Abstract
A new method for rapidly eliminating pathogenic microorganisms in large air space using spraying •OH radicals is presented in this paper. With a physical method of strong electric-field discharge, large numbers of •OH radicals were produced by the oxygen activated particles of O2 +, O( 1D), O( 3P), etc., and the introducing reagent HO2 −. The gram-positive bacteria Bacillus subtilis, the gram-negative bacteria Serratia marcescens, and Bacillus spores were used for the eliminating experiments. Results show that the different microorganisms were rapidly killed by •OH radicals with a concentration of 0.8 mg/L and spraying density of 21 μL/m2 within 4 sec. Cell morphological changes were also observed under microscope. The cells of B. subtilis and Bacillus spores in their cellular wall, cellular membrane, or cell protoplasm were greatly destroyed when being exposed to a killing dosage of •OH radicals.
Increasing air spread of pathogenic microorganisms has prompted serious concerns for their threat to environmental safety. However, there is no an effective method to rapidly eliminate these harmful microorganisms in large air space. In comparison with conventional disinfectants, advanced oxidation technology (AOT) based on hydroxyl radicals (•OH) has excellent advantages. The main problem of how to produce the •OH radicals with high concentration and large production is solved by a physical method of strong electric-field discharge. A new method for rapidly eliminating pathogenic microorganisms in large air space using spraying •OH radicals is described in this paper.
Introduction
As the environmental pollution is getting worse nowadays, pathogenic microorganisms suspended in air space are extraordinary active, resulting in a higher incidence of infectious diseases than ever before, and are extremely threatening human health and the survival of animals and plants. Between the months of November 2002 and July 2003, severe acute respiratory syndrome (SARS) rapidly spread from China to other 37 countries around the world, leading to 775 human deaths with an economic loss of 40 billion US dollars (CitationSmith, 2006). In early 2009, a new strain of swine-origin H1N1 spread worldwide from Mexico. This novel H1N1 was declared as an influenza pandemic by the World Health Organization (WHO) (CitationWorld Health Organization, 2010), causing about 17,000 human deaths by the start of 2010. Therefore, it is very important to develop a rapid and efficient method for the elimination of pathogenic microorganisms in large air space.
Currently, chlorine, alkali, and alkali-alcolol-amine as the three kinds of main chemical disinfectants are widely used for eliminating microbial contamination. However, some drawbacks (CitationRäber and McGuire, 2002; CitationRaber et al., 2001) are as follows: (1) With the selectivity, a kind of chemical disinfectant could only correspondingly kill one or several kinds of pathogenic microorganisms. (2) The processing time is long, in the range of 0.5∼1 hr owing to the low chemical reaction rate. (3) The lethal dosage value is very high, which could reach 9% (v/v), resulting in a serious secondary pollution by the remaining chlorine compounds. (4) The lethal processing is limited on the surface of objects, impossible to be applied to large air space.
In comparison with the above chemical disinfectants, advanced oxidation technology (CitationGlaze et al., 1987; CitationRoberto and Vincenzo, 1999) (AOT)-based on hydroxyl radicals (•OH) has several excellent advantages: (1) Without selectivity, the •OH radicals can kill any pathogenic microorganisms in low lethal dosage because of its strong oxidizability whose oxidation potential is 2.8 V, only less than that of fluorine. (2) The •OH processing time is very short, within several seconds. The reason is that chemical reaction rate of •OH is up to 109 L·mol−1·sec−1, which is 107 times higher than that of other oxidants such as O3, H2O2, Cl2, and so on. (3) As a green oxidant, the •OH radicals are decomposed into H2O and O2 without any residual oxidant after its biochemical reactions.
The main problem for rapidly eliminating pathogenic microorganisms in large air space is how to produce the •OH radicals with high concentration and large production. Currently, the main methods are Fenton catalysis (CitationShreya et al., 2007; CitationVeressinina and Trapido, 2008), photocatalysis (CitationMaurizio and Vincenzo, 2005), ozone (CitationNaoyuki and Yukako, 2005), water injection (CitationNick and Satoshi, 2010), as well as their collaborative effects. However, these technologies have some serious disadvantages: (1) The •OH radicals are obtained in a low concentration and low amount of production, so that the whole biochemical reaction time is long, in the range of 15∼360 min. The above-mentioned technologies are only applied to the small-scale experiments or applications. (2) Large numbers of medicaments or catalysts such as H2O2, TiO2, Fe2+, and so on are necessary in the process of •OH production, resulting in its high cost as well as the safety problem of H2O2. (3) In order to increase the •OH production, several kinds of technologies are collaborated together resulting in large-volume accessory equipments such as the bubble tower, rotating packed bed, and so on.
In our previous studies, large number of •OH radicals were produced by the ionization and dissociation of O2 in air and H2O at gas state using a physical method of strong electric-field discharge. And the •OH radicals have been successfully used in the treatment of ship's ballast water and red-tide in ocean (CitationBai et al., 2005a, Citation2005b). In this paper, the killing effects of •OH radicals on the pathogenic microorganisms and their cell morphological changes are presented.
Formation of •OH Radicals
In order to obtain larger number of •OH radicals, the electron energy has to be higher than the ionization potential of O2 and H2O (12.5 and 12.6 eV, respectively) and dissociation potential of O2 (8.4 eV), respectively. With a method of strong electric-field discharge (SEFD), the electrons have the average energy of 9 eV, accounting for a lot of electrons to have enough high energy to ionize and dissociate O2 and H2O.
The plasma processes of •OH formation are shown in , parts (a) to (c).
In part (a), the O2 molecules are ionized and dissociated into the oxygen active particles of O2 +, O(1D), O(3P), etc. With a very short life time, the O(1D) and O(3P) rapidly react with O2 to form ozone (O3). The concentration of O active particles reaches 240 mg/L (CitationBai et al., 2000b), 3 times higher than that of current plasma techniques of about 70 mg/L (CitationBai et al., 2000a).
In part (b), the H2O molecules at a gas state are ionized and excited into H2O+ and H2O* to further form H2O2. The content of H2O2 is about 0.34 μg/L. The H2O2 loses an H+ to form HO2 −, a key introducing reagent of •OH, by an equilibrium reaction. HO2 − reaction rate constant is 2.2 × 106L·mol−1·sec−1, which is 30 thousand times higher than that of HO− introducing reagent with only 70 L·mol−1·sec−1 (CitationXu and Zhao, 2003).
In part (c), the •OH radicals are generated by the three plasma processes (1), (2), and (3). When the electron energy is between 8.4 and 12.5 eV, the •OH radicals are formed mainly from the O3 with HO2 − following the reaction of (1) (CitationGlaze et al., 1987; CitationStaehelin and Hoigne, 1982). When the electron energy is higher than 12.5 eV, the •OH radicals come mainly from O2 + with H2O to form water cluster ions O2 + (H2O) and then the dissociative reactions following the reaction of (2) (CitationPenetrante et al., 1997). In addition, the •OH radicals are produced from H2O+ reacting with water cluster electrons e− aq following the reaction of (3).
The •OH radicals in water involve very complicating chain reactions, which are very quickly to convert into other active particles such as O2 −•, O3 −•, HO3 •, HO2 −, HO2 •, and so on, meanwhile rapidly killing the pathogenic microorganisms.
Experiment
Experimental system
The experimental system for killing pathogenic microorganisms in air space is shown in The O2 enriched from air with a purity of 98.5% and the H2O at gas state (3.5%, v/v) were introduced into the plasma reactor. With the strong electric-field discharge (SEFD), the active particles of O2 +, O3, and H2O+, H2O2 were produced to together inject into a gas/liquid dissolver 4. In water pipe with the high pressure injecting of dissolver 4, the •OH radicals and other oxygen active particles were formed to further dissolve into water through the gas/liquid separator 5. The remaining gases were removed by electric heating with eliminator 6. With a nozzle, large numbers of •OH fogdrops were sprayed to the body with microorganisms by BP-1035 electric sprayer. The treating capacity of the equipment is 0.5 m3/hr.
Figure 2. The system for killing pathogenic microorganisms in air space: 1, 11 = valves; 2 = liquid flowmeter; 3 = pump; 4 = gas/liquid dissolver; 5 = gas/liquid separator; 6 = eliminator of residual gas; 7 = fogdrops; 8 = body with microorganisms; 9 = controller; 10 = transformer; 12 = gas flowmeter; 13 = plasma reactor; 14 = check valve.
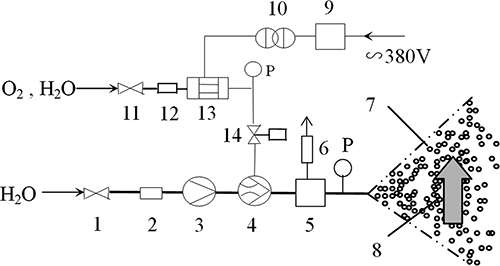
The closing configuration of plasma reactor is shown in , with the dimensions of 230 (length) × 150 (width) × 40 (height) mm3. The strong electric-field discharge plasma was filled in two microgaps (width = 0.47 mm) at an atmospheric pressure and ambient temperature. Two α-Al2O3 dielectric layers, with the thickness of 0.2 mm, dielectric constant of 10, and the insulation intensity of 350 kV/cm, were covered on the discharge electrode of Ag thin plate (180 × 90 mm2). The self-made power supply was applied on the discharge electrodes with the following parameters: applied voltage, 2.5 kV; frequency, 10.4 kHz; current pulse width, 5∼10 ns. The electric bridge techniques of high voltage are used to measure the discharge power (CitationZhang et al., 2003), which can efficiently eliminate the measure error from the capacitor of discharge gap.
Test of •OH radicals
As for the measurement of •OH concentration, ortho-hydroxybenzoic acid (salicylic acid, SA) was employed as a probe reagent, and the method was described previously (CitationJen et al., 1998; CitationQuan et al., 2007). When the SA was applied in the gas/liquid dissolver, the rapid reaction between SA and •OH radicals resulted in the formation of 2,3-dihydroxybenzoic acid (2,3-DHBA) and 2,5-dihydroxybenzoic acid (2,5-DHBA). Then the •OH concentration was obtained by testing the production of these two compounds through high-performance liquid chromatography (HPLC; Dionex, Sunnyvale, CA, USA). As shown in , the peaks of 2,3-DHBA and 2,5-DHBA are obviously identified in the liquid chromatogram of SA hydroxyl compounds, indicating that •OH radicals assuredly existed in water. The concentration of other oxygen active particles was converted into the •OH concentration according to their oxidation potential.
The spray density of •OH radicals was calculated from the flow rate of •OH solution, spray time, and spray area as shown in eq 1. The flow rate and the spray area were constants in this experimental system.
Testing methods
The gram-positive bacteria Bacillus subtilis, gram-negative bacteria Serratia marcescens, and Bacillus spores were used for the killing experiments of microorganisms. Cells were inoculated with a concentration of 107 colony-forming units (CFU)/mL in liquid cultures. Then 0.1 mL cell suspension was transferred onto Petri dish (diameter 60 mm) and distributed evenly in the center. The electric sprayer was placed vertically above the Petri dishes, working with different spray time to get various spray density of •OH radicals. After •OH radical treatment, sterile neutralizer (containing 5 g/L Na2S2O3 and 0.1% peptone) and dilution were added into the Petri dish immediately. The neutralizer was used to neutralize the redundant •OH radicals and other oxygen active particles for controlling the proceeding time. The survival numbers (CFU) of cells were determined by standard plate count method after incubated at 37 °C for 48 hr. Each experiment had three samples for each microorganism. The morphological changes of the microorganisms were also observed under microscope after •OH treatment.
Experimental Results
Effect of •OH concentration on killing efficiency
The effect of •OH concentration on killing efficiency of microorganisms is shown in under the conditions of spraying density of 21 μL/cm2 and processing time of 4 sec. For S. marcescens, the survival cells decreased dramatically when the •OH concentration was above 0.15 mg/L. There was nearly no survival cells when •OH concentration was 0.41 mg/L. Similar results were obtained for B. subtilis cells. The survival B. subtilis cells decreased to undetectable levels when the •OH concentration reached 0.5 mg/L. However, the results are different when Bacillus spores were treated. The survival cell numbers of Bacillus spores dropped significantly when the •OH concentration was greater than 0.3 mg/L, and cells became undetectable when the concentration was increased to 0.8 mg/L. In order to kill all kinds of microorganisms, the •OH concentration of 0.8 mg/L was applied to the following experiments.
Effect of •OH spraying density on killing efficiency
The effect of •OH spraying density on killing efficiency is shown in When the •OH concentration was 0.8 mg/L with the processing time of 4 sec on the infected plate, the CFU numbers of all the cells were decreased very quickly with the increase of •OH spray density from 0 to about 21 μL/cm2. There are three similar curves in , the lethal spraying density of •OH radicals for S. marcescens is 19 μL/cm2, and for B. subtilis and Bacillus spores are 21 μL/cm2.
Effect of •OH processing time on killing efficiency
Since the wide spreading of pathogenic microorganisms is usually very fast, it is important to control the spread within a short time. Therefore, the experiment about the effect of •OH processing time on kill efficiency was carried out, with the •OH concentration of 0.8 mg/L and spray density of 21 μL/cm2. The relationship between processing time and survival cell numbers is shown in One second after •OH treatment, the CFU numbers of B. subtilis decreased dramatically from 105 to about 100, and that of S. marcescens decreased from 105 to about 200. There were no survival cells for them after 3 sec. For Bacillus spores, the CFU numbers quickly dropped down 1 sec after •OH treatment, and then turned to 0 after 4 sec. Although the processing time of bacteria and spores are different, the killing time is very short, just in few seconds. The experimental results indicate that it is a rapid and efficient approach for eliminating pathogenic microorganisms in large air space by spraying •OH radicals.
Morphological changes of microorganisms
The morphological changes of B. subtilis and Bacillus spores with the •OH treatment were also observed under microscope. As shown in , before •OH treatment, the rod-shaped B. subtilis were clearly observed that cells were intact and healthy and the cytoplasm was evenly distributed. After •OH treatment, the membrane integrity was largely lost and their cytoplasm was distributed in clumps (). Compared with B. subtilis, the cell of Bacillus spores with a tough outer coating consists of several layers, such as exosporium, spore coat, cortex, core wall, and so on, so that it has very strong capacity to resist the destruction of bad living conditions (CitationHeninger et al., 2009). Therefore, Bacillus spores is impossible to be rapidly killed by conventional chemical disinfectants such as chlorine, alkali, and alkali-alcolol-amine. However, after •OH treatment, the Bacillus spores were also disrupted, lysed into debris, and the round-shaped cells disappeared ().
Figure 8. Cell micrographs (10 × 40) of Bacillus subtilis (a) and Bacillus spores (b) before and after •OH treatment.
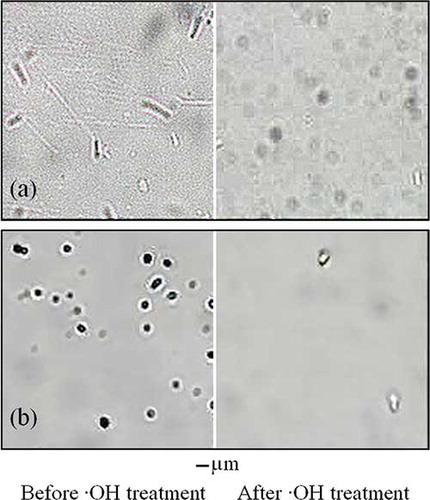
Because of the different cell structure, Bacillus spores are more difficult to be killed than B. subtilis and S. marcescens. Correspondingly, the Bacillus spores need higher •OH killing concentration, spray density, and time. The concentration, spray density, and processing time are the three important parameters for the killing effect of •OH radicals on microorganisms. Treating microorganisms with different cell structures, different concentration, spray density, and processing time of •OH radicals are needed.
Conclusions
A new method was successfully investigated for rapidly eliminating the pathogenic microorganisms in large air space using spraying •OH radicals. The results show that
1. | As a strong oxidant, the •OH radicals kill different microorganisms in low concentration of 0.8 mg/L, and that is equivalent to ten thousandth the dosage of conventional chemical disinfectants (9%). | ||||
2. | With a high reaction rate constant of 109L/mol·sec, the processing time of •OH radicals is very short within 4 sec, which is one thousandth that of chemical disinfectants. | ||||
3. | The •OH spraying density is 22 μL/cm2, which is one thousandth that of other disinfectants. | ||||
4. | Observed under microscope, the •OH radicals caused irreversible damage to cells and ultimately killed them. |
Acknowledgments
The work was supported by Projects of Distinguished Young and General Program from National Natural Science Foundation of China (NSFC: 61025001; 50877005), and National 863 Program from Education Ministry of China (No. 2012AA062609).
References
- Bai , X.Y. , Bai , M.D. , Zhang , Z.T. and Shen , L. 2000a . Development of ozone generation and its application . Chin. J. Nat. , 22 : 347 – 354 .
- Bai , X.Y. , Shen , L. , Bai , M.D. and Zhang , Z.T. 2000b . High concentration ozone produced by strong ionization discharge of dielectric barrier . Physics , 26 : 615 – 619 .
- Bai , M.D. , Bai , X.Y. , Zhang , Z.T. , Bai , M.D. and Yang , B. 2005a . Treatment of red tide in Ocean using non-thermal plasma based advanced oxidation technology . Plasma Chem. Plasma Process. , 25 : 539 – 550 .
- Bai , X.Y. , Zhang , Z.T. , Bai , M.D. and Yang , B. 2005b . Killing of invasive species of ship's ballast water in 20t/h system using hydroxyl radicals . Plasma Chem. Plasma Process. , 25 : 41 – 54 .
- Glaze , W.H. , Kang , J.W. and Chapin , D.H. 1987 . The chemistry of water treatment processes involving ozone, hydrogen peroxide and ultraviolet radiation . Ozone Sci. Eng. , 9 : 335 – 336 .
- Heninger , S. , Christine , A.A. , Gerald , B. and Andrew , B.O. 2009 . Decontamination of Bacillus anthracis spores: Evaluation of various disinfectants . Appl. Biosaf. , 14 : 7 – 10 .
- Jen , J.F. , Leu , M.F. and Yang , T.C. 1998 . Determination of hydroxyl radicals in an advanced oxidation process with salicylic acid trapping and liquid chromatography . J. Chromatogr. A , 796 : 283 – 288 .
- Maurizio , A. and Vincenzo , A. 2005 . Oxidation of oxalate ion in aqueous suspensions of TiO2 by photocatalysis and ozonation . Catal. Today , 107–108 : 612 – 618 .
- Naoyuki , K. and Yukako , M. 2005 . Advanced oxidation effect of ozonation combined with electrolysis . Water Res. , 39 : 4661 – 4672 .
- Nick , S. and Satoshi , H. 2010 . Microwaves in advanced oxidation processes for environmental applications . J. Photochem. Photobiol. C Photochem. Rev. , 11 : 114 – 131 .
- Penetrante , B.M. , Norman , B.J. and Hsial , M.C. 1997 . Kinetic analysis of non-thermal plasma used for pollution control . Japan J. Appl. Phys. , 36 : 5007 – 5017 .
- Quan , X. , Zhang , Y.B. , Chen , S. , Zhao , Y.Z. and Yang , F.L. 2007 . Generation of hydroxyl radical in aqueous solution by microwave energy using activated carbon as catalyst and its potential in removal of persistent organic substances . J. Mol. Catal. A Chem. , 263 : 216 – 222 .
- Raber , E. , Jin , A. , Noonan , K. , McGuire , R. and Kirvel , R.D. 2001 . Decontamination issues for chemical and biological warfare agents: How clean is clean enough? . Int. J. Environ. Health Res. , 11 : 128 – 148 .
- Raber , E. and McGuire , R. 2002 . Oxidative decontamination of chemical and biological warfare agents using L-Gel . J. Hazard. Mater. , 93 : 339 – 352 .
- Roberto , A. and Vincenzo , C. 1999 . Advanced oxidation processes (AOP) for water purification and recovery . Catal. Today , 53 : 51 – 59 .
- Shreya , S. , Boris , D. and Vishal , S. 2007 . Development of a new approach for microbial decontamination of water using modified Fenton's reaction . Environ. Pollut. , 148 : 674 – 678 .
- Smith , R.D. 2006 . Responding to global infectious disease outbreaks: Lessons from SARS on the role of risk perception, communication and management . Soc. Sci. Med. , 63 : 3113 – 3116 .
- Staehelin , J. and Hoigne , J. 1982 . Decomposition of ozone in water: Rate of initiation by hydroxide ion and hydrogen peroxide . Environ. Sci. Technol. , 16 : 676 – 681 .
- Veressinina , G.Y. and Trapido , M. 2008 . Degradation of salicylic acid by Fenton and modified Fenton treatment . Chem. Eng. J. , 143 : 1 – 9 .
- World Health Organization. 2010. Situation updates—Pandemic (H1N1). http://www.who.int/csr/disease/swineflu/updates/en (http://www.who.int/csr/disease/swineflu/updates/en) (Accessed: 10 September 2010 ).
- Xu , X.H. and Zhao , W.R. 2003 . The Ozone Treatment of Water and Wastewater , 84 Beijing : Chemical Industry Press .
- Zhang , Z.T. , Xian , Y.Z. and Bai , M.D. 2003 . Electrical characterization of dielectric barrier discharge plasma using the charge-voltage figure . J. Phys. , 32 : 458 – 462 .