ABSTRACT
Specialized science courses (SSCs) integrate content knowledge (CK) with pedagogical content knowledge (PCK) and prepare pre-service teachers (PSTs) for reform-oriented teaching. Studies of individual SSCs report positive short-term outcomes, including an increase in self-efficacy and CK. However, few studies explore the longer-term impact of SSCs on classroom teaching. We carried out an exploratory case study of three PSTs from a Norwegian teacher education program that included SSCs. In the context of their field practicum, we compared PSTs’ teaching of topics taught in SSCs (aligned lessons) with topics not taught in SSCs (unaligned lessons). Data collection consisted of field observations of one aligned and one unaligned lesson as well as stimulated recall interviews based on video recordings. In our analyses, we compared PSTs use of instructional strategies in aligned and unaligned lessons and how their knowledge for teaching informed these instructional decisions. We found that SSCs supported PSTs in using more topic-specific instructional strategies when teaching aligned lessons. In the aligned lessons, their teaching was better informed by knowledge of students’ understandings in science. We also examined PSTs’ perceptions of how they drew upon SSCs in their classroom teaching. They reported that SSCs had a major impact on their CK, PCK and self-efficacy for science teaching. Through this study, we provide unique insights into how PSTs draw on SSCs in their classroom teaching. We include implications for further research and the design of SSCs.
Overcoming the gap between theory and practice is a major challenge for pre-service teachers (Allsopp et al., Citation2006; Grossman et al., Citation2009). The challenge is evident in the Nordic context (Rasmussen & Dorf, Citation2010), and for the subject of science (Thompson et al., Citation2013). In reform-oriented science teaching, teachers consider students and content rather than delivery of content only, and they implement inquiry teaching (Anderson et al., Citation1994; Sawada et al., Citation2002). One challenge for teacher educators is to develop pre-service teacher’s (PSTs) theoretical and practical knowledge to prepare them to teach this way (Cochran-Smith & Villegas, Citation2016; McDonald et al., Citation2013). Another challenge occurs when PSTs attempt to introduce reform-oriented teaching practices in schools that may not be familiar with such practices (Crawford, Citation2007; Thompson et al., Citation2013).
To address these challenges, researchers and policymakers call for teacher education programs to shift toward a focus on teaching practices rather than theoretical knowledge about teaching (Blue Ribbon Panel, Citation2010; Darling-Hammond et al., Citation2017; Jenset, Citation2018). This movement provides a rationale for the inclusion of specialized science courses (SSCs) in teacher education programs. SSCs are science content courses, designed specifically for PSTs, in which instructors model reform-oriented teaching of science topics aligned with the grade levels that PSTs will teach. PSTs learn science content while also engaging in pedagogical discussions. These courses are built on the assumption that teachers tend to teach in the ways that they have been taught (Cochran-Smith & Villegas, Citation2016).
Teacher education programs can be an important starting point for developing science teachers’ pedagogical content knowledge (PCK) (Friedrichsen et al., Citation2009). However, we have scarce evidence about the effects of teacher education coursework on developing reform-oriented teaching practices (Jenset, Citation2018; Stroupe & Gotwals, Citation2018). SSCs aim to support PSTs’ development of PCK for reform-oriented science teaching. Many Nordic science teacher education programs for primary and middle school levels include SSCs. These programs focus on the development of teaching experts rather than content experts (Rasmussen & Dorf, Citation2010). However, separate science content and methods courses tend to be the international norm (Etkina, Citation2010; Fones et al., Citation1999). In this study, we addressed a gap in the literature on whether and how PSTs use what they have learned in their teacher education program (Cochran-Smith & Villegas, Citation2016; Jenset, Citation2018), specifically the influence of SSCs on PSTs’ practice. We carried out an exploratory case study of three PSTs from a Norwegian teacher education program that included SSCs. The study takes place within a subsequent field practica in a local school. We contrasted the PSTs’ teaching of topics that were taught in prior SSCs (aligned lessons) with topics not taught in their SSCs (unaligned lessons). Moon phases and seasons are an example of an aligned lesson; the PSTs learned about moon phases in a SSC and later taught this topic in their school practicum. An unaligned lesson is a lesson taught by the PST (e.g., animal cells and oxygenation); however, the PST had not learned the topic in a SSC. Specifically, we aimed to explore two questions: (1) In three Norwegian science PSTs’ practica in lower secondary school (ages 13–16), what were the differences, if any, between lessons aligned and unaligned with specialized science courses? (2) What were these PSTs’ perceptions of how they drew upon specialized science courses?
Theoretical framework
Shulman (Citation1986) proposed pedagogical content knowledge (PCK) as an important part of a teacher’s knowledge base. Teachers have specialized knowledge for teaching specific topics to specific students (Shulman, Citation1986). In the current study, PCK serves as the theoretical framework as we are examining PSTs’ specialized knowledge for teaching specific science topics. Some PCK researchers view PCK as topic-specific knowledge for teaching (e.g., how to teach a specific topic such as photosynthesis) (Gess-Newsome, Citation2015; Mavhunga, Citation2020), while others view PCK as specialized knowledge at the discipline level (e.g., how to teach argumentation in science courses) (Davis & Krajcik, Citation2005). In this study, we examine both topic-specific and science-specific PCK. Magnusson et al. (Citation1999) conceptualized topic-specific PCK as consisting of four components comprised of knowledge of science curricula, students’ understanding in science, instructional strategies, and assessment of scientific literacy. To examine science-specific PCK, we use the same Magnusson categories (Friedrichsen et al., Citation2009).
The most recent PCK model in science education () distinguishes between three realms of PCK: enacted PCK, personal PCK, and collective PCK (Carlson et al., Citation2020).
Figure 1. The refined consensus model of PCK.
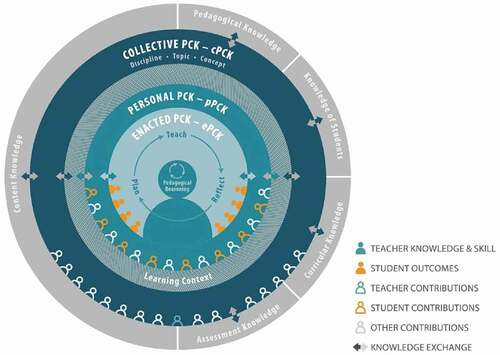
Each realm refers to the context in which PCK is manifested: in the classroom during enactment, in the individual teacher’s knowledge base, and collective knowledge held among colleagues or published in the field. The model weaves together knowledge (personal and collective PCK) and skills (enacted PCK) for science teaching. In this study, we researched personal and enacted PCK as we conducted stimulated recall interviews and related this to collective PCK from SSCs. High quality PCK is characterized by integration of PCK components (Abell, Citation2008; Chan & Hume, Citation2019). Integration between knowledge of students’ understanding of science and knowledge of instructional strategies is a critical step in developing highly integrated PCK (Akin & Uzuntiryaki-Kondakci, Citation2018; Park & Chen, Citation2012). In the current paper, this integration is studied in the realm of enacted PCK.
Literature review
SSCs are university courses designed specifically for education majors to support the development of science content knowledge (CK) and PCK. The main goal of the courses is to develop CK, while PCK is addressed through course instructors’ modeling of reform-oriented instructional practices, and by explicitly focusing on K-12 students’ common misconceptions related to the topic. In contrast, science teacher education programs are often based on content courses characterized by lectures in a science discipline, lab sessions, and separate science methods courses (Etkina, Citation2010; Fones et al., Citation1999). Such programs, though cost-effective, often provide PSTs with poorly taught CK of limited relevance for their future career and limited PCK for specific topics (Bergman & Morphew, Citation2015; Fones et al., Citation1999). Grossman et al. (Citation2009) called for teacher education to undo divisions between university and K-12 schools. One should not assume that learning about teaching practices through reading articles or writing papers is enough to prepare PSTs for classroom teaching, particularly student-centered or reform-oriented teaching (Horn & Campbell, Citation2015; Sun & van Es, Citation2015). In line with this, SSCs integrate CK and PCK in some of the following ways:
using a constructivist epistemology, requiring that learners are active in the knowledge-building process (McLoughlin & Dana, Citation1999).
being student-centered by engaging PSTs in their own learning process and connecting the course to science classroom teaching at relevant levels (Etkina, Citation2015).
implicitly learning about instructional strategies, such as through implementing scientific practices like inquiry (Bergman & Morphew, Citation2015; Cochran-Smith & Villegas, Citation2016; Knaggs & Sondergeld, Citation2015).
explicitly presenting instructional strategies and addressing issues regarding different approaches to teaching (McLoughlin & Dana, Citation1999).
using a variety of ways, building on the premise that teachers tend to teach in the same way in which they learned the content. (Avard, Citation2009; Bergman & Morphew, Citation2015; Cochran-Smith & Villegas, Citation2016).
Case studies of courses or programs using pre/posttests of content knowledge, beliefs, or teaching practices were often used in evaluating SSC outcomes. One outcome of SSCs was an increase in PSTs’ content knowledge. Studies of specialized physics courses (Etkina, Citation2010; Menon & Sadler, Citation2016) and a specialized astronomy course (Bell & Trundle, Citation2008) reported an increase in content knowledge test scores at the end of the courses. Additionally, PSTs have highlighted group work and discussion in specialized physics courses as helpful in their concept learning (Doster et al., Citation1997). PSTs with more prior knowledge in the topic were most comfortable with the reformed teaching model used in the SSC (Doster et al., Citation1997).
A second outcome is that reform-oriented learning in SSCs supported the development of PSTs’ knowledge of and approaches to teaching science. Cochran-Smith and Villegas (Citation2016) reported how PSTs adopted constructivist views of teaching and learning through experiences like problem-based learning, role playing, analyzing video cases, and performing collaborative research. Similarly, Varelas et al. (Citation2008) reported on four SSCs for elementary PSTs characterized by a focus on student understanding and a balance between attention to key concepts and science as inquiry. Participating PSTs noticed how constructivist instructional tools (i.e., concept maps, group work and projects) facilitated meaning and connection making. Although the main goal of the courses was CK learning, PSTs noticed curricular, instructional and assessment features and made connections to future classroom practice. Only one longitudinal study traced PSTs’ knowledge and practices from SSCs into classrooms. Etkina (Citation2010) evaluated a physics teacher education program featuring interactive-engagement pedagogy and frequent opportunities to practice instruction. Using an observation protocol (RTOP) over three years, she found the program supported PSTs in enacting reformed teaching practices in school practica.
A third outcome of SSCs was the development of self-efficacy for science teaching. Research has documented critical links between self-efficacy beliefs and teaching practices (Menon & Sadler, Citation2016). Several studies utilized the Science Teaching Efficacy Belief Instrument (STEBI-B) (Enochs & Riggs, Citation1990) as pre/posttests of PSTs’ self-efficacy. The studies reported gains in self-efficacy for teaching science from specialized courses in physics (Menon & Sadler, Citation2016), geoscience (Posnanski, Citation2007), and an integrated science course (Knaggs & Sondergeld, Citation2015). These gains in self-efficacy were attributed to implementation of constructivist instructional methods in the SSCs (Posnanski, Citation2007), such as using activity-based curriculum, pedagogically oriented assignments, and having opportunities to collaborate with both instructors and peers (McLoughlin & Dana, Citation1999). All of the studies reviewed above report PSTs’ self-efficacy gains at the completion of the SCCs; there were no longitudinal studies that studied PSTs’ self-efficacy as they taught in classrooms.
Need for follow-up classroom research of PSTs’ practice
Jenset (Citation2018) reported overall thin evidence of the effects of teacher education coursework due to the lack of longitudinal research on actual teaching practice. We identified only one study (Etkina, Citation2010) evaluating the influence of SSCs on PSTs’ teaching practice in actual classrooms. However, this study looked at teaching practices at a general level and did not compare classroom lessons to topics taught in the SSCs, and PSTs were not interviewed to capture a nuanced picture of the impact from the SSCs. By studying the impact of SSCs on classroom teaching in a field practicum setting, the current study addresses these gaps. The current study also is a response to calls for research on what aspects of science teacher preparation matter in terms of PSTs’ perspectives and teaching practices (Wilson et al., Citation2001; Zeichner, Citation2010).
Context
The current study was undertaken in a Norwegian five-year teacher education program for primary and lower secondary school teachers (grade 5–10, ages 10–16). At the end of the program, PSTs earned an undergraduate and master’s degree. In each year of the program, PSTs enrolled in specialized content courses for teachers in three subjects of choice. PSTs chose one subject as their main subject. The program align with the Nordic teacher education model due to its focus on teaching methods rather advanced CK in the subject. PSTs do not earn a degree in a subject, but they complete a 45 ECTS master thesis in teaching methods for the subject (European Credit Transfer and Accumulation System, 60 ECTS is equivalent to one-year full-time study). Alongside with their specialized content courses, the PSTs completed courses in Pedagogy and Student Knowledge as well as Research and Development in Education. These courses covered general pedagogical knowledge, additional teaching methods, and educational research. Each year included six weeks of mentored field practicum, approximately three weeks of full school days in each of the fall and spring semesters. Some of the PSTs’ lessons would be on topics in which the PSTs had received instruction in the SSCs (aligned lessons), and some which they had not (unaligned lessons). In the aligned lessons, topics aligned with main topics taught in SSCs. This study focus on the contrast between aligned and unaligned lessons.
Participants
Jakob, Pia, and Tina (pseudonyms) were PSTs with an emphasis on science and they agreed to participate in the study. They were assigned to two mentor teachers at the same lower secondary school (grade 8–10), and they were able to teach at least one unaligned and one aligned lesson. Another two PSTs mentored by the same mentor teachers were not able to teach two such contrasting lessons and were omitted from the study. shows the characteristics of Jakob, Pia, and Tina and the unaligned and aligned lessons in this study.
Table 1. Participants and their science lessons.
Practica contexts: mentor teachers
Prior to school practica in the third year of the program, two mentor teachers were recruited for the current study. These were invited because they each had 20+ years of teaching experience, more than five years of experience mentoring PSTs, were enthusiastic about school science, and agreed to facilitate opportunities for the PSTs to teach science during their practicum. The mentor teachers were asked by the first author to organize school practica so that every PST in their group would teach at least two 60-minute science lessons; one aligned lesson and one unaligned lesson. Pia and Tina taught a grade 8 class with 25 students, while Jakob taught a grade 10 class with 21 students. Within the groups, the PSTs cooperated in planning the lessons. The PSTs viewed the school culture as focused on following the textbook in the same pace as parallel classes, rather than providing student-centered and reform-oriented instruction. Pia and Tina were mentored by a male biologist with additional teacher education. He was not involved in the PSTs’ lesson planning. After the PSTs taught lessons, he gave feedback on CK rather than pedagogy. Jakob’s mentor teacher was educated as science teachers and gave specific teaching recommendations for his lesson plans, including the experiments to include.
Methods
This is an exploratory case study (Yin, Citation2014) of three PSTs who completed SSCs prior to their teaching practicum. This qualitative design was appropriate, as one purpose of the study was to identify themes for further investigation of classroom impact from SSCs. The study also has characteristics of a descriptive case study as we describe the impact from SSCs in the real-world context of classroom teaching (Yin, Citation2014). The case focuses on comparing a PST’s lesson that was aligned with the topics in the SSC to a lesson that was not aligned. The cases are bounded by the three PSTs teaching of aligned and unaligned science lessons.
Data collection
Stimulated recall interviews
The main data source was stimulated recall interviews (SRI). SRIs are suitable to reveal both reflection-in-action and reflection-on-action (Meade & McMeniman, Citation1992). Through the use of SRIs, we were able to gain insights into the PSTs’ rationale for their teaching practice (Gess-Newsome, Citation2015; Henderson & Tallman, Citation2006). The first author observed the entire 60-minute lesson and took field notes as a secondary data source. SRIs were conducted within a few hours after the lesson, in one case the following day. Pia and Tina were interviewed after two aligned lessons. In these cases, we selected one of the two, the one with clearest topical alignment with a prior SSC lesson. In the SRIs, the first author displayed segments of video from the lesson to the PST. Based on researcher’s field notes, video segments including the most significant instructional strategies enacted in the lesson were selected. Due to time constraints, not every strategy enacted in the lessons was viewed, and strategies for classroom management and communication with individual students were not viewed. Based on the video, the PSTs were prompted to share thoughts from planning and enactment of the instructional strategy. Follow-up questions from the researcher included, “Why did you do this?” and “From where did you get knowledge of such instruction?” A few general questions concluded the first of the two SRIs, e.g., “How has your knowledge for science teaching changed throughout the teacher education program?” Each interview lasted 45–55 minutes. SRIs were transcribed using QSR International’s NVivo 12 Pro software (Citation2019). The interviews, along with the corresponding audio of the video-recordings of the lesson, were transcribed.
Lesson plans
The PSTs’ lesson plans included descriptions of the instructional strategies planned for the lesson, and the PST’s short rationales for each strategy. Lesson plans were collected to get an overview of the lesson structure and triangulate PSTs’ reflections shared in the SRIs.
Video recordings
The first author recorded the classroom teaching with a video camera and a microphone carried by the PST. The camera overviewed the classroom, facing the PST. Before recording in classrooms, we considered ethical aspects. All recorded persons provided informed consent to participate. The study was conducted with approval from the Norwegian Center for Research Data, project number 54397. The lesson plans and video recordings were secondary data sources and served to triangulate the data.
Analysis
In the following, we explain the steps in analysis of the lesson plans, SRI transcripts, and video recordings. With this analysis, we aimed to answer the first research question describing any differences between aligned and unaligned lessons. First, SRI transcripts were divided into instructional segments. Every instructional segment was defined by enactment of a new instructional strategy, which was also discussed in the SRI. For example, one segment included initiation of a specific whole-class discussion about the cause of the seasons, while a second segment consisted of an explanation of a specific feature of the model of the sun, earth and moon. The segmentation facilitated a focused analysis of significant parts of the PSTs’ pedagogical reasoning. Next, data from lesson plans and video recordings related to a specific instructional segment were added to the instructional segment data. For example, from the lesson plan, an additional rationale for an enacted instructional strategy was added to a specific instructional segment in the SRI transcript.
Second, we classified the enacted strategies as topic-specific, science-specific or general pedagogical strategies using protocol coding (Miles et al., Citation2014). In the protocol coding we used the subcodes topic-specific activities and topic-specific representations from the Magnusson PCK model (Magnusson et al., Citation1999). shows the codes with definitions and examples of coding.
Table 2. Codes for classification of instructional strategies.
Third, we coded PSTs’ rationales for enacting instructional strategies. Rationale is defined as the reasons PSTs gave for enacting an instructional strategy. PSTs usually provided multiple rationales for enacting a single instructional strategy. We used provisional coding (Miles et al., Citation2014) based on a starting list of assumed rationales, to which we added emerging rationales. Rationales for PSTs’ enactment of instructional strategies were grouped in topic-specific PCK rationales, science-specific PCK rationales, and general PK rationales. shows the rationales with definitions and examples. The codebook was revised, and the material was re-coded several times until 100% agreement was reached between the authors.
Table 3. Codes for rationales, the reasons PSTs gave for enacting an instructional strategy.
Fourth, sources of PCK and PCK integrations were analyzed using causation coding (Miles et al., Citation2014). PCK integration refers to teachers simultaneously drawing on multiple components of PCK in planning and enactment of instruction. We focused on integration of knowledge of students’ understanding of science and knowledge of instructional strategies. This is the most central and frequently occurring PCK integration, critical to teacher knowledge development (Akin & Uzuntiryaki-Kondakci, Citation2018; Park & Chen, Citation2012; van Driel et al., Citation2014). In this analysis, we used causation coding (Miles et al., Citation2014), as we extracted not just what happened, but which sources caused the PSTs to enact instructional strategies the way they did. For example, in her aligned lesson Tina engaged students in an activity where they used a flashlight and a white ball in a darkened room to demonstrate moon phases. In the following SRI, she referred to experience with this instructional strategy in a SSC lesson as the source for her use of it. When in doubt about the analysis, we contacted the PST and asked for elaborated responses on the specific issue. Later, we did a member check where participating PSTs commented on interpretations in this manuscript. Overall, the PSTs agreed with the findings.
To answer the second research question about the PSTs’ perceptions of how they drew upon SSCs experiences in their instruction, we analyzed responses to the general question concluding the first SRI and other relevant PST statements. We did a cross-case analysis to identify themes representing how PSTs benefited from SSCs in their classroom teaching. Codes were defined inductively from reading the transcripts (e.g., the participants stated their self-efficacy for science teaching increased through SSCs) and deductively from the PCK components (Magnusson et al., Citation1999). In line with Menon and Sadler (Citation2016), we refer to self-efficacy as a person’s belief that his/her actions will produce desired outcomes, and confidence to execute these actions leading to achievement of the desired goal. Self-efficacy for science teaching was included in the codes as PSTs talked about how SSCs facilitated this, while knowledge of topic-specific instructional strategies was one of the codes from literature, defined as knowledge of instructional strategies developed and/or adopted for a specific science topic. With the cross-case analysis, we identified themes of SSCs’ impact on classroom teaching across the cases.
Results
: In three Norwegian science PSTs’ practica in lower secondary school, what were the differences, if any, between lessons aligned and unaligned with specialized science courses?
We present three assertions across the cases, with reference to data from the three PSTs’ lessons.
Assertion 1: In the aligned lessons, PSTs enacted more science- and topic-specific strategies.
When comparing unaligned and aligned lessons, we found that topic-specific strategies were enacted more often in the aligned lessons, and science-specific strategies were only used in aligned lessons ().
Figure 2. Instructional strategies in percentages of strategies reviewed in SRIs. Number of strategies in parentheses.
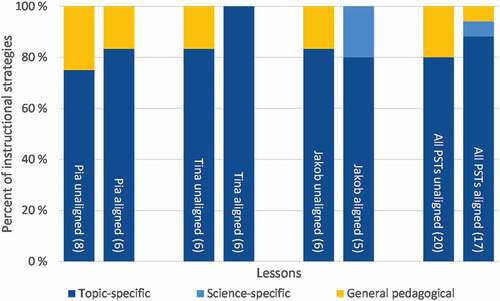
In a majority of the instructional segments, especially in aligned lessons, PSTs drew on their PCK to enact science- or topic-specific strategies. Ninety-four percent (16 of 17) of instructional strategies in aligned lessons were science- or topic-specific strategies, compared to 80% (16 of 20) of strategies in unaligned lessons. Differences among individual PSTs were small. Pia and Tina enacted more topic-specific strategies and Jakob enacted more science-specific strategies in the aligned lessons. In Tina’s aligned lesson on moon phases and seasons, all strategies in the instructional segments were topic-specific. She introduced the lesson with a topic-specific whole class discussion on the causes of seasons on earth. She used a Tellurium, a physical model of the sun, earth and moon, to explain seasons. She also used another topic-specific representation, a video explaining how moon phases occur. Later, students were engaged in a topic-specific activity as they used a flashlight and a white ball in a darkened room to demonstrate moon phases. In Pia’s aligned astronomy lesson, she enacted a topic-specific discussion about why some stars appear to be brighter than others. In his aligned lesson on oil, Jakob had students do an experiment with distillation of crude oil, which is a topic-specific activity. Afterward, he had students write a formal lab report as homework which is a science-specific strategy.
General pedagogical strategies were used slightly more often in unaligned lessons, four times in unaligned lessons compared to once in aligned lessons. One example was Pia assigning students to do textbook tasks such as answering the key questions at the end of the textbook chapter. In Jakob’s unaligned lesson on alcohols, he had students make posters summarizing the flame-color experiment, which is a general pedagogical strategy, suitable across subjects.
Assertion 2: In the aligned lessons, the PSTs used more topic- and science-specific PCK rationales for their instructional decisions.
In their lesson plans and during the SRIs, the PSTs provided rationales for the instructional strategies they enacted in their lessons. In the aligned lessons, the rationales were more often grounded in PCK (). Three groups of rationales emerged: topic-specific PCK rationales, science-specific PCK rationales, and PK rationales. Topic-specific PCK rationales included PSTs enacting instructional strategies to build on students’ prior science knowledge, focusing on key aspects of the topic, or addressing misconceptions. For example, in Tina’s rationale for using a Tellurium in her aligned lesson, she emphasized she wanted to address the misconception of seasons being caused by earth’s distance from the sun. Science-specific PCK rationales included PSTs enacting instructional strategies to use scientific models or to engage students in inquiry. For example, in her aligned lesson, Tina emphasized the importance of students getting to experience the phenomena of moon phases in her rationale for using an activity with flashlights and white balls. PK rationales included PSTs enacting instructional strategies to engage students, add variation to the instruction, make use of technology, or to follow the curriculum or mentor teacher’s advice. An example of a PK rationale was found in Pia’s reasoning for her use of a topic-specific activity in her unaligned lesson where students drew an animal cell and put names on the components. Pia emphasized the usefulness of every student having to think through what they had learned about the animal cell by drawing and labeling, and thereby engaging all the students.
Figure 3. Rationales for enacting instructional strategies in percentages of strategies reviewed in SRIs. Number of rationales in parentheses.
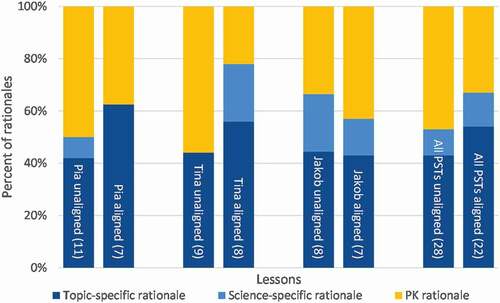
Topic-specific rationales were described in the greatest frequency, and increased from 43% of rationales in unaligned lessons to 54% of rationales in aligned lessons. Within the topic-specific rationales, focusing on key aspects and addressing misconceptions increased the most from unaligned to aligned lessons. As PSTs were more aware of the key aspects and misconceptions in the topics which was taught in SSCs, they designed their aligned lessons to address those key aspects and challenge misconceptions. For example, in her aligned lesson, Tina used a video to address a common misconception that we have a full moon when the moon is on the other side of earth. The PSTs’ focus on misconceptions indicate that their instructional decisions were more firmly grounded in PCK (i.e., knowledge of common misconceptions in a topic and knowledge of topic-specific instructional strategies) in the lessons when the topic was previously taught in a SSC.
Differences were noted among the PSTs; Pia and Tina showed a greater percentage of PCK rationales in their aligned lessons in comparison to their unaligned lessons. For example, Pia emphasized variation as a PK rationale for using a video on metabolism in plants and animals in her unaligned lesson; she stated: “You got things explained in a different way … . variation pretty much.” In her aligned lesson on astronomy, she used another video about distances in space. This time, she emphasized, “It is important to understand distances in space” as a topic-specific PCK rationale for using the specific video.
Jakob’s teaching showed the opposite development. He had a slightly higher use of PK rationales in his aligned lesson. This indicates that lessons aligned with SSC topics made a greater difference for Pia and Tina’s instruction than for Jakob’s. Although, the difference could be attributed to the restrictions set by Jakob’s mentor teacher. In his aligned lesson on oil, he had students distill crude oil. He explained his choice of strategy, “The experiment was scheduled. We were to do a distillation. I managed to fight through this that we did this with real oil.” The primary reason he enacted this topic-specific activity was the curriculum and the mentor teacher’s directive (PK rationale). Although, he modified the investigation by borrowing crude oil from the SSC instructor at the university instead of distilling Coke as described in the curriculum (Topic-specific rationale).
Assertion 3: Instructional strategies in the aligned lessons were more often informed by knowledge of students’ understanding of the topic.
In SRIs and lesson plans, we identified the instructional segments that included integration of knowledge of students’ understanding of science and knowledge of instructional strategies, two central components of PCK (Park & Chen, Citation2012). Such integration occurred more often in aligned lessons, as PSTs’ knowledge of students was more likely to inform their instruction. In contrast, instructional strategies in unaligned lessons were less often informed by PSTs’ knowledge of students’ understanding of the specific topic ().
Figure 4. Knowledge of students informed instruction, in percentages of instructional segments.
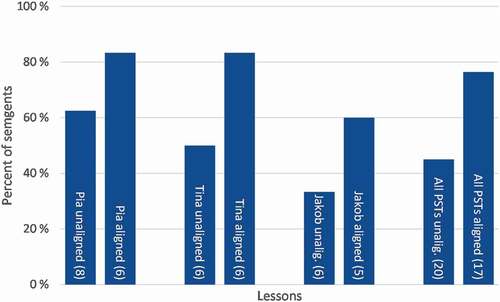
For example, topic-specific activities were more often informed by knowledge of students in aligned compared to unaligned lessons. Both Pia and Tina asked their students to draw representations of the topic but differed in their use of knowledge of students’ understanding of science. In Pia’s unaligned lesson she did not ground the task of drawing an animal cell in her knowledge of students’ understanding of animal cells. In Tina’s aligned lesson, the task of drawing an illustration of seasons on earth was clearly informed by her knowledge of students’ understanding of space. She knew that students’ drawings would have the potential to expose students’ disregard of the earth’s tilt as the explanation of seasons. In aligned lessons, topic-specific representations were also more often informed by knowledge of students’ understanding of the topic. Jakob introduced both his unaligned and aligned lessons with lectures. In the unaligned lesson about alcohols, he lectured on alcohols as a functional group on hydrocarbon chains. This choice was made without referring to his students’ prior knowledge, and the segment did not include integration of knowledge of students’ understanding of science and knowledge of instructional strategies. In contrast, such integration was evident in the first segment in his aligned lesson, where the opening lecture was grounded in students’ prior knowledge of the topic. He designed the lecture to build on students’ prior knowledge of oil, and he addressed a misconception about distillation towers becoming cooler toward the top.
For Pia and Tina, we also see the contrasting integration at the lesson level. During planning of their unaligned lessons about animal cells and oxygenation, they drew on prior knowledge from high school biology, practitioner literature, and the student textbook. Although this led to reasonable structuring of the lessons, the PSTs had limited support for planning student-centered instruction. In contrast, Pia’s and Tina’s aligned lessons on astronomy were based on their knowledge of students’ understanding of the topic. Their decisions were grounded in knowledge of what aspects of the topic were central, though difficult to understand for students, and common misconceptions held by students. Much of this knowledge was drawn from SSC lectures and course readings. We did not see a contrast on the lesson level of Jakob’s lessons. Both his unaligned and aligned lessons were built around laboratory experiments scheduled by the mentor teacher.
: What were these PSTs’ perceptions of how they drew upon specialized science courses?
The PSTs highlighted SSCs as an important influence in developing their knowledge and skills for teaching science. First, this was visible in PSTs’ references to sources when reflecting on instructional segments in the SRIs. Fifteen of a total of 26 references to instructional strategies, knowledge of students, and integrations of these PCK components were related back to the PSTs’ SSC experiences. Topic-specific instructional strategies, in particular, were drawn from SSCs. In the SRIs, there were ten occurrences where PSTs referred to SSCs as a source of topic-specific instructional strategies or integrations of such strategies with knowledge of students. The other sources were mentioned only two or three times each: student textbook, mentor teacher, peer PSTs, university courses other than SSCs, and personal learning experiences. Second, when looking at PSTs’ responses to the general SRI questions related to the development of their science teaching knowledge, we found that SSCs were valued as sources of relevant CK, PCK, and self-efficacy in teaching science. Within PCK, the components most frequently discussed were knowledge of students’ understanding of science, topic-specific instructional strategies, and science-specific instructional strategies. In the following sections, we give more detail on each of these aspects.
Relevant CK
The PSTs drew on CK from SSCs in their aligned lessons. For example, Tina learned about moon phases in a SSC astronomy lesson two years earlier, when she took part in a demonstration of the phenomena. In Tina’s perception, CK learning through engagement in practical strategies enhanced her science CK. Pia also discussed ways that SSCs increased her learning of science CK. She expressed that the most useful lessons in SSCs were limited to the content level of the students they were learning to teach (age 10–16), and not focused on “knowing very much advanced physics and chemistry which I think we have focused a lot on but which I, at least until now, have had no use for.” Through the first three years in the teacher education program, she had not seen the value of learning CK beyond what her future students were to learn. For example, the topic of stars’ lives was taught in a first year SSC. When Pia taught this topic in her aligned lesson, she viewed the CK and PCK from this SSC lesson as less useful, since it was taught with a fairly high level of detail. She made more use of the students’ textbook and online videos in planning the lesson. Pia thought she benefited little from advanced CK in SSCs. Pia provided an example of the kind of instruction she benefited from: “Like ‘let’s learn about transitions between states of matter.’ And we had the same illustration as they [school kids] have in their textbook and we learned how we can work with it in a practical way.” In Pia’s opinion, the useful components of the SSCs were those directly applicable to the science content level of the classrooms she was preparing to teach.
Knowledge of students’ understanding of science
For Pia and Tina, knowledge of lower secondary students gained from SSCs played a critical role in the design of their aligned lessons on astronomy. A SSC instructor had highlighted a book chapter that stated astronomy is a topic that is often interesting for both genders. This knowledge, accompanied with knowledge of common misconceptions about the universe, guided their lesson design. Pia read about student misconceptions on why we have seasons in SSC literature, which greatly informed her lesson design. She stated, “Many science textbooks state that 15-year-olds do not understand why we have seasons. They believe it is about us being closer to or further from the sun, and not that it is the angle.” Tina had also participated in an SSC activity challenging her own misconception of the cause of seasons. She stated, “That is a typical misconception. I could agree with that [misconception] too if it was not illustrated to me.” This shows that a SSC focus on student misconceptions also improved the PSTs’ own CK. Tina clearly stated what difference SSCs made to her knowledge for teaching when reflecting upon her unaligned lesson on animal cells, “Some topics are well presented to us and some are not. We have talked about student misconceptions in almost everything we went through [in SSCs]. In this [animal cells], we have not received instruction, and therefore nothing about misconceptions.” Here, she emphasized the value of discussing common student misconceptions in SSCs as a major component that made a difference to her professional development.
Knowledge of topic-specific instructional strategies
Several of the instructional strategies enacted in PSTs’ aligned lessons were drawn from SSCs. For example, Jakob learned how to distill crude oil from his SSC instructor. This included knowledge about safety issues related to the specific experiment: “The only risk here was getting hot oil over oneself. The temperature would be too high in the first distillation, but the second could be a little bit high.” Pia and Tina’s aligned lessons were largely built on knowledge of topic-specific strategies from SSCs. Pia used her notes from SSC lessons about stars as a rationale for emphasizing the fusion of hydrogen to become helium in her instruction on the birth of stars. For Tina, two of the main strategies in her lesson on moon phases and seasons were from a SSC astronomy lesson. She used a moon phase demonstration that was shown in a SSC. She also used a Tellurium, a model of the sun, moon, and earth, about which she stated:
It’s an extremely funny thing. I was so fascinated when we had that in teacher education. You get it so clear and visual without having to show a video where you afterwards must explain what happened. Because films often require a recap or summary. We can talk while looking at this.
The PSTs readily made use of the instructional strategies for specific topics that they experienced in SSC lessons.
Knowledge of science-specific instructional strategies
In both aligned and unaligned lessons, the PSTs benefited from knowledge of instructional strategies from SSCs. Such knowledge was transferred across science topics. PSTs benefited from use of models, practical work, and inquiry implemented as a way of learning in SSCs, and not just presented as a toolbox for future use in classrooms. Jakob stated that practical work in SSCs made the greatest impact on his knowledge for teaching science. He stated:
I have understood a lot more about how to perform practical work, which kinds of practical work there are, and the importance of doing and focusing on the work afterwards. That the final part is where students learn. Earlier, I did not know the importance of that part. And through the program we have actually seen various kinds of practical work.
As shown in this example, SSCs facilitated PSTs in selecting instructional strategies suitable across science topics.
Self-efficacy for science teaching
PSTs perceived that the SSCs gave them increased self-efficacy for teaching science which was related to learning CK and PCK. Conversely, the participants described a lack of confidence in teaching topics not covered by prior SSCs, particularly if they had no experience with the topic since their own compulsory school. Despite spending hours researching and learning about hydrocarbons and alcohols before teaching his unaligned lesson, Jakob stated “I do not feel that I am much ahead of students in this topic [alcohols] … I miss the certainty. … Since I am uncertain, I am influenced toward following the textbook.” Jakob’s lack of CK restricted his ability to deliver a pedagogically sound lesson about alcohols. In the SRI reflecting on her unaligned lesson on animal cells, Pia stated: “I feel seen through because I am very conscious that I am not confident in this. I never had biology myself. Last time I learned this was when I had their [students’] textbook myself at school.” On the positive side, experience with laboratory experiments from SSCs increased Jakob’s self-efficacy in carrying out experiments in his aligned and unaligned lessons. He stated: “We did quite a lot of laboratory stuff in teacher education. So, one become more confident at the lab.” Self-efficacy was partly transferred to new topics, but also gained as knowledge and experience accumulated throughout the teacher education experience. Despite uncertainty on the topic in his unaligned lesson, Jakob stated, “I have got more self-confidence in teaching. If this was the first year, I would have been more nervous.” Jakob felt that the combination of SSCs and school practica helped him develop his self-efficacy as a science teacher.
Lastly, PSTs’ self-efficacy was evident during observations of the lessons. Jakob enacted experiments in both lessons, as suggested by his mentor teacher. In the unaligned lesson, he had students burn alcohols and observe differences in flame color, although he thought the experiment was of little relevance to the topic at hand. In his aligned lesson, he had enough confidence to replace the suggested distilling of Coke with the more relevant distilling of crude oil. In Tina’s aligned lesson, she got a question about whether the South Pole gets midnight sun or not. She did not know the answer, but instead of turning to her peer PSTs and mentor teacher for answers (as she had in the unaligned lesson), she carried out a quick inquiry using the Tellurium. She did not arrive at a final answer to the question but, based on observations, she appeared more confident in exploring the question in comparison to a student question she had in her unaligned lesson. This indicates that SSCs may contribute to PSTs’ self-efficacy for exploring questions rather than quickly giving a correct answer.
Discussion
In this study, we look at the effects of SSCs up to two years later as three PSTs plan and teach lessons in their practica. Through the comparison of aligned and unaligned lessons, we show how SSCs had an impact on the PSTs’ science teaching practice and perceptions of self-efficacy. This study contributes to the literature as earlier SSC-related studies were limited to showing CK and self-efficacy gains based on tests and interviews conducted at the end of a SSC. One exception is Etkina (Citation2010), who followed PSTs into classrooms after they completed SSCs, and reported an increased ability to notice students’ understandings and engage them in active learning of physics. However, Etkina (Citation2010) did not compare the teachers’ SSC-aligned lessons with unaligned lessons, as we did in the current study. From our comparisons, we add to her findings of the impact from SSCs; PSTs enacted more instructional strategies that were informed by knowledge of students’ understandings in lessons aligned with SSC lessons. Further, we extend her work by adding nuance to research on classroom impact from SSCs. Through video recordings, lesson plans, and SRIs with PSTs, we identified they used more instructional strategies and rationales based on science- and topic-specific PCK in lessons aligned with SSCs compared to the unaligned lessons. Lastly, our study contributes PSTs’ perceptions on how they drew on SSCs in their teaching practice. In summary, our study addresses a knowledge gap about whether and how PSTs use what they learn in teacher education in classrooms (Cochran-Smith & Villegas, Citation2016; Jenset, Citation2018).
The study also responds to the call for research on how science PCK develops and transforms in classroom practice (Alonzo et al., Citation2012). From our study, we identified the transformation of collective PCK from SSCs, via PSTs development of their own personal PCK, to enactment in classrooms. This transformation represents the potential of SSCs supporting PSTs in connecting theory and practice—a major challenge in teacher development (Grossman et al., Citation2009; Thompson et al., Citation2013). The clearest connections were from SSC lessons where course instructors went into depth discussing PCK for teaching specific topics for grade 5–10 classrooms, including a focus on students’ understanding of the topic and modeling of student-centered instructional strategies. In SSCs, PSTs became aware of common misconceptions and strategies to challenge these misconceptions. Consequently, some of the aligned school practicum lessons were designed to address specific students’ misconceptions. Pia and Tina’s reasoning for their lessons on space are clear examples of the impact SSCs can have on teaching and learning. In a SSC, Pia and Tina learned about common misconceptions related to space. They used this information as they designed and taught their lessons. Such responsive teaching is known to be challenging for PSTs (Sun & van Es, Citation2015). The contrast between unaligned and aligned lessons indicates that building on students’ prior knowledge was influenced by SSC experiences. The identified differences in mentor teacher advice seemed to be of secondary importance for the PSTs’ practice. Our findings also add to PCK integration literature as we show that SSCs can support PSTs in adapting instruction based on student ideas. While all three PSTs used more reform-oriented teaching practices in their aligned lessons, SSCs made a greater difference in Pia and Tina’s teaching. This could be explained by Jakob’s mentor teacher’s recommendations for both his unaligned and aligned lesson. In stimulated recall interviews, Jakob explained how he would have designed the lessons from his own personal PCK if not constrained by the mentor teacher.
Beyond describing their rationale for their instruction, PSTs shared perceptions on how their instruction was supported by SSCs. All three of them stated that SSCs made an impact on their teaching. PSTs mentioned learning CK in SSCs as useful, but they emphasized their PCK learning. They highlighted opportunities to learn PCK from SSC instructors teaching science content at the level of the students they planned to teach (grade 5–10). This finding responds to calls that teacher education should be oriented toward classroom practice (Cochran-Smith & Villegas, Citation2016; Darling-Hammond et al., Citation2017; Menon & Sadler, Citation2016).
Our findings indicate that developing teachers’ self-efficacy for teaching science is possible through SSCs. The PSTs reported and demonstrated a higher self-efficacy in science teaching as a result of SSC experiences. Jakob, while constrained in his enactment of PCK by specific recommendations from his mentor teacher, was the PST with the strongest statements on SSCs strengthening his self-efficacy. He highlighted inquiry-based teaching in SSCs as beneficial to his self-efficacy for teaching science. His competency and self-efficacy for leading lab sessions was transferred across topics. Menon and Sadler (Citation2016) highlighted the importance of gaining self-efficacy early in teacher education programs and also found PST’s increased self-efficacy at the end of a physics SSC. In the current study, we added to this knowledge as we explored how alignment between SSCs and school practica helped PSTs increase their self-efficacy for science teaching.
Limitations
Findings from the current study cannot be generalized, as it is a case study with a limited number of three participants. We originally planned to have a larger number of participants, but in the end, only three participants taught both an aligned and unaligned lesson. Although the data was rich and from multiple sources, the study is also limited to the comparison of one aligned and one unaligned lesson taught in the practicum. Also, the two mentor teachers differed in their input on the lesson plans and their feedback on the PSTs’ teaching, as described in the context section.
Conclusion
The current study adds to the scant research base on the impact of SSCs on classroom teaching as we have found these courses contribute to the development of PSTs’ knowledge and practices for science teaching. In the SSCs, the PSTs benefited from learning CK while engaging in reform-oriented strategies. Based on what we observed in classrooms, PSTs’ reflections from SRIs, and their own perceptions, we conclude that SSCs can support more integrated PCK found to support students in learning (Coetzee et al., Citation2020; Kirschner et al., Citation2015). The study shows the process of connecting theory and practice as the three participating PSTs’ transformed collective PCK from SSCs to their own personal PCK and enacted PCK in their practica. We show some ways SSCs support PSTs not only in learning about science and science teaching, but also enacting teaching practices. This is a needed competency (Grossman et al., Citation2009; Thompson et al., Citation2013), and Nordic teacher education programs have the potential to better support this linking of theory and practice with their use of SSCs (Rasmussen & Dorf, Citation2010).
Implications for research and practice
The current exploratory case study has the potential to stimulate research interest in SSCs for teachers. More studies are needed using larger numbers of PSTs, a greater number of observed lessons, and a wider variety of classroom contexts and mentor teachers. These studies should aim to see if PCK is transferred from aligned topics to new topics. Furthermore, the specific designs of different SSCs should be studied to understand their impact. In this study, we found that SSC lessons aligned with the target grade level were valued more highly by the PSTs. Finally, longitudinal studies tracing experiences from SSCs into the beginning years of teaching are needed. We are aware of the additional resources required to develop and teach SSCs. However, SSCs appear to be a good investment in teacher education as this exploratory study shows that SSCs make a difference in supporting PSTs learning to teach.
Disclosure statement
No potential conflict of interest was reported by the authors.
References
- Abell, S. K. (2008). Twenty years later: Does pedagogical content knowledge remain a useful idea? International Journal of Science Education, 30(10), 1405–1416. https://doi.org/https://doi.org/10.1080/09500690802187041
- Akin, F. N., & Uzuntiryaki-Kondakci, E. (2018). The nature of the interplay among components of pedagogical content knowledge in reaction rate and chemical equilibrium topics of novice and experienced chemistry teachers. Chemistry Education Research and Practice, 19(1), 80–105. https://doi.org/https://doi.org/10.1039/C7RP00165G
- Allsopp, D. H., DeMarie, D., Alvarez-McHatton, P., & Doone, E. (2006). Bridging the gap between theory and practice: Connecting courses with field experiences. Teacher Education Quarterly, 33(1), 19–35. http://www.jstor.org/stable/23478732
- Alonzo, A. C., Kobarg, M., & Seidel, T. (2012). Pedagogical content knowledge as reflected in teacher–student interactions: Analysis of two video cases. Journal of Research in Science Teaching, 49(10), 1211–1239. https://doi.org/https://doi.org/10.1002/tea.21055
- Anderson, R. D. A., Beverly, L., Varanka-Martin, M. A., Romagnano, L., Bielenberg, J., Flory, M., Mieras, B., & Whitworth, J. (1994). Issues of curriculum reform in science, mathematics, and higher order thinking across the disciplines. U.S. Government Printing Office.
- Avard, M. (2009). Student-centered learning in an earth science, preservice, teacher-education course. Journal of College Science Teaching, 38(6), 24–29. https://www-proquest-com.mime.uit.no/scholarly-journals/student-centered-learning-earth-science/docview/200340237/se-2?accountid=17260
- Bell, R. L., & Trundle, K. C. (2008). The use of a computer simulation to promote scientific conceptions of moon phases. Journal of Research in Science Teaching, 45(3), 346–372. https://doi.org/https://doi.org/10.1002/tea.20227
- Bergman, D. J., & Morphew, J. (2015). Research and teaching: Effects of a science content course on elementary preservice teachers‘ self-efficacy of teaching science. Journal of College Science Teaching, 44(3), 73–81. https://doi.org/https://doi.org/10.2505/4/jcst15_044_03_73
- Blue Ribbon Panel. (2010). Transforming teacher education through clinical practice: A national strategy to prepare effective teachers. National Council for Accreditation of Teacher Education. http://caepnet.org/~/media/Files/caep/accreditation-resources/blue-ribbon-panel.pdf
- Carlson, J., Daehler, K. R., Alonzo, A. C., Barendsen, E., Berry, A., Borowski, A., Carpendale, J., Chan, K. K. H., Cooper, R., Friedrichsen, P., Gess-Newsome, J., Henze-Rietveld, I., Hume, A., Kirschner, S., Liepertz, S., Loughran, J., Mavhunga, E., Neumann, K., Nilsson, P., Park, S., Rollnick, M., Sickel, A., Schneider, R. M., Suh, J. K., van Driel, J., & Wilson, C. D. (2020). The refined consensus model of pedagogical content knowledge in science education. In HumeA., CooperR., & BorowskiA. (Eds.), Repositioning pedagogical content knowledge in teachers’ knowledge for teaching science (pp. 77–94). Springer Nature. https://doi.org/https://doi.org/10.1007/978-981-13-5898-2_15
- Chan, K. K. H., & Hume, A. (2019). Towards a consensus model: Literature review of how science teachers’ pedagogical content knowledge is investigated in empirical studies. In A. Hume, R. Cooper, & A. Borowski (Eds.), Repositioning pedagogical content knowledge in teachers’ knowledge for teaching science (pp. 3–76). Springer Nature. https://doi.org/https://doi.org/10.1007/978-981-13-5898-2_1
- Cochran-Smith, M., & Villegas, A. M. (2016). Research on teacher preparation: Charting the landscape of a sprawling field. In D. H. Gitomer & C. A. Bell (Eds.), Handbook of research on teaching (5th ed., pp. 439–547). American Educational Research Association.
- Coetzee, C., Rollnick, M., & Gaigher, E. (2020). Teaching electromagnetism for the first time: A case study of pre-service science teachers’ enacted pedagogical content knowledge. Research in Science Education. https://doi.org/https://doi.org/10.1007/s11165-020-09948-4
- Crawford, B. A. (2007). Learning to teach science as inquiry in the rough and tumble of practice. Journal of Research in Science Teaching, 44(4), 613–642. https://doi.org/https://doi.org/10.1002/tea.20157
- Darling-Hammond, L., Burns, D., Campbell, C., Goodwin, A. L., Hammerness, K., Low, E. L., McIntyre, A., Sato, M., & Zeichner, K. (2017). Empowered educators: How high-performing systems shape teaching quality around the world. Jossey-Bass.
- Davis, E. A., & Krajcik, J. S. (2005). Designing educative curriculum materials to promote teacher learning. Educational Researcher, 34(3), 3–14. https://doi.org/https://doi.org/10.3102/0013189X034003003
- Doster, E. C., Jackson, D. F., & Smith, D. W. (1997). Modeling pedagogical content knowledge in physical science for prospective middle school teachers: Problems and possibilities. Teacher Education Quarterly, 24(4), 51–65. http://www.jstor.org/stable/23478121
- Enochs, L. G., & Riggs, I. M. (1990). Further development of an elementary science teaching efficacy belief instrument: A preservice elementary scale. School Science and Mathematics, 90(8), 694–706. https://doi.org/https://doi.org/10.1111/j.1949-8594.1990.tb12048.x
- Etkina, E. (2010). Pedagogical content knowledge and preparation of high school physics teachers. Physical Review Physics Education Research, 6(2), 1–26. https://doi.org/https://doi.org/10.1103/PhysRevSTPER.6.020110
- Etkina, E. (2015). Using early teaching experiences and a professional community to prepare pre-service teachers for every-day classroom challenges, to create habits of student-centered instruction and to prevent attrition. In C. Sandifer & E. Brewe (Eds.), Recruiting and educating future physics teachers: Case studies and effective practices (pp. 249–266). American Physical Society.
- Fones, S. W., Wagner, J. R., & Caldwell, E. R. (1999). Promoting attitude adjustments in science for preservice elementary teachers. Journal of College Science Teaching, 28(4), 231–236. https://www-proquest-com.mime.uit.no/scholarly-journals/promoting-attitude-adjustments-science-preservice/docview/200272506/se-2?accountid=17260
- Friedrichsen, P., Abell, S. K., Pareja, E. M., Brown, P. L., Lankford, D. M., & Volkmann, M. J. (2009). Does teaching experience matter? Examining biology teachers' prior knowledge for teaching in an alternative certification program. Journal of Research in Science Teaching, 46(4), 357–383. https://doi.org/https://doi.org/10.1002/tea.20283
- Gess-Newsome, J. (2015). A model of teacher professional growth and skill including PCK: Results of the thinking from the PCK Summit. In A. Berry, P. Friedrichsen, & J. Loughran (Eds.), Re-examining pedagogical content knowledge in science education (pp. 28–42). Routledge.
- Grossman, P., Hammerness, K., & McDonald, M. (2009). Redefining teaching, re-imagining teacher education. Teachers and Teaching, 15(2), 273–289. https://doi.org/https://doi.org/10.1080/13540600902875340
- Henderson, L., & Tallman, J. (2006). Stimulated recall and mental models, tools for teaching and learning computer information literacy. The Scarecrow Press.
- Horn, I. S., & Campbell, S. S. (2015). Developing pedagogical judgment in novice teachers: Mediated field experience as a pedagogy for teacher education. Pedagogies: An International Journal, 10(2), 149–176. https://doi.org/https://doi.org/10.1080/1554480X.2015.1021350
- Jenset, I. S. (2018). Researching practice-based teacher education: Trends, challenges and recommendations for future research. Acta Didactica Norge, 12(3), 3. https://doi.org/https://doi.org/10.5617/adno.5933
- Kirschner, S., Taylor, J., Rollnick, M., Borowski, A., & Mavhunga, E. (2015). Gathering evidence for the validity of PCK measures: Connecting ideas to analytic approaches. In A. Berry, P. Friedrichsen, & J. Loughran (Eds.), Re-examining pedagogical content knowledge in science education (pp. 229–241). Routledge.
- Knaggs, C. M., & Sondergeld, T. A. (2015). Science as a learner and as a teacher: Measuring science self-efficacy of elementary preservice teachers. School Science and Mathematics, 115(3), 117–128. https://doi.org/https://doi.org/10.1111/ssm.12110
- Magnusson, S., Krajcik, J., & Borko, H. (1999). Nature, sources, and development of pedagogical content knowledge for science teaching. In J. Gess-Newsome & N. G. Lederman (Eds.), Examining pedagogical content knowledge (pp. 95–132). Springer. https://doi.org/https://doi.org/10.1007/0-306-47217-1_4
- Mavhunga, E. (2020). Revealing the structural complexity of component interactions of topic-specific PCK when planning to teach. Research in Science Education, 50(3), 965–986. https://doi.org/https://doi.org/10.1007/s11165-018-9719-6
- McDonald, M., Kazemi, E., & Kavanagh, S. S. (2013). Core practices and pedagogies of teacher education: A call for a common language and collective activity. Journal of Teacher Education, 64(5), 378–386. https://doi.org/https://doi.org/10.1177/0022487113493807
- McLoughlin, A. S., & Dana, T. M. (1999). Making science relevant: The experiences of prospective elementary school teachers in an innovative science content course. Journal of Science Teacher Education, 10(2), 69–91. https://doi.org/https://doi.org/10.1023/A:1009410130218
- Meade, P., & McMeniman, M. (1992). Stimulated recall — An effective methodology for examining successful teaching in science. The Australian Educational Researcher, 19(3), 1–18. https://doi.org/https://doi.org/10.1007/BF03219515
- Menon, D., & Sadler, T. D. (2016). Preservice elementary teachers’ science self-efficacy beliefs and science content knowledge. Journal of Science Teacher Education, 27(6), 649–673. https://doi.org/https://doi.org/10.1007/s10972-016-9479-y
- Miles, M. B., Huberman, A. M., & Saldaña, J. (2014). Qualitative data analysis: A methods sourcebook (3rd ed.). Sage.
- Park, S., & Chen, Y. C. (2012). Mapping out the integration of the components of pedagogical content knowledge (PCK): Examples from high school biology classrooms. Journal of Research in Science Teaching, 49(7), 922–941. https://doi.org/https://doi.org/10.1002/tea.21022
- Posnanski, T. J. (2007). A redesigned geocience content course’s impact on science teaching self-efficacy beliefs. Journal of Geoscience Education, 55(2), 152–157. https://doi.org/https://doi.org/10.5408/1089-9995-55.2.152
- QSR International. (2019). NVivo 12 pro (Version 12.6.0.959) [Computer software].
- Rasmussen, J., & Dorf, H. (2010). Challenges to Nordic teacher education programmes. In B. Hudson, P. Zgaga, & B. Åstrand (Eds.), Advancing quality cultures for teacher education in Europe: Tensions and opportunities (pp. 51–67). Umeå School of Education, Umeå University.
- Sawada, D., Piburn, M. D., Judson, E., Turley, J., Falconer, K., Benford, R., & Bloom, I. (2002). Measuring reform practices in science and mathematics classrooms: The reformed teaching observation protocol. School Science and Mathematics, 102(6), 245–253. https://doi.org/https://doi.org/10.1111/j.1949-8594.2002.tb17883.x
- Shulman, L. S. (1986). Those who understand: Knowledge growth in teaching. Educational Researcher, 15(2), 4–14. https://doi.org/https://doi.org/10.3102/0013189X015002004
- Stroupe, D., & Gotwals, A. W. (2018). “It’s 1000 degrees in here when I teach”: Providing preservice teachers with an extended opportunity to approximate ambitious instruction. Journal of Teacher Education, 69(3), 294–306. https://doi.org/https://doi.org/10.1177/0022487117709742
- Sun, J., & van Es, E. A. (2015). An exploratory study of the influence that analyzing teaching has on preservice teachers’ classroom practice. Journal of Teacher Education, 66(3), 201–214. https://doi.org/https://doi.org/10.1177/0022487115574103
- Thompson, J., Windschitl, M., & Braaten, M. (2013). Developing a theory of ambitious early-career teacher practice. American Educational Research Journal, 50(3), 574–615. https://doi.org/https://doi.org/10.3102/0002831213476334
- van Driel, J. H., Berry, A., & Meirink, J. (2014). Research on science teacher knowledge. In N. G. Lederman & S. K. Abell (Eds.), Handbook of research on science education (Vol. 2, pp. 848–870). Routledge. https://doi.org/https://doi.org/10.4324/9780203097267.ch16
- Varelas, M., Plotnick, R., Wink, D., Fan, Q., & Harris, Y. (2008). Inquiry and connections in integrated science content courses for elementary education majors. Journal of College Science Teaching, 37(5), 40–47. https://www-proquest-com.mime.uit.no/scholarly-journals/inquiry-connections-integrated-science-content/docview/200387423/se-2?accountid=17260
- Wilson, S. M., Floden, R. E., & Ferrini-Mundy, J. (2001). Teacher preparation research: Current knowledge, gaps, and recommendations (No. Document R-01–3). Center for the Study of Teaching and Policy.
- Yin, R. K. (2014). Case study research: Design and methods (5th ed.). SAGE.
- Zeichner, K. (2010). Rethinking the connections between campus courses and field experiences in college- and university-based teacher education. Journal of Teacher Education, 61(1–2), 89–99. https://doi.org/https://doi.org/10.1177/0022487109347671