Abstract
Following the observation of interleukin 3 receptor α chain (IL-3Rα; CD123) upregulation on leukemia stem cells (LSCs) almost two decades ago, targeted treatment via CD123-diptheria toxin conjugates has now been tested in patients with diverse myeloid malignancies. Targeted eradication of LSCs could result in effective treatments for many challenging diseases initiated by these cells. Consequently, considerable effort has been directed toward targeting CD123 as a potential strategy for treating patients with hematologic malignancies in which CD123 is overexpressed. However, these therapies have had limited success so far, highlighting the need for suitable criteria to identify patients who could benefit from them. Given the diversity in CD123 expression across different hematologic malignancies, understanding CD123 expression patterns and the functional pathogenetic significance is crucial. Here, we review the methodologies available for CD123 assessment and discuss the biological and clinical characteristics of patients for whom CD123-targeting therapies may have a clinical impact.
Introduction
CD123, the α chain of the interleukin (IL)-3 receptor (IL-3R), is overexpressed in cancer cells and is associated with the development of diverse myeloid and lymphoid malignancies, including acute lymphoblastic leukemia (ALL), acute myeloid leukemia (AML), blastic plasmacytoid dendritic cell neoplasm (BPDCN), hairy cell leukemia (HCL), Hodgkin lymphoma (HL), chronic myelomonocytic leukemia (CMML), and myelodysplastic syndrome (MDS) [Citation1–8]. Indeed, CD123 represents a potentially attractive biomarker for disease diagnosis and prognosis [Citation1,Citation7,Citation9,Citation10] and may serve as a marker to monitor treatment response [Citation11]. CD123 is also expressed, but not overexpressed, in normal hematopoietic stem cells, which are biologically distinct from leukemia stem cells (LSCs) [Citation1].
Additionally, CD123 has proven useful as a validated biomarker in the differential diagnosis of some hematologic malignancies, such as mature B-cell lymphoproliferative disorders, with CD123 positivity having 100% sensitivity and 87% specificity for the diagnosis of HCL [Citation12]. In pediatric patients with B-cell precursor ALL, CD123 overexpression correlated well with hyperdiploid karyotype, a frequent genetic abnormality in childhood ALL [Citation11]. CD123 is expressed in most leukemic blasts in patients with AML and ALL and serves as a measurable residual disease (MRD) monitoring tool [Citation11,Citation13]. In patients with untreated AML, CD123 expression significantly correlated with poor overall survival (OS) and failure to achieve a complete response (CR) after induction chemotherapy [Citation14].
CD123 is being investigated as a targeted therapy for hematologic malignancies [Citation15]. Indeed, the first-ever prospective study with a CD123-targeted treatment heralded as a pivotal clinical research success, is the multicenter, phase 2 registration trial of tagraxofusp (previously known as SL-401), a recombinant fusion protein consisting of human IL-3 conjugated to truncated diphtheria toxin [Citation16–18]. The outcomes of this trial led to the first approval of a CD123-targeting agent by the US Food and Drug Administration (FDA); tagraxofusp is approved for the treatment of BPDCN in adults, and pediatric patients aged 2 years and older [Citation19]. Clearly, the potential clinical use of CD123 relies on the availability of detection methods with high specificity and sensitivity for diagnosis and disease monitoring [Citation20]. Increased understanding of CD123 expression in hematologic malignancies and its role in disease development and/or maintenance would provide a clinical context and potential strategy for treating patients with a high unmet clinical need.
Literature search strategy and selection criteria
We searched the MEDLINE® Public Library of Medicine (PubMed®) database for relevant CD123 expression studies published in English between 1 January 2000 and 31 March 2020 using two search strategies: (1) all article types except reviews, among results retrieved from the search in ‘Title or Abstract’ for the following terms: ‘blastic plasmacytoid dendritic cell neoplasm,’ ‘acute myeloid leukemia,’ ‘acute lymphoblastic leukemia,’ ‘chronic myeloid leukemia,’ ‘chronic lymphocytic leukemia,’ ‘chronic myelomonocytic leukemia,’ ‘myeloproliferative neoplasms’ AND ‘CD123’ or ‘IL-3R;’ (2) all article types containing following terms anywhere in the article: ‘chronic eosinophilic leukemia,’ ‘hairy cell leukemia,’ ‘Hodgkin lymphoma,’ ‘myelodysplastic syndrome,’ ‘myelofibrosis,’ ‘myeloproliferative neoplasms,’ ‘multiple myeloma,’ ‘non-Hodgkin lymphoma,’ ‘systemic mastocytosis’ AND ‘CD123’ or ‘IL-3R.’ Retrieved studies were reviewed, and duplicate studies and patient case reports were excluded.
For clinical studies with agents targeting CD123, ClinicalTrials.gov and Embase® were searched on 24 March 2020, for all studies containing ‘CD123’ or ‘IL-3R’ terms.
Additional references were identified by the authors through searches of their own files or were selected on the basis of relevance to the scope of this review.
Prior to the publication of the manuscript, an additional search was performed using ‘CD123 targeting in hematologic malignancies’ and ‘CD123 targeting in hematological malignancies,’ to identify the most relevant original research articles published in PubMed from 31 March 2020 to 1 April 2021, focusing on the topic of this review. Additionally, ClinicalTrials.gov and Embase® were searched on 1 April 2021, to include the most recent studies containing ‘CD123’ or ‘IL-3R’ terms and to update the recruitment status of all studies.
CD123 biology
Members of the β common (βc) chain cytokine family, including IL-3, IL-5, and granulocyte-macrophage colony-stimulating factor (GM-CSF), regulate cell proliferation, survival, differentiation, and migration in addition to modulating innate immunity and inflammatory responses by binding their respective receptors (IL-3R, IL-5R, and GM-CSFR) [Citation21–24]. IL-3 and IL-3R are primarily active in immune cells [Citation22,Citation25]. IL-3 is expressed mainly by activated T lymphocytes and mast cells. The heterodimeric receptor, IL-3R, comprises the high-affinity, cytokine-specific α chain, CD123, and the common βc subunit [Citation21,Citation22,Citation24]. CD123 is the major ligand-binding subunit, while the βc subunit is responsible for receptor assembly and intracellular signaling [Citation21,Citation24]. CD123 is expressed in IL-3–responsive cells, including B lymphocytes, plasmacytoid dendritic cells (pDCs), hematopoietic stem or progenitor cells, megakaryocytes, and monocytes [Citation7].
Upon IL-3 binding to CD123, IL-3R heterodimerization promotes high-affinity ligand binding and stable IL-3/IL-3R complex assembly, leading to activation of Janus kinase (JAK)/signal transducers and activators of transcription (STAT), mitogen-activated protein kinase (MAPK), and phosphoinositide 3-kinase (PI3K)/protein kinase B (AKT) signaling pathways [Citation22,Citation23,Citation26,Citation27] () [Citation24]. This activation drives hematopoietic cell development and promotes terminal differentiation of myeloid cells [Citation21,Citation25]. In mature immune cells, it regulates the activation and effector function of mast cells, basophils, and pDCs [Citation25,Citation28]. IL-3/IL-3R pathway activation also plays a role in disease by promoting the proliferation and survival of malignant hematopoietic cells [Citation21,Citation27]. Increased CD123 expression contributes to the development of various hematologic malignancies [Citation1,Citation9,Citation29–32] and allergic inflammatory conditions [Citation21,Citation27].
Figure 1. The IL-3R system signaling pathway. AKT: protein kinase B; ERK: extracellular signal-regulated kinases; Grb2: growth factor receptor-bound protein 2; GTP: guanosine-5'-triphosphate; IL-3: interleukin 3; IL-3R: interleukin 3 receptor; JAK: Janus kinase; MAPK: mitogen-activated protein kinase; P: phosphate; PI3K: phosphoinositide 3-kinase; Shc: Src homology 2 domain-containing transforming protein; STAT: signal transducers and activators of transcription. Data from Martinez-Moczygemba and Huston, 2003 [Citation24].
![Figure 1. The IL-3R system signaling pathway. AKT: protein kinase B; ERK: extracellular signal-regulated kinases; Grb2: growth factor receptor-bound protein 2; GTP: guanosine-5'-triphosphate; IL-3: interleukin 3; IL-3R: interleukin 3 receptor; JAK: Janus kinase; MAPK: mitogen-activated protein kinase; P: phosphate; PI3K: phosphoinositide 3-kinase; Shc: Src homology 2 domain-containing transforming protein; STAT: signal transducers and activators of transcription. Data from Martinez-Moczygemba and Huston, 2003 [Citation24].](/cms/asset/55803842-6bc5-4346-84a5-ab91f6c363c7/ilal_a_1927021_f0001_c.jpg)
CD123 detection methods
Most studies analyze CD123 protein expression using immunohistochemistry (IHC) and/or flow cytometry (FC). These techniques have different detection sensitivities, with multiparametric FC considered to be the most sensitive. CD123 detection is affected by the following variables: sample source (e.g. bone marrow biopsy or aspirate, peripheral blood, lymph node biopsy, or skin biopsy); tissue/cell processing techniques; anti-CD123 antibody clone used [Citation20]; use of bulk versus purified cells; and cell purification techniques (e.g. fluorescence-activated cell sorting versus magnetic cell sorting) for FC specifically.
CD123 ribonucleic acid (RNA) expression can be detected in sorted single cells [Citation33,Citation34] or cell subsets using genome-wide microarray chips [Citation35,Citation36]. While not routinely performed in clinical practice, this technique characterizes the gene expression profile of CD123-expressing cells and identifies new biomarkers in tumor cells and the tumor microenvironment [Citation33,Citation34,Citation36,Citation37]. Such techniques detect CD123 with various degrees of sensitivity, specificity, and reproducibility, but require varying resources.
IHC
IHC involves staining of formalin-fixed, paraffin-embedded specimens with an anti-CD123 antibody followed by semiquantitative analysis. Tissue samples are often counterstained with hematoxylin and eosin or Giemsa to detect nucleated cells. A representative image of CD123 staining by IHC in pDCs from a patient with AML is shown in . Different anti-CD123 monoclonal antibody (mAb) clones are commercially available (e.g. 6H6 [Citation38,Citation39], 7G3 [Citation40], 9F5 [Citation41], and NCL-L-CD123 [Citation36]). Traditionally, staining assessments and analyses have been performed by two independent pathologists through direct visualization [Citation14,Citation41,Citation42]. More recently, a semiquantitative method using high-power fields acquired with a digital camera or from whole-slide scanning coupled with an image-analysis program has been developed [Citation43–45]. Consequently, the use of digital imaging in the assessment and quantification of IHC assays has become routine in clinical practice.
Figure 2. CD123 expression by immunohistochemistry in plasmacytoid dendritic cells in bone marrow biopsy of a patient with AML. Dual-color immunohistochemistry demonstrating membranous CD123 (brown) and nuclear TCF4 (red) staining; detailed section with ×20 magnification. AML: acute myeloid leukemia; TCF4: Transcription factor 4. Stemline Therapeutics, Inc. grants you non-exclusive permission to use the attached image in the article entitled ‘Targeting CD123 in hematologic malignancies: identifying suitable patients for targeted therapy’ authored by MM Patnaik, TI Mughal, C Brooks, R Lindsay, and N Pemmaraju and scheduled for publication in the journal entitled Leukemia & Lymphoma that is published by Informa Healthcare.
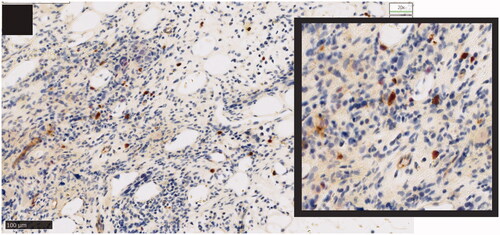
The threshold used to determine positivity for CD123 expression varies among studies. CD123 positivity has been defined as the staining of >30% of cells [Citation38,Citation41] or >20% of cells [Citation40], or >10% of blasts having moderate/strong staining [Citation14]. One study also defined CD123 negativity as the staining of <10% of cells [Citation41]. For better identification and characterization of CD123-expressing cells, IHC has been performed by sequential staining of tissue samples using an anti-CD123 antibody together with a second antibody (CD68) specific for the detection of HL cells [Citation36].
IHC analysis may also be performed using tissue microarray blocks, which allows for the automated high-throughput analysis of multiple specimens simultaneously [Citation42]. Tissue microarray slides are stained using automated methods that are rapid and consistent. Samples are analyzed semiquantitatively and defined as negative (no stained cells), positive homogeneous (>90% cells stained), or positive heterogeneous (≤90% cells stained).
Guideline recommendations exist for IHC analysis of biomarkers associated with breast cancer [Citation46], and thus the development of similar guidelines for hematologic malignancies would improve the standardization and reproducibility of IHC data and aid in identifying patients suitable for CD123-targeted therapy.
Fc
FC and new emerging technology platforms, such as single-cell mass cytometry (cytometry by time-of-flight [CyTOF]), are the most widely used techniques for detecting LSCs and CD123 membrane expression [Citation47]. Cells are stained with fluorescently labeled antibodies and channeled as a single-cell suspension through a laser beam, were scattered and fluorescent light signals are detected and analyzed by a computer. The advantages of this approach over IHC include the rapid analysis of large numbers of cells (≥10,000 cells/s), simultaneous analysis of multiple parameters (commonly ≥8), automated quantitation of cell frequency and fluorescence intensities, and sorting of predefined cell subsets. The disadvantages include loss of tissue structure, costly instrumentation, and the requirement for trained specialists. Characterization of typical CD123+ cells from a patient with BPDCN by multiparametric FC is shown in .
Figure 3. Phenotypic analysis of plasmacytoid dendritic cells by flow cytometry in bone marrow biopsy of a patient with BPDCN. pDCs expressing intermediate CD45 levels (top panels) are enriched for CD123 and CD303 expression (86.9%; bottom left panel). In this example, CD123+ CD303+ pDCs are characterized by co-expression of CD4, CD56, and TCL1 (bottom right panels). BPDCN: blastic plasmacytoid dendritic cell neoplasm; pDC: plasmacytoid dendritic cell; TCL1: T cell leukemia 1. Stemline Therapeutics, Inc. grants you non-exclusive permission to use the attached image in the article entitled ‘Targeting CD123 in hematologic malignancies: identifying suitable patients for targeted therapy’ authored by MM Patnaik, TI Mughal, C Brooks, R Lindsay, and N Pemmaraju and scheduled for publication in the journal entitled Leukemia & Lymphoma that is published by Informa Healthcare.
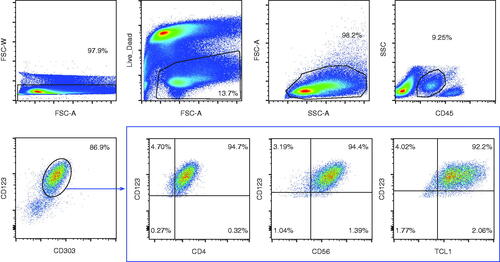
FC data are subject to considerable variability because of factors such as the anti-CD123 clone and fluorophore used, cell subset analyzed, isolation method, number of events analyzed, source of positive/negative samples, data analysis methods (including thresholds for CD123 positivity and expression levels [low/moderate/high]), and instrument sensitivity. Although guidance for the standardization of FC protocols and antibody panels exists [Citation48,Citation49], its implementation in clinical practice remains suboptimal.
The EuroFlow Consortium, an independent scientific European Union-funded group established in 2005 to advance the field of flow cytometric immunophenotyping, and now a Scientific Working Group of the European Hematology Association, has issued guidance on standardizing FC protocols [Citation50,Citation51] and antibody panels [Citation52] for immunophenotyping. A retrospective, multicenter study evaluating CD123 expression in patients with acute leukemia using standardized EuroFlow immunophenotyping [Citation53] analyzed samples from 846 patients and 1736 normal cell populations. CD123 expression in normal nucleated red blood cells (NRBCs) and normal pDCs served as negative and positive controls, respectively. CD123 positivity was defined as the highest 98th percentile among NRBCs. Average CD123 expression levels were quantitated by CD123 median fluorescence intensity for normal (lowest in NRBCs, highest in pDCs) and leukemic populations. CD123-expression data in samples from patients with leukemia were found to be stable and reproducible over time and across centers using the standardized EuroFlow protocol.
One group evaluated five commercially available antibodies for CD123 detection by FC (9F5, 6H6, 7G3, FAB301, and AC145) and concluded that two antibody clones, 9F5 (BD Biosciences, Franklin Lakes, NJ, USA) and 6H6 (BioLegend, San Diego, CA, USA), were more predictive of the AML phenotype and correlated better with gene transcription levels compared with the other clones [Citation20]. Most studies analyzing malignant stem cells rely on staining with an anti-CD123 antibody to define the CD34+ CD38− CD45+ stem cell population as well as lineage-specific antibodies (lin) for faster quantification of CD123+ stem cells and single-cell isolation. Zeijlemaker et al. [Citation54] designed a simple FC assay for the rapid quantification of LSCs in patients with AML. One fluorescence channel was used to detect eight colors for common markers (CD45, CD34, and CD38), specific markers (CD45RA, CD123, CD33, and CD44), and a marker cocktail (CLL-1/TIM-3/CD7/CD11b/CD22/CD56) in a single sample. This approach showed comparable accuracy, used fewer samples, and required less time for quantifying leukemic cells compared with the multitube approach. The development of standardized protocols for the detection, isolation, and analysis of CD123-expressing cells in hematologic malignancies would improve our knowledge surrounding the etiology and evolution of the disease and facilitate the selection of patients most suitable for CD123-targeted therapy.
Advanced diagnostic techniques
Advances in the chemistry, antibody design and automation of assays and analyses have led to substantial improvements in sensitivity, specificity, and reproducibility in IHC assays [Citation55]. Clinical assays using dual-color stains and mutation-specific antibodies provide novel tools for clinical diagnostics, allowing the potential to enhance biomarker detection specificity. Analysis of digitized whole-slide images has yielded highly precise and reproducible data, which when coupled to artificial intelligence, could improve IHC readouts substantially [Citation56]. Progress in cyclic labeling and optical imaging methods has improved the spatial resolution and accuracy of surface protein detection in multiplex assays [Citation57]. CO-Detection by indEXing (CODEX®; Akoya Biosciences, Inc., CA, USA), which is a multiparametric imaging technology designed for sequential labeling of tissue sections, use a library of antibodies conjugated to dye-labeled deoxyribonucleic acid (DNA). CODEX® allows for the characterization of the three-dimensional structure at a single-cell level and in the tissue microenvironment.
FC is continuously evolving to develop user-friendly instruments with greater sensitivity, faster analysis of larger cell numbers, and the use of more parameters, such as CytoFLEX (Beckman Coulter Life Sciences, Indianapolis, IN, USA). However, the number of parameters that can be analyzed simultaneously is limited by the spectral overlap between different fluorophores and cell autofluorescence. To overcome this barrier, single-cell mass cytometry, a combination of FC and mass spectrometry, was developed, allowing for the detection of >40 antigens using CyTOF instrumentation. This technique uses antibodies labeled with stable heavy-metal isotopes and is highly accurate at distinguishing isotopes of different atomic weights with minimal signal overlap [Citation58]. Mass cytometry allows for the detection of cell-surface markers to characterize cells and enables the simultaneous measurement of modifications of intracellular proteins involved in signaling pathways, messenger RNA transcription, and DNA synthesis, thereby providing a complete picture of ongoing cellular processes at the single-cell level.
CD123 expression in normal tissues/organs
CD123 expression is relatively high in CD34+ myeloid and B-lymphoid progenitor cells in fetal and adult hematopoietic tissues, whereas it is negative or low in CD34+ CD38− hematopoietic stem cells and erythroid progenitors [Citation59]. FC quantitation of CD123-expressing cells in adult bone marrow demonstrated CD123 expression in <1% of the total nucleated cells [Citation3,Citation60]. Among CD34+ cells, 46–53% express CD123 [Citation3,Citation61]. In the CD34+ CD38− lin − subset, 20% express CD123 in adult bone marrow [Citation28,Citation61], while ∼9% of CD34+ CD38+ cells express CD123 [Citation62]. In the myeloid progenitor compartment (CD34+ CD33+ CD19−), CD123 was shown to have a dim/moderate expression intensity on 63% of cells, whereas cells in the lymphoid progenitor compartment (CD34+ CD33− CD19+ CD10+) were CD123 − [Citation3]. FC analyses in peripheral blood showed that dendritic cells had the strongest CD123 expression [Citation3], and 98% of lin − CD11c– pDCs are CD123 + [Citation63]. Monocytes are CD123+ generally with low staining intensity, whereas granulocytes are CD123– [Citation3]. In addition, a low percentage of B cells express CD123 [Citation3]. In lymph nodes, CD123 expression intensity was dim on B cells and negative on T cells [Citation3].
Therapeutic implications of CD123 therapy in hematologic malignancies
CD123 expression in hematologic malignancies
It is generally agreed that Craig Jordan provided, in 2000, the first concrete evidence that most, if not all, LSCs from patients with AML, then defined phenotypically as CD34+ CD38− cells, overexpressed the CD123 surface antigen, in Lexington, Kentucky, US in 2000 [Citation1]. As a result, a potential pathogenetic role was speculated, but the precise mechanisms remain unknown. This landmark work did, however, establish that CD123+ CD34+ CD38− cells initiated and maintained the AML phenotype in vivo, suggesting that they represent the LSC population and provide a strong scientific rationale for testing CD123-targeted therapies for the treatment of CD123+ AML [Citation1]. CD123 overexpression has since been observed in a variety of other hematologic malignancies [Citation3,Citation4]; depicts the CD123 expression levels in different hematologic malignancies. The percentage of CD123-expressing cells varies substantially across diseases, with the highest frequency found in BPDCN, AML, B-ALL, HCL, MDS, and chronic eosinophilic leukemia, where it can be detected in up to 100% of samples analyzed by FC, with the caveat that expression data may be affected by the different methodologies employed for CD123 detection [Citation20,Citation33–45,Citation47–55].
Table 1. CD123 expression in hematologic malignancies.
In a multicenter study, all 20 patients with BPDCN had CD123+ neoplastic cells (FC assessed), with high expression intensity in 18 (90%) patients [Citation64]. In another single-center study, CD4+ CD56+ tumor cells were CD123+ (by IHC) in all seven (100%) patients with BPDCN [Citation39]. A study analyzing samples from patients with BPDCN by IHC and/or FC found CD123 positivity in 18 of 24 (75%) cases [Citation41]. Finally, a study showed a higher proportion of CD123+ cells among CD4+ and/or CD56+ cells from patients with cutaneous (91%; 32/35) versus non-cutaneous (50%; 3/6) BPDCN [Citation38].
In a study of 846 patients with acute leukemia, including 139 pediatric and 316 adult patients with AML, ≥80% of leukemic cells were CD123+ in 55% of patients, with CD123high expression in most cases (98%; 444/455) [Citation53]. No differences in expression frequency or levels were found between pediatric and adult patients. Another study showed CD123high expression in >95% of CD34+ CD38− LSCs from all ten patients [Citation4]. Recently, CD123 has been found in LSCs in 67% of samples from pediatric patients with AML. Interestingly CD123 and CD200 were co-expressed in the majority (57%) of the samples, and co-expression of these molecules correlated with poor prognosis [Citation84]. Finally, a study of 157 bone marrow biopsies demonstrated a correlation between CD123 expression and FMS-like tyrosine kinase 3 internal tandem duplication and/or nucleophosmin 1 mutations [Citation44].
In patients with ALL, CD123 expression appears to be more prevalent in the B-cell subtype, compared with the T-cell subtype [Citation3,Citation11,Citation53,Citation69] and in patients with Philadelphia chromosome (BCR-ABL1)-positive than -negative B-ALL [Citation53,Citation69]. ALL with hyperdiploid karyotype correlated with high frequencies and expression levels of CD123-expressing cells [Citation11,Citation53]. The frequency and expression levels of CD123-expressing malignant cells were higher in pediatric compared with adult patients with B-ALL [Citation53] and conversely, in adult compared with pediatric patients with T-ALL [Citation53,Citation70]. In patients with T-ALL, CD123 expression was associated with the maturation stage of malignant cells, with frequencies of CD123+ cells decreasing from 83% to 40% and 21% in early T-precursor, T-precursor, and mature T-ALL, respectively [Citation70]. No correlation was found between CD123 expression and MRD status following induction therapy [Citation69,Citation70]. For patients with T-ALL, the relapse-free survival rate was higher in those with a CD123− than CD123+ phenotype (p = 0.03). No such correlation was found in patients with B-ALL [Citation69].
Interestingly, recent studies of CD34+ CD38− lin − primary MDS stem cells revealed overexpression of CD123, which progressively increases during MDS evolution [Citation6,Citation79,Citation80]. The precise significance of this observation is an enigma at present since no specific molecular/functional findings have been described. Regardless, the upregulation of CD123 expression in the CD34+ CD38− MDS stem cell compartment, complemented in some instances by a higher rate of CD96 but not CD90 expression, could provide a useful tool to identify and distinguish the low-risk disease from the high-risk disease [Citation6,Citation79]. Studies have also shown that CD123+ MDS stem cells are impaired by B-cell lymphoma 2 (BCL-2) inhibition, providing support for use of a CD123-targeted therapy plus an anti-BCL-2 agent combination in patients with high-risk MDS [Citation79].
Higher frequencies and levels of CD123 expression are seen in malignant cells from patients with HCL than in from patients with the HCL variant phenotype [Citation13,Citation75,Citation76], and CD123 is co-expressed with CD103 in most HCL cells [Citation12,Citation76]. CD123 expression has also been detected in malignant cells of patients with various non-Hodgkin lymphomas, with the highest frequency seen in patients with mediastinal gray zone lymphoma (MGZL; 71%) [Citation77]. Its expression may aid in ruling out primary mediastinal large B-cell lymphoma in the differential diagnosis of MGZL.
In patients with CMML, clusters of clonally related CD123+ pDCs were found in the bone marrow of 20% (32/159) of patients in one case series [Citation71]; skin lesions from these patients also demonstrated the presence of CD123+ mature pDCs [Citation72]. Furthermore, progression to secondary AML is associated with CD123 upregulation [Citation85]. In patients with chronic myeloid leukemia (CML), the percentage of CD123-expressing cells is higher in those with blast crisis phase CML versus those with de novo chronic phase CML, suggestive of a correlation between CD123 expression and disease progression [Citation29].
Peripheral blood from patients with primary and secondary myelofibrosis is characterized by increased CD123-expressing cells compared with healthy individuals. In primary myelofibrosis, an approximately 40-fold increase in the percentage of CD123high CD34+ cells over total CD34+ cells has been reported [Citation81]. Interestingly, CD123 expression was predominantly increased in peripheral blood CD34− CD38+ cells [Citation60]. CD123 is overexpressed in tumor microenvironment macrophages in patients with HL, which could influence prognosis and response to therapy [Citation36].
CD123-targeted therapies
Currently, tagraxofusp is the only FDA-approved CD123-targeted therapy; it is approved for the treatment of newly diagnosed and relapsed/refractory (R/R) BPDCN in adult and pediatric patients [Citation19]. MB-102 T cells are genetically modified to express a costimulatory CD123-specific chimeric antigen receptor (CAR) and a truncated epidermal growth factor receptor (CD123CAR-CD28-CD3ζ-EGFRt) that were granted Orphan Drug Designation in 2018 for the treatment of BPDCN and in 2019 for AML [Citation86]. depicts some of the anti-CD123 therapies that are being tested for the treatment of CD123+ hematologic malignancies.
Table 2. Clinical trials of CD123-targeting agents.
Recombinant diphtheria toxin/IL-3 fusion protein: tagraxofusp
The pivotal open-label, multicohort, phase 1/2 study of tagraxofusp consisting of stages 1 (dose escalation), 2 (expansion), 3 (pivotal, confirmatory), and 4 (continued access) enrolled 47 adult patients with untreated or relapsed BPDCN in the USA [Citation87,Citation88]. The primary outcome was the combined CR and clinical CR (CRc) rate in untreated patients. Of 29 untreated patients receiving tagraxofusp, 21 (72%) had CR/CRc, with 65% of patients achieving CR by cycle 2; the overall response rate (ORR) was 90%. Thirteen (45%) patients underwent stem cell transplantation. The median OS was 26 months, with an OS rate at 24 months of 52%. For the 15 relapsed patients receiving tagraxofusp, the ORR was 67%, including 2 patients with CR/CRC, and the median OS was 8.5 months. Capillary leak syndrome was reported in 19% of patients and was associated with two deaths. In tandem with the pivotal study, three pediatric patients with BPDCN received tagraxofusp treatment on compassionate grounds. The treatment was well tolerated and resulted in significant, albeit transient, clinical improvement in two patients [Citation89]. Single-agent tagraxofusp is being evaluated in a phase 2 study as maintenance therapy in patients with BPDCN who have undergone hematopoietic stem cell transplantation (HSCT) [Citation90]. Furthermore, tagraxofusp as a monotherapy or combination therapy is currently being investigated in several other CD123-enriched hematologic malignancies, including newly diagnosed and R/R AML, R/R ALL, high-risk MDS, R/R myelofibrosis, newly diagnosed and R/R CMML, and R/R multiple myeloma (MM). A phase 1/2 trial in patients with myelofibrosis or CMML is currently ongoing (NCT02268253). Preliminary results showed that, in the R/R myelofibrosis cohort (n = 36), which included patients for whom previous treatment with a JAK inhibitor (ruxolitinib) had failed, treatment with tagraxofusp monotherapy resulted in 56% of patients with spleen responses [Citation91] with 48% of patients reporting an improvement in quality of life, measured with the Myelofibrosis Symptom Assessment Form [Citation92], at week 24. Additionally, 56% of patients achieved stable disease, and the median OS was 31 months [Citation93]. Three patients experienced capillary leak syndrome; no treatment-related deaths occurred. The CMML arm (n = 29) included patients who were R/R to previous treatment, mostly with hypomethylating agents (HMAs), and treatment-naive patients (CMML-1, n = 19; CMML-2, n = 10). Fourteen of 29 patients had splenomegaly at baseline, all fourteen (100%) of whom experienced a spleen response following tagraxofusp monotherapy treatment; three patients achieved bone marrow CR, including one patient who was successfully bridged to an allogeneic stem cell transplant. Capillary leak syndrome was reported in seven patients (four with grade 1 or 2; three with grade 3) [Citation94]. These interim results with tagraxofusp in myelofibrosis and CMML are encouraging in an unmet clinical scenario and the study is ongoing. pDCs expressing high levels of CD123 can be found in the bone marrow microenvironment of patients with MM. Tagraxofusp has been evaluated in combination with pomalidomide and dexamethasone in a phase 1/2 study enrolling patients with R/R MM [Citation95]. The treatment was well tolerated with 56% (five out of nine) patients achieving a partial response, which was associated with a decrease in pDCs [Citation95] and a reduced ability of this pDCs to promote MM cell survival and growth [Citation96]. Further investigation of antimyeloma treatments that include combination studies with tagraxofusp is warranted.
mAbs
Talacotuzumab, a humanized anti-CD123 mAb, was investigated as monotherapy and in combination with HMAs, but further clinical development was halted because of an unfavorable risk-benefit profile. In a phase 2 trial, talacotuzumab alone showed limited efficacy and considerable toxicity in elderly high-risk patients with MDS or AML who had relapsed on previous HMA treatment [Citation97,Citation98]. In a multicenter, phase 2/3 study of talacotuzumab plus decitabine versus decitabine alone in elderly patients with AML not eligible for intensive chemotherapy [Citation99], there was no improved efficacy with talacotuzumab plus decitabine versus decitabine alone. Enrollment was terminated early and talacotuzumab treatment was discontinued on the recommendation of the independent data monitoring committee. A phase 1 study of KHK2823 (NCT02181699), a nonfucosylated, fully human mAb that mediates antibody-dependent cell-mediated cytotoxicity without inducing complement-dependent cytotoxicity [Citation100], was also terminated because of failed treatment response. These studies suggest that anti-CD123 antibodies alone may be insufficiently effective, and additional strategies, such as coupling of cytotoxic payloads, synergistic activation of patient immune cells, or cellular immunotherapy, could be necessary.
Antibody-drug conjugates (ADCs)
IMGN632 is a novel ADC consisting of a humanized anti-CD123 antibody conjugated to a DNA mono-alkylating payload via a protease-cleavable linker [Citation101]. A phase 1 trial of IMGN632 monotherapy in patients with R/R AML or BPDCN demonstrated promising antitumor activity and a manageable safety profile [Citation102,Citation103], including in patients who had received prior anti–CD123-targeted therapy [Citation103] and in those with TP53 mutations [Citation104]. IMGN632 has recently received FDA Breakthrough Therapy Designation in R/R BPDCN [Citation105]. A phase 1 b/2 study of IMGN632 monotherapy or combined with venetoclax and/or azacitidine in patients with R/R AML is ongoing [Citation106].
Other CD123-ADCs, such as SGN-CD123A and the novel ADC BAY-943, are currently in preclinical or clinical development and have shown antileukemic effects in preclinical models of AML [Citation107, Citation108]. A phase 1 trial of SGN-CD123A in patients with AML (NCT02848248) was terminated prematurely; thus, the feasibility of these ADCs for the treatment of patients with hematologic malignancies requires further evaluation.
Bispecific antibodies
Flotetuzumab is a CD123 × CD3 dual-affinity retargeting antibody that recognizes CD123+ leukemia cells, inducing T-cell activation with resulting cytotoxicity [Citation109,Citation110]. It is currently in phase 1/2 trials as a monotherapy in patients with R/R AML and MDS. Preliminary results have shown anticancer activity with a manageable safety profile [Citation111]. Flotetuzumab in combination with blinatumomab, a CD19 × CD3 bispecific antibody, is being evaluated in preclinical studies in cells from patients with B-precursor ALL [Citation112]. A phase 1 trial of flotetuzumab combined with MGA012, an anti-programmed cell death protein 1 mAb, in patients with R/R AML is planned [Citation113]. Finally, an expanded access program has started recently with flotetuzumab as monotherapy in patients with AML with a positive potential benefit-risk balance (NCT04678466). Three additional CD123 × CD3 bispecific mAbs, JNJ-63709178, XmAb14045, and APVO436, are being evaluated in phase 1 trials [Citation110, ].
CAR T cells
The past decade has witnessed promising results in a variety of hematologic malignancies following treatment with either allogeneic or autologous-derived T cells engineered with CARs [Citation114]. A number of autologous CAR T cells that express CD123-specific antigen receptors have been developed and are currently in the early stages of clinical investigation (). CAR T-cell therapy has been associated with toxicities such as cytokine release syndrome and on-target/off-tumor toxicity. CARs have evolved to improve on-target activity and to incorporate strategies, including combinatorial target antigen recognition, bispecific T-cell engagement, or promotion of cell suicide to decrease toxicity [Citation115].
MB-102 is a self-inactivating CD123 CAR construct composed of an anti-CD123 single-chain variable fragment, an optimized immunoglobulin G4 CH2-CH3 linker, a CD28 co-stimulatory domain, and a CD3ζ signaling domain together with a truncated EGFR expressed in engineered T cells [Citation116]. A first-in-human, dose-escalation phase 1 trial (NCT02159495) demonstrated the feasibility and safety of targeting CD123 in patients with AML and BPDCN, and MB-102 showed encouraging antitumor activity with no myeloablative effects. MB-102 was granted Orphan Drug Designation by the FDA for treatment of BPDCN and AML.
UCART123 are allogeneic T cells expressing CD123 CARs. While the clinical study in patients with BPDCN was terminated prematurely [Citation117], UCART123 are still under investigation in a phase 1 trial in patients with R/R AML [Citation118].
UniCAR is a rapidly switchable universal CAR T platform that enables highly controlled and dose-dependent activation of CAR T. UniCAR T cells recognize a small peptide that is not presented on the cell surface and thus inactive under physiologic conditions. This platform has been optimized for targeting CD123+ acute leukemia cells by expressing the UniCAR T cells together with TM123 (anti–CD123 mAb–CD28/CD3ζ) to drive T-cell activation [Citation119] and is being assessed in a phase 1 study (NCT04230265).
Discussion
Although CD123 overexpression has now been described in several hematologic malignancies, where it is associated with poor prognosis, its precise biological significance remains enigmatic. CD123 is an attractive biomarker for the diagnosis and prognostic assessment of many hematologic malignancies and has potential use in monitoring response to treatment. Knowing the frequency of CD123-expressing cells and levels of CD123 expression is key to selecting patients who would benefit most from CD123-targeted therapies, and therefore techniques that detect CD123 with high specificity and sensitivity are required. FC and IHC are the primary methods used for CD123 detection. Analysis of CD123 transcription levels and the use of advanced diagnostic techniques will provide more accurate information on CD123 expression and help in the discovery of new biomarkers.
Novel CD123-targeted therapies, with different mechanisms of action, have been developed with markedly different clinical results. While the recombinant fusion protein tagraxofusp is currently the only FDA-approved CD123-targeted therapy, several anti-CD123 ADCs, bispecific antibodies, and new-generation CAR T-cell therapies are in early clinical investigation, and results of these studies will be important in determining the feasibility of CD123-targeted therapies for hematologic malignancies [Citation120,Citation121].
Tagraxofusp is currently being assessed in patients with BPDCN, as maintenance therapy after HSCT (NCT04317781). It is also under active clinical investigation for various hematologic malignancies as monotherapy, including CMML and myelofibrosis (NCT02268253) and in patients with CD123+ or with ‘BPDCN-like’ AML (also known as pDC-AML) (NCT04342962), or in combination with azacitidine with or without venetoclax for patients with AML and high-risk MDS (NCT03113643) [Citation122]. Due to CD123 overexpression in both lymphoid and myeloid lineages, its potential use in HL, B-ALL, and other CD123-expressing hematologic malignancies is of special interest. Indeed, several pan-CD123 positive studies in children and adults assessing both chemotherapy-based and chemotherapy-free combinations with tagraxofusp are currently in the final stages of development. Furthermore, the recent elucidation of RUNX1 mutations in pDC-AML, known to express CD123, supports the notion of targeting this rare subtype of AML with anti-CD123 therapies [Citation123].
Progress in this field is impacted by the use of accurate methods for detecting CD123 expression in candidate malignancies. Unlike in BPDCN, where CD123 is overexpressed uniformly in malignant pDCs [Citation64,Citation86], its expression in other malignancies is limited to certain cell types and sometimes the microenvironment, pre-inflammatory, and inflammatory contexts [Citation124]. Advances in detection techniques, such as FC, CyTOF, and CODEX®, might overcome these limitations, thus allowing the individualization of therapy. These methods can also lead to the development of biomarker-based clinical trials and subsequent biomarker discovery.
Resistance to tagraxofusp can be developed by downregulation of the diphthamide synthesis pathway, which is important for diphtheria toxin-mediated cytotoxicity [Citation125]. In experimental models of BPDCN and AML, sensitivity to tagraxofusp was restored by the combination of azacitidine and tagraxofusp. Therefore, this combination should be considered for those patients with CD123-expressing tumor cells that are resistant to tagraxofusp monotherapy. Further research to uncover additional resistance mechanisms that may involve CD123 downregulation would be needed to optimize the use of CD123-targeting therapies as monotherapy or in combination for different hematologic malignancies.
Regarding CD123-targeting therapies, a major limitation is the occurrence of important side effects, such as the capillary leak syndrome observed with tagraxofusp and cytokine release syndrome and neurologic toxicity associated with CAR T cells [Citation126]. The development of drugs or CAR T-cell therapies that have minimal off-target effects would make CD123-targeting agents safer and easier to administer. As various disease subtypes are included in the growing field of CD123-expressing tumors, in this new era of combination targeted therapy it will be of strong interest to investigate novel strategies that combine CD123 targeting with BCL-2–targeted agents, HMAs, and cytotoxic agents, with continuous monitoring for the emergence of new toxicities and drug–drug interactions.
Author contributions
All authors participated in the writing of the draft and final version of the manuscript and approved the submitted version.
Disclosure statement
MMP: advisory board for Stemline Therapeutics. TIM: royalties from Oxford University Press and Informa; equity in and employment at Stemline Therapeutics. CB and RL: equity in and employment at Stemline Therapeutics. NP: consulting and honoraria from AbbVie, Blueprint Medicines, Celgene, Incyte, LFB Biotechnologies, Mustang Bio, Novartis, Pacylex Pharmaceuticals, Roche Diagnostics, and Stemline Therapeutics; Research funding/clinical trials support from AbbVie, Affymetrix, Cellectis, Daiichi Sankyo, Novartis, Sager Strong Foundation, Samus, Stemline Therapeutics, and Plexxikon.
Additional information
Funding
References
- Jordan CT, Upchurch D, Szilvassy SJ, et al. The interleukin-3 receptor alpha chain is a unique marker for human acute myelogenous leukemia stem cells. Leukemia. 2000;14(10):1777–1784.
- Chaperot L, Bendriss N, Manches O, et al. Identification of a leukemic counterpart of the plasmacytoid dendritic cells. Blood. 2001;97(10):3210–3217.
- Muñoz L, Nomdedéu JF, López O, et al. Interleukin-3 receptor alpha chain (CD123) is widely expressed in hematologic malignancies. Haematologica. 2001;86(12):1261–1269.
- Florian S, Sonneck K, Hauswirth AW, et al. Detection of molecular targets on the surface of CD34+/CD38– stem cells in various myeloid malignancies. Leuk Lymphoma. 2006;47(2):207–222.
- Fromm JR. Flow cytometric analysis of CD123 is useful for immunophenotyping classical Hodgkin lymphoma. Cytometry B Clin Cytom. 2011;80(2):91–99.
- Xie W, Wang X, Du W, et al. Detection of molecular targets on the surface of CD34 + CD38– bone marrow cells in myelodysplastic syndromes. Cytometry. 2010;77A(9):840–848.
- Liu K, Zhu M, Huang Y, et al. CD123 and its potential clinical application in leukemias. Life Sci. 2015;122:59–64.
- Testa U, Pelosi E, Frankel A. CD 123 is a membrane biomarker and a therapeutic target in hematologic malignancies. Biomark Res. 2014;2(1):4.
- Testa U, Riccioni R, Militi S, et al. Elevated expression if IL-3Ralpha in acute myelogenous leukemia is associated with enhanced blast proliferation, increased cellularity, and poor prognosis. Blood. 2002;100(8):2980–2988.
- Yue LZ, Fu R, Wang HQ, et al. Expression of CD123 and CD114 on the bone marrow cells of patients with myelodysplastic syndrome. Chin Med J. 2010;123:2034–2037.
- Djokic M, Björklund E, Blennow E, et al. Overexpression of CD123 correlates with the hyperdiploid genotype in acute lymphoblastic leukemia. Haematologica. 2009;94(7):1016–1019.
- Venkataraman G, Aguhar C, Kreitman RJ, et al. Characteristic CD103 and CD123 expression pattern defines hairy cell leukemia: usefulness of CD123 and CD103 in the diagnosis of mature B-cell lymphoproliferative disorders. Am J Clin Pathol. 2011;136(4):625–630.
- Al-Mawali A, Pinto AD, Al-Zadjali S. CD34 + CD38-CD123+ cells are present in virtually all acute myeloid leukaemia blasts: a promising single unique phenotype for minimal residual disease detection. Acta Haematol. 2017;138(3):175–181.
- Arai N, Homma M, Abe M, et al. Impact of CD123 expression, analyzed by immunohistochemistry, on clinical outcomes in patients with acute myeloid leukemia. Int J Hematol. 2019;109(5):539–544.
- Testa U, Pelosi E, Castelli G. CD123 as a therapeutic target in the treatment of hematological malignancies. Cancers. 2019;11:1358.
- Alfayez M, Konopleva M, Pemmaraju N. Role of tagraxofusp in treating blastic plasmacytoid dendritic cell neoplasm (BPDCN). Expert Opin Biol Ther. 2020;20(2):115–123.
- Economides MP, Konopleva M, Pemmaraju N. Recent developments in the treatment of blastic plasmacytoid dendritic cell neoplasm. Ther Adv Hematol. 2019;10:204062071987473. 2040620719874733.
- Lee SS, McCue D, Pemmaraju N. Tagraxofusp as treatment for patients with blastic plasmacytoid dendritic cell neoplasm. Expert Rev Anticancer Ther. 2020;20(7):543–550.
- Elzonris (tagraxofusp-erzs) injection, for intravenous use [prescribing information]. New York, NY: Stemline Therapeutics, Inc.; 2018.
- Cruz NM, Sugita M, Ewing-Crystal N, et al. Selection and characterization of antibody clones are critical for accurate flow cytometry-based monitoring of CD123 in acute myeloid leukemia. Leuk Lymphoma. 2018;59(4):978–982.
- Broughton SE, Dhagat U, Hercus TR, et al. The GM-CSF/IL-3/IL-5 cytokine receptor family: from ligand recognition to initiation of signaling. Immunol Rev. 2012;250(1):277–302.
- Hercus TR, Kan WLT, Broughton SE, et al. Role of the β common (βc) family of cytokines in health and disease. Cold Spring Harb Perspect Biol. 2018;10(6):a028514.
- Lopez AF, Hercus TR, Ekert P, et al. Molecular basis of cytokine receptor activation. IUBMB Life. 2010;62(7):509–518.
- Martinez-Moczygemba M, Huston DP. Biology of common beta receptor-signaling cytokines: IL-3, IL-5, and GM-CSF. J Allergy Clin Immunol. 2003;112(4):653–665.
- Ihle JN. Interleukin-3 and hematopoiesis. Chem Immunol. 1992;51:65–106.
- Hara T, Miyajima A. Function and signal transduction mediated by the interleukin 3 receptor system in hematopoiesis. Stem Cells. 1996;14(6):605–618.
- Hercus TR, Broughton SE, Ekert PG, et al. The GM-CSF receptor family: mechanism of activation and implications for disease. Growth Factors. 2012;30(2):63–75.
- Borriello F, Galdiero MR, Varricchi G, et al. Innate immune modulation by GM-CSF and IL-3 in health and disease. IJMS. 2019;20(4):834.
- Nievergall E, Ramshaw HS, Yong AS, et al. Monoclonal antibody targeting of IL-3 receptor α with CSL362 effectively depletes CML progenitor and stem cells. Blood. 2014;123(8):1218–1228.
- Frankel AE, Woo JH, Ahn C, et al. Activity of SL-401, a targeted therapy directed to interleukin-3 receptor, in blastic plasmacytoid dendritic cell neoplasm patients. Blood. 2014;124(3):385–392.
- Pardanani A, Lasho T, Chen D, et al. Aberrant expression of CD123 (interleukin-3 receptor-α) on neoplastic mast cells. Leukemia. 2015;29(7):1605–1608.
- Pardanani A, Reichard KK, Zblewski D, et al. CD123 immunostaining patterns in systemic mastocytosis: differential expression in disease subgroups and potential prognostic value. Leukemia. 2016;30(4):914–918.
- See P, Dutertre C-A, Chen J, et al. Mapping the human DC lineage through the integration of high-dimensional techniques. Science. 2017;356(6342):eaag3009.
- Chen Y-L, Gomes T, Hardman CS, et al. Re-evaluation of human BDCA-2+ DC during acute sterile skin inflammation. J Exp Med. 2020;217.jem.20190811.
- Lindstedt M, Lundberg K, Borrebaeck CAK. Gene family clustering identifies functionally associated subsets of human in vivo blood and tonsillar dendritic cells. J Immunol. 2005;175(8):4839–4846.
- Ruella M, Klichinsky M, Kenderian SS, et al. Overcoming the immunosuppressive tumor microenvironment of Hodgkin lymphoma using chimeric antigen receptor T cells. Cancer Discov. 2017;7(10):1154–1167.
- Tang X, Huang Y, Lei J, et al. The single-cell sequencing: new developments and medical applications. Cell Biosci. 2019;9:53.
- Suzuki Y, Kato S, Kohno K, et al. Clinicopathological analysis of 46 cases with CD4+ and/or CD56+ immature haematolymphoid malignancy: reappraisal of blastic plasmacytoid dendritic cell and related neoplasms. Histopathology. 2017;71(6):972–984.
- An HJ, Yoon DH, Kim S, et al. Blastic plasmacytoid dendritic cell neoplasm: a single-center experience. Ann Hematol. 2013;92(3):351–356.
- Safaei A, Monabati A, Mokhtari M, et al. Evaluation of the CD123 expression and FLT3 gene mutations in patients with acute myeloid leukemia. Iran J Pathol. 2018;13(4):438–446.
- Hashikawa K, Niino D, Yasumoto S, et al. Clinicopathological features and prognostic significance of CXCL12 in blastic plasmacytoid dendritic cell neoplasm. J Am Acad Dermatol. 2012;66(2):278–291.
- Bénet C, Gomez A, Aguilar C, et al. Histologic and immunohistologic characterization of skin localization of myeloid disorders: a study of 173 cases. Am J Clin Pathol. 2011;135(2):278–290.
- ImageJ [website]. Available at https://imagej.nih.gov/ij/index.html [cited 2021 April 20].
- Rollins-Raval MA, Roth CG. The value of immunohistochemistry for CD14, CD123, CD33, myeloperoxidase and CD68R in the diagnosis of acute and chronic myelomonocytic leukaemias. Histopathology. 2012;60(6):933–942.
- Rollins-Raval M, Pillai R, Warita K, et al. CD123 immunohistochemical expression in acute myeloid leukemia is associated with underlying FLT3-ITD and NPM1 mutations. Appl Immunohistochem Mol Morphol. 2013;21(3):212–217.
- Hammond MEH, Hayes DF, Dowsett M, et al. American Society of Clinical Oncology/College of American pathologists guideline recommendations for immunohistochemical testing of estrogen and progesterone receptors in breast cancer (unabridged version). Arch Pathol Lab Med. 2010;134:e48–72.
- Han L, Qiu P, Zeng Z, et al. Single-cell mass cytometry reveals intracellular survival/proliferative signaling in FLT3-ITD-mutated AML stem/progenitor cells. Cytometry A. 2015;87(4):346–356.
- Finak G, Langweiler M, Jaimes M, et al. Standardizing flow cytometry immunophenotyping analysis from the human immunophenotyping consortium. Sci Rep. 2016;6:20686.
- Cossarizza A, Chang HD, Radbruch A, et al. Guidelines for the use of flow cytometry and cell sorting in immunological studies (second edition). Eur J Immunol. 2019;49(10):1457–1973.
- Kalina T, Flores-Montero J, van der Velden VHJ, et al. EuroFlow standardization of flow cytometer instrument settings and immunophenotyping protocols. Leukemia. 2012;26(9):1986–2010.
- Kalina T, Flores-Montero J, Lecrevisse Q, et al. Quality assessment program for EuroFlow protocols: summary results of four-year (2010–2013) quality assurance rounds. Cytometry A. 2015;87(2):145–156.
- van Dongen JJM, Lhermitte L, Böttcher S, et al. EuroFlow antibody panels for standardized n-dimensional flow cytometric immunophenotyping of normal, reactive and malignant leukocytes. Leukemia. 2012;26(9):1908–1975.
- Bras AE, de Haas V, van Stigt A, et al. CD123 expression levels in 846 acute leukemia patients based on standardized immunophenotyping. Cytometry B Clin Cytom. 2019;96(2):134–142.
- Zeijlemaker W, Kelder A, Oussoren-Brockhoff YJM, et al. A simple one-tube assay for immunophenotypical quantification of leukemic stem cells in acute myeloid leukemia. Leukemia. 2016;30(2):439–446.
- Sukswai N, Khoury JD. Immunohistochemistry innovations for diagnosis and tissue-based biomarker detection. Curr Hematol Malig Rep. 2019;14(5):368–375.
- Aeffner F, Zarella MD, Buchbinder N, et al. Introduction to digital image analysis in whole-slide imaging: a white paper from the Digital Pathology Association. J Pathol Inform. 2019;10(1):9.
- Goltsev Y, Samusik N, Kennedy-Darling J, et al. Deep profiling of mouse splenic architecture with CODEX multiplexed imaging. Cell. 2018;174(4):968–981.
- Spitzer MH, Nolan GP. Mass cytometry: single cells, many features. Cell. 2016;165(4):780–791.
- Huang S, Chen Z, Yu JF, et al. Correlation between IL-3 receptor expression and growth potential of human CD34+ hematopoietic cells from different tissues. Stem Cells. 1999;17(5):265–272.
- Lasho T, Finke C, Kimlinger TK, et al. Expression of CD123 (IL-3R-alpha), a therapeutic target of SL-401, on myeloproliferative neoplasms [abstract]. Blood. 2014;124(21):5577.
- Taussig DC, Pearce DJ, Simpson C, et al. Hematopoietic stem cells express multiple myeloid markers: implications for the origin and targeted therapy of acute myeloid leukemia. Blood. 2005;106(13):4086–4092.
- Perna F, Berman SH, Soni RK, et al. Integrating proteomics and transcriptomics for systematic combinatorial chimeric antigen receptor therapy of AML. Cancer Cell. 2017;32(4):506–519.
- Chauhan D, Singh AV, Brahmandam M, et al. Functional interaction of plasmacytoid dendritic cells with multiple myeloma cells: a therapeutic target. Cancer Cell. 2009;16(4):309–323.
- Tsagarakis NJ, Kentrou NA, Papadimitriou KA, et al. Acute lymphoplasmacytoid dendritic cell (DC2) leukemia: results from the Hellenic Dendritic Cell Leukemia Study Group. Leuk Res. 2010;34(4):438–446.
- Haubner S, Perna F, Köhnke T, et al. Coexpression profile of leukemic stem cell markers for combinatorial targeted therapy in AML. Leukemia. 2019;33(1):64–74.
- Ehninger A, Kramer M, Röllig C, et al. Distribution and levels of cell surface expression of CD33 and CD123 in acute myeloid leukemia. Blood Cancer J. 2014;4:e218.
- Weinberg OK, Hasserjian RP, Baraban E, et al. Clinical, immunophenotypic, and genomic findings of acute undifferentiated leukemia and comparison to acute myeloid leukemia with minimal differentiation: a study from the bone marrow pathology group. Mod Pathol. 2019;32(9):1373–1385.
- Jain S, Mehta A, Kapoor G, et al. Evaluating new markers for minimal residual disease analysis by flow cytometry in precursor B lymphoblastic leukemia. Indian J Hematol Blood Transfus. 2018;34(1):48–53.
- Angelova E, Audette C, Kovtun Y, et al. CD123 expression patterns and selective targeting with a CD123-targeted antibody-drug conjugate (IMGN632) in acute lymphoblastic leukemia. Haematologica. 2019;104(4):749–755.
- Du W, Li J, Liu W, et al. Interleukin-3 receptor α chain (CD123) is preferentially expressed in immature T-ALL and may not associate with outcomes of chemotherapy. Tumor Biol. 2016;37(3):3817–3821.
- Lucas N, Duchmann M, Rameau P, et al. Biology and prognostic impact of clonal plasmacytoid dendritic cells in chronic myelomonocytic leukemia. Leukemia. 2019;33(10):2466–2480.
- Vitte F, Fabiani B, Bénet C, et al. Specific skin lesions in chronic myelomonocytic leukemia: a spectrum of myelomonocytic and dendritic cell proliferations: a study of 42 cases. Am J Surg Pathol. 2012;36(9):1302–1316.
- Dunphy CH. Comparative analysis of detecting monocytic cells and their aberrancy. Appl Immunohistochem Mol Morphol. 2011;19(4):336–340.
- Orazi A, Chiu R, O'Malley DP, et al. Chronic myelomonocytic leukemia: the role of bone marrow biopsy immunohistology. Mod Pathol. 2006;19(12):1536–1545.
- Del Giudice I, Matutes E, Morilla R, et al. The diagnostic value of CD123 in B-cell disorders with hairy or villous lymphocytes. Haematologica. 2004;89(3):303–308.
- Shao H, Calvo KR, Grönborg M, et al. Distinguishing hairy cell leukemia variant from hairy cell leukemia: development and validation of diagnostic criteria. Leuk Res. 2013;37(4):401–409.
- Pelland K, Mathews S, Kamath A, et al. Dendritic cell markers and PD-L1 are expressed in mediastinal gray zone lymphoma. Appl Immunohistochem Mol Morphol. 2018;26(10):e101–e106.
- Aldinucci D, Poletto D, Gloghini A, et al. Expression of functional interleukin-3 receptors on Hodgkin and Reed-Sternberg cells. Am J Pathol. 2002;160(2):585–596.
- Stevens BM, Khan N, D'Alessandro A, et al. Characterization and targeting of malignant stem cells in patients with advanced myelodysplastic syndromes. Nat Commun. 2018;9(1):3694.
- Li LJ, Tao JL, Fu R, et al. Increased CD34 + CD38 -CD123 + cells in myelodysplastic syndrome displaying malignant features similar to those in AML. Int J Hematol. 2014;100(1):60–69.
- Bao Y, Hu W, Guo Y, et al. Phenotypic characterization of malignant progenitor cells in patients with idiopathic myelofibrosis. Hematol Oncol Stem Cell Ther. 2019;12(3):146–154.
- Ratta M, Fagnoni F, Curti A, et al. Dendritic cells are functionally defective in multiple myeloma: the role of interleukin-6. Blood. 2002;100(1):230–237.
- Dasilva-Freire N, Mayado A, Teodosio C, et al. Bone marrow mast cell antibody-targetable cell surface protein expression profiles in systemic mastocytosis. IJMS. 2019;20(3):552.
- Kandeel EZ, Madney Y, Eldin DN, et al. Overexpression of CD200 and CD123 is a major influential factor in the clinical course of pediatric acute myeloid leukemia. Exp Mol Pathol. 2021;118:104597.
- Eisenwort G, Sadovnik I, Keller A, et al. Phenotypic characterization of leukemia-initiating stem cells in chronic myelomonocytic leukemia. Leukemia. 2021.
- Laribi K, Baugier de Materre A, Sobh M, et al. Blastic plasmacytoid dendritic cell neoplasms: results of an international survey on 398 adult patients. Blood Adv. 2020;4(19):4838–4848.
- Pemmaraju N, Lane AA, Sweet KL, et al. Tagraxofusp in blastic plasmacytoid dendritic-cell neoplasm. N Engl J Med. 2019;380(17):1628–1637.
- Pemmaraju N, Lane A, Sweet K, et al. Tagraxofusp, a CD123-targeted therapy, in patients with blastic plasmacytoid dendritic cell neoplasm (BPDCN): results of a landmark clinical trial [abstract]. Clin Lymphoma Myeloma Leuk. 2020;20(Suppl. 1):S209–S210.
- Sun W, Liu H, Kim Y, et al. First pediatric experience of SL-401, a CD123-targeted therapy, in patients with blastic plasmacytoid dendritic cell neoplasm: report of three cases. J Hematol Oncol. 2018;11(1):61.
- Bashir Q, Rezvani K, Saini N, et al. Phase 2 study of tagraxofusp therapy for BPDCN patients post-autologous or post-allogeneic hematopoietic cell transplantation [abstract. Blood. 2020;136(Supplement 1):5–5. ].
- Tefferi A, Cervantes F, Mesa R, et al. Revised response criteria for myelofibrosis: International Working Group-Myeloproliferative Neoplasms Research and Treatment (IWG-MRT) and European LeukemiaNet (ELN) consensus report. Blood. 2013;122(8):1395–1398.
- Mesa RA, Schwager S, Radia D, et al. The Myelofibrosis Symptom Assessment Form (MFSAF): an evidence-based brief inventory to measure quality of life and symptomatic response to treatment in myelofibrosis. Leuk Res. 2009;33(9):1199–1203.
- Pemmaraju N, Gupta V, Ali H, et al. A multicenter phase 1/2 clinical trial of tagraxofusp, a CD123-targeted therapy, in patients with poor-risk primary and secondary myelofibrosis [poster #137572]. Poster session presented at 62nd American Society of Hematology Annual Meeting and Exposition; 2020 Dec 5–8.
- Patnaik M, Ali H, Yacoub A, et al. Interim results from an ongoing phase 1/2 clinical trial of tagraxofusp, a CD123-targeted therapy, in patients with chronic myelomonocytic leukemia (CMML) [abstract. Clin Lymphoma Myeloma Leuk. 2020;20(Suppl. 1):S339. ].
- Richardson PG, Htut M, Gasparetto C, et al. Results from phase 1/2 trial of tagraxofusp in combination with pomalidomide and dexamethasone in relapsed or refractory multiple myeloma [abstract]. Blood. 2019;134(Supplement_1):3145.
- Ray A, Song Y, Du T, et al. A high throughput quantitative seroproteomics analysis of multiple myeloma patients on tagraxofusp therapy identifies novel cytokine-assisted mechanism of action [abstract]. Blood. 2020;136(Supplement 1):34.
- Kubasch AS, Schulze F, Götze KS, et al. Anti-CD123 targeted therapy with talacotuzumab in advanced MDS and AML after failing hypomethylating agents - final results of the Samba trial [abstract]. Blood. 2018;132(Supplement 1):4045.
- Kubasch AS, Schulze F, Giagounidis A, et al. Single agent talacotuzumab demonstrates limited efficacy but considerable toxicity in elderly high-risk MDS or AML patients failing hypomethylating agents. Leukemia. 2020;34(4):1182–1186.
- Montesinos P, Roboz GJ, Bulabois CE, et al. Safety and efficacy of talacotuzumab plus decitabine or decitabine alone in patients with acute myeloid leukemia not eligible for chemotherapy: results from a multicenter, randomized, phase 2/3 study. Leukemia. 2021;35(1):62–74.
- Cuesta-Mateos C, Alcaraz-Serna A, Somovilla-Crespo B, et al. Monoclonal antibody therapies for hematological malignancies: not just lineage-specific targets. Front Immunol. 2017;8:1936.
- Kovtun Y, Jones GE, Adams S, et al. A CD123-targeting antibody-drug conjugate, IMGN632, designed to eradicate AML while sparing normal bone marrow cells. Blood Adv. 2018;2(8):848–858.
- Daver NG, Montesinos P, DeAngelo DJ, et al. Clinical profile of IMGN632, a novel CD123-targeting antibody-drug conjugate (ADC), in patients with relapsed/refractory (R/R) acute myeloid leukemia (AML) or blastic plasmacytoid dendritic cell neoplasm (BPDCN) [abstract]. Blood. 2019;134(Supplement_1):734–734.
- Pemmaraju N, Martinelli G, Todisco E, et al. Clinical profile of IMGN632, a novel CD123-targeting antibody-drug conjugate (ADC), in patients with relapsed/refractory (R/R) blastic plasmacytoid dendritic cell neoplasm (BPDCN) [abstract]. Blood. 2020;136(Supplement 1):11–13.
- Vadakekolathu J, Lai C, Reeder S, et al. TP53 abnormalities correlate with immune infiltration and associate with response to flotetuzumab immunotherapy in acute myeloid leukemia [abstract]. Blood Adv. 2020;4(20):5011–5024.
- Immunogen. ImmunoGen announces FDA Breakthrough Therapy designation for IMGN632 in relapsed or refractory blastic plasmacytoid dendritic cell neoplasm [Internet]. Investors & Media; 2020 Oct 5 [cited 2020 Nov 20]. [1 page]. Available from: https://investor.immunogen.com/news-releases/news-release-details/immunogen-announces-fda-breakthrough-therapy-designation-imgn632
- Daver N, Sweet KL, Montesinos P, et al. A phase 1b/2 study of IMGN632, a CD123-targeting antibody-drug conjugate (ADC), as monotherapy or in combination with venetoclax and/or azacitidine for patients with CD123-positive acute myeloid leukemia [abstract]. Blood. 2020;136(Supplement 1):50–51.
- Li F, Sutherland MK, Yu C, et al. Characterization of SGN-CD123A, a potent CD123-directed antibody-drug conjugate for acute myeloid leukemia. Mol Cancer Ther. 2018;17(2):554–564.
- Kirchhoff D, Stelte-Ludwig B, Lerchen HG, et al. IL3RA-targeting antibody-drug conjugate BAY-943 with a kinesin spindle protein inhibitor payload shows efficacy in preclinical models of hematologic malignancies. Cancers. 2020;12(11):3464.
- Campagne O, Delmas A, Fouliard S, et al. Integrated pharmacokinetic/pharmacodynamic model of a bispecific CD3xCD123 DART molecule in nonhuman primates: evaluation of activity and impact of immunogenicity. Clin Cancer Res. 2018;24(11):2631–2641.
- Guy DG, Uy GL. Bispecific antibodies for the treatment of acute myeloid leukemia. Curr Hematol Malig Rep. 2018;13(6):417–425.
- Uy GL, Godwin J, Rettig MP, et al. Preliminary results of a phase 1 study of flotetuzumab, a CD123 × CD3 bispecific Dart® protein, in patients with relapsed/refractory acute myeloid leukemia and myelodysplastic syndrome [abstract]. Blood. 2017;130(Suppl_1):637–637.
- Slavkovic Lukic D, Duell J, Davidson-Moncada J, et al. Combination of flotetuzumab, a CD123 × CD3 bispecific DART® molecule, and blinatumomab, a CD19 X CD3 BiTE molecule, to prevent antigen escape in B-cell precursor acute lymphoblastic leukemia [abstract]. HemaSphere. 2019;3(S1):29–30.
- Wei AH, Fong CY, Montesinos P, et al. A phase 1 study of flotetuzumab, a CD123 x CD3 DART® protein, combined with MGA012, an anti-PD-1 antibody, in patients with relapsed or refractory acute myeloid leukemia [abstract]. Blood. 2019;134(Supplement_1):2662.
- Boyiadzis MM, Dhodapkar MV, Brentjens RJ, et al. Chimeric antigen receptor (CAR) T therapies for the treatment of hematologic malignancies: clinical perspective and significance. J Immunother Cancer. 2018;6(137)
- Yu S, Yi M, Qin S, et al. Next generation chimeric antigen receptor T cells: safety strategies to overcome toxicity. Mol Cancer. 2019;18(1):125.
- Budde L, Song JY, Del Real M, et al. CD123CAR displays clinical activity in relapsed/refractory (r/r) acute myeloid leukemia (AML) and blastic plasmacytoid dendritic cell neoplasm (BPDCN): safety and efficacy results from a phase 1 study [abstract]. Proceedings of the AACR Special Conference on Tumor Immunology and Immunotherapy; 2018 Nov 27–30; Miami Beach, FL. Philadelphia (PA): AACR; Cancer Immunol Res; 2020. 8(4 Suppl.):PR14.
- Cellectis. Cellectis’ UCART123 administered to first patient with BPDCN in phase I clinical trial at MD Anderson Cancer Center [Internet]. 2017 Aug 17 [cited 2020 Nov 20]; Press releases [1 page]. Available from: https://www.cellectis.com/en/press/cellectis-ucart123-administered-to-first-patient-with-bpdcn-in-phase-i-clinical-trial-at-md-anderson-cancer-center/
- Roboz GJ, DeAngelo DJ, Sallman DA, et al. Ameli-01: phase I, open label dose-escalation and dose-expansion study to evaluate the safety, expansion, persistence and clinical activity of UCART123 (allogeneic engineered T-cells expressing anti-CD123 chimeric antigen receptor), administered in patients with relapsed/refractory acute myeloid leukemia [abstract]. Blood. 2020;136(Supplement 1):41–42.
- Loff S, Dietrich J, Meyer JE, et al. Rapidly switchable universal CAR-T cells for treatment of CD123-positive leukemia. Mol Ther Oncolytics. 2020;17:408–420.
- Pemmaraju N. Novel pathways and potential therapeutic strategies for blastic plasmacytoid dendritic cell neoplasm (BPDCN): CD123 and beyond. Curr Hematol Malig Rep. 2017;12(6):510–512.
- Pemmaraju N, Konopleva M. Approval of tagraxofusp-erzs for blastic plasmacytoid dendritic cell neoplasm. Blood Adv. 2020;4(16):4020–4027.
- Pemmaraju N. Targeting the p-D-C: easy as C-D-1-2-3? Blood. 2021;137(10):1277–1278.
- Xiao W, Chan A, Waarts MR, et al. Plasmacytoid dendritic cell expansion defines a distinct subset of RUNX1-mutated acute myeloid leukemia. Blood. 2021;137(10):1377–1391.
- Beird HC, Khan M, Wang F, et al. Features of non-activation dendritic state and immune deficiency in blastic plasmacytoid dendritic cell neoplasm (BPDCN). Blood Cancer J. 2019;9(12):99.
- Togami K, Pastika T, Stephansky J, et al. DNA methyltransferase inhibition overcomes diphthamide pathway deficiencies underlying CD123-targeted treatment resistance. J Clin Invest. 2019;129(11):5005–5019.
- Brudno JN, Kochenderfer JN. Recent advances in CAR T-cell toxicity: mechanisms, manifestations and management. Blood Rev. 2019;34:45–55.