. 2009;44:793–797.
- Jaekel N, Lieder K, Albrecht S, et al. Efficacy and safety of deferasirox in non-thalassemic patients with elevated ferritin levels after allogeneic hematopoietic stem cell transplantation. Bone Marrow Transplant. 2016;51:89–95.
- Eisfeld AK, Krahl R, Jaekel N, et al. Kinetics of iron removal by phlebotomy in patients with iron overload after allogeneic hematopoietic cell transplantation. Am J Blood Res. 2012;2:243–253.
- Sivgin S, Eser B, Bahcebasi S, et al. Efficacy and safety of oral deferasirox treatment in the posttransplant period for patients who have undergone allogeneic hematopoietic stem cell transplantation (alloHSCT). Ann Hematol. 2012;91:743–749.