ABSTRACT
Laser printers are one of the common indoor equipment in schools, offices, and various other places. Laser printers have recently been identified as a potential source of indoor air pollution. This study examines the characteristics of ultrafine particles (UFPs, diameter <100 nm) and volatile organic compounds (VOCs) emitted from laser printers housed in a commercial printing center. The results indicated that apart from the printer type, the age of printers, and the number of pages printed, the characteristics of UFPs emitted from printers also depend on indoor ventilation conditions. It was found that at reduced ventilation rates of indoor air, there was a rise in the number concentration of UFPs in the printing center. Interestingly, the contribution of UFPs to the total number of submicrometer-sized particles was observed to be higher at a sampling point far away from the printer than the one in the immediate vicinity of the printer. Black carbon (BC) measurements showed a good correlation (r S = 0.82) with particles in the size range of 100–560 nm than those with diameters less than 100 nm (r S = 0.33 for 50–100 nm, and r S = −0.19 for 5.6–50 nm particles). Measurements of VOCs in the printing center showed high levels of m-, o-, and p-xylene, styrene, and ethylbenzenes during peak hours of printing. Although toluene was found in higher levels, its concentration decreased during peak hours compared to those during nonoperating hours of the printing center.
This study reports the emission of ultrafine particles (UFPs) and volatile organic compounds (VOCs) from laser printers in a commercial printing center. It was found that the number concentration of UFPs released was not only influenced by the printer-related parameters, but also by indoor ventilation conditions. The fraction of UFPs was high at lower air change rate and at distances away from printers, presumably due to the formation of new particles. The results imply that laser printers equipped with particle traps and high ventilation rates in the commercial printing center are needed to reduce the exposure of occupants to UFPs and thus the associated health risk.
INTRODUCTION
Office environments have been changing rapidly from the beginning of information era. More sophisticated and high-technology computers, photocopiers, laser printers, and fax machines are being increasingly used in workplace these days. These electronic equipments have improved the efficiency of work, but also brought adverse changes in indoor air quality (IAQ). In the recent decade, several studiesCitation1,Citation2 have identified laser printers as one of the potential sources of indoor air pollution. Ultrafine particles (UFPs) and volatile organic compounds (VOCs), which pose serious threats to human health, were found in printer emissions.Citation1,Citation3–6 UFPs are either released during printing activities, or formed as a consequence of reactions between ozone (O3) and VOCs that are released from printersCitation7. StudiesCitation3,Citation7–10 were initiated to characterize emission profiles for UFPs, and to determine concentrations of VOCs and other chemical substances emitted during printing activities so that the possible health impacts from printer emissions can be understood and estimated thoroughly. He et al.Citation9 investigated particle emissions from 62 printers and classified the printers into three types based on their emission characteristics. They found that UFPs contributed up to 98–99% of total submicrometer-sized particles emitted from the printers with peak diameters down to 40 nm. In the same year, Kagi et al.Citation7 found that printers release a large amount of VOCs. They found that concentration levels of VOCs (e.g., styrene and xylenes) increased during printing activities and the source of styrene was toner dust in printer cartridges. Recently, Wensing et al.Citation10 monitored printer emissions in a chamber and found that along with UFPs, some SVOCs (semivolatile organic compounds) were also released during the printing activity.
Most of the studies on printer emissions conducted so far used controlled environmental chambers to characterize the UFPs and VOCs emitted from printers. To gain a better and comprehensive understanding of health impacts, characterization of printer emissions in workplaces such as commercial printing centers is needed. Very few studiesCitation9–11 were found in the literature that have reported printer emissions in real office indoor environments. Even in those studies, the duration of sampling was limited to a few days (2–3 days). Commercial printing centers have quite different indoor environments when compared to offices because of the heavy usage of a number of printers throughout the day. People working in such places are exposed to very high concentration levels of printer emissions. For example, Dufresne et al.Citation12 reported that the concentration level of lanthanides in the lung tissue of a person who worked in a printing shop for 14 years was higher than the average concentrations measured in 41 other workers who had died of cancer at various other sites. This finding highlights the importance of and the need for conducting emission studies from laser printers in commercial printing centers.
This study was initiated to gain insights into the emission profiles of laser printers used in a commercial printing center with specific reference to UFPs and VOCs. Indoor air of a commercial printing center located at the National University of Singapore was investigated to characterize the emissions of UFPs and VOCs from printers. A complementary study was conducted using a custom-designed chamber to find out the similarities and dissimilarities in the emission characteristics of airborne particles between the real workplace and the controlled chamber study.
EXPERIMENTAL
Sampling Site
Air sampling was done in a commercial printing center located on the 6th floor of a multistorey building (Yusof Ishak Hall) at the National University of Singapore. It is a printing facility that is heavily used by the University students. shows the layout of the printing center. It has a total of 40 computer desktops (Dell Optiplex GX280), 2 HP laser monochrome printers (model 4300dtn), and 1 HP color laser printer (model 5500dn). The printing center has both ceiling-mounted and wall-mounted air conditioning units and two doors. One of the doors was permanently closed, wherease the other door was in use for both entry as well as exit. Business hours, room dimensions, environmental conditions, and types of ventilation are presented in . At night time after business hours, the air conditioning units were shut down. Air change per hour is measured by using sulfur hexafluoride (SF6) as a tracer gas and observing the decay concentrations at indoor locations using a monitor based on infrared photoacoustic spectroscopic (PAS) method. Tracer gas was introduced in the air-handling unit under normal occupancy. After sufficient time of mixing, the air exchange rates in the sampling locations were determined using the concentration decay method.Citation13 The air change rate inside the printing center was maintained at 1.63 ACH (air change per hour) during normal business hours.
Table 1. Operation hours, room dimensions, environmental conditions, and types of ventilation
Experimental Chamber
The experimental chamber (volume = 1 m3) was designed to study the emissions from a laser printer housed in a controlled environment. The chamber was constructed using galvanized steel. The inner walls of the chamber were polished to minimize the removal of airborne particles. The chamber consisted of inlet and outlet ducts for the exchange of air. A HEPA (high-efficiency particulate air) filter was placed at the air inlet to remove outdoor particulates entering the chamber. The laser printer (HP Laser Jet 4250n) was placed in the center of the chamber. A tiny inlet was provided for air sampling purposes directly above the printer.
Instrumentation
Fast mobility particle sizer (FMPS; model 3091; TSI Incorporated, Shoreview, MN, USA) was used for a continuous monitoring of submicrometer particles in the size range of 5.6–560 nm. Black carbon (BC) concentrations were measured using Aethalometer (AE-31; Magee Scientific, Berkeley, CA, USA) for every 2 minutes continuously throughout the sampling period. The sample air was drawn at a rate of 4.8 LPM (liters per minute), and was passed through a quartz filter tape through which a beam of light with different frequencies passed and reached the photodiode detector. The instrument calculated the rate of increase of attenuation (ATN), which was linearly proportional to the loading of aerosol black carbon. As the loading of the filter increased, existing particles on the filter gradually reduced the accuracy in the analytical detection of BC concentrations. Corrections were made to the data using the “Virkkula” modelCitation14 to obtain real BC concentrations. Stainless steel, passivated air canisters were used for the VOC sampling in the printing center. The canisters were then transported to a central laboratory at the University of California, Irvine, and were analyzed for VOCs using gas chromatography (GC) equipped with mass spectrometry (MS), electron capture detector (ECD), and flame ionization detector (FID) detectors. Further details of VOCs analysis are given elsewhere.Citation15
Sampling Procedure
Field experiments were conducted for a period of 1 month. A continuous monitoring of particle number, mass, and BC concentrations was done during both operating and nonoperating hours of the printing center. Air sampling was done at a height of 1.5 m from the ground (nose levels of an average human being), directly above the printers. During the sampling period, the printing center was open for normal business, with students moving in and out of the room for using laser printers. Measurements were also taken simultaneously at a distance of 4.5 m away, at the opposite end from where the printers were located, at the same height using an additional FMPS for a week.
Air samples were collected in the middle of the room for the analysis of VOCs by using air canisters. The samples were collected three times a day: (a) before the opening of the center (i.e., prior to printing or idling mode, at 06:00 a.m.); (b) when the center was just opened for students (the start of the printing activity, 09:00 a.m.); and (c) during the heavy usage of printers (peak printing, 12:00 p.m. to 03:00 p.m.), for two consecutive days.
For chamber experiments, background particle number concentrations inside the chamber were measured for 30 minutes prior to the start of the experiment after adjusting the flow rates of the compressed air and the exhaust fan in the chamber to attain the desired ACH. A standard format (ECMA-328) was used for printing on A4 size blank pages (80 g/m2). The printing rate for the printer was 45 pages per minute. A continuous monitoring of particle number concentrations and size distributions was conducted during the printing process and was continued after printing until the concentrations in the chamber reached their background levels. These experiments were repeated three times for consistency.
RESULTS AND DISCUSSION
VOCs in the Printing Center
Due to extreme temperatures of the fusion roller (∼200 °C), laser printers emit a considerable amount of VOCs than any other types of printers.Citation6,Citation7 In order to examine and assess the contribution of laser printers to VOCs in the indoor air of the printing center, air samples were collected during three operating conditions of the printing center typically encountered in a day (before the printing center was open: 07:30 a.m.; when the printing center was just open: 09:00 a.m.; and during peak printing hours: 12:00 p.m.). presents the average concentrations of 15 selected VOCs. As shown in the table, compounds such as freons, methyl chloride, carbon tetrachloride, and 1,1,1-trichloroethane did not show any significant variation in their concentration levels during the different operating conditions of the printing center. This observation indicates that these compounds were not emitted within the printing center, but were likely transported from outdoor air into the printing center through the ventilation system. Concentrations of ethylbenzene, xylene (o-, m-, p-), isoprene, and styrene associated with the toner were higher than those in the background levels during peak printing hours. Unlike the environmental chamber where the only emissions source is the printer, in the printing center there are many other emissions sources for VOCs. VOCs such as α- and β-pinene, (associated with paints, wall coatings) and methylene chloride (associated with adhesives, tapes, etc.) were also found in significant levels in the printing center. It should be noted that although toluene was present in higher levels before the printing center was open, its concentration decreased during peak printing hours. Similarly, styrene was also found to decrease during peak printing hours. This reduction in their concentrations is probably because of the release of ozone during peak hours. Ozone concentrations were not measured in the printing center. However, it is reported by Lee et al.Citation6 that laser printers on an average emit ozone in the range of 1.2–1.0 μg/copy, which are much higher compared to emissions from other printer types such as inkjet printer (0.05 μg/copy). This observation is supported by Kagi et al.Citation7 who found that ozone levels increased by nearly 4 times from the background levels during the course of printing activity (from 1.5 to 6 ppb). It is therefore reasonable to assume that ozone emitted from laser printers would react with VOCs to form secondary aerosol particles. Benzaldehyde, formed due to toluene reacting with ozone, and styrene are some VOCs that have relatively high potential to form secondary organic aerosol than other VOCs.Citation16 Also, a rise in nuclei-mode particles and lowest peak diameter () was observed around the same time (peak printing hours: 12:00 p.m.). We hypothesize that the decrease in the concentrations of toluene and styrene during peak hours was due to new particle formation. More research is needed to verify this hypothesis.
Table 2. Mean and standard deviations of VOCs (μg/m3) measured inside the printing center during different operating modes of printers
Number Concentrations and Size Distributions in the Printing Center
A statistical summary of the characteristics of submicrometer-sized particles for three size ranges (5.6–50, 50–100, and 100–560 nm), as measured in the printing center during operating and nonoperating hours, are shown in for the entire sampling period. The changes in the particle number concentrations for all three size ranges during operating and nonoperating hours were analyzed statistically using analysis of variance (ANOVA), two factors with replication. UFPs in the size range of 5.6–100 nm showed a significant increase (P < 0.01) during operating hours and accounted for about 87% and 82% of the total submicrometer particles for weekdays and weekends in the printing center, respectively. During operating hours, the number concentration of nuclei-mode particles (5.6–50 nm) increased by a factor of 2.7 and 1.8 during weekdays and weekends, respectively, when compared to those measured during nonoperating hours. On the other hand, particles in the size range of 100–560 nm showed an insignificant increase (P > 0.1) in their concentrations during operating hours. The observed increase in the particles with the nuclei mode and UFPs during operating hours suggests that those particles were freshly emitted due to the intense printing activity. On an average, a total of 5000∼9000 pages were printed daily in weekdays and 1000–2000 pages on weekends during operating hours. Smoking was not allowed inside and even in the vicinity of the printing center. In the absence of smoking activity, other human activities such as the movement of users of the printing facility are not likely to have any major influence on the number concentration of particles with aerodynamic diameter less than 1 μm.Citation17 Therefore, the only major source of particles other than the resuspension of the settled particles and the ventilation blown dust is the release of particles from laser printers. As can be seen in , the variation of particle number concentrations (as represented by the standard deviation) was high for UFPs (5.6–100 nm) during operating hours. This high variation could be due to variations in the intensity of printing activity during operating hours (5000∼9000 pages in weekdays and 1000–2000 pages on weekends). The little variation observed in the number concentration of 100–560 nm particles suggests that these particles were less dependent on the printing activity. These results indicate that the large counts of UFPs found in the indoor air of the printing center were mainly due to the printing activity. A previous study conducted in an office environment also observed an increase in the concentration of UFPs released during operating hours due to printing activity.Citation7
Table 3. Statistical summary of particle number concentrations during operating and nonoperating hours
shows particle size distributions in the printing center as measured at different times in a day. As shown in the figure, the smallest peak diameter (∼50 nm) was observed around 12:00 p.m., and the largest (100 nm) at 06:00 a.m., before the center was open for business. The activity in the printing center was high in the morning session (09:00 a.m. to 12:00 p.m.) than in the afternoon and decreased drastically after school hours (06:00 p.m.). The smallest peak diameter observed at 12:00 p.m. corresponds to the peak printing period, indicating that large numbers of UFPs were released during printing activity. The peak diameter increased slightly after 12:00 p.m., with reduction in the printing activity, and after 06:00 p.m., the peak concentrations decreased drastically.
Due to the routine maintenance activities at the condenser side of the air conditioning unit, which was located outside the printing center, one of the air conditioning systems was shut down for 1 day. The air change rate was reduced, by about 35% (ACH ∼1.04 hr−1) until 06:00 p.m. Particle size distributions at the reduced ACH (shown in ) exhibited a bimodal distribution, which was contrary to the unimodal size distribution observed during normal air change rates. The nuclei-mode particles (diameter <50 nm) increased nearly 3 times as compared to that of normal air change rates and their percentage contribution increased from 44% during normal conditions to 61% of the total submicrometer particles concentration. From , it can be observed that when some of the air conditioning units were shut down at 09:00 a.m., an additional peak with diameter between 10 and 20 nm appeared in the particle size distributions. The concentration of these particles continued to increase till late afternoon and then started decreasing. The influence of human activity on these changes can be ruled out because it has little or no effect on the concentration of UFPs.Citation17 Instead, this variation could be attributed to the nucleation of emitted VOCs. The smaller-diameter particles observed in the size range of 10–20 nm could be formed freshly due to the reaction of ozone with the emitted VOCs.Citation18 The formation of the secondary peak and its rise cannot be solely due to the printing activity, as evident from . Although printing activity was high at 12:00 p.m. compared to other times, the particle distribution was still unimodal similar to other time points. Therefore, the secondary peak observed might be due to the reduced air exchange rate, under which condition the precursors of new particles have a longer residence time and therefore undergo gas-to-particle conversion. When the air change rate was in the normal range with all air conditioning units being functional, the emitted VOCs and ozone were likely to be well-dispersed and diluted or vented out. As a consequence, there will not be sufficient time for the reaction between the precursors to take place and go to completion. However, when there was a reduction in the air change rate, there was an increase in the residence time of the contaminants such as VOCs and O3 emitted from the printers and also the room temperature rose up to 29.3 °C as compared to normal average room temperature of 22 °C. Under these favorable conditions, it is likely that there was an increase in the rate of vapor-to-particles formation, that is, secondary aerosol formation.Citation19 Similar results were reported recently by Fadeyi et al.Citation18 and also by Zurami et al.Citation20 in their study on secondary organic aerosol formation in indoor environments. They found that when recirculation rates were decreased in their environmental chambers, particle number concentrations in the size range of 20–30 nm were increased. When all the air-conditioning units were back in operation at 06:00 p.m., the particle size distribution showed a drop in the number concentration and returned to the unimodal size distribution. During normal ventilation rates, the maximum total submicrometer particle concentration was observed around 12:00 p.m., when the printing activity was high. However, with reduced recirculation rates, the concentration increased till 03:00 p.m. when it reached the maximum values.
Figure 3. Particle size distributions measured in the printing center at reduced recirculation rates.
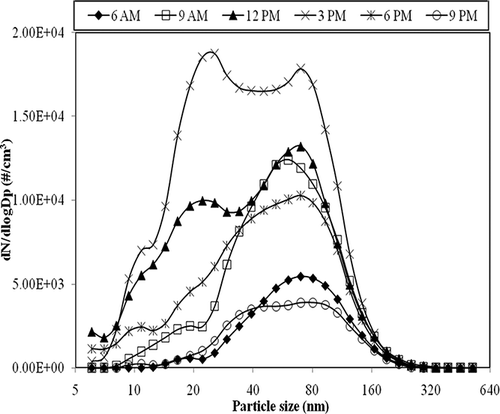
To gain a better understanding of the exposure levels of occupants within the room, particle number concentrations were also monitored at a distance of 4.5 m away from the printers. The height at which measurements were made was maintained at 1.5 m. As shown in , the fraction of particles in the size range of 5.6–50 nm increased to 50% of total submicrometer particle concentration at the sampling point away from the printer, whereas it was 38% at a point near the printer. The standard deviation values indicated that the variation of the particle number concentrations near the printer was greater compared to that at the distant sampling point. In the immediate vicinity of the printers, the particle number concentrations were affected by the intensity of printing activities; the particle number concentrations were high when there was more printing activity and were low when there was no activity. However, at the distant sampling point away from the printer, the particles tend to be dispersed efficiently and be distributed uniformly, thereby reducing the variations in the particle number concentrations.
Table 4. Statistical summary of particle number concentrations measured near and away from printers
shows particle size distributions at the distant sampling point (4.5 m away from the printers) in the printing center during different times in a day. Unlike the particle size distributions shown in with the peak diameter above 50 nm, the peak diameters at time points 09:00 a.m. to 12:00 p.m. were close to or less than 40 nm, as shown in . For size distribution profiles at time points 03:00 p.m. to 09:00 p.m., the peak diameters were greater than 40 nm. However, for all the other time points the peak diameters were significantly lower compared to their respective peak diameters near the printer, with an exception of that at 09:00 p.m. At 09:00 p.m. the size distribution profile was similar to that observed near the printer. Also, the particle concentrations in the range of 5.6–20 nm were considerably higher as compared to those observed near the printer. The VOCs emitted from the printer could nucleate during the course of their transport, resulting in the new particle formation in the nuclei-mode range and was responsible for the shift in the size distribution. A delayed response in the peak particle concentration was observed, presumably due to particle agglomeration. The peak concentration was observed only at 03:00 p.m., whereas near the printer, the peak concentration was observed around 12:00 p.m. These observations suggest that the occupants of the printing center were exposed to UFPs formed from reaction between ozone (O3) and VOCs emitted during printing apart from the immediate users of the printers.
BC concentrations were also monitored during the study period. The correlations between hourly average particle number concentrations in the three size ranges (5.6–50, 50–100, 100–560 nm) and BC concentrations are shown in . Spearman rank order correlation coefficients (r S) were calculated. As shown in and b, there was no significant correlation between BC concentration and UFPs in the size range of 5.6–50 nm (r S = −0.19) and a modest correlation in the size range of 50–100 nm (r S = 0.33) at P = 0.05. However, there was a strong correlation with fine particles in the size range of 100–560 nm (r S = 0.82), as shown in . Although the chemical analysis of airborne particles emitted from printers remains to be done to understand the chemical nature of the particles, it appears that the UFPs were not primarily released from the toner dust and represent secondary particle formation from emitted VOCs. UFPs released from the toner dust, which are primarily made of BC, should show a good correlation with BC concentrations measured simultaneously, which was not observed. Also, the observed increase in UFP concentrations during peak hours and at reduced recirculation rates when VOCs and ozone concentrations were high supports the idea that UFPs might not represent primary emission from the toner dust.
Particles Number Concentrations and Size Distributions in the Chamber
A complementary study in a custom-designed chamber was conducted to confirm the trends observed in the printing center. shows the particle size distribution inside the chamber during printing at different air change rates. While the air change per hour (ACH) was maintained at 1, particle distributions showed a peak at 70 nm. However, as the air flow rate was decreased to 0.6 and subsequently to 0.4 ACH, the peak shifted towards smaller diameters in the range of 34–52 nm. An additional peak at the diameter around 16 nm was also found with the decrease in ACH. The trends observed in the chamber at reduced ACH are very similar to those observed in the printing center when the recirculation rate was reduced due to the routine maintenance of air-conditioning units. UFPs accounted for approximately 85% at 1 ACH, which increased to 95% and 97% of the total submicrometer particle number concentrations in the printing chamber when the air circulation rate was reduced to 0.6 and 0.4 ACH, respectively. These results suggest that the number of UFPs released is not only dependent on the printer type and age as reported elsewhere,Citation6,Citation7 but also on the indoor room conditions. Reduced or poor ventilation in the printing center can exacerbate the IAQ problems associated with the release of UFPs from printers.
Figure 6. Particle size distribution measured at different air exchange rates in the controlled chamber.
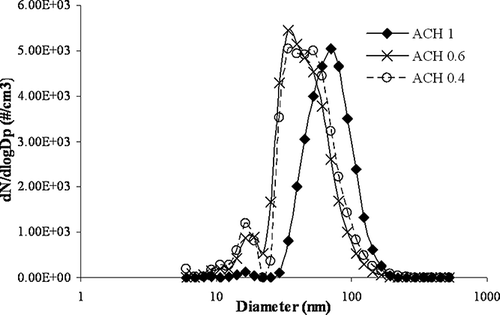
Variations of particle number concentrations with the total number of pages printed in the chamber and in the printing center are presented in . It was observed that with the increase in number of pages printed, the particle number concentrations increased correspondingly in the chamber study, whereas in the printing center the variation of particle number concentration was erratic and did not follow any particular trend. This is because the chamber studies were conducted in a controlled environment and the background concentrations remained the same for all experiments. However, in the case of the printing center, the background concentration varied constantly due to continuous printing operations, fluctuations in the ventilation flow rates, and movement of people in and out of the printing center. In addition, the controlled chamber experiments had a standardized printing pattern such as ECMA-328, whereas the printing patterns changed for almost every single page in the printing center. All these factors mask the true effects of the number of pages printed on the particle emissions in the printing center.
CONCLUSIONS
In this study, the indoor air of a commercial printing center was investigated for submicrometer particles and VOCs. VOCs such as freons, carbon tetrachloride, methyl chloride, 1,1,1-trichloroethane did not show any variation in their concentrations during the entire sampling period, indicating that these compounds were not emitted indoors. Compounds such as styrene, ethylbenzene, and o, m, and p-xylene were high during peak printing hours than other times of the day. However, some VOCs (toluene, benzene, and styrene) showed lower concentration levels during peak printing hours than during nonoperating hours. Particle number concentrations, especially those of UFPs, increased during peak printing activity and were dependent on indoor conditions such as air change rates. At lower ACH values, nuclei-mode particles (diameter <50 nm) increased several times and contributed to a large fraction of total submicrometer particles (∼60%). These particles were likely formed from the reactions between ozone and VOCs emitted from printers. Also, it was found that the particle peak diameters measured at a distant point away from printers were smaller when compared to those in the immediate vicinity of printers. Based on correlation observed between black carbon and UFPs (r S = −0.19 for nuclei-mode particles and r S = 0.33 for 50–100-nm particles), we suggest that the direct release of UFPs from the toner cartridge was unlikely, because the toner particles were primarily made up of carbon black. Instead, it is likely that UFPs represent secondary aerosols that might have resulted from the ozone-induced oxidation of VOCs emitted during the printing activity.
REFERENCES
- Destaillats , H. , Maddalena , R.L. , Singer , B.C. , Hodgson , A.T. and McKone , T.E. 2008 . Indoor Pollutants Emitted by Office Equipment: A Review of Reported Data and Information Needs . Atmos. Environ. , 42 : 1371 – 1388 .
- Brown , S.K. Pollutant Emission Properties of Photocopiers and Laser Printers . 8th International Conference on Indoor Air Quality and Climate . August 8-13 1999 , Edinburgh , Scotland. pp. 123 – 128 . CA , , USA : International Society of Indoor Air quality and Climate: Santa Cruz .
- Bake , D. and Moriske , H.J. 2006 . Investigations about Fine and Ultrafine Particles by Using Laser Printers . Unweltmed. Forsch. Prax. , 11 : 301 – 308 .
- Uhde , E. , He , C. and Wensing , M. Characterization of Ultra-fine Particle Emissions from a Laser Printer . Proccedings of International Conference on Healthy Buildings, (Vol II) . June 4-8 2006 , Lisbon , Portugal. pp. 479 – 482 . CA , , USA : International Society of Indoor Air quality and Climate: Santa Cruz .
- Wolkoff , P. , Wilkins , C.K. , Clausen , P.A. and Larsen , K. 1993 . Comparison of Volatile Organic Compounds from Processed Paper and Toners from Office Copiers and Printers: Methods, Emission Rates, and Modeled Concentrations . Indoor Air. , 3 : 113 – 123 .
- Lee , S.C. , Lam , S. and Fai , H.K. 2001 . Characterization of VOCs, Ozone, and PM10 Emissions from Office Equipment in an Environmental Chamber . Build. Environ , 36 : 837 – 842 .
- Kagi , N. , Fujii , S. , Horiba , Y. , Namiki , N. , Ohtani , Y. , Emi , H. , Tamura , H. and Kim , Y.S. 2007 . Indoor Air Quality for Chemical and Ultrafine Particle Contaminants from Printers . Build. Environ. , 42 : 1949 – 1954 .
- Schripp , T. , Wensing , M. , Uhde , E. , Salthammer , T. , He , C. and Morawska , L. 2008 . Evaluation of Ultrafine Particle Emissions from Laser Printers Using Emission Test Chambers . Environ Sci Technol. , 42 : 4338 – 4343 .
- He , C. , Morawska , L. and Taplin , L. 2007 . Particle Emission Characteristics of Office Printers . Environ Sci Technol. , 41 : 6039 – 6045 .
- Wensing , M. , Schripp , T. , Uhde , E. and Salthammer , T. 2008 . Ultra-fine Particles Release from Hardcopy Devices: Sources, Real-Room Measurements and Efficiency of Filter Accessories . Sci. Total Environ. , 407 : 418 – 427 .
- Luoma , M. and Batterman , S.A. 2001 . Characterization of Particulate Emissions from Occupant Activities in Offices . Indoor Air. , 11 : 35 – 48 .
- Dufresne , A. , Krier , G. , Muller , J.F. , Case , B. and Perrault , G. 1994 . Lanthanide Particles in the Lung of a Printer . Sci. Total Environ. , 151 : 249 – 252 .
- Lagus , P. and Persily , A. 1985 . A Review of Tracer Gas Techniques for Measuring Airflows in the Buildings . ASHRAE Trans. , 91 : 1075 – 81 .
- Hansen , A.D.A. , Turner , J.R. and Allen , G.A. An Algorithm to Compensate Aethalometer™ Data for the Effects of Optical Shadowing and Scattering . Presented at 5th Asian Aerosol Conference . August 26-29 2007 , Kaohsiung , Taiwan. Mumbai , , India : Indian Aerosol Science and Technology Association .
- Colman , J.J. , Swanson , A.L. , Meinardi , S. , Sive , B.C. , Blake , D.R. and Rowland , F.S. 2001 . Description of the Analysis of a Wide Range of Volatile Organic Compounds in Whole Air Samples Collected during PEM-Tropics A and B . Analy. Chem. , 73 : 3723 – 3731 .
- Derwent , D. , Jenkin , M. , Utembe , S. and Passant , N. International Aerosol Modeling Algorithms, xx . Secondary Organic Aerosol (SOA) Formation from a Large Number of Reactive Organic Compounds under European Conditions . December 9-11 2009 , CA . December 9-11 . Sacramento , CA : Davis, CA . California Rental Association, 2009
- Luoma , M. and Battersman , S.A. 2001 . Characterization of Particulate Emissions from Occupant Activities in Offices . Indoor Air. , 11 : 35 – 48 .
- Fadeyi , M.O. , Weschler , C.J. and Tham , K.W. 2009 . The Impact of Recirculation, Ventilation and Filters on Secondary Organic Aerosols Generated by Indoor Chemistry . Atmos. Environ. , 43 : 3538 – 3547 .
- Artelt , C. , Schmid , H.J. and Peukert , W. 2005 . On the Impact of Accessible Surface and Surface Energy on Particle Formation and Growth from the Vapour Phase . J. Aerosol. Sci. , 36 : 147 – 172 .
- Zuraimi , M.S. , Weschler , C.J. , Tham , K.W. and Fadeyi , M.O. 2007 . The Impact of Building Recirculation Rates on Secondary Organic Aerosols Generated by Indoor Chemistry . Atmos. Environ. , 41 : 5213 – 5223 .