Abstract
Hydrogen sulfide (H2S) is one of the most toxic and offensively odorous gases and is generated in anaerobic bioreactors. A middle-thermophilic sulfur-oxidizing bacterium (SOB), Thiomonas sp. strain RAN5, was isolated and applied for H2S removal from both artificial and anaerobically digested gas. When a bioreactor containing medium inoculated with RAN5 was aerated continuously with artificial gas (containing 100 ppm H2S) at 45 ° C for 156 hr, the H2S concentration in the vented gas was reduced by 99%. This was not affected by the presence of other microbes in the bioreactor. The H2S removal efficiency of the RAN5 bioreactor for anaerobically digested gas was greater than 99% at influent H2S concentrations ranging from 2 to 1800 ppm; the efficiency decreased to 90% at influent H2S concentrations greater than 2000 ppm. Thiomonas sp. strain RAN5 cannot survive at room temperature, and thus its leakage from a wastewater treatment plant would not damage sewage systems. These data suggest that Thiomonas sp. strain RAN5 may be a useful microorganism for H2S removal.
The hydrogen sulfide removal efficiency of the RAN5 bioreactor was greater than 99% over the long term, for influent H2S concentrations of up to 2000 ppm. Removal was not affected by the presence of other microbes. Leakage of strain RAN5 would not damage sewage systems.
Introduction
The emission of sulfur compounds into the environment totals 2.8×1014 g/year (CitationUchida, 1995); the amount derived from biological activity is estimated to be 70 TgS/year (CitationHuwa, 1994). Hydrogen sulfide (H2S) is one of the most toxic and offensively odorous gaseous sulfide compounds. It is commonly a component of emitted volcanic gas and is generated by the petrochemical industry and in anaerobic bioreactors such as wastewater treatment plants. H2S is generated during anaerobiosis by sulfur-reducing microorganisms and released into the atmosphere, occasionally causing a noxious odor when present at a sufficient concentration. Moreover, anaerobically digested gas, a type of biofuel, is composed of primarily methane, carbon dioxide, and H2S. The H2S component causes significant difficulties in power plants that use anaerobically digested gas for power generation.
Like photosynthetic bacteria, chemolithotrophic sulfur-oxidizing bacteria (SOB) utilize CO2 as a carbon source and acquire energy by oxidizing sulfur (and sulfide compounds) to sulfate. The removal of H2S by SOB has been investigated (CitationChung et al., 2001; CitationLee et al., 2005; CitationNicolai and Janni, 2001) and SOB bioreactors have been tested for their ability to remove excess H2S at anaerobic wastewater treatment plants. H2S is oxidized to sulfate by SOB and thus removed from the gas. However, a decline in the activity of SOB over time can lead to a decrease in H2S removal efficiency. In addition, contamination of H2S removal bioreactors by mesophilic environmental microorganisms can cause a marked decrease in H2S removal efficiency.
Generally, the sulfate generated in H2S removal bioreactors is removed by replacing the culture medium. In the context of a wastewater treatment plant, this replacement may release SOB into the environment, which in turn may corrode the facility's structural components, as SOB can degrade both concrete and metal (CitationAviam et al., 2004; CitationVincke et al., 1999). However, due to relatively low environmental temperatures, thermophilic SOB have little effect on the environment after being released into wastewater.
In this study, we isolated a middle-range thermophilic SOB from a hot spring and assessed its sulfur-oxidizing efficiency in a bioreactor contaminated with environmental mesophilic microorganisms such as those derived from soil or waste material treatment processes. Because the optimum growth temperature of the strain was 45 ° C, most environmental microorganisms would not be expected to grow in this H2S removal system. On the other hand, thermophilic SOB may not grow at room temperature or oxidize sulfur under mesophilic conditions. We investigated the influence of contamination with mesophilic environmental microorganisms on the sulfur-oxidizing ability of this strain under thermophilic conditions and the effect of releasing this strain into the environment.
Materials and Methods
Isolation of SOB
The SOB strain described herein was isolated from hot spring drainage sediment in Miyagi prefecture, Japan. SOB were selectively cultured from environmental samples using Cha medium (CitationCha et al., 1999), which contained (per L), 4.0 g K2HPO4, 4.0 g KH2PO4, 0.8 g MgSO4, 0.5 g Na2EDTA, 0.22 g ZnSO4, 0.05 g CaCl2, 0.01 g MnCl2, 0.001 g FeSO4, 0.01 g (NH4)6Mo7O24, 0.01 g (NH4)6Mo7O24, 10.0 g Na2S2O3, and 0.02 g yeast extract. Growth was allowed for 10 days at 45 ° C; then an aliquot was inoculated into fresh medium and again incubated at 45 ° C for 10 days. This procedure was repeated at least 20 times. Isolated SOB were preserved in glycerol (25%, v/v) at −80 ° C. The characteristics of the isolates, including incubation temperature, medium pH, and substrate utilization, were determined. Isolates were identified by 16S rRNA gene sequencing. A 1395-bp fragment of the 16S rRNA gene was amplified by polymerase chain reaction (PCR) using Ex Taq DNA polymerase (TaKaRa, Shiga, Japan) and universal bacterial primers (E8f and U1406r) (CitationBaker et al., 2003). Amplification reaction conditions were an initial denaturation at 94 ° C for 10 min, followed by 35 cycles of 94 ° C for 30 sec, 57.5 ° C for 20 sec, and 72 ° C for 1 min 30 sec, with a final extension at 72 ° C for 10 min. PCR products were purified using a MagExtractor-PCR & Gel Clean Up kit (Toyobo, Osaka, Japan) and sequenced using an ABI PRISM Big Dye Terminator Cycle Sequencing kit (Applied Biosystems, Foster City, CA, USA) and an ABI PRISM 310 Genetic Analyzer (Applied Biosystems) according to the manufacturer's instructions. The GenBank database was searched for the most similar sequence types, using the BLAST program.
The 16S rRNA gene sequence of the isolated strain, designated RAN5, was deposited in the DDBJ nucleotide sequence database under accession no. AB308269.
H2S removal from artificial gas
H2S removal was performed as described by CitationJin et al. (2005), shows a schematic of H2S removal from artificial gas. A 500-mL Erlenmeyer flask was filled with Cha medium lacking Na2S2O3 and inoculated with approximately 107 colony forming units (CFUs) of the RAN5 strain. The operating temperature was maintained at 45 ° C by using a water bath. H2S was introduced by passing an air stream over a 1 M HCl solution into which a solution of Na2S was dripped. The gas phase H2S concentration was controlled between 1 and 100 ppm by altering the Na2S concentration. Air containing H2S was injected into the culture medium as bubbles, approximately 1 mm in diameter, at 0.1 L/min by a diffuser. Gas sampling outlets were located upstream and downstream of the H2S removal bioreactor. Medium and influent/effluent gases were sampled simultaneously. The H2S concentration was determined using a gas detector tube (Gastec, Kanagawa, Japan), the SO4 2− concentration in the medium was measured using an ion chromatography system (Dionex Corp., Sunnyvale, CA, USA), and the pH was determined using a D-55 pH meter (Horiba, Kyoto, Japan). To determine the contaminating effects of environmental microorganisms, composted livestock manure (feedstock was cattle feces and sawdust; water content, 60%) was added to cultures to a final concentration of 0.2 g wet weight/L. The compost was added as a suspension in saline. SOB counts in the compost were determined based on CFUs on Cha plate medium at 30 and 45 ° C. To facilitate the identification of SOB, Cha medium was amended with bromothymol blue (final concentration, 0.05 g/L) as a pH indicator.
H2S removal from anaerobically digested gas
shows a schematic of H2S removal from anaerobically digested gas. RAN medium contained (per L): 0.17 g casein peptone, 0.03 g soy peptone, 0.05 g NaCl, 0.025 g K2HPO4, 3 g KH2PO4, and 0.025 g glucose. A 2-L medium bottle was filled with RAN medium and inoculated with 107 CFUs of strain RAN5. The operating temperature was maintained at 45 ° C by using a water bath. To control the pH and supply nutrients to the SOB, RAN medium (100 mL/day) was replaced continuously using a peristaltic pump. When the influent H2S concentration increased to greater than 1000 ppm, NaHCO3 (10 g/L) was added to the inflowing medium to counteract acidification. Anaerobically digested gas, with H2S, methane, and CO2 concentrations of approximately 2500 ppm, 60%, and 40%, respectively, was obtained from a livestock (swine) anaerobic wastewater treatment system (mesophilic upflow anaerobic sludge blanket [UASB]), diluted with air as necessary, and pumped into the culture medium at 0.5 L/min. The H2S concentration gradually increased from 2 to 2200 ppm. Sampling outlets were placed upstream and downstream of the SOB culture bottle.
Survival of RAN5 in wastewater
A wastewater sample (pH 6.3, chemical oxygen demand 1700 mg O/L) containing mesophilic microorganisms at approximately 108 CFU/mL (CitationHirooka et al., 2009) was obtained from a milk parlor and inoculated with the RAN5 strain. The sulfate concentration was determined at time 0 and after 1 and 3 days of anaerobic incubation 25 ° C, and the middle-thermophilic SOB counts were determined at the same times, by the most probable number (MPN) method using cha medium at 45 ° C.
Results
Isolation of SOB
A SOB strain was isolated by enrichment culture and purified by repeated dilution cultivation. This microorganism, designated as strain RAN5, was capable of oxidizing thiosulfate and producing sulfate. After cultivation of RAN5 for 10 days, the maximum amount of accumulated sulfate was observed at pH 6.5 and 45 ° C (). The characteristics of RAN5 and related species are shown in . The 16S rRNA gene sequence of strain RAN5 showed 99% similarity to that of the genus Thiomonas. Furthermore, RAN5 and the Thiomonas genus shared morphological and physiological characteristics such as growth temperature, substrate utilization, and optimum growth on mixotrophic medium ().
Table 1. Characteristics of isolates and related species
H2S removal from artificial gas
To determine the efficiency of H2S removal by this strain, Cha medium was inoculated with the RAN5 strain and air containing 1–100 ppm H2S was passed through the culture, at 0.1 L/min. The efficiency was defined as the difference in H2S concentration between the influent and effluent gases (). The H2S concentration decreased by 62% after 24 hr. The H2S removal efficiency increased for a period of 60 hr and then remained at 99% from 60 to 120 hr. The sulfate concentration in the medium increased concomitantly and reached 2.7 mM after 120 hr of incubation.
Figure 4. Influence of contamination with environmental microorganisms on H2S removal. Open circle: compost + RAN5; open square: RAN5; open triangle: compost.
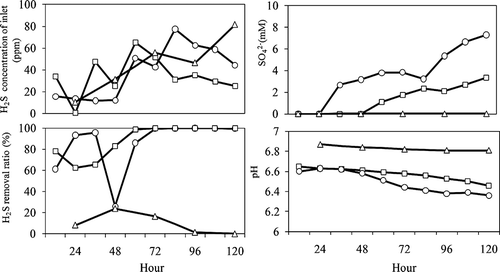
To assess the effect of contamination by environmental bacteria on H2S removal, compost was added to the Cha medium inoculated with RAN5, and the H2S removal efficiency was determined (). Although no SOB from the compost were detected on Cha plates, non-SOB microorganisms were detected from the compost, with 5.8 × 107 and 2.3 × 104 CFU/g appearing on Cha plates incubated at 30 and 45 ° C, respectively. Under contaminated conditions, H2S removal was 99% from 80 to 120 hr after contamination. Moreover, the sulfate concentration in the medium increased after 36 hr and subsequently accumulated to reach 7.2 mM at 120 hr. No marked H2S removal or sulfate accumulation occurred when the medium contained compost, but no RAN5. The pH of the medium decreased slightly in all experiments.
H2S removal from anaerobically digested gas
The RAN5 strain was inoculated into RAN medium and aerated with anaerobically digested gas (at 0.5 L/min), in which the H2S concentration was varied from 2 to 2200 ppm. The influent H2S concentrations and H2S removal efficiencies are shown in When the influent H2S concentration was between 2 and 1800 ppm, the removal efficiency was greater than 99%. However, at influent H2S concentration greater than 2000 ppm (at 948–961 and 1063–1088 hr), the effluent H2S concentration increased to 200 ppm and the removal efficiency decreased to 90%. Sulfate accumulated in the medium, reaching 52.8 mM at 1274 hr. At the final time point (1063 hr), H2S removal per unit volume and time was 46.67 g S/m3·hr.
Survival of RAN5 in wastewater
The survival of RAN5 in wastewater under environmental conditions was determined (). In wastewater maintained at 25 ° C, the RAN5 number (measured using the MPN method) decreased by 90% on day 1 and 99.2% on day 3. The sulfate concentration was measured at the same times. When the RAN5 strain was inoculated into sterilized wastewater, the sulfate concentration was increased slightly on day 3 (). In contrast, the inoculation of wastewater with the RAN5 strain resulted in a reduction of the sulfate concentration, from 0.246 to 0.062 mM, by day 3.
Table 2. Numbers of SOB in livestock wastewater inoculated with the RAN5 strain (MPN/mL)
Table 3. Amount of sulfate in livestock wastewater inoculated with the RAN5 strain. (SO4 2− mM)
Discussion
The RAN5 strain was isolated from hot spring wastewater drainage sediment. The 16S rRNA gene sequence of RAN5 showed 99% similarity to that of Thiomonas sp. Furthermore, the morphology and optimal growth conditions of RAN5 were similar to those of members of the genus Thiomonas. The genus Thiomonas, proposed by CitationMoreira and Amils (1997), belongs to the beta subclass of Proteobacteria, which also includes facultative autotrophic SOB of the genus Thiobacillus (CitationRobertson and Kuenen, 2006). SOB of the genus Thiomonas obtain energy by oxidizing sulfur, and the yeast extract contained in Cha medium promotes the growth of Thiomonas (CitationKelly and Wood, 2005). Bacteria of the genus Thiomonas have been isolated from activated sludge (CitationChen et al., 2004; CitationShooner et al., 1996) and mining acid drainage (CitationMichel et al., 2007).
Bioleaching by thermophilic sulfur-oxidizing microorganisms has been widely studied. CitationOkabe et al. (2005) isolated Leptospirillum MT6 from a pilot plant stirred-tank bioleaching operation. Sulfur-oxidizing archaea have been isolated from a bioleaching plant maintained at 78 ° C (CitationMikkelsen et al., 2006). CitationSalo-Zieman et al. (2006) isolated strain VS2 (98.5% 16S rRNA gene sequence similarity to Sulfolobus metallicua) from a mine. However, few studies have assessed the application of thermophilic SOB to H2S removal. Hydrogen sulfide is soluble in water as an acid, and its solubility depends more on the pH of the water than on the temperature (CitationMillero, 1986). Therefore, it is thought that the decrease in solubility with increasing temperature can be overcome by maintaining a neutral pH. Recently, CitationRyu et al. (2009) investigated the ability of the thermophilic SOB Bacillus sp. TSO3 to remove H2S from hot gases, considering that the use of thermophilic microorganisms for treatment of hot gases may be economically attractive because expensive precooling of gases would not be required. Similarly, the thermophilic growth conditions required by SOB may ameliorate the contamination of bioreactors with environmental mesophilic microorganisms. Furthermore, thermophilic SOB are unable to survive under mesophilic conditions and therefore would have little effect on the environment if released from a reactor.
Culturing RAN5 in the experimental bioreactor decreased the H2S concentration and increased the sulfate concentration. Contamination of the medium with environmental microorganisms did not inhibit the H2S removal efficiency. CitationSasaki et al. (2002), reported that ammonia assimilation by strain L2510 (99% 16S rRNA gene sequence similarity to Buttiauxella agrestis) decreased by 80% in the presence of microorganisms from lagoon water. As Buttiauxella spp. are heterotrophs (CitationKämpfer, 2005), this suggests that strain L2510 competes with environmental heterotrophic microorganisms for energy sources. Moreover, because L2510 is a mesophile, this phenomenon also occurred at 25 ° C. In contrast, when strain RAN5 was cultured in the presence of compost, 99% of the H2S was still removed between 80 and 120 hr. Thus, contamination by environmental microorganisms did not decrease bioreactor H2S removal efficiency, indicating that other microorganisms were unable to successfully compete with strain RAN5. The number of cultivable non-SOB microorganisms in compost decreased with an increase in incubation temperature. Nevertheless, approximately 10Citation4 CFU/g of microorganisms were able to grow under the same conditions as in the bioreactor. We propose that the maintenance of H2S removal by the RAN5 strain in the contaminated medium originated from not only the middle thermophilic conditions but also other factors such as chemolithotrophic growth by this strain.
Anaerobically digested gas is composed primarily of methane, carbon dioxide, and H2S. H2S causes significant problems in power plants that use anaerobically digested gas for power generation. H2S removal bioreactors are used at anaerobic wastewater treatment plants, which typically generate high concentrations of H2S. CitationSchieder et al. (2003), reported that for an influent H2S concentration of approximately 1000 ppm, removal efficiency by the bioreactor was over 90%. In the present study, the removal efficiency of the RAN5 bioreactor was greater than 99% when the influent H2S concentration was 2–1800 ppm and decreased to 90% when the influent H2S concentration was greater than 2000 ppm. This decrease might have been caused by insufficient oxygen availability, because the amount of influent air decreased as the H2S concentration increased. Our data suggest that it is necessary to ensure that the influent gas contains at least 30% air (6% O2) for maximum H2S removal efficiency. In an investigation of the conversion of sulfide to sulfur by Thiobacillus sp. strain A1, CitationAlcantara et al. (2004), suggested that a O2/S2− ratio of 0.5 or higher is required to completely consume sulfide. In this study, the H2S removal efficiency decreased at higher O2/S2− ratios (approximately 30). This decrease probably originated from the difference in solubility between H2S and O2. At 40 ° C, the solubilities of H2S and O2 are 1.66 and 0.023 cm3/cm3, respectively (CitationKobayashi et al., 2009). The maximum hydrogen sulfide removal per unit volume and time was 46.67 g S/m3·hr, which is very low compared with that reported by Lee et al. (810 g S/m3·hr) (CitationLee et al., 2005). It is thought that an increase in O2 solubility enables the removal of higher H2S concentrations.
SOB are often the main causal agents of corrosion and degradation of concrete in facilities such as sewage systems and wastewater treatment plants (CitationAviam et al., 2004). In the present study, the RAN5 strain did not survive in wastewater at 25 ° C. Because SOB were not detected in the wastewater prior to RAN5 inoculation, it seems likely that RAN5 was the only SOB present after inoculation. The sulfate concentration decreased in the wastewater inoculated with RAN5, possibly because of the activity of sulfate-reducing bacteria. The data suggest that strain RAN5 is incapable of sulfur oxidation at 25 ° C and would most likely have little effect on wastewater treatment facilities or drainage.
Acknowledgments
This work was supported financially by the Foundation of the Ministry of Education, Culture, Sports, Science and Technology of Japan as a “Project of Integrated Compost Science” and by a grant from the Livestock Technology Association, Japan.
References
- Alcantara , S. , Velasco , A. , Muñoz , A. , Cid , J. , Revah , S. and Razo-Flores , E. 2004 . Hydrogen sulfide oxidation by a microbial consortium in a recirculation reactor system: sulfur formation under oxygen limitation and removal of phenols . Environ. Sci. Technol. , 38 : 918 – 923 .
- Aviam , O. , Bar–Nes , G. , Zeiri , Y. and Sivan , A. 2004 . Accelerated biodegradation of cement by sulfur-oxidizing bacteria as a bioassay for evaluating immobilization of low-level radioactive waste . Appl. Environ. Microbiol. , 70 : 6031 – 6036 .
- Baker , G.C. , Smith , J.J. and Cowan , D.A. 2003 . Review and re-analysis of domain– specific 16s primers . J. Microbiol. Methods , 55 : 541 – 555 .
- Cha , J.M. , Cha , W.S. and Lee , J. 1999 . Removal of organo -sulphur odour compounds by thiobacillus novellus SRM, sulphur-oxidizing microorganisms . Process Biochem. , 34 : 659 – 665 .
- Chen , X.G. , Geng , A.L. , Yan , R. , Gould , W.D. , Ng , Y.L. and Liang , D.T. 2004 . Isolation and characterization of sulphur -oxidizing thiomonas sp. And its potential application in biological deodorization . Lett. Appl. Microbiol. , 39 : 495 – 503 .
- Chung , Y.C. , Huang , C. and Tsen , C.P. 2001 . Biological elimination of H2S and NH3 from waste gases by biofilter packed with immobilized heterotrophic bacteria . Chemosphere , 43 : 1043 – 1050 .
- Hirooka , K. , Asano , R. , Yokoyama , A. , Okazaki , M. , Sakamoto , A. and Nakai , Y. 2009 . Reduction in excess sludge production in a dairy wastewater treatment plant via nozzle-cavitation treatment: case study of an on-farm wastewater treatment plant . Bioresour. Technol. , 100 : 3161 – 3166 .
- Huwa , K. 1994 . Environmental Handbook , Tokyo : Asakura Publishing . (in Japanese)
- Jin , Y. , Veiga , M.C. and K.,C , Autotrophic . 2005 . Deodorization of hydrogen sulfide in a biotrickling filter . J. Chem. Technol. Biot. , 80 : 998 – 1004 .
- Kämpfer , P. 2005 . “ Genus Buttiauxella ” . In Bergey's Manual of Systematic BacteriologyPart B , 2nd , Edited by: Garrity , G.M. 641 – 648 . New York : Springer .
- Kelly , D.P. and Wood , A.P. 2005 . “ Genus Thiomonas ” . In Bergey's Manual of Systematic Bacteriology , 2nd , Edited by: Garrity , G.M. and Part , C. Vol. 2 , 757 – 758 . New York : Springer .
- Kobayashi , T. , Li , Y.Y. , Kubota , K. , Harada , H. , Maeda , T. , Kawai , K. and Yoshida , T. 2009 . Hydrogen sulfide removal from biogas by sulfur oxidizing bacteria under microaerophilic environment in the anaerobic digester . J. Environ. Syst. Eng. , 65 : 104 – 113 . (in Japanese)
- Lee , E.Y. , Cho , K.S. and Ryu , H.W. 2005 . Simultaneous removal of H2S and NH3 in biofilter inoculated with acidithiobacillus thiooxidans TAS . J. Biosci. Bioeng. , 99 : 611 – 615 .
- Michel , C. , Jean , M. , Coulon , S. , Dictor , M.C. , Delorme , F. , Morin , D. and Garrido , F. 2007 . Biofilms of as (III)-oxidising bacteria: formation and activity studies for bioremediation process development . Appl. Microbiol. Biot. , 77 : 457 – 467 .
- Mikkelsen , D. , Kappler , U. , McEwan , A.G. and Sly , L.I. 2006 . Archaeal diversity in two thermophilic chalcopyrite bioleaching reactors . Environ. Microbiol. , 8 : 2050 – 2056 .
- Millero , F.J . 1986 . The thermodynamics and kinetics of the hydrogen sulfide system in natural waters . Mar. Chem. , 18 : 121 – 147 .
- Moreira , D. and Amils , R. 1997 . Phylogeny of thiobacillus cuprinus and other mixotrophic thiobacilli: proposal for thiomonas gen. Nov . Int. J. Syst. Evol. Micr. , 47 : 522 – 528 .
- Nicolai , R.E. and Janni , K.A. 2001 . Biofilter media mixture ratio of wood chips and compost treating swine odors . Water Sci. Technol , 44 : 261 – 267 .
- Okabe , S. , Ito , T. , Sugita , K. and Satoh , H. 2005 . Succession of internal sulfur cycles and sulfur-oxidizing bacterial communities in microaerophilic wastewater biofilms . Appl. Environ. Microbiol. , 71 : 2520 – 2529 .
- Robertson , L.A. and Kuenen , J.K. 2006 . “ The genus Thiobacillus ” . In The Prokaryotes: A Handbook on the Biology of Bacteria: Proteobacteria: Alpha and Beta Subclass , Edited by: Dworkin , M. , Falkow , S. , Rosenberg , E. , Schleifer , K.H. and Stackebrandf , E. 812 – 827 . Oxford , , UK : Pergamon .
- Ryu , H.W. , Yoo , S.K. , Choi , J.M. , Cho , K.S. and Cha , D.K. 2009 . Thermophilic biofiltration of H2S and isolation of a thermophilic and heterotrophic H2S-degrading bacterium, bacillus sp. TSO3 . J. Hazard. Mater. , 168 : 501 – 506 .
- Salo-Zieman , V.L. , Sivonen , T. , Plumb , J.J. , Haddad , C.M. , Laukkanen , K. , Kinnunen , P.H. , Kaksonen , A.H. , Franzmann , P.D. and Puhakka , J.A. 2006 . Characterization of a thermophilic sulfur oxidizing enrichment culture dominated by a sulfolobus sp. Obtained from an underground hot spring for use in extreme bioleaching conditions . J. Ind. Microbiol. Biot. , 33 : 984 – 994 .
- Sasaki , H. , Maruyama , G. , Suzuki , H. , Nonaka , J. , Sato , M. , Sasaki , T. , Ohta , M. and Nakai , Y. 2002 . Characterization of ammonia-assimilating bacteria in a lagoon for wastewater from a paddock of dairy cattle . Anim. Sci. J. , 73 : 73 – 76 .
- Schieder , D. , Quicker , P. , Schneider , R. , Winter , H. , Prechtl , S. and Faulstich , M. 2003 . Microbiological removal of hydrogen sulfide from biogas By Means Of a separate biofilter system: experience with technical operation . Water Sci. Technol. , 48 : 209 – 212 .
- Shooner , F. , Bousquet , J. and Tyagi , R.D. 1996 . Isolation, phenotypic characterization and phylogenetic position of a novel, facultatively autotrophic, moderately thermophilic bacterium, thiobacillus thermosulfatus sp. Nov . Int. J. Syst. Bacteriol. , 46 : 409 – 415 .
- Uchida , A. 1995 . Gas Metabolism of Microorganisms and Environment , Tokyo : Japan Scientific Societies Press . (in Japanese)
- Vincke , E. , Verstichel , S. , Monteny , J. and Verstraete , W.A. 1999 . New test procedure for biogenic sulfuric acid corrosion of concrete . Biodegradation , 10 : 421 – 428 .