ABSTRACT
Stationary diesel engines, especially diesel generators, are increasingly being used in both developing countries and developed countries because of increased power demand. Emissions from such engines can have adverse effects on the environment and public health. In this study, particulate emissions from a domestic stationary diesel generator running on ultra-low-sulfur diesel (ULSD) and biodiesel derived from waste cooking oil were characterized for different load conditions. Results indicated a reduction in particulate matter (PM) mass and number emissions while switching diesel to biodiesel. With increase in engine load, it was observed that particle mass increased, although total particle counts decreased for all the fuels. The reduction in total number concentration at higher loads was, however, dependent on percentage of biodiesel in the diesel-biodiesel blend. For pure biodiesel (B100), the reduction in PM emissions for full load compared to idle mode was around 9%, whereas for ULSD the reduction was 26%. A large fraction of ultrafine particles (UFPs) was found in the emissions from biodiesel compared to ULSD. Nearly 90% of total particle concentration in biodiesel emissions comprised ultrafine particles. Particle peak diameter shifted from a smaller to a lower diameter with increase in biodiesel percentage in the fuel mixture.
There has been an increased usage of stationary diesel engines, especially backup power generators to meet the growing energy demand. Biodiesel derived from waste cooking oil has received increasing attention as an alternative fuel. However, data are only sparsely available in the literature on particulate emissions from stationary engines, fueled with blends of diesel and biodiesel. This study provides insights into the influence of waste-cooking-oil-derived biodiesel on engine performance and the particulate emissions from a stationary engine. The results of the study form a scientific basis to evaluate the impact of biodiesel emissions on the environment and human health.
INTRODUCTION
Emissions of airborne particulate matter (PM) from diesel engines have been of great concern globally because of their close association with adverse effects on human healthCitation1–3 and the environment.Citation4 Most of the previous research on diesel PM characterization has been mainly focused on vehicle exhaust in order to improve urban air quality.Citation5–9 However, very little attention has been paid towards the PM emissions from off-road diesel engines until recent times when the U.S. Environmental Protection Agency (EPA) finalized and adopted emission regulatory standards for off-roadCitation10 and stationaryCitation11 engines. Although these engines might be smaller in number when compared to on-road vehicles, PM emissions from them can account for a significant fraction of total diesel PM due to the less advanced control of their emissions. For example, it has been reported that off-road diesel engines account for 47% of mobile-source fine particles (PM of aerodynamic diameter ≤2.5 μm [PM2.5]) in the United States and this number is estimated to increase to 70% by 2020 if regulatory measures are not undertaken.Citation10 Moreover, increased usage of stationary engines such as backup power generators to meet growing energy requirements can only make the current situation worse.Citation12,Citation13 This scenario is especially applicable to fast-growing and developing countries due to the lack of regulatory standards for off-road and stationary diesel engines.
In order to reduce PM emissions from diesel engines and to meet the future energy requirements, biodiesel is considered as a potential alternative to diesel fuel.Citation14,Citation15 Several studies have been conducted to assess the environmental benefits associated with the use of biodiesel as a replacement to diesel fuel.Citation9,Citation16–22 Most of these studies used heavy- and light-duty vehicles for their emission studies. The operation mode of stationary engines such as backup generators is distinct from that of automobiles, because they operate near steady state conditions at nearly constant rpm and load.Citation23 Therefore, emission characteristics of PM from such engines can be significantly different from those of on-road vehicles. Moreover, the emission profiles of ultrafine particles (UFPs; aerodynamic diameter ≤100 nm) from diesel generators remain largely unknown. UFPs contribute a small fraction to the mass concentration of ambient aerosol particles, but may contribute disproportionately to their toxicity because of their high number concentration and surface area, high deposition efficiency in the pulmonary region, and high propensity to penetrate the epithelium.Citation24
It is well known that the quality of the exhaust from diesel generator is dependent on the type and quality of feedstock used for biodiesel production. Among the different types of biodiesel, the one made by the transesterification of waste cooking oil has received attention as a replacement for diesel fuel because of its lower cost and the added advantage of reducing waste oil disposal.Citation25–27 The evaluation of a diesel generator fueled by waste-cooking-oil-derived biodiesel indicated an increase in engine performance by nearly 10% when compared to ultra-low-sulfur diesel (ULSD), but lower power generation.Citation25 However, the PM emission profile was not provided in that study, because the focus was mainly on studying engine performance and power generation. Data available in the literature on PM emissions from stationary engines, fueled with blends of ULSD and biodiesel, are relatively sparse.Citation28–30 Durbin et al.Citation31 in their study of exhaust emissions from various on/off-road applications found inconsistencies in trends of PM and other exhaust emissions between on-road and stationary engines. They observed a significant reduction of PM emissions for different types of biodiesel in comparison to ULSD in heavy-duty vehicles, whereas stationary backup generators showed a slight reduction and sometimes even an increase in their PM emissions. Recently, Chung et al.Citation30 measured particle size distributions from a diesel generator at different loads. This study revealed that at idle mode, particles emitted from biodiesel combustion have a mobility peak diameter nearly 2 times that of diesel particles, but at higher loads a reverse trend was observed. With the emerging growth in the use of backup diesel generators, it is important to understand and characterize their PM emissions from ULSD and biodiesel blends to develop and implement subsequent control measures.
In this study, the physical characteristics of PM emitted from a stationary diesel generator, fueled by blends of ULSD and waste-cooking-oil-derived biodiesel, were evaluated. PM emissions from a commonly available domestic diesel generator were sampled through a dilution system. Particle mass and size-differentiated number concentrations were measured for different loads and for different blends of biodiesel. The engine performance for different blends was also evaluated and is discussed in this article.
EXPERIMENTAL
Test Engine and Fuel Properties
Experiments were carried out on a single-cylinder, four-stroke, air-cooled, direct fuel injection, diesel backup power generator (L70AE; Yanmar Corp, China) that is meant for domestic use. The specifications of the engine are given in . The engine was within its first 100 hr of operation.
Table 1. Engine specifications
The fuels used in this study include ULSD and biodiesel derived from local waste cooking oil generated in Singapore. Blends of ULSD and biodiesel, B20 (20% biodiesel) and B50 (50% biodiesel), were prepared by mixing the two base fuels in volumetric fractions. Density, viscosity, and other fuel properties of the ULSD and biodiesel are shown in . Fuel properties for blends were calculated based on the mass fraction of each component in the blended fuel wherever applicable.
Table 2. Characteristics of fuels
Experimental Setup and Procedures
shows the schematic of the experimental system used in this study. Engine exhaust was sampled through a sampling probe (DI-1019; Dekati Ltd.) inserted into the main exhaust stream. The exhaust was then directed into a dilution tunnel, where the hot exhaust was mixed with a stream of pressurized, particle-free, dry air in two stages using Dekati diluters (DI-2000; Dekati). In the first stage, the exhaust was transferred into the primary dilution tunnel, where the hot exhaust is mixed with a stream of pressurized (∼2 bar) particle-free dry air preheated to a temperature of 200 °C, close to the temperature of the exhaust, to avoid particle nucleation, condensation, etc. The primary dilution tunnel was also heated to a temperature close to 200 °C to avoid thermophoretic deposition of particles onto the walls of the dilution tunnel. In stage 2, some portion of the diluted exhaust from the primary dilution tunnel was transferred to the secondary dilution tunnel. During the primary dilution, vapor pressures of volatile compounds decreased, allowing the secondary dilution with cold dilution air without condensing the volatile components. At this stage, the exhaust was mixed with dilution air at ambient temperature (∼35 °C) to bring down the temperature of the hot exhaust to ambient temperature for sampling. The completely diluted exhaust was then directed to particle measuring instruments. The dilution ratio was calculated by measuring the CO2 concentrations in the raw exhaust, dilution air and diluted exhaust. The dilution ratio was found to be in the range of 59–64. A fast-mobility particle sizer (FMPS; model 3091; TSI Incorporated) was used to measure the particle size distribution and number concentrations in the diluted exhaust. The number concentration measurements were then corrected based on the dilution ratio. Aerosol particles were collected on 47-mm Teflon membrane (Pall Corporation Inc., USA) and on precombusted 47-mm quartz fiber filters (Whatman, USA) using air samplers (Minivol; Air Metrics Ltd.) equipped with a cyclone (cut size = 2.5 µm). The exhaust was drawn at flow rate of 8 L/min for 20 min onto the filters. These filters were conditioned in a dry box at a constant relative humidity (RH) and temperature (T) (T = 22 °C and RH = 32%) for 24 hr prior to weighing in a microbalance (Sartorius, MC 5). Gravimetric mass concentrations of PM2.5 were obtained by dividing the mass of particles collected onto the filter by the volume of the exhaust sampled through the filters.
Exhaust emission experiments were carried out at steady state at rated rpm (see ) and four different engine loads. Before changing the fuel, exhaust sampling lines and dilution tunnels were cleaned with acetone to remove the soot deposited onto the walls. After adding the fuel, the engine was warmed up for 1 hr to purge any other sampling artifacts. For every increase in load, the engine was warmed up for 10 min. Brake-specific fuel consumption (BSFC) and brake thermal efficiency (BTE) were calculated by measuring the fuel consumption rate of the engine during each test condition using a fuel measuring gauge and a stop watch. The exhaust was then measured for particle number concentrations and size distributions using the FMPS. The diesel generator was operated at four different load conditions: idle, 30%, 70%, and 100% of maximum output power. The load onto the generator was applied using three different electric pumps (0.4, 0.5, and 2.1 kW). The pumps were operated in combinations to apply the load of 0.9, 2.1, and 3 kW onto the generator which corresponded to 30%, 70%, and full load on the generator. The emission experiments were carried out using ULSD, biodiesel, and their blends for each load.
RESULTS AND DISCUSSION
Engine Performance
Brake-specific fuel consumption (BSFC) and brake thermal efficiency (BTE) are both commonly used parameters for evaluating engine performance. These parameters can be calculated by measuring the volumetric fuel consumption rate and power generated by the engine. BSFC, the ratio of fuel mass consumption to output power, and BTE, the ratio of output energy to input energy, were calculated at each load for different fuel blends and are shown in and b.
Figure 2. Effect of different fuels on (a) brake-specific fuel consumption and (b) brake thermal efficiency of engine.
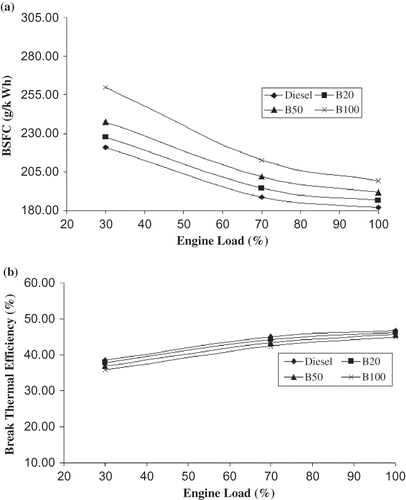
As shown in , for a given load, BSFC was higher for biodiesel and biodiesel blends when compared to ULSD. The higher fuel consumption of biodiesel was due to its lower heating value. The loss of heating value while using biodiesel was compensated by higher fuel consumption by the engine in order to generate the same power as for the ULSD. This higher fuel consumption may explain an increase in BSFC for biodiesel. BTE for biodiesel, on the other hand, was not significantly different from that for ULSD. As shown in , for lower loads, the BTE for biodiesel and its blends was only slightly lower than that of ULSD and the difference was not significant at higher loads.
Particulate Mass Concentration
Biodiesel and blends of ULSD–biodiesel have a significant effect on particulate emissions. PM2.5 (aerodynamic diameter [AED] ≤2.5 μm) concentrations for ULSD and biodiesel blends were evaluated gravimetrically, and are shown in . PM2.5 mass collected on filters ranged from 129 to 397 µg for various fuel samples. It was found that with an increase in percentage of biodiesel in the fuel mixture, particle mass was reduced by 16.3% for B20, 20.2% for B50, and 35.1% for pure biodiesel (B100) during idle mode and by 12.2% for B20, 26.9% for B50 and 36.6% for pure biodiesel (B100) at full load. A two-sample t test assuming unequal variances was performed to determine if the reduction in the PM concentration between ULSD, biodiesel, and blends of biodiesel was significant. The reduction of PM concentration observed for the case of B20 was not statistically significant (t stat < t critical) at all loading conditions while for B50 and B100 the PM reduction was significant (t stat > t critical at P value <0.05), with the exception at 70% load. For a particular fuel blend, the PM mass increased with load.
Lapuerta et al.Citation9 reported that many studies observed particulate matter reductions in the range of 40–70% when biodiesel was used. The percentage reduction of PM2.5 observed in this study (∼35%) is slightly lower than the range reported by Lapuerta et al.Citation9. This is mainly because most of the studies, as reported by Lapuerta et al.Citation9 in their review paper, compared biodiesel to conventional low-sulfur diesel (sulfur content <500 ppm), whereas in our study ULSD (sulfur content <15 ppm) was used. The PM emissions from ULSD were reduced by 9.2–56.6% when compared to conventional diesel.Citation32 Therefore, the percentage reduction in PM emissions observed with biodiesel in comparison to ULSD was relatively lower than those reported by Lapuerta et al.Citation9 The reduction in PM emissions when using biodiesel can be attributed to the following reasons: (1) the absence of aromatics,Citation17 which are considered as soot precursors, in biodiesel reduces the amount of PM formed during combustion; (2) the higher oxygen content in biodiesel tends to enhance the combustion process resulting in lower particulate emissions.
In addition, as shown in , the higher viscosity and density of biodiesel compared to ULSD can lead to an increase in the injection pressure. Likewise, higher bulk modulus of compressibility of vegetable oils and their methyl esters can lead to advanced injection timingCitation33 while using biodiesel. As a result, the biodiesel fuel enters the combustion chamber relatively quicker compared to ULSD.Citation9 This advancement in combustion process while using biodiesel increases the residence time of soot particles in the combustion chamber, and thus they undergo further oxidation,Citation34 leading to reduction in PM emissions.
Particle Number Concentrations and Size Distribution
A summary of particle number concentration of diesel and biodiesel emissions during different engine operating conditions is provided in and their size distributions are shown in –d. In contrast to PM mass emissions, particulate number concentrations decreased with an increase in load for all the fuels (shown in ). At higher biodiesel-ULSD blend ratios, the percentage decrease in particle number concentration with increasing load was relatively small compared to ULSD. For ULSD, total particle number concentration decreased by 26% at full load when compared to idle or no load conditions, whereas for biodiesel, it decreased by only around 9%.
Table 3. Total particle number concentrations for ULSD and biodiesel blends at various loads
Figure 4. Particle size distributions of emissions at different loads for (a) ULSD, (b) B20, (c) B50, and (d) B100.
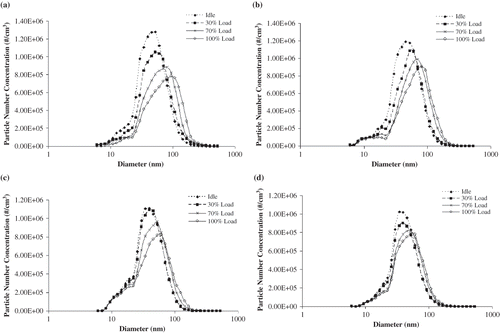
It is expected that in diesel engines, particle counts would increase with an increase in load. However, a decrease in particle number concentration with increasing load was observed in this study. Chung et al.Citation30 also observed a reduction in particle concentration at higher loads in their study using a Yanmar backup generator similar to the one used in this study. This observed decrease in particle number concentration was probably due to the transfer of particles from nucleation to accumulation or coarser modes at higher loads. It was observed that at higher loads, nucleation-mode particles, which contribute to a major fraction of total number, decreased and accumulation-mode particles increased. As a result, there was an overall decrease in total particle number concentration. This shift in particles from the nucleation mode to the accumulation mode was evident from the particle size distribution shown in –d. From the size distributions shown in , it can be observed that with the increase in load, particle peak diameters increased for all fuels. The magnitude of the increase in particle peak diameters at higher loads was greater for the ULSD than the biodiesel. For ULSD, the peak diameter increased from 52.3 nm at idle mode to 93 nm at full load and for biodiesel (B100) peak diameter increased from 34 nm at idle to 52.3 nm at full load.
In their study on particle emissions from stationary diesel engines, Di et al.Citation35 and Zhu et al.Citation36 reported an increase in total number concentrations when engine load was increased. Although there is no definitive explanation for the difference in particle emission trends observed by the above-mentioned studies and trends observed in this study as well as the study by Chung et al.,Citation30 a possible reason can be the differences in engine capacities and operating conditions. Both Di et al.Citation35 and Zhu et al.Citation36 conducted their studies on diesel engines which had much larger capacity and power (∼4000 cc and 88 kW). However, the stationary diesel generator used in this study (296 cc and 4.5 kW) and the one used by Chung et al.Citation30 (4.8 kW) have lower capacity and power. In addition, they are within their first 100 operating hours.
From , it can be observed that particle peak diameters shifted to lower size with an increase in the percentage of biodiesel in the fuel. A shift in the peak to lower diameters with the increase in percentage of biodiesel has been reported by several studies.Citation37,Citation38 This peak shift was likely due to a large reduction in particles with larger diameters as can be seen in . The reduction in the accumulation-mode particles was mainly due to the combustion advance in the case of biodiesel. As explained earlier, with an increase in the residence time of soot in the combustion chamber due to advancement in fuel injection for biodiesel, the soot generated through combustion can get further oxidized, thus reducing the particle size and thus the number of accumulation and fine particles.
shows the relative number percentage of nucleation-mode (diameter <50 nm), accumulation-mode (50–100 nm), and fine (diameter >100 nm) particles emitted from diesel and biodiesel (B100) fuels at various loads. Biodiesel had relatively a higher fraction of nucleation-mode particles, ranging from 55% to 70% at different loads when compared to diesel (35–60%). A decrease in the nucleation-mode particles (diameter <50 nm) and an increase in accumulation-mode and fine particles (diameter >100 nm) were observed with an increase in load for both the fuels. For the stationary engine running with ULSD at full load, nucleation-mode (32%), accumulation-mode (40%) and fine (28%) particles shared almost a similar fraction of particles to the total number concentration. However, for biodiesel, nucleation- (51%) and accumulation- (43%) mode particles were major contributors to the total number concentrations. The fraction of accumulation-mode particles increased from 30% during idle mode to 40% at full load in the case of ULSD, and a similar increase was observed for biodiesel as well (from 30% at idle mode to 43% at full load). This observation implies that diesel engines emit more accumulation particles at higher loads. At higher loads, more fuel is injected into the combustion chamber to generate additional torque needed and also the residence time for the particles in the combustion chamber decreases relatively. Therefore, the oxidation of particulate soot tends to be reduced, leading to the release of a large fraction of accumulation and fine particles. In the case of biodiesel, the inherent oxygen in the fuel improves the oxidation of soot. Therefore, the percentage increase of fine particles is relatively less for biodiesel.
CONCLUSION
Particulate emissions from a stationary diesel generator running on ULSD and biodiesel derived from waste cooking oil were investigated in this study. The engine performance of biodiesel measured in terms of BSFC and BTE showed that the fuel consumption was higher in case of biodiesel at almost the same combustion efficiency as with ULSD. Both particulate mass and number decreased at higher biodiesel–ULSD blend ratios. A large proportion of UFPs was observed in biodiesel than in ULSD. At higher engine loads, total particle counts decreased, which may be attributed to a large reduction in nucleation-mode particles.
REFERENCES
- 2002 . U.S. Environmental Protection Agency. Health Assessment Document for Diesel Engine Exhaust , U.S. EPA : Research Park Triangle, NC .
- Oberdorster , G. 2001 . Pulmonary Effects of Inhaled Ultrafine Particles . Int. Arch. Occup. Environ. Health. , 74 : 1 – 8 .
- Nel , A. 2005 . Air Pollution—Related Illness: Effects of Particles . Science. , 308 : 804 – 806 .
- Hansen , J. , Sato , M. , Ruedy , R. , Lacis , A. and Oinas , V. 2000 . Global Warming in the Twenty-First Century: An Alternative Scenario . Proc. Natl. Acad. Sci. U. S. A. , 97 : 9875 – 9880 .
- Maricq , M.M. 2007 . Chemical Characterization of Particulate Emissions from Diesel Engines: A Review . J. Aerosol. Sci. , 38 : 1079 – 1118 .
- Kittelson , D.B. 1998 . Engines and Nanoparticles: A Review . J. Aerosol. Sci. , 29 : 575 – 588 .
- Kleeman , M.J. , Schauer , J.J. and Cass , G.R. 2000 . Size and Composition Distribution of Fine Particulate Matter Emitted from Motor Vehicles . Environ. Sci. Technol. , 34 : 1132 – 1142 .
- Holmen , B.A. and Ayala , A. 2002 . Ultrafine PM Emissions from Natural Gas, Oxidation- Catalyst Diesel, and Particle-Trap Diesel Heavy-Duty Transit Buses . Environ. Sci. Technol. , 36 : 5041 – 5050 .
- Lapuerta , M. , Armas , O. and Rodriguez-Fernandez , J. 2008 . Effect of Biodiesel Fuels on Diesel Engine Emissions . Prog. Energy Combust. Sci. , 34 : 198 – 223 .
- 2004 . U.S. Environmental Protection Agency. Final Regulatory Analysis: Control of Emissions of Air Pollution from Nonroad Diesel Engines and Fuel; Code of Federal Regulations Part 9, Title 40, 69
- 2006 . Code of Federal Regulations Standards of performance for new stationary sources. Part 60, Title 40 Chapter I
- Ryan , N.E. , Larsen , K.M. and Black , P.C. 2002 . Smaller, Closer, Dirtier: Diesel Backup Generators in California , New York : Environmental Defense .
- Pistenmaa , C. , Amar , P. and J , Rodrick. T. 2003 . Stationary Diesel Engines in the Northeast: An Initial Assessment of the Regional Pollution, Control Technology Options and Air Quality Policy Issues , Boston , MA : NESCAUM .
- Murugesan , A. , Umarani , C. , Subramanian , R. and Nedunchezhian , N. 2009 . Bio-diesel as an Alternative Fuel for Diesel Engines—A Review . Renew. Sustain. Energy Rev. , 13 : 653 – 662 .
- Ramadhas , A.S. , Jayaraj , S. and Muraleedharan , C. 2004 . Use of Vegetable Oils as IC Engine Fuels—A Review . Renew. Energy , 29 : 727 – 742 .
- Garboski , M.S. , McCormick , R.L. , Alleman , T.L. and Herring , A.M. 2003 . The Effect Of Biodiesel Compostion on Engine Emissions from a DDC Series 60 Diesel Engine , Colorado , , USA : National Renewable Energy Laboratory .
- Knothe , G. , Sharp , C.A. and Ryan , T.W. 2006 . Exhaust Emissions of Biodiesel, Petrodiesel, Neat Methyl Esters, and Alkanes in a New Technology Engine . Energy Fuels , 20 : 403 – 408 .
- Turrio-Baldassarri , L. , Battistelli , C.L. , Conti , L. , Crebelli , R. , De Berardis , B. , Iamiceli , A.L. , Gambino , M. and Iannaccone , S. 2004 . Emission Comparison of Urban Bus Engine Fueled with Diesel Oil and 'Biodiesel' Blend . Sci. Total Environ. , 327 : 147 – 162 .
- Wang , W.G. , Lyons , D.W. , Clark , N.N. , Gautam , M. and Norton , P.M. 2000 . Emissions from Nine Heavy Trucks Fueled by Diesel and Biodiesel Blend without Engine Modification . Environ. Sci. Technol. , 34 : 933 – 939 .
- Durbin , T.D. , Collins , J.R. , Norbeck , J.M. and Smith , M.R. 2000 . Effects of Biodiesel, Biodiesel Blends, and a Synthetic Diesel Emissions from Light Heavy-Duty Diesel Vehicles . Environ. Sci. Technol. , 34 : 349 – 355 .
- Liu , Y.Y. , Lin , T.C. , Wang , Y.J. and Ho , W.L. 2009 . Carbonyl Compounds and Toxicity Assessments of Emissions from a Diesel Engine Running on Biodiesels . J. Air Waste Manage Assoc. , 59 : 163 – 171 . doi: 10.3155/1047-3289.59.2.163
- Frey , H.C. , Kim , K. , Pang , S.H. , Rasdorf , W.J. and Lewis , P. 2009 . Characterization of Real-World Activity, Fuel Use, and Emissions for Selected Motor Graders Fueled with Petroleum Diesel and B20 Biodiesel . J. Air Waste Manage Assoc. , 58 : 1274 – 1287 . doi: 10.3155/1047-3289.58.10.1274
- Shah , S.D. , Cocker , D.R. , Johnson , K.C. , Lee , J.M. , Soriano , B.L. and Miller , J.W. 2006 . Emissions of Regulated Pollutant from in Use Diesel Back-Up Generators . Atmos. Environ. , 40 : 4199 – 4209 .
- Donaldson , K. , Stone , V. , Gilmour , P.V. , Brown , D.M. and MacNee , W. 2000 . Ultrafine Particles: Mechanisms of Lung Injury . Phil. Trans. R. Soc. A , 358 : 2741 – 2750 .
- Cetinkaya , M. and Karaosmanoglu , F. 2005 . A New Application Area for Used Cooking Oil Originated Biodiesel: Generators . Energy Fuels , 19 : 645 – 652 .
- Zhang , Y. , Dube , M.A. , McLean , D.D. and Kates , M. 2003 . Biodiesel Production from Waste Cooking Oil: 2. Economic Assessment and Sensitivity Analysis . Bioresour. Technol. , 90 : 229 – 240 .
- Kulkarni , M.G. and Dalai , A.K. 2006 . Waste Cooking Oil—An Economical Source for Biodiesel: A Review . Ind. Eng. Chem. Res. , 45 : 2901 – 2913 .
- Kalligeros , S. , Zannikos , F. , Stournas , S. , Lois , E. , Anastopoulos , G. , Ch , Teas and Sakellapoulos , F. 2003 . An Investigation of Using Biodiesel/Marine Diesel Blends on the Performance of a Stationary Diesel Engine . Biomass Bioenergy , 24 : 141 – 149 .
- Lin , Y.C. , Lee , W.J. , Wu , T.S. and Wang , C.T. 2006 . Comparison of PAH and Regulated Harmful Matter Emissions from Biodiesel Blends and Paraffinic Fuel Blends on Engine Accumulated Mileage Test . Fuel , 85 : 2516 – 2523 .
- Chung , A. , Lall , A.A. and Paulson , S.E. 2008 . Particulate Emissions by a Small Non-Road Diesel Engine: Biodiesel and Diesel Characterization and Mass Measurements Using the Extended Idealized Aggregates Theory . Atmos. Environ. , 42 : 2129 – 2140 .
- Durbin , T.D. , Cocker , D.R. , Sawant , A.A. , Johnson , K. , Miller , J.W. , Holden , B.B. , Helgeson , N.L. and Jack , J.A. 2007 . Regulated Emissions from Biodiesel Fuels from On/Off-Road Applications . Atmos. Environ. , 41 : 5647 – 5658 .
- Zhang , J. , He , K. , Ge , Y. and Shi , X. 2009 . Influence of Fuel Sulfur on the Characterization of PM10 from a Diesel Engine . Fuel , 88 : 504 – 510 .
- Boehman , L.A. , Morris , D. and Szybist , J. 2004 . The Impact of Bulk Modulus of Diesel Fuels on Fuel Injection Timing . Energy Fuel , 18 : 1877 – 1882 .
- Cardone , M. , Prati , M.V. , Rocco , V. , Seggiani , M. , Senatore , A. and Vitolo , S. 2002 . Brassica carinata as an Alternative Oil Crop for the Production of Biodiesel in Italy: Engine Performance and Regulated and Unregulated Exhaust Emissions . Environ. Sci. Technol. , 36 : 4656 – 4662 .
- Di , Y. , Cheung , C.S. and Huang , Z.H. 2010 . Experimental Investigation of Particulate Emissions from a Diesel Engine Fueled with Ultralow-Sulfur Diesel Fuel Blended With Diglyme . Atmos. Environ. , 44 : 55 – 63 .
- Zhu , L. , Zhang , W.G. , Liu , W. and Huang , Z. 2010 . Experimental Study on Particulate And NOx Emissions of a Diesel Engine Fueled with Ultra Low Sulfur Diesel, RME-Diesel Blends and PME-Diesel Blends . Sci. Total Environ. , 408 : 1050 – 1058 .
- Lapuerta , M. , Fernandez , J.R. and Agudelo , J.R. 2008 . Diesel Particulate Emissions from Used Cooking Oil Biodiesel . Bioresour. Technol. , 99 : 731 – 740 .
- Krahl , J. , Munack , A. , Bahadir , M. , Schumacher , L. and Eslen , N. 1996 . Review: Utilization of Rapeseed Oil, Rapeseed Oil Methyl Ester or Diesel Fuel: Exhaust Gas Emissions and Estimation of Environmental Effects , SAE Paper; Warrendale, PA: SAE