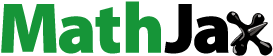
Abstract
Multiple myeloma (MM) is a malignant, incurable neoplastic disease. The currently used treatment significantly improves the prognosis and extends the survival time of patients. Unfortunately, a common side effect of the therapy is peripheral neuropathy, which may lead to dose reduction or complete treatment discontinuation/modification. In this study, we examined the changes in plasma levels of circulating miRNAs in myeloma patients to define potential factors characteristic for drug-induced peripheral neuropathy (DiPN). Global miRNA expression profile in the plasma of patients with MM during treatment was determined using miRNA microarray technology. Receiver operating characteristic (ROC) analysis allowed the identification of three miRNAs (miR-22-3p; miR-23a-3p; miR-24-3p) that could be a potential biomarker of PN. The most promising results were obtained for miR-22-3p, which was characterized by ROC area under curve (AUC) = 0.807. Our results suggest a relationship between the DiPN in patients with MM and the level of selected miRNAs in the plasma.
Keywords:
1. Introduction
Multiple myeloma (MM) is a systemic disease caused by the multifocal proliferation of plasma cells capable of producing monoclonal, homogeneous immunoglobulins which genes have been recombined or somatically hypermutated. The conversion of normal plasmocytes into an expansive myeloma clone occurs as a result of the disturbance of various processes, i.e. damage of the cytokine network, angiogenesis as well as chromosomal aberrations (e.g. monosomy 13, 14q32 translocation) and genetic changes including ras/p53. MM is the third most common neoplastic disease of the lymphoid system in adults [Citation1]. Roughly 30% of cases are asymptomatic, and the diagnosis is made randomly during routine laboratory tests, usually noting hypercalcemia, anemia, excessive plasma viscosity and coagulopathy. In the remaining 70% of cases, patients most often report bone pain, recurrent infections, nephro- or neuropathy. The course of the disease and the survival time of patients are very heterogenous [Citation2].
The introduction of new drugs, including proteasome inhibitors such as bortezomib and carfilzomib or immunomodulatory drugs i.e. thalidomide, lenalidomide, pomalidomide for the treatment of MM was a significant breakthrough that improved prognosis and prolonged the average survival time of patients [Citation3,Citation4]. Like the vast majority of drugs, bortezomib and thalidomide have a specific spectrum of side effects. Particular attention is paid to drug-induced peripheral neuropathy (DiPN), mainly due to the frequency of symptoms and their inconvenience for patients. DiPN symptoms are observed in approximately 40% of myeloma patients treated with bortezomib [Citation5]. The incidence of bortezomib-induced peripheral neuropathy depends on schedule, dose, and route of administration [Citation6,Citation7]. Common forms of bortezomib-induced peripheral neuropathy include cold hypesthesia, allodynia, heat sensitivity, painful paresthesia and motor impairment [Citation8]. In addition, studies by Terpos et al. have shown that the method of administration of bortezomib is related to the degree of neuropathy development. Subcutaneous administration of the drug reduced the development of PN by 15% compared to prior intravenous administration [Citation7]. In contrast, symptoms of PN during treatment with thalidomide affect approximately 70% of patients with long-term exposure (approximately 12 months). The overall incidence of thalidomide-induced peripheral neuropathy is 25 to 83%, leading to treatment discontinuation in approximately 15% of cases [Citation9]. Patients usually have symptoms associated with bilateral and symmetrical sensory disorders, seldom motor disorders or dysautonomia. The clinical manifestations include trembling, numbness or stinging sensations, distal paresthesia/hyperesthesia, which initially affects the toes and may extend proximally [Citation10].
DiPN in patients with MM is a common and serious clinical problem. Its pathomechanism is not fully understood, and discovery of PN predicting factor would allow quicker diagnosis and elimination of this side effect. The optimal marker would be an objectively measurable biological factor, such as a substance, physiological property, gene, or miRNA that indicates the presence of a disease, physiological or mental disorder. It could also be used to control the body’s response to therapeutic actions [Citation11]. There are several types of markers: diagnostic, monitoring, pharmacodynamic/response, predictive, and prognostic [Citation12]. microRNAs (miRNAs) are small, non-coding RNAs (17–25 nucleotides) that are engaged in regulating a range of physiological and pathological processes. Additionally, circulating miRNAs are useful in diagnostics as biomarkers for many diseases such as cancers, viral infections, nervous system disorders, diabetes [Citation13]. The miRNA may also be a marker of the efficacy of bortezomib-based therapy in MM patients. Identification of the expression of miR-215-5p, miR-181a-5p and miR-376c-3p in the blood of MM patients allows to distinguish between susceptible and treatment-resistant patients [Citation14].
In this study, we examined the clinical data of our MM patients and their miRNAs expression profiles, both individually and in combination, in order to look for possible associations which could be used as predictors of the development of drug-induced-peripheral neuropathy. According to the current knowledge, this is the first such study being an introduction to further search for the marker of DiPN.
2. Materials and methods
2.1. Subjects and initial management
The study was conducted on 60 patients with MM enrolled from Department of Bone Marrow Transplantation, Pomeranian Medical University, Szczecin, Poland and Chair and Department of Haematooncology and Bone Marrow Transplantation, Medical University of Lublin, Lublin, Poland. Prior to the enrollment, all patients signed informed consent in accordance with the Declaration of Helsinki.
PN was assessed and graded in MM patients during treatment based on a medical anamnesis and physical examination conducted in accordance with the guidelines of the International Myeloma Working Group (IMWG) using National Cancer Institute Common Terminology Criteria for Adverse Events (NCI-CTCAE) scale [Citation15].
2.2. Material
Peripheral blood samples (∼7.5 ml) collected in EDTA tubes were centrifuged (2000 rpm, 4 °C, 10 min), then the plasma was collected in a new tube and centrifuged again under the same conditions. miRNA was isolated from plasma (900 µl) using the NucleoSpin miRNA Plasma, Mini kit for circulating miRNA (Macherey-Nagel, Düren, Germany). Isolation was performed according to the manufacturer’s protocol. The concentration of miRNA isolated from plasma was determined using the Qubit 4 Fluorometer (Thermo Fisher Scientific, Waltham, MA, USA) and the Qubit™ microRNA Assay Kit (Thermo Fisher Scientific, Waltham, MA, USA).
2.3. miRNA analysis
2.3.1. Affymetrix_GeneChip miRNA microarray and data analysis
miRNA was isolated from plasma of all patients. However, miRNA microarrays were made only on representative samples. Affymetrix miRNA 4.1 Array Stripes (Affymetrix, Santa Clara, CA, USA) were used for study and performed in n = 3 technical replicates for each miRNA probe. Microarrays were made and analyzed according to the methods described in the previous articles [Citation16,Citation17].
2.3.2. qRT-PCR
The results of the miRNA microarray allowed the separation of specific miRNAs whose expression was altered in plasma of myeloma patients with symptoms of DiPN in comparision to asymptomatic patients. Expressions of selected miRNAs (miR-191-5p, miR-23a-3p, miR-24-3p, miR-26a-5p, miR-17-5p, miR-185-5p, miR-92a-3p, miR-22-3p, miR-486-5p) were examined by qRT-PCR on samples from all patients.
First, miRNA reverse-transcription was carried out using qScript microRNA cDNA Synthesis Kit (Quanta Biosciences, Beverly, MA, USA), following the manufacturer’s instructions. Then, all primers for miRNAs were designed by miRPrimer, and purchased from the Laboratory of DNA Sequencing and Oligonucleotide Synthesis (Institute of Biochemistry and Biophysics, Polish Academy of Sciences, Warsaw, Poland). The qRT-PCR program contained: 10-min initial denaturation at 95 °C; denaturation at 95 °C for 15 s; annealing at 54–57 °C (depending on the primer Supplemental Table S1) for 30 s; and extension at 30 °C for 60 s. The relative gene expression was quantified using the comparative Ct method (2ΔCt, where ΔCt = [Ct of miRNA] − [Ct of endogenous control miR93]). All products were characterized by high specificity, which was checked by determining melting points (0.1 °C/s transition rate). The mixture for the qRT-PCR reaction consisted of: 5 μL PerfeCTa SYBR Green SuperMix (Bio-Rad Inc., Hercules, CA, USA), 1 μL microRNA cDNA, 0.2 μL microRNA specific primer (Supplemental Table S1), 0.2 μL PerfeCTa Universal PCR Primer, 4.6 μL nuclease-free water. Reaction was carried out on a Bio-Rad CFX96 Real-Time PCR Detection System (Bio-Rad Inc., Hercules, CA, USA). All miRNAs expression were performed in n = 2 technical replicates.
2.4. Statistical analysis
Mann-Whitney test was used to compare quantitative parameters between groups, while Spearman’s rank correlation coefficient (Rs) was calculated to measure the strength of associations between miRNA expression levels and quantitative clinical characteristics. Fisher’s exact test was used to compare qualitative parameters between groups. A multivariate analysis of treatment-induced peripheral neuropathy as a dependent variable was performed using logistic regression with logarithmically transformed miRNA expression values as independent variables. Odds ratio values with 95% confidence intervals (95% CI) were calculated to measure the strength of associations between independent variables and presence of PN. Receiver-operating characteristic (ROC) curves and area under the ROC curve (AUC) were used to assess the diagnostic value of miRNAs as biomarkers of peripheral neuropathy. p < 0.05 was considered statistically significant. Statistica 13 software (Dell Inc., OK, United States) was used for statistical analyses.
3. Results
3.1. Study group characteristics
The patients were divided into two groups based on the presence of symptoms of peripheral neuropathy related to treatment. The most common scheme (36 patients from both groups) was VTD (bortezomib, thalidomide, dexamethasone). The remaining patients were treated with the following regimens: n = 8 VMP (bortezomib, melphalan, prednisone); n = 3 VD (bortezomib, dexamethasone); n = 3 VCD (bortezomib, cyclophosphamide, dexamethasone); n = 1 VRD (lenalidomide, bortezomib, dexamethasone), RD n = 10 (lenalidomide, dexamethasone). 32 patients had symptoms of neuropathy while 28 were classified as a control group without symptoms.
PN was determined according to the NCI-CTCAE scale, which includes three types of neuropathy. 66.66% of enrolled patients from the neuropathic group had sensory neuropathy, 16.66% sensory-autonomic, and 16.66% sensorimotor, 12.50 of patients had grade 1 sensory neuropathy, 65.52% grade 2, and 21.87 grade 3 according to toxic sensory neuropathy criteria (sNCI-CTCAE). Furthermore, 65.62% of enrolled patients with neuropathy were treated with the VTD regimen, 15.62% VD; 9.37% RD, and 9.37% VCD. The time to onset of DiPN was very heterogeneous in patients enrolled in the study. Neuropathy appeared at the earliest after the first cycle of treatment and at the latest after the 23rd cycle. Taking into account only the VTD regimen, which was the most frequently used regimen in the neuropathic group, the minimum number of cycles needed to develop neuropathy was 1 and the maximum number was 6 cycles. Moreover, we observed that patients with grade 3 neuropathy, compared to other patients with lower grade neuropathy, showed higher expression of three miRNAs: (a) miR-23a-3p ( 6497.13) was higher by ∼19%; (b) miR-24-3p expression (
4288.66) was higher by ∼25%; (c) miR-22-5p expression (
8536.5) was higher by ∼7.5%. However, our observation requires confirmation in a greater number of patients with grade 3 neuropathy. A complete blood count analysis, Ig levels, FLCs kappa and lambda concentration were performed in all enrolled subjects ().
Table 1. Clinical characteristics of the study groups.
3.2. miRNAs expression profile in plasma
3.2.1. Microarray analysis
Microarray analysis showed that the expression of 7 miRNAs was down regulated at least two-fold (log2_fold change −13.63 to −2) and the expression of 65 miRNAs was up regulated at least two-fold (log2_fold change 112.26 to 2) in plasma samples from myeloma group with neuropathy compared to control group (Figure S1). The detailed fold values of most 17 up- or down regulated miRNAs are presented in Supplemental Table S2.
3.2.2. David miRNA analysis
miRNAs with altered expression were assigned to specific biological processes/function according to the gene ontology classification (GO). The bubble plot illustrates the overrepresented terms (Supplemental Figure S2). Analysis of functional annotations identified 306 processes (criteria: adjusted p < 0.05; Benjamini method; minimum number of miRNAs per group = 5; bubble size indicates the number of genes represented in corresponding annotation).
The most important processes are related to the nervous system (GO:0051402∼neuron apoptotic process; GO:0043524∼negative regulation of neuron apoptotic process; GO:0097150∼neuronal stem cell population maintenance; GO:0001843∼neural tube closure) and apoptosis (GO:2001243∼negative regulation of intrinsic apoptotic signaling pathway; GO:2001237∼negative regulation of extrinsic apoptotic signaling pathway; GO:1902042∼negative regulation of extrinsic apoptotic signaling pathway via death domain receptors; GO:0097193∼intrinsic apoptotic signaling pathway; GO:0043066∼negative regulation of apoptotic process; GO:0008637∼apoptotic mitochondrial changes).
3.2.3. qRT-PCR analysis
The results of the miRNA microarray allowed the separation of specific miRNAs whose expression was altered in the plasma of myeloma patients with symptoms of DiPN compared with asymptomatic patients. Expressions of selected miRNAs (miR-191-5p, miR-23a-3p, miR-24-3p, miR-26a-5p, miR-17-5p, miR-185-5p, miR-92a-3p, miR-22-3p, miR-486-5p) was examined by qRT-PCR on samples from all patients. Out of all analyzed miRNAs, five showed significantly higher expression in neuropathy group vs. control group: mi-R-191-5p, miR-23a-3p, miR-24-3p, miR-185-5p, miR-92a-3p, miR-22-3p (). 4 tested miRNAs (miR-191-5p; miR-23a-3p; miR-26a-5p; miR-486) showed statistical significance in the neuropathic group (p < 0.05) in relation to treatment with the VTD regimen.
Table 2. Plasma miRNA profiles in peripheral neuropathy patients and controls.
In our study, one of the largest differences in expression between the neuropathic group compared to the control group as well as the most promising marker of DiPN in patients with myeloma was miR-22-3p. According to the Target Scan Human (Prediction of microRNA targets) database, miRNA-22-3p regulates the expression of over 600 genes. Analyzing the genes regulated by miR-22-3p in the ToppGene database, showed their influence on neurogenesis (NTRK2; NYAP2; ERBB3; SNAP91), neuronal differentiation (ERBB4; GLDN; NFIB), generation of neurons (GLDN; HIPK1; WNT1), as well as the process of cell differentiation (CDX2; CEBPD; TP53INP1), chromatine organization (ESR1; EYA3; EZH1), histone modification (PER1; MECOM; EYA3).
3.3. miRNA correlations
In order to demonstrate the importance of the analyzed miRNA profiles in MM patients, the correlations between the expression of miRNAs and clinical data in the control (Supplemental Tables S3 and S4) and neuropathy group (Supplemental Tables S5 and S6) were assessed. Interestingly, we observed more relevant correlations in the control group than in the neuropathy group. Patient age, total protein, IgA and IgM concentration were not statistically significantly correlated with any miRNAs. We observed the most significant correlations between miRNAs expression and IgG and FLCs lambda concentrations. miRNA-191-5p and miRNA-26a-5p were negatively correlated with IgG and positively with FLCs lambda, while miRNA-92a-3p and miRNA-486-5p were positively correlated with both IgG, FLCs lambda and WBC (Supplemental Table S3). Additionally, an analysis of the correlation between the miRNAs themselves was performed. The most significant correlations were observed for_miRNA-191-5p, miRNA-23a-3p, miRNA-26a-5p, miRNA-24-3p, miRNA-486-5p (Supplemental Table S4).
Correlation analysis in the group with neuropathy showed only three statistically significant correlations between miRNA and clinical data. miRNA-191-5p and miRNA-26a-5p were positively correlated with total protein and only miRNA-191-5p was also positively correlated with FLCs kappa (Supplemental Table S5).
However, many statistically significant correlations miRNA-miRNA were observed and a lot of them were characteristic only for the neuropathy group (Supplemental Table S6; marked with gray color).
The most statistically significant correlations were observed for miR-22-3p, which additionally were unique only for the group with neuropathy (Supplemental Table S6).
3.4. ROC analysis
Next, we performed Receiver Operating Characteristic (ROC) analysis. The Area Under the Curve (AUC) of ROC plots show the discriminatory ability of the biomarkers. Graphs present sensitivity on the Y axis and 1-specificity on the X axis. The maximum value for the AUC is 1.0 (which means 100% sensitive and 100% specific). ROC curves with an AUC > 0.7 are clinically useful. ROC analysis showed three miRNAs with AUC > 0.7 () (Figure S3). miRNA-22-3p was the most promising marker of drug-induced neuropathy in myeloma patients (AUC = 0.807, p = 0.00000003, Youden’s index = 0.49).
Table 3. ROC analysis results.
3.5. Multiple logistic regression
As a final part of our analysis, we performed multiple logistic regression. Multivariate analysis revealed that two pairs of logarithmically transformed miRNA expression results (miRNA-24a-3p and miRNA-22-3p; miRNA-191-5p and miRNA-22-3p), both containing miRNA-22-3p, can be considered as strong risk factors independently associated with treatment-induced neuropathy in myeloma patients. In the first model, expression of miRNA higher by e times (2.718-fold) was associated with 6.17-times higher odds of neuropathy for miRNA-24-3p and 10.38-times higher odds of neuropathy for miRNA-22-3p. In the second model, e-times higher miRNA expression was associated with 5.78-times higher odds of neuropathy for 191-5p and 33.89-times higher odds of neuropathy for miRNA-22-3p (). miRNA-24-3p and 191-5p were not independent markers of neuropathy, since the significance of their association with neuropathy was lost when they were included into the same logistic regression model. The above observation is connected with a strong positive correlation between their expression in the control group (Supplemental Table S4). Moreover, statistical multiple logistic regression analysis revealed a relationship between treatment with the VTD regimen and selected pairs miRNAs from the first and second model, which can be considered a strong risk factors for neuropathy in patients with MM. As a result, we identified two additional models. Thus, in the third model, treatment with the VTD regimen was associated with a 5.22-times higher odds of neuropathy, while higher miRNAs expression was associated with a 5.09-times higher odds of neuropathy for miR-191-5p and 38.67-times higher for miR-22-3p. In the fourth model, treatment with the VTD regimen was associated with a 7.77-times higher odds of neuropathy, while higher miRNAs expression was associated with a 7.4-times higher odds of neuropathy for miR-24-3p and 12.3-times higher for miR-22-3p ().
Table 4. Multivariate logistic regression models with logarithmically transformed miRNA expression levels as independent variables and neuropathy as dependent variable.
4. Discussion
miRNAs are one of the epigenetic mechanisms that cause changes in gene expression without altering the nucleotide sequence of DNA. All epigenetic modifications are reversible and can be inherited [Citation18]. Epigenetic modifications can be induced by external factors, such as treatment, diet, climate, stress [Citation19], as well as by permanent changes in the DNA sequence, such as single nucleotide polymorphisms (SNPs) which can generate changes in the expression of factors necessary for epigenetic processes, such as methylation/demethylation, acetylation/deacetylation or miRNAs expression [Citation20].
miRNAs perform various functions in the body. Primarily, they are involved in the regulation of the expression of majority of genes. However, research conducted in this area has revealed their function as a biomarker of many diseases [Citation21] e.g. breast cancer [Citation22], head and neck squamous cell carcinoma [Citation23], pancreatic cancer [Citation24] and showed the potential to apply them in the treatment of various diseases [Citation25] e.g. Huntington’s disease [Citation26], liver cancer [Citation27], malignant pleural mesothelioma [Citation28], melanoma [Citation29], cardiological diseases [Citation30], Alzheimer’s disease [Citation31].
Due to the potential of miRNAs as biomarkers, in this study we attempted to identify specific miRNAs in the plasma of MM patients with DiPN. Our research was based on the identification of a broad panel of miRNAs using the miRNA microarray method, which then allowed to pinpoint several miRNAs that could potentially serve as neuropathy biomarkers. Then, selected miRNAs were assessed in the plasma of all patients recruited into the study and extensive statistical analysis was performed. Using ROC analysis, we identified 3 independent miRNAs with a diagnostically valuable AUC > 0.7 (miR-22-3p, miR-23a-3p, miR-24-5p) and thus with a neuropathy biomarker potential. However, using multiple logistic regression, two specific pairs of miRNAs (miRNA-24-3p and miRNA-22-3p; miRNA-191-5p and miRNA-22-3p) were identified featuring strong risk factors independently associated with DiPN in myeloma patients. According to the available literature, there is currently no information about changes in the expression levels of miRNAs in the plasma of myeloma patients with symptoms of drug-induced neuropathy. However, in some cases, damage to the nervous system causes an increase in the level of individual miRNAs that are expected to have a neuroprotective effect on the nervous system [Citation32]. Our bioinformatics analysis of the obtained results revealed the involvement of altered miRNAs in processes related to the nervous system, in particular negative regulation of neuron apoptotic process and neuronal stem cell population maintenance.
In a mouse model study of chemotherapy-induced peripheral neuropathy, an increase in the level of miR-124 in plasma was observed, which is one of the important factors regulating neurogenesis and plasticity of neurons [Citation33]. However, in our study in MM patients with DiPN, we observed no difference in the expression levels of this miRNA between analyzed groups. On the other hand, miR-22 inhibits neuronal apoptosis by reducing the expression of pro-apoptotic proteins, including Tp53inp1 and MAPK14/p38 [Citation32]. In vitro studies by Jovicic et al. showed the neuroprotective potential and neuroregenerative role of miR-22, through a reduction of neurodegeneration in primary neuronal cultures exposed to 3-nitropropionic acid (3-NP) and increase in neuronal viability in an in vitro model of brain aging [Citation34]. Parallelly, the neuroprotective effect of miR-22 was also demonstrated in a mouse model [Citation35]. miR-23a also exhibits neuroprotective effects by promoting the synthesis of the myelin sheath in the nervous system [Citation36] and inhibiting neuronal apoptosis [Citation37]. Moreover, studies conducted by Pan et al. showed the influence of miR-23a-3p on the regulation of neuropathic pain. Increased levels of miRNA-23a-3p inhibit the CXC chemokine receptor 4 (CXCR4) in spinal glial cells, which is directly responsible for neuropathic pain.
miR-17-92 cluster (containing: miR-17, miR-18a, miR-19a, miR-20a, miR-19b-1, and miR-92a-1) is an important factor regulating neurogenesis, neuronal differentiation and showing great potential in the treatment of neurodegenerative diseases [Citation38,Citation39]. In our study, we observed an increase in the expression of most of the miRNAs in this family. The increase in expression of miR-18a (fold 10.02), miR-19a (fold 22.99), miR-20a (fold 13.05), miR-92a (fold 60.85), miR-17 (fold 45.68) was observed by analyzing the results from the miRNA array, while the expression of two of them (miR-92a-3p and miR-17-5p) was additionally confirmed by qRT-PCR. miR-92a participates in proliferation of neural stem cells (NSCs) through maintaining NSC self-renewal in developing cortex [Citation40]. In the in vitro model, it was shown that deletion of the miR-17-92 cluster reduces neurogenesis, while the increase in its expression intensifies this process [Citation41]. miR-17-92 regulates neurogenesis by influencing genes related to the glucocorticoid pathway, especially by affecting the Sgk1 protein kinase. Consequently, miR-17-92 knockout mice exhibited depressive and anxiety behaviors [Citation41]. Xin et al. demonstrated that intranasal administration of miR-17-92 cluster-enriched exosomes to rats, improves neurological function and enhances oligodendrogenesis, neurogenesis and neurite remodeling /neuronal plasticity of dendrites in the ischemic boundary zone after stroke. The observed effect is probably caused by the involvement of miR-17-92 in the regulation of the phosphatidylinositol 3-kinase (PI3K) signaling pathway [Citation42]. Another interesting observation in our research is a significant increase in the expression of miR-103, which also shows neuroprotective effects. miR-103 promotes neurite growth and inhibits cell apoptosis by targeting PTGS2 gene in Alzheimer’s disease cell models [Citation43].
5. Study limitations
Our study provides interesting and innovative results, however, does not lack some drawbacks. In fact, our research could be regarded as a preliminary study and an introduction to further research on a very important clinical problem which is DiPN in patients with MM. Since our study group was relatively small, the obtained results set the direction for future research that should be confirmed on a larger cohort of patients.
6. Conclusions
In our study we observed an increase in the expression levels of miR-22-3p, miR-23a-3p, miR-17-92 cluster and miR-103 in peripheral neuropathy group that may reflect the organism’s response to the damage to the nervous system. Additionally, since several miRNAs analyzed in our study were strong risk factors independently associated with DiPN in myeloma patients, they could potentially serve as markers of the neuropathy. In this regard, miR-22-3p remains the most promising candidate from the analyzed set.
Overall, the research data available currently in the literature may open new therapeutic avenues in treating peripheral nerve injury-induced nociceptive hypersensitivity [Citation44]. The analysis of the function that these miRNAs play in various biological processes, explains the increase of their expression levels in response to damage to the nervous system observed in our study, which additionally confirms the validity of further research on these miRNAs as biomarkers of chemotherapy-induced neuropathy in myeloma patients.
GLAL-2021-0272-File014.pdf
Download PDF (89.7 KB)GLAL-2021-0272-File013.pdf
Download PDF (94.1 KB)GLAL-2021-0272-File012.pdf
Download PDF (67.6 KB)GLAL-2021-0272-File011.pdf
Download PDF (73.9 KB)GLAL-2021-0272-File010.pdf
Download PDF (77.9 KB)GLAL-2021-0272-File009.pdf
Download PDF (446.5 KB)GLAL-2021-0272-File008.pdf
Download PDF (360.4 KB)GLAL-2021-0272-File007.pdf
Download PDF (1.8 MB)GLAL-2021-0272-File006.pdf
Download PDF (95.3 KB)Disclosure statement
No potential conflict of interest was reported by the author(s).
Additional information
Funding
References
- Siegel RL, Miller KD, Jemal A. Cancer statistics, 2019. CA A Cancer J Clin. 2019;69(1):7–34.
- Dimopoulos MA, Terpos E. Multiple myeloma. Ann Oncol. 2010;21(7):43–50.
- Michels TC, Petersen KE. Multiple myeloma: diagnosis and treatment. Am Fam Physician. 2017;95(6):373–383.
- Castella M, Fernandez de Larrea C, Martin-Antonio B. Immunotherapy: a novel era of promising treatments for multiple myeloma. Int J Mol Sci. 2018;19:11.
- Richardson PG, Sonneveld P, Schuster MW, et al. Reversibility of symptomatic peripheral neuropathy with bortezomib in the phase III APEX trial in relapsed multiple myeloma: impact of a dose-modification guideline. Br J Haematol. 2009;144(6):895–903.
- Chen D, Frezza M, Schmitt S, et al. Bortezomib as the first proteasome inhibitor anticancer drug: current status and future perspectives. CCDT. 2011;11(3):239–253.
- Terpos E, Kleber M, Engelhardt M, et al. European Myeloma Network guidelines for the management of multiple myeloma-related complications. Haematologica. 2015;100(10):1254–1266.
- Duggett NA, Flatters SJL. Characterization of a rat model of bortezomib‐induced painful neuropathy. Br J Pharmacol. 2017;174(24):4812–4825.
- Prince HM, Schenkel B, Mileshkin L. An analysis of clinical trials assessing the efficacy and safety of single-agent thalidomide in patients with relapsed or refractory multiple myeloma. Leuk Lymphoma. 2007;48(1):46–55.
- Mileshkin L, Stark R, Day B, et al. Development of neuropathy in patients with myeloma treated with thalidomide: patterns of occurrence and the role of electrophysiologic monitoring. J Clin Oncol. 2006;24(27):4507–4514.
- Califf RM. Biomarker definitions and their applications. Exp Biol Med. 2018;243(3):213–221.
- Mayeux R. Biomarkers: potential uses and limitations. NeuroRx. 2004;1(2):182–188.
- Wang J, Chen J, Sen S. MicroRNA as biomarkers and diagnostics. J Cell Physiol. 2016;231(1):25–30.
- Robak P, Dróżdż I, Jarych D, et al. The value of serum MicroRNA expression signature in predicting refractoriness to bortezomib-based therapy in multiple myeloma patients. Cancers. 2020;12(9):2569.
- Richardson PG, Delforge M, Beksac M, et al. Management of treatment-emergent peripheral neuropathy in multiple myeloma. Leukemia. 2012;26(4):595–608.
- Łuczkowska K, Rogińska D, Ulańczyk Z, et al. Molecular mechanisms of bortezomib action: novel evidence for the miRNA-mRNA interaction involvement. IJMS. 2020;21(1):350.
- Łuczkowska K, Rogińska D, Ulańczyk Z, et al. Effect of bortezomib on global gene expression in PC12-derived nerve cells. IJMS. 2020;21(3):751.
- Herceg Z, Vaissière T. Epigenetic mechanisms and cancer: an interface between the environment and the genome. Epigenetics. 2011;6(7):804–819.
- Bollati V, Baccarelli A. Environmental epigenetics. Heredity. 2010;105(1):105–112.
- Zaina S, Pérez-Luque EL, Lund G. Genetics talks to epigenetics? The interplay between sequence variants and chromatin structure. Curr Genomics. 2010;11(5):359–367.
- Condrat CE, Thompson DC, Barbu MG, et al. miRNAs as biomarkers in disease: latest findings regarding their role in diagnosis and prognosis. Cells. 2020;9(2):276.
- Salahandish R, Ghaffarinejad A, Omidinia E, et al. Label-free ultrasensitive detection of breast cancer miRNA-21 biomarker employing electrochemical nano-genosensor based on sandwiched AgNPs in PANI and N-doped graphene. Biosensbioelectron. 2018;120:129–136.
- Cao Y, Green K, Quattlebaum S, et al. Methylated genomic loci encoding microRNA as a biomarker panel in tissue and saliva for head and neck squamous cell carcinoma. Clin Epigenetics. 2018;10:43.
- Vila-Navarro E, Vila-Casadesús M, Moreira L, et al. MicroRNAs for detection of pancreatic neoplasia: biomarker discovery by next-generation sequencing and validation in 2 independent cohorts. Ann Surg. 2017;265(6):1226–1234.
- Paul S, Vázquez LAB, Uribe SP, et al. Current status of microRNA-based therapeutic approaches in neurodegenerative disorders. Cells. 2020;9(7):1698.
- Monteys AM, Wilson MJ, Boudreau RL, et al. Artificial miRNAs targeting mutant huntingtin show preferential silencing in vitro and in vivo. Mol Ther Nucleic Acids. 2015;4:e234.
- Beg MS, Brenner AJ, Sachdev J, et al. Phase I study of MRX34, a liposomal miR-34a mimic, administered twice weekly in patients with advanced solid tumors. Invest New Drug. 2017;35(2):180–188.
- Van Zandwijk N, Pavlakis N, Kao SC, et al. Safety and activity of microRNA-loaded minicells in patients with recurrent malignant pleural mesothelioma: a first-in-man, phase 1, open-label, dose-escalation study. Lancet Oncol. 2017;18(10):1386–1396.
- Noguchi S, Iwasaki J, Kumazaki M, et al. Chemically modified synthetic microRNA-205 inhibits the growth of melanoma cells in vitro and in vivo. Mol Ther. 2013;21(6):1204–1211.
- Hullinger GH, Montgomery RL, Seto AG, et al. Inhibition of miR-15 protects against cardiac ischemic injury. Circres. 2012;110(1):71–81.
- Parsi S, Smith PY, Goupil C, et al. Preclinical evaluation of miR-15/107 family members as multifactorial drug targets for Alzheimer's disease. Mo Mol Ther Nucleic Acids. 2015;4(10):e256.
- Wang C, Ji B, Cheng B, et al. Neuroprotection of microRNA in neurological disorders. Biomed Rep. 2014;2(5):611–619.
- Peng Q, Mechanic J, Shoieb A, et al. Circulating microRNA and automated motion analysis as novel methods of assessing chemotherapy-induced peripheral neuropathy in mice. PLoS One. 2019;14(1):e0210995.
- Jovicic A, Jolissaint JFZ, Moser R, et al. MicroRNA-22 (miR-22) overexpression is neuroprotective via general anti-apoptotic effects and may also target specific Huntington's disease-related mechanisms. PLoS One. 2013;8(1):e54222.
- Yu S, Zeng YJ, Sun XC. Neuroprotective effects of p53/microRNA‑22 regulate inflammation and apoptosis in subarachnoid hemorrhage. Int J Mol Med. 2018;41(4):2406–2412.
- Lin ST, Huang Y, Zhang L, et al. MicroRNA-23a promotes myelination in the central nervous system. Proc Natl Acad Sci U S A. 2013;110(43):17468–17473.
- Chen Q, Xu J, Li L, et al. MicroRNA-23a/b and microRNA-27a/b suppress Apaf-1 protein and alleviate hypoxia-induced neuronal apoptosis. Cell Death Dis. 2014;5(3):e1132.
- Xia X, Wang Y, Zheng JC. The microRNA-17 ∼ 92 family as a key regulator of neurogenesis and potential regenerative therapeutics of neurological disorders. Stem Cell Rev Rep. 2020. doi: 10.1007/s12015-020-10050-5
- Cho KHT, Xu B, Blenkiron C, et al. Emerging roles of miRNAs in brain development and perinatal brain injury. Frontphysiol. 2019;10:227.
- Fei JF, Haffner C, Huttner WB. 3' UTR-dependent, miR-92-mediated restriction of Tis21 expression maintains asymmetric neural stem cell division to ensure proper neocortex size. Cell Rep. 2014;7(2):398–411.
- Jin J, Kim SN, Liu X, et al. miR-17-92 cluster regulates adult hippocampal neurogenesis, anxiety, and depression. Cell Rep. 2016;16(6):1653–1663.
- Xin H, Katakowski M, Wang F, et al. MicroRNA cluster miR-17-92 cluster in exosomes enhance neuroplasticity and functional recovery after stroke in rats. Stroke. 2017;48(3):747–753.
- Yang H, Wang H, Shu Y, et al. miR-103 promotes neurite outgrowth and suppresses cells apoptosis by targeting prostaglandin-endoperoxide synthase 2 in cellular models of Alzheimer's disease. Front Cell Neurosci. 2018;12:91.
- Pan Z, Shan Q, Gu P, et al. miRNA-23a/CXCR4 regulates neuropathic pain via directly targeting TXNIP/NLRP3 inflammasome axis. Jneuroinflamm. 2018;15(1):29.