Abstract
Portable 24-hr sampling units were used to collect air samples from eight biofilters on four animal feeding operations. The biofilters were located on a dairy, a swine nursery, and two swine finishing farms. Biofilter media characteristics (age, porosity, density, particle size, water absorption capacity, pressure drop) and ammonia (NH3), hydrogen sulfide (H2S), sulfur dioxide (SO2), methane (CH4), and nitrous oxide (N2O) reduction efficiencies of the biofilters were assessed. The deep bed biofilters at the dairy farm, which were in use for a few months, had the most porous media and lowest unit pressure drops. The average media porosity and density were 75% and 180 kg/m3, respectively. Reduction efficiencies of H2S and NH3 (biofilter 1: 64% NH3, 76% H2S; biofilter 2: 53% NH3, 85% H2S) were close to those reported for pilot-scale biofilters. No N2O production was measured at the dairy farm. The highest H2S, SO2, NH3, and CH4 reduction efficiencies were measured from a flat-bed biofilter at the swine nursery farm. However, the highest N2O generation (29.2%) was also measured from this biofilter. This flat-bed biofilter media was dense and had the lowest porosity. A garden sprinkler was used to add water to this biofilter, which may have filled media pores and caused N2O production under anaerobic conditions. Concentrations of H2S and NH3 were determined using the portable 24-hr sampling units and compared to ones measured with a semicontinuous gas sampling system at one farm. Flat-bed biofilters at the swine finishing farms also produced low amounts of N2O. The N2O production rate of the newer media (2 years old) with higher porosity was lower than that of older media (3 years old) (P = 0.042).
Media characteristics and gas reduction efficiencies of eight field-scale biofilters were evaluated. The media characteristics measured in this study indicated that media older than 3 years should be tested for high-pressure drops and nitrous oxide (N2O) production. The results also indicated that regular water addition can improve gas reduction efficiencies of biofilters but adding excessive amounts of water can cause N2O production. Uniform water distribution can be a challenge.
Introduction
Biofiltration can be an effective technology for removing odors and gases in air from livestock systems. Advantages of biofiltration include high removal efficiency, low cost, and low energy requirements (CitationSattler et al., 2009). Biofilters can be used to treat exhaust of wall and pit fans of mechanically ventilated livestock buildings, exhaust of pit fans on naturally ventilated buildings, and exhaust of manure storage and treatment systems (CitationNicolai et al., 2008). Numerous laboratory-scale and pilot-scale biofiltration studies have been reported in the literature (CitationChen and Hoff, 2009; CitationChen et al., 2005; CitationChen et al., 2009; CitationChung et al., 2007; CitationDeng et al., 2009; CitationHong and Park, 2005; CitationNicolai and Janni, 2001; CitationNicolai and Janni, 2001; CitationPagans et al., 2007). Laboratory-scale biofilters have been commonly used to treat odors and selected compounds found in the exhaust air of livestock systems under controlled conditions and experiments (Chen et al., 2005; Chung et al., 2007; Hong and Park, 2005). Pilot-scale biofilters have been evaluated while treating gas emissions directly from various sources, including livestock barns, manure storages, and composting facilities (Chen et al., 2009; Deng et al., 2009; Nicolai and Janni, 2001; Pagans et al., 2007). In a review, it was noted that few full-scale biofiltration studies have been conducted (CitationChen and Hoff, 2009). One full-scale biofiltration study reported on the treatment of NH3 emissions from livestock manure composting facilities using rockwool-packed biofilters (CitationYasuda et al., 2009).
Biofiltration studies have mainly focused on reduction of odor, H2S, NH3, and specific volatile organic compound (VOC) emissions. Little information is available in the literature about CH4 and N2O reduction capabilities of gas-phase biofilters. CitationClemens and Chuls (2003) reported that biofilters with shredded root media did not reduce CH4 concentrations in exhaust air from municipal solid waste composting facilities and that N2O concentrations increased after biofiltration. CitationAkdeniz et al. (2011) reported that pilot-scale biofilters with lava rock media at 90% relative humidity and 5-sec empty bed contact time had reduction efficiencies of 25% and 0.7% for CH4 and N2O, respectively.
The main media characteristics include media water absorption capacity, porosity, density, particle size distribution, and pressure drop across the media (CitationChen and Hoff, 2009; CitationPoulsen and Jensen, 2007). Besides media characteristics, biofilter structure (flat-bed or vertical) is also important. Flat-bed biofilters with vertical airflow are commonly used to treat exhaust air from animal buildings. Vertical biofilters with horizontal airflow generally require less area and have lower pressure drops than flat-bed biofilters (CitationSadaka et al., 2002). The media in vertical biofilters settle and compact at the bottom of the biofilter which reduces the horizontal airflow. Also it is difficult to distribute moisture evenly in vertical biofilter media (CitationChen and Hoff, 2009).
High-moisture-content biofilter media improves gas absorption into the biofilm layer but can create anaerobic zones that cause additional gas production (CitationChianese et al., 2009; CitationDavidson et al., 2000; CitationRo et al., 2008). An example of additional gas production is N2O production from NH3. CitationClemens and Cuhls (2003) studied biofiltration of municipal solid waste exhaust and reported that N2O production was significantly correlated to biofilter NH3 reduction efficiency. Their results indicated that when the NH3 was the main nitrogen (N) source, about 26% of the NH3-N retained in the biofilter was converted to N2O. CitationDel Nero Maia et al. (2008) studied N2O production from NH3 and reported that the media moisture content affected microbial activity and N2O production.
Biofilter removal efficiencies are often measured by collecting grab samples of inlet and outlet air. Air samples are either collected in 10-L sampling bags for laboratory analysis or directly run through gas analyzers. Another common way to measure greenhouse gas concentrations is to collect air samples in glass vials using a syringe and analyzing the contents with a gas chromatography (GC). So far, few continuous or semicontinuous monitoring studies have been reported for full-scale biofilters. A recent National Air Emission Monitoring Study (NAEMS) used a semicontinuous sampling method to monitor gas emissions with multiple gas analyzers (CitationHeber et al., 2008). CitationLim et al. (2011) used this system to collect air samples from full-scale biofilters. This semicontinuous sampling method was accepted by the U.S. Environmental Protection Agency (EPA) but it was labor intensive and expensive. CitationJanni et al. (2010) described a 24-hr air sampling method for testing the effectiveness of mitigation technologies. The portable units fill 50-L air sample bags over 24-hr sampling periods for later analysis with gas analyzers in a laboratory. The same portable 24-hr sampling units were used in this study to collect air samples from eight existing biofilters located in western and southeastern Minnesota. The purpose of the study was to measure H2S, SO2, NH3, CH4, and N2O gas reduction efficiencies of full-scale biofilters used by dairy and swine producers and the biofilter media characteristics (age, media depth, porosity, density, particle size, water absorption capacity, and pressure drop).
Materials and Methods
Sampling sites
Site 1 was a dairy farm with two deep-bed biofilters located near Morris, MN. The biofilter located to the east was labeled biofilter 1 and the one to the west was labeled biofilter 2. Biofilters 1 and 2 were about 8 km apart. They treated air from the effluent outlet of anaerobic digesters. Biofilter 2 also treated air from a space where solid-separators treated the digester effluent. The media at this farm was several months old. The media depth of both biofilters was 2.0 m. The design empty bed contact times (EBCTs) of the biofilters were 64 sec ().
Table 1. Biofilter characteristics
Site 2 was a swine nursery farm with an A-frame-shaped vertical biofilter with horizontal airflow and a flat-bed biofilter. This farm was also located near Morris, MN. The A-frame biofilter was labeled biofilter 1 and the flat-bed biofilter was labeled biofilter 2. These biofilters treated air from two separate swine nursery rooms and manure pits. The media of the A-frame biofilter was about 1.5 years old and the flat bed biofilter media was about 3.5 years old. The flat-bed biofilter was designed and built using guidelines by CitationSchmidt et al. (2004). The media depth and design empty bed contact time of the flat-bed biofilter were 0.4 m and 5 sec, respectively (). The schematic of the A-frame biofilter is shown in The media depth through the A-frame legs ranged from 0.3 m at the bottom to 0.6 m at the top (CitationNicolai et al., 2005). Media depth across the top was 0.3 m and design EBCT was 4 sec (). The nursery barn airflow rates farm were not constant. At minimum ventilation rates during cold weather, the biofilter EBCTs increased up to 63 sec for the flat-bed biofilter and 51 sec for the A-frame biofilter.
Site 3 was a swine finishing farm with two flat-bed biofilters located near Dodge Center, MN. During the first visit, air samples were collected from two biofilters located on the west ends of the north and south finishing barns. The north biofilter was labeled biofilter 1 and the south biofilter was labeled biofilter 2. During subsequent visits, air samples were collected from the biofilters connected to the south barn because the north barn was empty. The biofilter on the west end of the south barn was labeled biofilter 1 and the one on the east end of the south barn was labeled biofilter 2. The biofilters at this farm treated pit fan exhaust from the barns with deep manure pits. The media was about 2 years old. The media depth of both biofilters was 0.4 m and design EBCT was 5 sec ().
Site 4 was a swine finishing farm with two flat-bed biofilters. This farm was also located near Dodge Center, MN. Air samples were collected from two biofilters located on the south side of the swine finishing barn. The biofilter on the west end was labeled biofilter 1 and the one on the east end was labeled biofilter 2. The biofilters at this farm treated pit fan exhaust from the barn with deep manure pits. The media at this farm was about 3 years old. The media depths of the biofilters were 0.4 m. The design EBCTs of biofilters 1 and 2 were 7 and 6 sec, respectively ().
Media characteristics
Approximately 75 L of media was collected from each biofilter to measure five media characteristics (porosity, density, water absorption capacity, particle size, and pressure drop across media). In order to have representative samples, media were collected from different locations and depths of the biofilters. At farms 1 and 2, media samples collected from biofilter 1 and 2 were analyzed separately. At farms 3 and 4, the media used for both biofilters at each farm was the same so media samples collected from the two biofilters at each farm were combined and analyzed together.
Media porosity (as-received media) was measured using two buckets and a simple method for filling and packing one bucket with media (CitationAkdeniz et al., 2011; CitationNicolai and Janni, 2001). Water was added to fill up all the voids. Percent porosity was calculated by multiplying the ratio of the water volume to the media volume by 100.
Media density (as-received media) was measured by weighing an empty 1-L glass container, filling the glass container with media and weighing the container again. Density (kg/m3) was calculated as the ratio of the weight of the media to 1 L (CitationAkdeniz et al., 2011).
Water absorption capacity (as-received media) was measured by soaking media in water. Water was added until the media was completely covered. After waiting for 3 min, the excess water was drained off. After waiting another 5 min, excess water was drained off again. The media weight prior to and after soaking with water was measured. Water absorption capacity (%) was calculated by multiplying the ratio of the weight of the absorbed water to the weight of the initial (presoaked) media by 100 (CitationAkdeniz et al., 2011).
Media particle size distributions were measured using seven Tyler brass sieves with 38.1-, 25.4-, 19.1-, 15.9-, 12.7-, 9.4-, and 4.8-mm openings and a solid tray at the bottom. Media particle size distribution (%) was calculated by multiplying the ratio of the weight of the media on each sieve to total media weight by 100 (CitationAkdeniz et al., 2011).
Media unit pressure drop (Pa/m) versus the unit airflow rate (m3/s per m2) was measured using a media column described by CitationNicolai and Janni (2001), CitationJanni et al. (2009), and CitationAkdeniz et al. (2011). After filling the column and settling the media, the static pressure was measured with a pressure sensor (267; Setra, Boxborough, MA) at six fan (AXC150A; Continental Fan, Buffalo, NY) settings (20%, 30%, 40%, 60%, 80%, and 100% power) and inlet airflow rate was measured with a vane anemometer (RVA801; Alnor, Shoreview, MN).
Gas-phase reduction efficiencies
Air sample collection
Air samples were collected using portable 24-hr air sampling units developed by CitationJanni et al. (2010). The schematic of the system is shown in Two inlet and two outlet air samples were collected from each biofilter using four sampling units per biofilter. To collect inlet samples, 6.3-mm-diameter holes were made in either wooden or hard plastic ducting that connected the fans blowing from the barn source to the biofilters. About 10-cm-long PTFE (Teflon) tubing (6.3 mm outer diameter [O.D.]) was inserted through the holes. This tubing was connected to two PTFE filters (1-μm openings, 47 mm diameter; Advantec MFS, Dublin, CA). Small holes were made with a needle on the first filter so that air could pass through easily. This filter served as a moisture trap. A moisture trap was necessary to protect the sampling pump in case condensation occurred as temperatures dropped during the night. No holes were made on the second filter, which served as a dust filter.
Figure 2. Top view of the 24-hr air sampling at the swine nursery farm (air sampling units were enlarged).
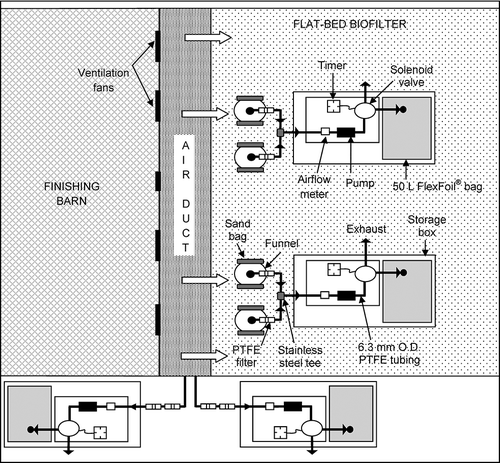
Outlet samples were collected using 12.5-cm-diameter stainless steel funnels. A similar funnel system was used by CitationChen et al. (2008). Two funnels were used per sampling unit. The two funnels were about 25 cm apart and connected with a stainless steel tee and PTFE tubing (). Two sets of funnels (total four funnels) were placed on each biofilter about 1 m apart. Funnels were buried about 5 cm deep inside the biofilter media. Two small sand bags (about 1.3 kg each) were placed on each funnel to prevent wind from tipping over the funnels and drawing ambient air during sampling. No moisture trap was used for outlet sampling since the relative humidity and therefore condensation risk in the outlet air stream was low.
The 24-hr sampling unit included an airflow meter, a pump, timer, three-way solenoid valve, and 50-L FlexFoil bag. FlexFoil bags (0.6 m × 0.9 m) were prepared by SKC (Eighty Four, PA). These bags were previously tested to store NH3, H2S, and greenhouse gases during 24-hr sampling without significant losses (CitationAkdeniz et al., 2011). During sampling, air passed through a flow meter, pump, and three-way solenoid valve. The solenoid valve was controlled by a timer; either exhausted the air or filled the 50-L FlexFoil sampling bag. The pump ran continuously to maintain fresh sample in the system. Teflon tubing and stainless steel connections were used throughout the system. The airflow rate was about 415 mL/min and samples were collected 4 min every 1 hr during 24 hr (total 40 L air sample). This sampling time was chosen based on previous experiments reported by CitationJanni et al. (2010).
Air sample analysis
After sampling was completed, the FlexFoil sampling bags were immediately taken to the laboratory and concentrations of five gases were measured within 4 hr. Ammonia (NH3), methane (CH4), and nitrous oxide (N2O) concentrations were measured with an infrared analyzer (INNOVA 1413; LumaSense Technologies A/S, Ballerup, Denmark) and hydrogen sulfide (H2S) and sulfur dioxide (SO2) concentrations were measured with a pulsed fluorescence analyzer (TEC450i; Thermo Electron Corporation, Franklin, MA). The sampled air inside the bags was run through the analyzers for 10 min and the readings after 10-min instrument stabilization time were recorded manually. Analyzers were checked using zero-grade air and calibration gases every week. Zero-grade air and calibration cylinders were purchased from Oxygen Service Company (St Paul, MN).
Quality assurance
There was concern about drawing ambient air in through the funnels. To test this, each time bags were filled, carbon dioxide (CO2) concentrations of the inlet and outlet bags were measured with the infrared analyzer (INNOVA 1412; LumaSense Technologies A/S).
Another quality assurance test was conducted by comparing 24-hr sample concentrations of H2S and NH3 to the concentrations measured using a semicontinuous gas sampling system. The semicontinuous gas sampling system was located at the swine nursery farm (site 2) and used by another research group to collect air samples from the inlets and outlets of the A-frame and flat-bed biofilters. The semicontinuous system sequentially drew sample air from six sample locations (one inlet and two outlet sample locations on both biofilters). The air samples were delivered to analyzers via a gas sampling system (GSS), which had PTFE tube sample lines connected to a computer controlled sampling manifold. Hydrogen sulfide was measured with a pulsed fluorescence analyzer (TEC 450i; Thermo Electron) and NH3 was measured with a chemiluminescence analyzer (TEC 17C; Thermo Electron). Measurements of H2S and NH3 were averaged every minute. The GSS sampled the six sample lines and an ambient line for 15 min 14 times a day. The first five measurements were not included in the calculations. The last ten measurements were used to calculate sample averages. Daily averages were calculated using the 14 sample averages from the time the 24-hr bag sampling started until it finished. Most of the time sampling started at noon and finished the following day at noon. More information about the semicontinuous gas sampling system was reported by CitationJanni et al. (2010, Citation2011).
Weather data
Weather data (temperature, dew point, relative humidity, and precipitation) was taken from the closest weather stations to the farms. These stations were within 10 km. Data were recorded every 20 min. Daily averages of temperature, dew point, and relative humidity data were calculated from the time sampling started to the time sampling finished. Precipitation values were summed for the 24-hr sampling period. Precipitation values 24 hr prior to sampling were also summed and reported.
Statistical analysis
Media characteristics were measured in triplicate. Gas concentrations were measured four times from the inlets and outlets of the biofilters. Samples were collected from two inlet and two outlet locations. Statistical analyses were conducted using JPM software version 9.0.0 from SAS (SAS, Cary, NC). Media characteristics (porosity, density, and water absorption capacity) and gas reduction efficiencies of the biofilters were compared using one-way analysis of variance (ANOVA). The 24-hr sampling method was compared to the semicontinuous sampling method using two-way analysis of ANOVA with sampling method and gas type (H2S and NH3) as main effects (P < 0.05).
Results and Discussion
Media characteristics
Media characteristics of the biofilters were reported in and and
Table 2. Biofilter media characteristics (n = 3)
Figure 3. Biofilter media unit pressure drop versus unit airflow rates for biofilters at four sites: (a) site 1, (b) site 2, (c) site 3, (d) site 4.
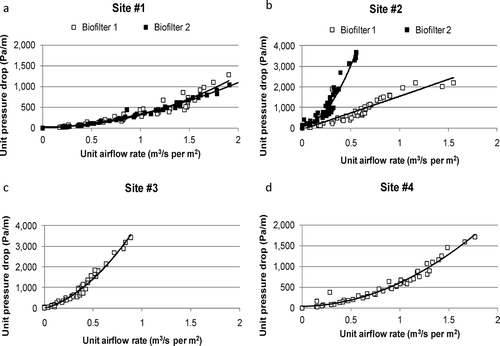
Figure 4. Particle size distribution by weight of the biofilter media at four sites: (a) site 1, (b) site 2, (c) site 3, (d) site 4.
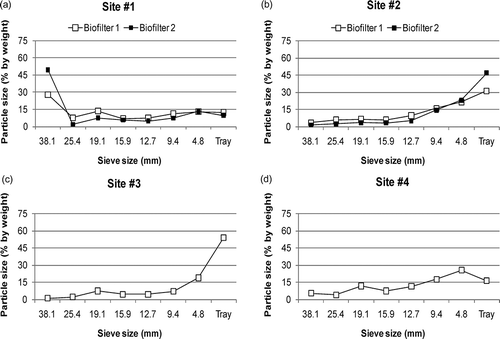
Site 1
The biofilter media at the dairy farm had been in use only a few months and was the most porous and least dense media (). The unit pressure drop across the media was the lowest and media particle size was the biggest ( and ).
Porosity, density, and pressure drop characteristics of the two dairy biofilters were similar to each other. Media particle size was slightly different. About 87.7% of the media of biofilter 1 was bigger than 4.8 mm, whereas this value was about 90.2% for biofilter 2. Water absorption capacities of the media were significantly different. The water absorption capacity of the media of biofilter 1 was low (32.6%), whereas water absorption capacity of the media of biofilter 2 was higher (54.3%) (P < 0.0001).
Site 2
The A-frame biofilter (biofilter 1) media had better characteristics compared to the flat-bed biofilter media (, and ). The A-frame biofilter media particle size distribution indicated that the media had more large particles than the flat-bed biofilter (). About 68.7% of the media was bigger than 4.8 mm. The unit pressure drop across the A-frame biofilter media was much lower than the pressure drop of the flat-bed biofilter. The water absorption capacity of the A-fame biofilter media was the highest (71.9%) among all the media tested. This might have been due to low moisture content of the media.
The flat-bed biofilter (biofilter 2) media was dense and it had the lowest porosity among all biofilters assessed in this study (). It had the highest unit pressure drop values, about two times higher than the unit pressure drop values of the dairy farm media (). The particle size of the media was small (). Only 53.2% of the media was bigger than 4.8 mm. At the dairy farm, about 90.0% of the media was bigger than 4.8 mm. These results support the observation that smaller media particles cause higher pressure drops across the media (CitationYang et al., 2010). There are no certain criteria in the literature, but based on the media characteristics and our observations, it can be concluded that replacement of the flat-bed biofilter media will be necessary soon.
Site 3
The media was less porous compared to the media at the other swine finishing (site 4) and dairy farms (). The media was dense (435 kg/m3) and particle size of the media was small. Only 45.8% of the media was larger than 4.8 mm (). At the other swine finishing farm, 83.6% of the media was larger than 4.8 mm. The unit pressure drop across the site 3 media was about twice higher than the unit pressure drops of the other swine finishing (site 4) and dairy media (). These results and our observations indicated that media replacement will be necessary soon.
Site 4
The media from site 4 was more porous compared to the media at the other finishing (site 3) and nursery farms (site 2). The water absorption capacity of the media was the lowest among all media. Media particle size was bigger (83.6% of the media was bigger than 4.8 mm) than the media particles at the other finishing and nursery farms. The unit pressure drop across the media was lower than the unit pressure drop of the media of the other finishing (site 3) and nursery (site 2) farms but higher than the dairy farm (site 1). Particle size and unit pressure drop data showed that media had good characteristics and it was not degraded as much as the media at the other finishing and nursery barns.
Quality assurance
Comparison of inlet and outlet CO2 concentrations
The percent difference between the inlet and outlet CO2 concentrations are shown in For all sampling events, the percent difference between the inlet and outlet CO2 concentrations was small (less than 1.4%). These results were promising but not considered sufficient to conclude that no ambient air was drawn in through the funnels. Because CO2 is a fairly water-soluble gas and some CO2 might be absorbed on the moist biofilm. Also, some amount of CO2 might be produced by degradation of organic material or oxidation of CH4. Therefore, CO2 data and semicontinuous sampling data were evaluated together to make a conclusion.
Table 3. Comparison of inlet and outlet air CO2 concentrations of biofilters
Comparison of 24-hr and semicontinuous sampling H2S and NH3 concentrations
Hydrogen sulfide and NH3concentrations measured by 24-hr and semicontinuous sampling systems were shown in Except for one case, the percent difference between H2S and NH3 concentrations of two sampling systems was less than 11.0%. Only during the fourth sampling day, the percent difference for H2S was 26.5% (biofilter 1). Although some differences were observed, there was no statistically significant difference between the gas reduction efficiencies of the 24-hr sampling method and semicontinuous sampling method. Based on the CO2 and semicontinuous sampling data, it was concluded that no significant amount of ambient air was drawn in through the funnels during sampling with the portable 24-hr sampling units.
Table 4. Comparison of H2S and NH3 reduction efficiencies measured by semicontinuous sampling and 24-hr sampling systems
Gas-phase reduction efficiencies
Average inlet gas concentrations were given in shows overall biofilter gas reduction efficiencies and shows specific gas reduction efficiencies.
Table 5. Inlet gas concentrations
Figure 5. Overall percent gas reduction efficiencies of the sites (averages of eight measurements were reported for each gas): (a) site 1, (b) site 2, (c) site 3, (d) site 4.
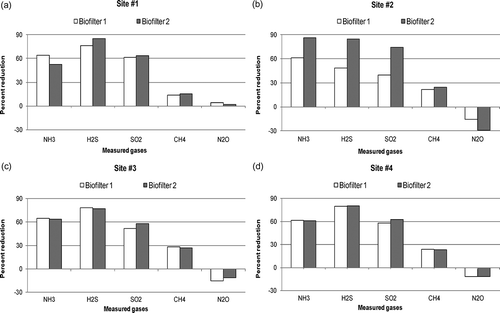
Figure 6. Percent gas reduction efficiencies of the biofilters (averages of two measurements were reported for each sampling date): (a) site 1, biofilter 1, (b) site 1, biofilter 2, (c) site 2, biofilter 1, (d) site 2, biofilter 2, (e) site 3, biofilter 1, (f) site 3, biofilter 2, (g) site 4, biofilter 1, (h) site 4, biofilter 2.
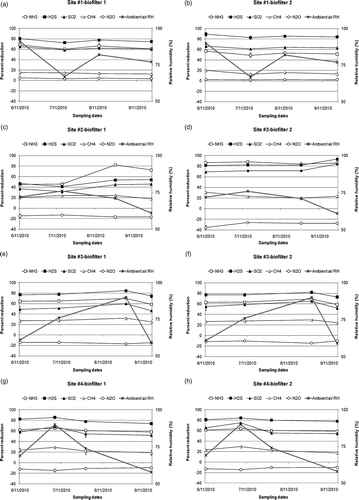
Site 1
Inlet concentrations of biofilter 2 (especially H2S and SO2) were significantly higher than the inlet concentrations of other biofilters (P < 0.0001 for H2S and SO2). H2S concentrations were about 50 times and SO2 concentrations were about 35 times higher than the inlet concentrations of other biofilters (). This was expected since biofilter 2 was treating air coming from an anaerobic digester and solid-liquid separator space.
Reduction efficiencies of biofilters 1 and 2 were slightly different. Gas reduction efficiencies of biofilter 1 were 64.0%, 76.0%, 61.0%, 14.0%, and 4.0% (, ), whereas the reduction efficiencies of biofilter 2 were 53.0%, 85.0%, 64.0%, 15.0%, and 2.2% (, ) for NH3, H2S, SO2, CH4, and N2O, respectively. No SO2 reduction efficiency has been reported in the literature for animal source biofilters. The NH3, H2S, CH4, and N2O reduction efficiencies were close to the ones previously reported for pilot-scale biofilters. Chen et al. (2009) reported average H2S reduction efficiencies as 81.8% and 88.6% for hardwood and wood chips media, respectively. NH3 reduction efficiency of the hardwood media was 43.4% and woodchips media was 74.0%. The NH3, H2S, CH4, and N2O reduction efficiencies were close to the ones previously reported for pilot-scale biofilters. CitationAkdeniz et al. (2011) tested lava rock at 5-sec empty bed contact time and reported reduction efficiencies as 56.0%, 88.0%, 25.0%, and 0.7% for NH3, H2S, CH4, and N2O, respectively.
At site 1, up to 4.0% N2O reduction was measured. At the other sites, biofilter media had a less porous structure and N2O production rather than reduction was observed. N2O generation from NH3 has been reported when aerobic and anaerobic conditions both exist. At the beginning, the media must be handled aerobically to let NOx be produced and then handled anaerobically to let NOx be reduced to N2 with intermediate production of N2O and NO (CitationChianese et al., 2009; CitationDavidson et al., 2000). This usually happens when dry media gets wet in heavy rain and media pores are filled with water. Production of N2O is less likely to occur when the media is new, since theoretically it is harder to have anaerobic zones inside porous new media.
Site 2
Inlet gas concentrations of the swine nursery farm were significantly lower than the inlet gas concentrations of the dairy and swine finishing farms (). Percent gas reduction efficiencies of the A-frame and flat-bed biofilters were significantly different. Average gas reduction efficiencies of the A-frame biofilter were 61.0%, 49.0%, 40.0%, 21.5%, and −15.3% for NH3, H2S, SO2, CH4, and N2O, respectively (, ). Reduction efficiencies of the A-frame biofilter were close to the reduction efficiencies of site 1 except H2S and N2O. H2S reduction efficiency of the biofilter was the lowest among all the biofilters tested. Negative reduction efficiency of N2O showed that N2O was generated rather than being reduced.
The flat-bed biofilter average gas reduction efficiencies were 86.0%, 85.0%, 74.0%, 24.0%, and −29.2% for NH3, H2S, SO2, CH4, and N2O, respectively (, ). Gas reduction efficiencies of the flat-bed biofilter were the highest among all the biofilters assessed in the study. However, the highest N2O generation rate was also measured from this biofilter. At the other sites (sites 1, 3, and 4), no water was added to biofilter media except rainwater. But at this site, a garden sprinkler was used to add water to the flat-bed biofilter and soaker hoses were used to add water to the A-frame biofilter. During sampling it was observed that the media of the flat-bed biofilter was wet and had a soil-like structure. The moistness and soil-like structure of the flat-bed media might have increased gas reduction efficiencies but at the same time caused N2O generation. Although water was added to the A-frame biofilter, since the biofilter sides were 2.4 m high and 0.3 to 0.6 m thick, water distribution with soaker hoses could not be done uniformly. The high water absorption capacity (71.9%) of the media also indicated that the media was dry.
Site 3
Inlet gas concentrations of site 3 were slightly higher than the inlet concentrations of the other finishing farm (site 4). The animal numbers at the finishing farms were different and this may have affected inlet gas concentrations. Gas concentrations of site 3 were significantly higher than the gas concentrations of the nursery swine farm but lower than the average concentrations of the dairy farm () (P < 0.0001 for NH3, H2S, SO2, CH4, and P = 0.040 for N2O).
Percent gas reduction efficiencies of the biofilters were similar to each other. Only SO2 and N2O reduction efficiencies of the biofilters were slightly different. Average gas reduction efficiencies of the biofilters were about 64.0%, 77.5%, 55.0%, 27.5%, and −13.0% for NH3, H2S, SO2, CH4, and N2O, respectively (, , f). Ammonia and H2S reduction efficiencies of the biofilters were close to the reduction efficiencies of biofilter 1 at site 1 with new media. Although some N2O production was observed, the production rate was significantly lower than the N2O production of the flat-bed biofilter at the nursery farm.
Site 4
Inlet gas concentrations of the site were significantly higher than the gas concentrations of the nursery farm but lower than the average gas concentrations of the dairy farm () (P < 0.0001 for NH3, H2S, SO2, and CH4 and P = 0.040 for N2O).
Percent gas reduction efficiencies of the biofilters were close to each other. Only a slight difference was observed for SO2. Average gas reduction efficiencies of the biofilters were about 61.0%, 80.0%, 60.0%, 23.0%, and −12.0% for NH3, H2S, SO2, CH4, and N2O, respectively (, , h). Reduction efficiencies of NH3, H2S, SO2, and CH4 were close to the gas reduction efficiencies of the other finishing farm. The average N2O production of the site was lower than the N2O production of the other finishing and nursery farms. This was expected, since media tests showed that media at this site had a more porous structure.
The effect of ambient air relative humidity on gas reduction efficiencies
Average temperature, dew point, relative humidity, and total precipitation of the sites were shown in Ambient air relative humidity data is also shown on the secondary y-axis of The ambient temperature ranged from 9.5 to 25.0 °C. The ambient air relative humidity ranged from 58.0% to 91.0%. The highest relative humidity (RH) values were recorded for the first sampling day at site 1 (91.0% RH and 3.3 cm precipitation) and third sampling day at site 3 (90.0% RH and 5.7 cm precipitation).
Table 6. Average temperature, dew point, relative humidity, and total precipitation of the sites
There was no water management at sites 1, 3, and 4. For these sites, a similar trend was observed for NH3, H2S, and SO2 reduction efficiencies and ambient air relative humidity ( and ). The highest NH3, H2S, and SO2 reduction efficiencies were measured when the relative humidity of the ambient air was highest and the lowest reduction efficiencies were measured when the relative humidity values were lowest. Since there was no water addition, the moisture content of the media was affected by relative humidity of air. Higher relative humidity helped the media to gain moisture and improve gas reduction efficiencies by absorbing gases on the moist media surface. A garden sprinkler or a soaker with a timer could be used to facilitate watering during sunny and windy days. But, while adding water, it should be remembered that adding excessive amounts of water will cause leachate and N2O production.
Conclusions
The 24-hr sampling system performed well collecting air samples from field-scale biofilters. Gas reduction efficiencies of the biofilters ranged from 53.0% to 86.0% for NH3, 49.0% to 85.0% for H2S, 40.0% to 74.0% for SO2, 14.0% to 27.5% for CH4, and −29.2% to 4.0% for N2O. The media at the dairy farm that was in use only a few months was the most porous and had the lowest unit pressure drop. NH3 and H2S reduction efficiencies of the biofilters were close to ones previously reported for pilot-scale biofilters. No N2O production was observed.
The flat-bed biofilter with media over 3 years old had the lowest porosity, the highest pressure drop across the media, and the highest H2S, SO2, NH3, and CH4 reduction efficiencies. This biofilter, however, had the highest N2O production. A watering system was used to add water to this biofilter. It is believed that the moist soil-like structure of the media increased reduction efficiencies of H2S, SO2, NH3, and CH4 but at the same time caused N2O generation. It was not one of the objectives of this paper but in future studies, the effect of the media moisture content on gas reduction efficiencies of field-scale biofilters should be investigated. Leachate content can be also assessed.
Acknowledgments
This project was funded by Rapid Agricultural Response Fund. The authors acknowledge Ilya Salnikov for his assistance during experiments and Brian Hetchler for providing semicontinuous sampling data for the swine nursery farm. The authors also acknowledge Ken Folie, Lee Johnston, and Minnesota livestock producers for their cooperation and support.
References
- Akdeniz , N. , Janni , K.A. , Jacobson , L.D. and Hetchler , B.P. 2011 . Comparison of gas sampling bags to temporarily store hydrogen sulfide, ammonia, and greenhouse gases . Trans. ASABE. , 54 : 653 – 661 .
- Akdeniz , N. , Janni , K.A. and Salnikov , I.A. 2011 . Biofilter performance of pine nuggets and lava rock as media . Bioresour. Technol. , 102 : 4974 – 4980 . doi: 10.1016/j.biortech. 2011.01.058
- Chen , L. and Hoff , S.J. 2009 . Mitigation odors from agricultural facilities: a review of literature concerning biofilters . Appl. Eng. Agric. , 25 : 751 – 766 .
- Chen , L. , Hoff , S.J. , Cai , L. , Koziel , J.A. and Zelle , B. 2009 . Evaluation of wood chip based biofilters to reduce odor, hydrogen sulfide, and ammonia from swine barn ventilation air . J. Air Waste Manage. Assoc. , 59 : 520 – 530 . doi: 10.3155/1047-3289.59.5.520
- Chen , L. , Hoff , S.J. , Koziel , J.A. , Cai , L. , Zelle , B. and Sun , G. 2008 . Performance evaluation of a wood chip-based biofilter using solid-phase microextraction and gas chromatography-mass spectrometry-olfactometry . Bioresour. Technol. , 99 : 7767 – 7780 . doi: 10.1016/j.biortech. 2008.01.085
- Chen , Y.X. , Yin , J. and Wang , K.X. 2005 . Long-term operation of biofilters for biological removal of ammonia . Chemosphere. , 58 : 1023 – 1030 . doi: 10.1016/j.chemosphere.2004.09.052
- Chianese , D.S. , Rotz , C.A. and Richard , T.L. 2009 . Whole-farm greenhouse gas emissions: a review with application to a Pennsylvania dairy farm . Appl. Eng. Agric. , 25 : 431 – 442 .
- Chung , Y.C. , Ho , K.L. and Tseng , C.P. 2007 . Two-stage biofilter for effective NH3 removal from waste gases containing high concentrations of H2S . J. Air Waste Manage. Assoc. , 57 : 337 – 347 .
- Clemens , J. and Cuhls , C. 2003 . Greenhouse gas emissions from mechanical and biological waste treatment of municipal waste . Environ. Technol. , 24 : 745 – 754 .
- Davidson , E.A. , Keller , M. , Erickson , H.E. , Verchot , L.V. and Veldkamp , E. 2000 . Testing a conceptual model of soil emissions of nitrous and nitric oxide . Bioscience. , 50 : 667 – 680 .
- Del Nero Maia , G. , Gates , R.S. , Day , G.B. , Sales , T. , Singhand , A. and Taraba , S.J.L. Contaminant gas generation as products of degraded ammonia during biofiltration . Proceedings of ASABE Annual International Meeting . June 29–July 2 2008 , Providence , RI . St. Joseph , MI : ASABE . paper no. 084630
- Deng , L. , Chen , H. , Chen , Z. , Liu , Y. , Pu , X. and Song , L. 2009 . Process of simultaneous hydrogen sulfide removal from biogas and nitrogen removal from swine wastewater . Bioresour. Technol. , 100 : 5600 – 5608 . doi: 10.1016/j.biortech. 2009.06.012
- Heber , A.J. , Bogan , W.W. , Ni , J.-Q. , Lim , T.T. , Ramirez-Dorronsoro , J.C. , Cortus , E.L. , Diehl , C.A. , Hanni , S.M. , Xiao , C. , Casey , K.D. , Gooch , C.A. , Jacobson , L.D. , Koziel , J.A. , Mitloehner , F.M. , Ndegwa , P.M. , Robarge , W.P. , Wang , L. and Zhang , R. The National Air Emissions Monitoring Study: overview of barn sources . Proceedings of 8th International Livestock Environment Symposium . September 1–5 2008 , Iguassu Falls , Brazil. pp. 199 – 205 . St. Joseph , MI : ASABE .
- Hong , J.H. and Park , K.J. 2005 . Compost biofiltration of ammonia gas from bin composting . Bioresour. Technol. , 96 : 741 – 745 . doi: 10.1016/j.biortech. 2004.10.008
- Janni , K.A. , Akdeniz , N. , Schmidt , D.R. , Jacobson , L.D. and Hetchler , B.P. A 24-hour air sample collection system . Proceedings of ASABE Annual International Meeting . June 20–23 2010 , Pittsburgh , PA . St. Joseph , MI : ASABE . paper no. 1009597
- Janni , K.A. , Jacobson , L.D. , Hetchler , B.P. and Johnston , L.J. Comparing semi-continuous air sampling to 24-h bag samples . Proceedings of ASABE Annual International Meeting . August 7–10 2011 , Louisville , KY . St. Joseph , MI : ASABE . paper no. 1111163
- Schmidt , D.R. , Goldman , A. , Schaar , T. and Janni , K.A. Alternative gas phase biofilter media characteristics and performance . Proceedings of ASABE Annual International Meeting . June 21–24 2009 , Reno , NV . St. Joseph , MI : ASABE . paper no. 097037
- Lim , T.T. , Jin , Y. , Ni , J.W. and Heber , A.J. Field tests of biofilters in reducing aerial pollutant emissions from commercial finishing barn . Proceedings of ASABE Annual International Meeting . August 7–10 2011 , Louisville , KY . St. Joseph , MI : ASABE . paper no. 1111336
- Nicolai , R.E. and Janni , K.A. 2001 . Biofilter media mixture ratio of wood chips and compost treating swine odors . Water Sci. Technol. , 44 : 261 – 267 .
- Nicolai , R.E. and Janni , K.A. Determining pressure drop through compost-woodchip biofilter media . Proceedings of ASAE Annual International Meeting . July 29–August 1 2001 , Sacramento , CA . St. Joseph , MI : ASAE . paper no. 014080
- Nicolai, R.E., K.J. Janni, and D.R. Schmidt. 2008. Biofiltration: mitigation for odor and gas emissions from animal operations http://www.extension.org/pages/Biofiltration:_Mitigation_for_Odor_and_Gas_Emissions_from_Animal_ Operations (http://www.extension.org/pages/Biofiltration:_Mitigation_for_Odor_and_Gas_Emissions_from_Animal_ Operations) (Accessed: 6 June 2011 ).
- Nicolai , R.E. , Lefers , R. and Pohl , S.H. Configuration of a vertical biofilter . Proceedings of 7th International Livestock Environment Symposium . May 18–20 2005 , Beijing , China. pp. 358 – 364 . St. Joseph , MI : ASABE .
- Pagans , E. , Font , X. and Sanchez , A. 2007 . Coupling composting and biofiltration for ammonia and volatile organic compound removal . Biosyst. Eng. , 97 : 491 – 500 . doi: 10.1016/j.biosystemseng.2007.03.03S
- Poulsen , T.G. and Jensen , A.H.B. 2007 . Gaseous ammonia uptake in compost biofilters as related to compost water content . J. Air Waste Manage. Assoc. , 57 : 940 – 946 . doi: 10.3155/1047-3289.57.8.940
- Ro , K.S. , McConnell , L.L. , Johnson , M.H. , Hunt , P.G. and Parker , D. 2008 . Livestock air treatment using PVA-coated powdered activated carbon biofilter . Appl. Eng. Agric. , 24 : 791 – 798 .
- Sadaka , S. , Magura , C.R. and Mann , D.D. 2002 . Vertical and horizontal airflow characteristics of wood/compost mixtures . Appl. Eng. Agric. , 18 : 735 – 741 .
- Sattler , M.L. , Garrepalli , D.T. and Nawal , C.S. 2009 . Carbonyl sulfide removal with compost and wood chip biofilters, and in the presence of hydrogen sulfide . J. Air Waste Manage. Assoc. , 59 : 1458 – 1467 . doi: 10.3155/1047-3289.59.12.1458
- Schmidt, D.R., K.J. Janni, and R.E. Nicolai. 2004. Biofilter design information http://www.manure.umn.edu/assets/baeu18.pdf (http://www.manure.umn.edu/assets/baeu18.pdf) (Accessed: 6 June 2011 ).
- Yang , L. , Wang , X. , Funk , T.L. , Gates , R.S. and Jiang , N. Optimization of livestock air pollutants removal biofilter part 1: media selection . Proceedings of ASABE Annual International Meeting . June 20–23 2010 , Pittsburgh , PA . St. Joseph , MI : ASABE . paper no. 1009802
- Yasuda , T. , Kuroda , K. , Fukumoto , Y. , Hanajima , D. and Suzuki , K. 2009 . Evaluation of full-scale biofilter with rockwool mixture treating ammonia gas from livestock manure composting . Bioresour. Technol. , 100 : 1568 – 1572 . doi: 10.1016/j. biortech. 2008.09.033