ABSTRACT
A novel two-stage wet electrostatic precipitator (ESP) has been developed using a carbon brush pre-charger and collection plates with a thin water film. The electrical and particle collection performance was evaluated for submicrometer particles smaller than 0.01∼0.5 μm in diameter by varying the voltages applied to the pre-charger and collection plates as well as the polarity of the voltage. The collection efficiency was compared with that calculated by the theoretical models. The long-term performances of the ESP with and without water films were also compared in tests using Japanese Industrial Standards dust. The experimental results show that the carbon brush pre-charger of the two-stage wet ESP had approximately 10% particle capture, while producing ozone concentrations of less than 30 ppb. The produced amounts of ozone are significantly lower than the current limits set by international agencies. The ESP also achieved a high collection rate performance, averaging 90% for ultrafine particles, as based on the particle number concentration at an average velocity of 1 m/sec corresponding to a residence time of 0.17 sec. Higher particle collection efficiency for the ESP can be achieved by increasing the voltages applied to the pre-charger and the collection plates. The decreased collection efficiency that occurred during dust loading without water films was completely avoided by forming a thin water film on the collection plates at a water flow rate of 6.5 L/min/mCitation2.
Current two-stage electrostatic precipitators (ESPs) have several technical problems such as a drop in collection efficiencies by small-particle re-entrainment during rapping and corrosion of metallic electrodes of the ESPs by corrosive gases. This paper evaluates a novel two-stage ESP that uses a nonmetallic pre-charger and water film collection plates to avoid the above mentioned problems of other ESPs. This ESP can be used not only for industrial applications but also for residential purposes because it has a high removal performance for fine particles with low ozone generation and maintains its efficiency due to the continuous cleaning of the collection plates with water film.
INTRODUCTION
Two-stage electrostatic precipitators (ESPs) have been widely applied to remove fine particles from industrial pollutants and also to enhance indoor air quality.Citation1–Citation3 This type of ESP differs from single-stage ESPs, which use high voltage to charge particles and subsequently collect them within the same chamber on surfaces of opposite charge. Two-stage ESPs consist of a charging stage, utilizing thin wires equally spaced from the parallel or cylindrical grounded plates or tubes, and a collection stage involving separate parallel metal plates that collect negatively (positively) charged particles at positively (negatively) charged plates.Citation4,Citation5 Yoo et al.,1 Li and Christofides,4 and Talaie et al.Citation6 conducted theoretical and experimental research on particle collection performance of general two-stage ESPs with a wire-plate-type pre-charger and parallel-plate-type collection cell.
Jayaram et al.Citation7 and Zukeran et al.Citation8 applied a cooled pre-charger before using an ESP and enhanced the collection efficiency of the standalone ESP via stabilization of the corona discharge by water cooling and pulse energization. Zukeran et al.2 examined the re-entrainment phenomenon under diesel flue gas using a two-stage ESP. They noted that, even with the two-stage ESP, the collection efficiency decreased due to re-entrainment of particles with low resistivity. Zhu et al.Citation9 developed an ESP with a bipolar pre-charger and achieved a collection efficiency exceeding 95% for micrometer and submicrometer particles, attributable to particle agglomeration by the bipolar pre-charger. However, previous studies for two-stage ESPs used metallic wires or spikes as high-voltage electrodes. These wires or spikes could exhibit corrosion problems when treating particles in corrosive and acidic gaseous materials such as SO3, HF, and HCl. In addition, the polarity of the voltage applied to the pre-charger in two-stage ESPs is generally positive; however, a pre-charger with negative polarity could produce high ozone concentrations at the voltage levels necessary to increase the collection efficiency of very small particles.Citation5
The ozone that is normally generated as a by-product of corona discharge in an ESP can damage respiratory organs and degrade many materials. Consequently, the daily 8-hr maximum ozone concentration is set to less than 60∼80 ppb by environmental regulatory agencies such as the World Health Organization, U.S. Environmental Protection Agency, and European Commission.Citation10–Citation13 The ozone production by particle charging in the metallic corona pre-charger of general ESPs depends on a number of factors, including flow rate, relative humidity, materials, and the diameter or thickness of high-voltage electrode as well as the operational voltage and current, and the polarity of the corona discharge.Citation11,Citation14–Citation16 In particular, the thickness of the corona discharge electrode at the same discharge current results in reduction of ozone generation from the charger because ionization and dissociation areas of oxygen decreases with decreasing the thickness of discharge electrode in the pre-charger.Citation17
Wet ESPs are used for industrial applications in cases of high potential for explosion or very sticky, corrosive, or highly resistive dust. A water flow is applied continuously or intermittently to wash particles from the collection electrodes into a reservoir used to collect liquid. Wet ESPs have the advantage of avoiding problems with rapping re-entrainment and back corona. These features make wet ESPs more effective for controlling ultrafine particlesCitation18–Citation20 and efficiently removing corrosive liquid phase particulates such as SO3, polyaromatic hydrocarbon (PAH), and HCl compared to dry ESPs.Citation21–Citation25 However, wet ESPs might experience disruptions caused by spraying (misting) of water, the formation of dry spots due to effects of water surface tension (channeling), and corrosion of the collector surfaces. These factors limit the applicability of current wet precipitators.Citation26
Bayless et al.26 and Pasic et al.Citation27 developed a novel hydrophilic membrane-based wet ESP to minimize dry spot formation on collection plates, which could be attacked by corrosive gases, and achieved more than 95% removal efficiency based on particle mass. For the same purpose, Lin et al.Citation28 developed a novel wet ESP with a thin water film on its collection plates to control fine and nanosized particles and achieved over 95% collection efficiency for corn oil mists at a residence time of 0.39 sec. However, these wet ESPs also used metallic spikes and wires as the high-voltage electrodes of the positive charger.
Carbon fiber ionizers are made of graphite, an anticorrosive material that can be utilized easily and inexpensively by applying a voltage of a few kilovolts to a bundle of approximately 300 carbon fibers, a few micrometers in diameter, to generate ions.Citation29 These ionizers can produce stable unipolar ions at sufficiently high concentrations, while generating negligible amounts of ozone due to the fine diameters of carbon fibers. As a result, they have been used in indoor air purifiers to generate ions as an alternative to corona discharge from thin metallic wires and spikes.Citation30–Citation34
We are unaware of any previous attempts to combine a carbon brush charger with wet collection plates cleaned by a thin water film for a two-stage wet ESP for an industrial purpose. In this study, the electrical and particle collection performance characteristics of the newly developed two-stage wet ESP were investigated by changing the applied voltage and its polarity. The particle collection result was compared with theoretical results obtained from theoretical models for ESPs. The concentration of ozone produced as a by-product of ESP usage was also measured by changing the voltage and its polarity applied to the pre-charger. Dust loading tests with Japanese Industrial Standards (JIS) dust was also performed to assess the long-term performance of the ESP with and without a water film.
EXPERIMENTAL SETUP
shows a schematic diagram of the two-stage wet ESP consisting of the carbon brush charger and collection cell that were developed in this study. As shown in , the carbon brush pre-charger was composed of a bundle of approximately 300 carbon fibers with a diameter of a few micrometersCitation31–Citation34; the bundle of brushes was 6 mm long. The brush was connected to a stainless steel rod, which was in turn connected to a high-voltage power supply. The high-voltage electrode was inserted into the center of a 1-inch stainless steel tube (S/S tube), and the tip of the carbon brushes was located 104 mm from the left side of the tube. To prevent particle deposition on the carbon brushes, the high-voltage electrode was insulated by a cylindrical Teflon housing and shielded from a 10 L/min airflow streaming into the 1 mm slit between the housing and the electrode. As shown in , the wet-type collection cell consisted of upper and lower water reservoirs, which both connected to a water circulation pump (model PW-S3545MA; WILO SE, Dortmund, Germany), water film collection plates, and a plate-type high-voltage electrode. The upper water reservoir (54 × 160 × 13 mm) was attached to the back side of the collection plate, and the make-up water from the circulation pump was supplied at the bottom of the reservoir. The water from the reservoir flowed through 2-mm-diameter holes (separated by a distance of 5 mm) in the collection plates. To enhance the hydrophilicity of the collection plates, which increases the water film uniformity and minimizes water consumption, the collection surfaces of the plates were first sand-blasted and then coated with an alcohol solution (model P&T-100HX; Nanopac Co., Ltd., Yong-In, Korea) in which approximately 5∼10 nm TiO2 nanoparticles were dispersed. The plates were then dried and calcined with an electrical dryer at 500 °C for 30 min to strengthen the bonding of the TiO2 particles onto the collection plates. The process of making the hydrophilic collection surfaces was based on the method described by Lin et al.Citation28 and Tsai et al.Citation35 To generate strong electrostatic fields for the collection plates, the polished S/S plate (80 × 160 × 5 mm) was inserted at the center of the collection cell and was connected to a high-voltage power supply. The gap between the high-voltage power supply and the collection plates was 6.4 mm, and test air was streamed through the central area (17.8 × 98 mm).
Figure 1. Schematics of the two-stage wet ESP developed in this study. The stages consist of the carbon brush pre-charger (a) and the collection cell (b).
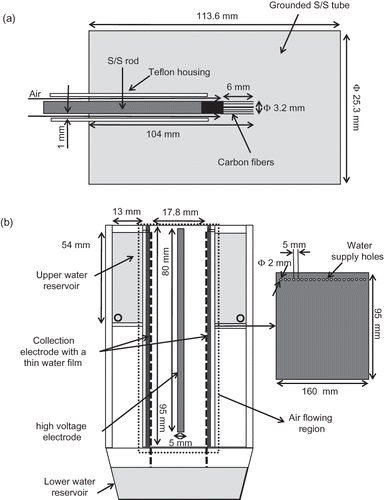
shows the experimental setup used in this study. Potassium chloride (KCl) aerosol particles with diameters in the range of 0.01∼0.5 μm were generated by nebulizing a 0.05% KCl-water solution using a constant-output atomizer (model 3076; TSI Inc., Shoreview, MN, U.S.A.) and passing this through a Kr-85 neutralizer and a diffusion dryer. The number mean diameter, number concentration, and geometric standard deviation of the particles generated were approximately 0.045 μm, 2.5 × 105 particles/cm3, and 1.7, respectively. The charged fractions of the test particles were removed using a plate condenser (to which a voltage of −10 kV was applied), and uncharged particles were then introduced into the upstream portion of the carbon brush pre-charger. Clean compressed air was mixed with these particles and the mixture subsequently passed through the carbon brush pre-charger and collection cell. The total air flow rate through the wet-type ESP was maintained at 95 L/min using mass flow controllers (MFCs; model Tylan FC-2920 Series-100 slpm; Mykrolis Corporation, Billerica, MA, U.S.A.); this flow rate corresponded to flow speeds of 4 m/sec through the carbon brush pre-charger and 1.0 m/sec through the collection cell. High-voltage power supplies (max. ±30 kV/10 mA; Korea Switching, Seoul, Korea) were connected to the pre-charger and the collection cell. The applied voltages and corona currents were measured by using digital multimeters (model 286; Fluke Corporation, Everett, WA, U.S.A). The voltage to the pre-charger was allowed to vary from 2 to 8 kV, and from 4 to 8 kV, to understand the effect of the applied voltages on the performances of the charger and the whole ESP. The polarity of the voltage applied to the pre-charger was also changed to understand the effect of polarity changes on the pre-charger and ESP performances. For wetting the collection plates, the water flow rate per collection surface area was 6.5 L/min/m2 (0.16 × 0.095 m2). The size distributions of the test particles downstream of the collection cell were measured using a scanning mobility particle sizer (SMPS; model 3081; TSI, Shoreview, MN, U.S.A.) system, as well as a condensation particle counter (CPC; model 3076; TSI, Shoreview, MN, U.S.A.) The concentration of ozone emitted from the pre-charger was measured using an ozone monitor (model API 400E; Teledyne Technologies Inc., Thousand Oaks, CA, U.S.A), while varying the voltage applied to the pre-charger.
The performance of the ESP used in this study was expressed in terms of the particle collection efficiency (η), which may be obtained by using the following equation:
where η is the particle collection efficiency, C 1 is the number concentration of the particles downstream from the ESP without any applied voltage, and C 2 is the number concentration of the particles downstream from the ESP with voltages applied to its charger or collection cell.
The long-term collection performances of dry- and wet-type ESPs during dust loading were compared using JIS standard dust (Class 8, mass mean diameter 1.6∼2.3 μm; JIS standard dust, Japan) for the dust loading of the collection plates, and submicrometer KCl particles for measuring the transient collection efficiency of the ESP during the test. A venture-type dust dispersion device was used to generate and supply the dusts upstream to the pre-charger (). The mass concentration of the loading dust that flowed into the ESP was maintained at approximately 50 mg/m3. Prior to dust loading, the collection efficiency of the ESP without any dust collected on the collection plates was measured. The transient collection efficiency was then measured every 15 min after each dust loading of 1 g, by means of KCl particles. The polluted water was removed periodically from the lower water reservoir. During the experiments, the operational parameters of the pre-charger and collection cell were varied to investigate their effect on the performance of the wet-type ESP. summarizes the experimental conditions.
Table 1. Parameters and test conditions used to study wet ESP performance
PARTICLE CHARGING AND COLLECTION THEORY FOR THE TWO-STAGE WET ESP
For the charging theory of an ESP, it seems to be reasonable to consider charging theories describing charging processes continuously from small to larger particles. Usually charging process is divided into a field charging region for the particles larger than 1 μm, and a diffusion charging region for particles smaller than 0.1 μm. In this study, the Cochet's charging model was mainly used because it allowed an easy calculation and the correlation to actual ESP conditions is quite reasonable in the critical size range from 0.1 to 1 μm.Citation36,Citation37
The Deutsch model is a well-known fundamental model used to calculate the collection efficiency of a dry ESP.Citation36–Citation38 The Deutsch model assumes that there is complete mixing transverse to the flow direction due to turbulent dispersion; no axial dispersion is considered.Citation39,Citation40 As a result of this assumption, a uniform particle concentration profile is maintained, and thus the concentration decays exponentially in the flow direction. This leads to the Deutsch efficiency formula as follows:
where η is the collection efficiency, W m is the migration velocity to the collection plate caused by the electric field for the collection (E C), v is the mean air flow velocity, L is the length of the collection plate, and s is the distance between the high-voltage electrode and the collection plate of the ESP. Assuming that Stokes' drag law is valid, the theoretical migration velocity of particles at a steady state resulting from a balance of the drag and electrical forces on the particles is as follows:
where μ is the viscosity of air and Q p is the particle saturation charge, which is given by the equationCitation41,Citation42
where E p is the electrical field for the particle charging, λ is the mean free path of the gas molecules, and ϵ r and ϵ0 are the relative electrical permittivity of gas and of a vacuum, respectively. The Cunningham correction factor, Cu, as a slip correction factor applied whenever the particle sizes approach molecular sizes, is also given as followsCitation43:
The diffusion charging theory based on the Fuchs limiting sphere theory is also used for the particles smaller than 0.1 μm because the diffusion charging is predominantly used for nanoparticle charging, and nanoparticle collection in ESPs depends primarily on particle charging efficiency within an ESP and therefore on particle size.Citation44
The diffusion charging of aerosol particles induced by unipolar ions can be expressed as followsCitation45:
where n is the number concentration of particles, N i is the ion concentration, and β is the attachment coefficient of an ion with a particle. The subscript 0 indicates the neutral state and the superscript s denotes the polarity of the ions. Equation 6 represents the time-dependent change in number concentration for uncharged particles. If the initial particles are all uncharged, from the eq 6 the following analytical solution can be obtained.
For the value of β, Fuchs' limiting sphere theory is used.Citation46 The Fuchs theory is most commonly used for the diffusion charging of particles in the transient regime, and the equation for combination coefficient, β, based on Fuchs theory is as follows:
where
The subscript p indicates the number of the elementary charges and their polarity. C i, D i, and m i are the thermal velocity, diffusion coefficient, and mass of an ion, respectively. k, T, and a are the Boltzman constant, absolute temperature, and particle radius, respectively. φ and r represent the electrostatic potential of the ion and particle, and the distance between the centers of the particle and ion, respectively. ϵ0 and ϵ1 are the vacuum permittivity and the dielectric constant of the particle, respectively.
σ is the radius of the limiting sphere, and is a function of the mean free path of the ion, λi. α is the fraction of ions entering the limiting sphere that reach the particle surface, expressed as the ratio of minimum impact parameter, b m and the radius of the limiting sphere, σ. L m is the minimum apsoidal distance. β p depends significantly on the ion properties such as C i, D i, and λ i , which can be derived using the mass, m i, and electrical mobility, Z i of ions as follows:
where M is the molecular weight of the carrier gas. Therefore, at least mass and electrical mobility of ions have to be known to calculate β p .
RESULT AND DISCUSSION
Electrical Performance and Ozone Emission of the Carbon Brush Pre-Charger
shows current variation against the voltages applied to the carbon brush pre-charger. The test air flow rate was maintained at 95 L/min, which corresponded to a flow velocity of 4 m/sec through the carbon brush pre-charger. A negative corona led to higher corona currents than a positive corona for the same applied voltage because of higher electrical mobility of negative ions due to lower clustering of the moleculesCitation47 and the photoelectric effects from the surface of the discharging electrodes at the negative corona.Citation48 shows the variation in the concentration of ozone emitted from the carbon brush pre-charger, plotted against the voltage applied to the pre-charger for different polarities at a constant air velocity of 4 m/sec. The average ozone concentration was proportional to the voltage applied to the pre-charger, and ozone generation was much higher with a negative high voltage than with a positive high voltage.Citation11,Citation49 In particular, when the voltage applied to the charger was less than 4 kV, the ozone concentration was less than 30 ppb, which was significantly lower than the 60∼120 ppb limit set out in the Federal Aviation Administration-recommended level and the U.S. Environmental Protection Agency's National Ambient Air Quality Standard.Citation50
Figure 3. Voltage-current curves of the carbon brush pre-charger for negative and positive polarities.
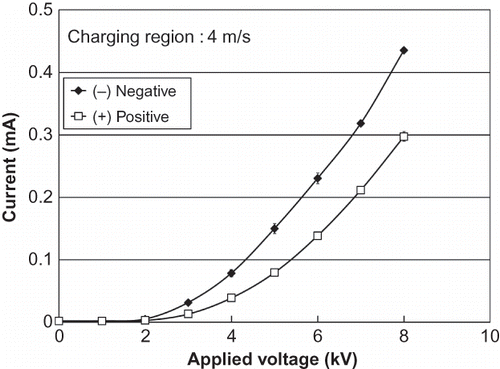
Figure 4. Ozone emission as a function of the voltage applied to the pre-charger for negative and positive polarities.
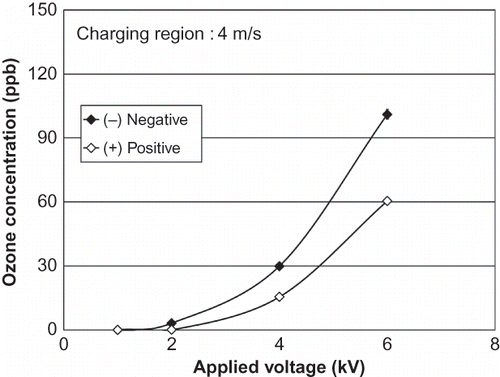
The lower ozone emission from the carbon fiber pre-charger resulted from the thinner diameter of the fibers, only about 5 μm which is significantly thinner than the diameter of wires or rigid type electrodes for the general ESPs, approximately several hundred micrometers or millimeters in dimension because the ionization and dissociation areas of oxygen decreases with decreasing the thickness of discharge electrodes in the pre-charger.Citation17
Particle Capture in the Carbon Brush Pre-Charging Zone
In general, for the industrial purpose it is necessary to minimize particle capture in the pre-charing zone of two-stage ESPs because particle contamination on the ground tube by the particle capture in the zone for a long operation time could decrease the electrical field in the pre-charging zone, and thus reduce the particle charging efficiency in the charging zone.
The particle capture only in the pre-charging zone was measured with particle size distributions at upstream and downstream of the pre-charging zone with and without an applied voltage to find out a range of applied voltage to the pre-charger to minimize the particle contamination in the zone.
shows the relationship between the particle-capture efficiency of submicrometer particles in the pre-charger and the voltage applied to it. The polarity of the applied voltage was negative, and air velocity was 4 m/sec. As the applied voltage increased, the particle-capture efficiency increased across the whole range of particle sizes. It should be noted that increased particle capture could result in a reduction of the electrical field for particle charging due to particle contamination on the grounded tube. The smallest particle capture in the pre-charger was observed for particles between 100 and 200 nm in mobility diameter, regardless of the applied voltage. In particular, particle capture of less than 10% within the entire size range was acquired with the applied voltages of 2 and 4 kV for a negative polarity.
Figure 5. Collection efficiency of submicrometer particles against particle size with different voltages applied to the pre-charger.
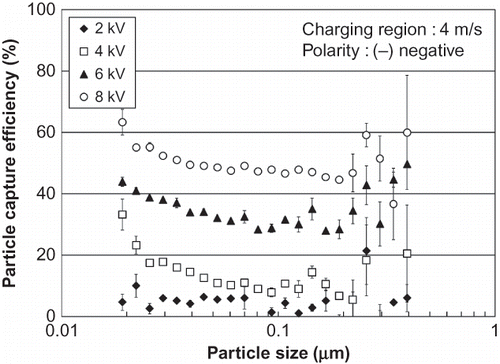
shows the variation in submicrometer particle-capture efficiency with applied voltage at different corona polarities for particle sizes of 0.045, 0.093, and 0.191 μm. The particle capture for both polarities was proportional to the voltage applied to the pre-charger. For a given applied voltage, particle capture was higher with a negative corona than with a positive corona due to the higher ionic mobility of negative ions.Citation47 However, for both polarities, less than 10% particle capture was obtained with an applied voltage of less than 4 kV.
Figure 6. Collection efficiency of submicrometer particles against voltage applied at different high voltage polarities for particle sizes of 0.045, 0.093, and 0.191 μm.

To minimize ozone generation to less than 30 ppb and particle capture in the pre-charger to less than 10%, the voltage applied to the pre-charger was kept at 2 or 4 kV during testing the particle collection performance of the ESP developed in this study.
Collection Performances for Submicrometer Particles of the Two-Stage Wet ESP
shows the relationship between the collection efficiency of submicrometer particles in the ESP and the voltage applied to the collection plates. Positive polarity voltages were applied to the pre-charger and the high-voltage between ground plates in the middle of the collection cell; voltages of 2 and 4 kV were applied to the pre-charger. The test air flow rate was 95 L/min, again corresponding to flow velocities of 4 and 1.0 m/sec through the charger and collection cell, respectively. The water supply onto the collection plates was maintained at 6.5 L/min/m2, and the particle capture in the pre-charger without any voltage applied to the collection cell was less than approximately 10%. Increasing the voltage applied to collection plates increased the collection efficiency for almost all particles because the higher electrostatic intensity increased the migration velocity of the particles to the plates, as shown in .Citation51 However, for particles smaller than 0.05∼0.06 μm in diameter, as particle size decreased, collection efficiency decreased at all applied voltages due to the partial charging effect in this size range; this finding agreed with the results of previous studies.Citation1,Citation4,Citation52,Citation53 In particular, for particles smaller than 0.03 μm, the increase in electrostatic intensity between the collection plates had almost no effect on the collection efficiency. Particle collection was restricted by the charging fraction of particle size ranges in the charger.Citation4 This phenomenon can be regarded as a non-ideal effect, which cannot be explained by the Deutsch theory.Citation1 As shown in , the collection efficiency was also enhanced by increasing the voltage applied to the collection plates. The collection efficiency in the peak sizes with 4 kV voltage applied to the pre-charger averaged 85% and was enhanced by 10∼30%. In particular, compared with results for 2 kV applied to the pre-charger, with 4 kV, the collection efficiency for particles smaller than 0.03 μm in diameter increased significantly (by 20∼40%), with the peak efficiency being shifted to smaller particles. This increased collection efficiency in the smaller size range could result from the collection efficiency becoming significantly high when there was a large enough number of ions, as particles are almost fully charged by acquiring just one ion. Particles in the ultrafine size range usually have a very high electrostatic migration velocity. However, as the number of ions is decreased, the efficiency for very small particles drops dramatically as a result of the decreased charging fraction.4
Figure 7. Collection efficiency of submicrometer particles as a function of particle size for different positive voltages applied to the collection plates. (a) 2 kV and (b) 4 kV applied to the carbon brush pre-charger.

shows the relationship between the collection efficiency of submicrometer particles in the ESP and the voltage applied to the collection plates within the ESP. The polarity of the voltage applied to the pre-charger and collection plates was negative. Voltages of 2 and 4 kV were applied to the pre-charger, and the test air flow rate was 95 L/min, again corresponding to flow velocities of 4 m/sec through the charger and 1.0 m/sec through the collection cell. The water supply onto the collection plates was maintained at 6.5 L/min/m2. Particle capture in the pre-charger was approximately 10% with both 2 and 4 kV. As shown in , the size-dependent and electrostatic-field-dependent collection efficiencies agreed well with those from the experiments with positive voltage. However, the entire collection efficiencies were enhanced by approximately 20% and 10% for voltage levels of 2 and 4 kV, respectively, applied to the pre-charger, in comparison to those for the same applied voltage levels for positive polarity. In particular, the average particle collection efficiency exceeded 90% for submicrometer particles when negative voltages of 4 and 8 kV were applied to the pre-charger and collection plates, respectively.
Figure 8. Collection efficiency of submicrometer particles as a function of particle size for different negative voltages applied to the collection plates. (a) −2 kV and (b) −4 kV applied to the carbon brush pre-charger.
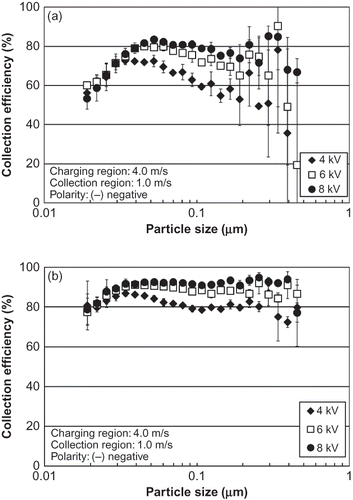
shows the comparison of collection efficiency as a function of particle size, as found in this study, and the efficiency that was predicted by different theories that were based on Equationeqs 2 Equation Equation–Equation15. Voltages applied to the pre-charger and the collection plates were 4 kV and 4, 6, and 8 kV, respectively, and the air velocities through the pre-charger and collection plates were 4 and 1.0 m/sec, respectively. For particles larger than 0.05 μm, the experimental collection efficiencies were similar to those predicted by Deutsch ESP collection theory, based on the Cochet's charging theory.Citation39,Citation41 However, differences between the results and those predicted by the collection theory increased as particle sizes decreased to smaller than 0.05 μm because the charging for the particles in the size range is significantly related to diffusion charging.Citation54 Therefore, the theoretical collection efficiency for the particles smaller than 0.05 μm, based on the Fuchs diffusion charging theory, was calculated with the product of ion number concentration, N i, and charging time, t, which was approximately 6.0 × 10Citation12 sec/m3 with the test conditions of this study. For the collection efficiency, it was assumed that charged particles in the charging section were completely collected in the collection zone, regardless of the increase in applied voltage to collection plates.Citation44 Shown in , for the particles smaller than approximately 0.05 μm, the efficiency was also fairly similar to those predicted by the theoretical calculation. The efficiency was decreased as particle size decreased below 0.05 μm, and these results were also observed in previous studies because of the poor diffusion charging and the lower ion attachment coefficients for the particles.Citation16,Citation45,Citation53
Long-Term Collection Performances of Two-Stage Dry- and Wet-Type ESPs during Dust Loading
shows variation in collection efficiency of the ESPs plotted against particle size for different particle loadings with and without water films on the plates. The collection plates were intentionally polluted using dust loading with a mass concentration of approximately 50 mg/m3. Applied voltages to the pre-charger and collection plates were 4 and 8 kV, respectively, and air velocities through the pre-charger and collection plates were 4 and 1.0 m/sec. As shown in , the collection efficiency for submicrometer particles was approximately 90% for the clean collection plates at the beginning of the experiment over the entire size range. The average collection efficiency of the ESP fell from 90% to 75% after the first dust loading and to 70% after the second, whereas particle capture in the pre-charging zone without any voltage to the collection cell was maintained at approximately 10%. This result is explained by reduced electric field strength in the collection cell due to dust accumulation on the collection plates,28 whereas no significant particle deposition occurred in the pre-charger. However, as shown in , for the wet ESP, in which collection plates were continuously cleaned by water, collection efficiency was maintained at approximately 90% of the collection efficiency of the initial clean ESP after the two dust loadings.
Figure 10. Collection efficiency of the ESP plotted against particle size for different particle loadings with and without a water film on the plates. (a) Without the water film; (b) with the water film flowing at a rate of 6.5 L/min/m2.
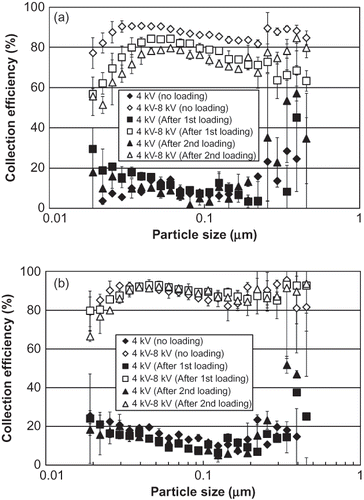
This finding shows that high-efficiency collection performance could be achieved over a long term by continuously cleaning the collection plates with a thin water film. In contrast, conventional dry ESPs require rapping and water spraying of the collection plates to maintain their collection efficiency. The dry devices also generate particle re-entrainment, which results in a low collection efficiency for submicrometer particles.
CONCLUSION
We have developed a novel two-stage wet ESP that uses a carbon brush pre-charger and collection plates with a thin water film. The performance of the ESP was evaluated experimentally for ultrafine particles in the size range of 0.01∼0.5 μm by varying the voltage levels and polarity that were applied to the pre-charger and the collection plates. The results were compared with the theoretical predictions for ESPs. The long-term performance of the ESP with and without water films was also investigated using a standard JIS dust with a size range measured in micrometers.
The experiments showed that the two-stage wet ESP with the carbon brush pre-charger resulted in approximately 10% particle capture, while producing ozone concentrations of less than 20∼30 ppb at voltages up to 4 kV, regardless of polarity, which is significantly lower than the current limits set by international agencies. In particular, the ESP achieved a high collection performance, averaging 90% with negative voltage and 80% with positive voltage, for ultrafine particles based on number concentration. The experimental results were similar to those from the theoretical calculation with the same parameters. Furthermore, a high ESP collection efficiency could be achieved by increasing the voltages applied to the pre-charger and collection plates. In addition, the decreased collection efficiency that occurred during dust loading without water films was completely eliminated by forming a thin water film at a water flow rate of 6.5 L/min/m2.
The ESP described herein is thus a promising posttreatment system for removing ultrafine particles, especially in industries where small particulates are emitted in acidic and corrosive gases that could degrade the performance of conventional dry ESPs. Using positive polarity, this process could also be applied to air-cleaning devices to achieve high removal efficiency of over 80% for indoor ultrafine particles with negligible ozone production.
ACKNOWLEDGMENTS
This research was partially funded by the Energy Resources Technology Development Project (NE3760), run under the auspices of the Korean Government, and was also supported by the Basic Research Fund (NK163C) and the General Research Fund (SC0820) of the Korea Institute of Machinery and Materials.
REFERENCES
- Yoo , K.H. , Lee , J.S. and Oh , M.D. 1997 . Charging and Collection of Submicron Particles in Two-Stage Parallel-Plate Electrostatic Precipitators . Aerosol. Sci. Tech , 27 : 308 – 323 .
- Zukeran , A. , Ikeda , Y. , Ehara , Y. , Matsuyama , M. , Ito , T. , Takahashi , T. , Kawakami , H. and Takamatsu , T. 1999 . Two-Stage-Type Electrostatic Precipitator Re-entrainment Phenomena under Diesel Flue Gases . IEEE. Trans. Ind. Appl , 35 : 346 – 351 .
- Grinshpun , S.A. , Mainelis , G. , Trunov , M. , Adhikari , A. , Reponen , T. and Willeke , K. 2005 . Evaluation of Ionic Air Purifiers for Reducing Aerosol Exposure in Confined Indoor Spaces . Indoor Air , 15 : 235 – 245 .
- Li , M.; D. and Christofides , P. 2006 . Collection Efficiency of Nanosize Particles in a Two-Stage Electrostatic Precipitator . Ind. Eng. Chem. Res , 45 : 8484 – 8491 .
- Electrostatic Precipitator Knowledgebase. ESP Operation; Neundorfer, Inc.: Willoughby, OH, 2007; vol. 1, pp 10–11 http://www.neundorfer.com/FileUploads/CMSFiles/ESP%20Operation[0].pdf (http://www.neundorfer.com/FileUploads/CMSFiles/ESP%20Operation[0].pdf)
- Talaie , M.R. , Fathikaljahi , J , Taheri , M. and Bahri , P. 2001 . Mathematical Modelling of Double-Stage Electrostatic Precipitators Based on a Modified Eulerian Approach . Aerosol. Sci. Technol , 34 : 512 – 519 .
- Jayaram , S. , Castle , G.S.P. , Chang , J-S. , Berezin , A.A. , Looy , P.C. , Mangal , R. and Mozes , M.S. 1996 . Semi Pilot Plant Pulse Energized Cold-Pre-charger Electrostatic Precipitator Tests for Collection of Moderately High Resistivity Flyash Particles . IEEE Trans. Ind. Appl , 323 : 851 – 857 .
- Zukeran , A. , Looy , P.C. , Berezin , A.A. , Chang , J-S. and Ito , T. 1997 . Enhancement of Electrostatic Precipitator Ultrafine Particle Collection Efficiency by Pre-chargers . J. Aerosol. Sci , 28 ( Suppl. 1 ) : S281 – S282 .
- Zhu , J. , Zhang , X. , Chen , W. , Shi , Y. and Yan , K. 2010 . Electrostatic Precipitator of Fine Particles with a Bipolar Pre-charger . J. Electrostat , 68 : 174 – 178 .
- Liu , L. , Guo , J. , Li , J. and Sheng , L. 2000 . The Effect of Wire Heating and Configuration on Ozone Emission in a Negative Ion Generator; J . Electrostat , 48 : 81 – 91 .
- Yehia , A. , Abdel-Salam , M. and Mizuno , A. 2000 . On Assessment of Ozone Generation in DC Coronas . J. Phys. D Appl. Phys , 33 : 831 – 835 .
- Yehia , A. , Mizuno , A. and Takashima , K. 2000 . On the Characteristics of the Corona Discharge in a Wire-Duct Reactor . J. Phys. D. Appl. Phys , 33 : 2807 – 2807 .
- Bell , M.L. , Peng , R.D. and Dominici , F. 2006 . The Exposure-Response Curve for Ozone and Risk of Mortality and the Adequacy of Current Ozone Regulations . Environ. Health. Perspect , 114 : 532 – 536 .
- Cardello , N. , Volckens , J. , Tolocka , M.P. , Wiener , R. and Buckley , T.J. 2002 . Performance of a Personal Electrostatic Precipitator Particle Sampler . Aerosol Sci. Technol , 36 : 162 – 165 .
- Goheen , S.C. , Larkin , E.C. and Bissell , M.G. 1984 . Ozone Produced by Corona Discharge in the Presence of Water . Int. J. Biometeorol , 28 : 157 – 161 .
- Kulkarni , P. , Namiki , N. , Otani , Y. and Biswas , P. 2002 . Charging of the Particles in Unipolar Coronas Irradiated by In-Situ Soft X-ray: Enhancement of Capture Efficiency of Ultrafine Particles . J. Aerosol Sci , 33 : 1279 – 1296 .
- Yasumoto , K. , Zukeran , A. , Takagi , Y. , Ehara , Y. , Takahashi , T. and Yamamoto , T. 2008 . Effect of Electode Thickness for Reducing Ozone Generation in Electrostatic Precipitator . Denkkai Ronbunshi , 128–A : 689 – 694 .
- Jaasund , S.S. 1981 . Control of Fine Particle Emissions with Wet Electrostatic Precipitation . Environ. Int , 6 : 233 – 238 .
- Musdalslien , U.I. , Standal , N.A. , Johansen , J.G. and Oehme , M. 1991 . Pilot Plant Tests with a Wet Electrostatic Precipitator for Reducing PCDD/PCDF in Corrosive Off-Gas from Magnesium Production . Chemosphere , 23 : 1097 – 1108 .
- Altman , R. , Offen , G. , Buckley , W. and Ray , I. 2001 . Wet Electrostatic Precipitation Demonstrating Promise for Fine Particulate Control—Part I . Power Eng , 105 : 37 – 39 .
- Lee , W.-J. , Chen , S.J. , Liow , M.C. , Wang , L.C. and Huang , K.L. 1998 . Removal Efficiencies of PAHs by the Electrostatic Precipitator and Wet Scrubber . J. Aerosol. Sci , 29 ( Suppl. 1 ) : S1081 – S1082 .
- Staehle , R.C. , Triscori , R.J. , Ross , G. , Kumar , K.S. and Pasternak , E. May 19-22 2003 . “ The Past, Present and Future of Wet Electrostatic Precipitators in Power Plant Applications ” . In Proceedings of the Power Plant Air Pollutant Control “Mega” Symposium 2003 , May 19-22 , BR – 1742 . Washington , DC : Babcock & Wilcox Company: Barberton, OH, 2003 .
- Saiyasitpanich , P. , Keener , T.C. , Lu , M. and Liang , F. 2008 . Control of Diesel Gaseous and Particulate Emissions with a Tube-Type Wet Electrostatic Precipitator . J. Air Waste Manage. Assoc , 58 : 1311 – 1317 . doi: 10.3155/1047-3289.58.10.1311.
- Snyer , R.E. , Moretti , A.L. , Tonn , D.P. , Silva , A.A. , Kumar , S. and Lau , A. August 25-28 2008 . “ SO3 and Fine Particulate Mitigation at AES Deepwater ” . In Proceedings of the Power Plant Air Pollutant Control “Mega” Symposium 2008 , August 25-28 , BR – 1814 . Baltimore , MD : Babcock and Wilcox Power Generation, Inc.: Barberton, OH, 2008 .
- Bologa , A. , Paur , H. , Seifert , H. , Wascher , T. and Woletz , K. 2009 . Novel Wet Electrostatic Precipitator for Collection of Fine Aerosol . J. Electrostat , 67 : 150 – 153 .
- Bayless , D.J. , Alam , M.K. , Radcliff , R. and Caine , J. 2004 . Membrane-Based Wet Electrostatic Precipitation . Fuel. Process. Technol , 85 : 781 – 798 .
- Pasic , H. , Alam , M.K. and Bayless , D.J. 2001 . Membrane Electrostatic Precipitator . U.S. Patent 6, 231, 643, B1 , May 15
- Lin , G. , Tsai , C. , Chen , S. , Chen , T. and Li , S. 2010 . An Efficient Single-Stage Wet Electrostatic Precipitator For Fine And Nanosized Particle Control . Aerosol. Sci. Technol , 44 : 38 – 45 .
- Peterson , M.S. , Zhang , W. , Fisher , T.S. and Garimella , S.V. 2005 . Low-Voltage Ionization of Air with Carbon-Based Materials . Plasma Sources Sci. Technol , 14 : 654 – 660 .
- Chen , C. , Huang , B. , Lin , T. , Chen , I. and Hsu , C. 2006 . A New Negative Ion Generator Using ZnO Nanowire Array . J. Electrochem. Soc , 153 : G894 – G896 .
- Han , B. , Hudda , N. , Ning , Z. , Kim , Y.J. and Sioutas , C. 2008 . Enhanced Unipolar Charging of Concentration-Enriched Particles Using Water-Based Condensational Growth . J. Aerosol. Sci , 39 : 770 – 784 .
- Han , B. , Kim , H.J. , Kim , Y.J. and Sioutas , C. 2008 . Unipolar Charging of Fine and Ultra-Fine Particles Using Carbon Fiber Ionizers . Aerosol Sci. Technol , 42 : 793 – 800 .
- Han , B. , Hudda , N. , Ning , Z. , Kim , H.J. , Kim , Y.J. and Sioutas , C. 2009 . A Novel Bipolar Charger for Submicron Aerosol Particles Using Carbon Fiber Ionizers . J. Aerosol. Sci , 40 : 285 – 294 .
- Han , B. , Hudda , N. , Ning , Z. , Kim , Y.J. and Sioutas , C. 2009 . Efficient Collection of Atmospheric Aerosols with a Particle Concentrator-Electrostatic Precipitator Sampler . Aerosol Sci. Technol , 43 : 757 – 766 .
- Tsai , C.J. , Lin , G.Y. and Chen , S.C. 2008 . A Parallel Plate Wet Denuder for Acidic Gas Measurement . AICHE J , 54 : 2198 – 2205 .
- Parker , K.R. 1997 . “ Basic and theoretical Operation of ESPs ” . In Applied Electrostatic Precipitation , 1st , 5267 London : Blackie Academic & Professional .
- Park , J.H. and Chen , C.H. 2002 . An Improved Modeling for Prediction of Grade Efficiency of Electrostatic Precipitators with Negative Corona . J. Aerosol. Sci , 33 : 673 – 694 .
- Xiangron , Z. , Lianze , W. and Keqin , Z. 2002 . An Analysis of a Wire–Plate Electrostatic Precipitator . J. Aerosol. Sci , 33 : 1595 – 1600 .
- Deutsch , W. 1922 . Bewegung und Ladung der Elektrizitätsträger im Zylinderkondensator . Ann. Phys , 68 : 335 – 344 .
- Gutiérrez Ortiz , F.J. , Navarrete , B. and Cañada , L. 2010 . Dimensional Analysis for Assessing the Performance of Electrostatic Precipitators . Fuel Process. Technol , 91 : 1783 – 1793 .
- Cochet , R . 1961 . Lois Charge des Fines Particules (Submicroniques) Etudes Théoriques—Controles Récents Spectre de Particules . Colloque International la Physique des Forces Electrostatiques et Leurs Application , 102 : 331 – 338 .
- Bai , H. , Lu , C. and Chang , C.L. 1995 . A Model to Predict the System Performance of an Electrostatic Precipitator for Collecting Polydisperse Particles . J. Air Waste Manage. Assoc , 45 : 908 – 916 .
- Flagan , R.C. and Seinfeld , J.H. 1988 . Fundamentals of Air Pollution Engineering , Upper Saddle River , NJ : Prentice-Hall, Inc .
- Suriyawong , A. , Hogan , C.J Jr. , Jiang , J. and Biswas , P. 2008 . Charged Fraction And Electrostatic Collection of Ultrafine and Submicrometer Particles Formed during O2-CO2 Coal Combustion . Fuel , 87 : 673 – 682 .
- Adachi , M. , Kousaka , Y. and Okuyama , K. 1985 . Unipolar and Bipolar Diffusion Charging of Ultrafine Aerosol Particles . J. Aerosol Sci , 16 : 109 – 123 .
- Fuchs , N. A. 1963 . On the Stationary Charge Distribution on Aerosol Particles in a Bipolar Ionic Atmosphere . Geofis. Pura Appl , 56 : 185 – 193 .
- Cabane , M. and Playe , P. 1980 . Mass Spectra of Negative Ions in Air-Like Gas Mixtures at Atmospheric Pressure . J. Aerosol. Sci , 11 : 475 – 482 .
- Trichel , G.W. 1938 . The Mechanism of the Negative Point to Plane Corona Near Onset . Phys. Rev , 54 : 1078 – 1084 .
- Nomoto , Y , Ohkubo , T. , Kanazawa , S. and Adachi , T. 1995 . Improvement of Ozone Yield by a Silent-Surface Hybrid Discharger Ozonizer . IEEE Trans. Ind. Appl , 31 : 1458 – 1462 .
- Spengler , J.D. , Ludwig , S. and Weker , R.A. 2004 . Ozone Exposures during Trans-Continental and Trans-Pacific Flights . Indoor Air , 14 ( Suppl 7 ) : 67 – 73 .
- White , H.J. 1963 . Industrial Electrostatic Precipitation , 1st , Boston , MA : Addison-Wesley Publication Company, Inc .
- Huang , S.H. and Chen , C.C. 2002 . Ultrafine Aerosol Penetration through Electrostatic Precipitators . Environ. Sci. Technol , 36 : 4625 – 4632 .
- Zhuang , Y. , Kim , Y.J. , Lee , T.G. and Biswas , P. 2000 . Experimental and Theoretical Studies of Ultra-Fine Particle Behavior in Electrostatic Precipitators . J. Electrostat , 48 : 245 – 260 .
- Hinds , W.C. 1999 . Aerosol Technology: Properties, Behavior, and Measurement of Airborne Particles , 2nd , New York : John Wiley & Sons, Inc .