Abstract
The heat shock protein 70 (HSP70) gene family plays a crucial role in the response of organisms to environmental stress. However, it has not been systematically characterized in shrimp. In this study, we identified 25 PcHsp70 genes in the Penaeus chinensis genome. The encoded proteins were categorized into six subgroups based on phylogenetic relationships. Tandem duplication was the main driver of amplification in the PcHsp70 family, and the genes have experienced strong purifying selection during evolution. Transcriptome data analysis revealed that the 25 PcHsp70 members have different expression patterns in shrimp under conditions of low temperature, low salinity, and white spot syndrome virus infection. Among them, PcHsp70.11 was significantly induced under all three stress conditions, suggesting that this gene plays an important role in response to environmental stress in P. chinensis. To the best of our knowledge, this is the first study to systematically analyze the Hsp70 gene family in shrimp. The results provide important information on shrimp Hsp70s, contributing to a better understanding of the role of these genes in environmental stress and providing a basis for further functional studies.
1. Introduction
Heat shock protein 70 (HSP70) is a crucial class of molecular chaperones widely present in various organisms. These proteins maintain cellular functions and respond to environmental stress, thereby safeguarding proteins from denaturation caused by external stimuli and boosting cellular resistance. HSP70s play a significant role in protein folding, depolymerization, and degradation.Citation1 Metazoan cells classify HSP70s into two categories based on their expression patterns: constitutive and inducible.Citation2 Constitutive members are consistently expressed in cells to maintain normal cellular functions, while inducible members are activated in response to environmental stressors and allow cells to adapt to changes in their surroundings.Citation3 The diversity of HSP70 members enables them to display distinct expression patterns under various physiological conditions, external inductions, and different subcellular locations,Citation4 thus fulfilling specific biological functions.
Penaeus chinensis (P. chinensis) is a significant group in aquaculture primarily found in the Bohai and Yellow Seas, with a high commercial value owing to its rich protein content, essential amino acids, unsaturated fatty acids, astaxanthin, and trace elements.Citation5,Citation6 P. chinensis is known for its antioxidant, pro-angiogenic, and anti-inflammatory bioactivities, together with its nutritional and flavor qualities.Citation7,Citation8 However, shrimp growth and production are severely limited by environmental factors, such as unsuitable temperature and salinity, together with pathogenic microorganism infections.Citation9,Citation10 These environmental factors can negatively impact physiological metabolism, growth, reproduction, geographic distribution of production, breeding time, and survival rate. Therefore, understanding the mechanisms by which shrimps adapt to unfavorable environments will be beneficial for cultivation and have positive economic implications.
HSP70 is closely associated with shrimp’s environmental adaptability.Citation11 In Macrobrachium nipponense, Hsp70 expression significantly increases during saline stress,Citation12 and a high HSP70 level is a key adaptation strategy of Penaeus vannamei in low salt concentrations.Citation13 Similarly, early ammonia exposure induces Hsp70 expression in Marsupenaeus japonicusCitation14 and cold conditions trigger a comparable response.Citation15 Moreover, HSP70 is essential in shrimp’s defense against pathogenic infections.Citation16 Injecting rLvHSP70 into Litopenaeus vannamei boosts immunity against pathogenic microbial intrusion.Citation17 This immunoregulatory effect might result from LvHSP70 binding to LvLGBP, which activates the phenoloxidase system, leading to melanin and toxic intermediates production that inhibit pathogens.Citation18 Previous studies also demonstrated the close association between HSP70 and P. chinensis adaptation to the environment, including its role in temperatureCitation10 and salinity adaptationCitation19 and in the process of white spot syndrome virus (WSSV) infection.Citation20 HSP70 plays a key role in the growth and development of organisms and their response to environmental stress. Members of the Hsp70 gene family have been identified and characterized in plants,Citation21 mammals,Citation22 fish,Citation23 shellfish,Citation24 and crabs;Citation25 however, information in shrimp is currently unavailable.
Gaining fundamental knowledge about the Hsp70 gene family and its expression under abiotic and biotic stresses is crucial to comprehending stress adaptation mechanisms and conducting physiological and biochemical studies in shrimp. This study presents the initial comprehensive identification of Hsp70 family members in P. chinensis at the genome level. It provides valuable insights into their physicochemical properties, chromosomal distribution, cellular localization, gene structure, phylogenetic relationships, and expression profiles under abiotic and biotic stress. The findings of this study enhance the understanding of Hsp70 in P. chinensis, offer valuable genetic information, and establish the groundwork for future functional studies and molecular breeding.
2. Material and methods
2.1. Identification and characterization of Penaeus chinensis Hsp70s
The genome files for P. chinensis (assembly ASM1920278v2) and the corresponding protein sequences and their annotations were downloaded from the NCBI database (https://www.ncbi.nlm.nih.gov/genome/?term=Penaeus+chinensis). PcHSP70 was identified through two distinct approaches. First, zebrafish HSP70 sequences were retrieved from the NCBI and Ensembl databases and used as queries in a BLASTP search, with an E-value threshold of e-5. Secondly, the Hidden Markov Model of Hsp70 (PF00012) was employed to perform a targeted scan of the shrimp protein library using Hmmer 3.0 software and the same E-value threshold of e-5. All candidate sequences obtained from both methods were subjected to validation using the NCBI Conserved Domain Database (CDD)Citation26 and the SMART tool.Citation27 The ExPASy platformCitation28 was utilized to analyze various physicochemical properties of PcHSP70s, including theoretical molecular weight, theoretical pI, instability index, aliphatic index, and grand average of hydropathicity. Subcellular localization was determined using WoLF PSORT.Citation29
2.2. Chromosomal localization, gene structural attributes, and phylogenetic analysis of PcHsp70
Conserved motifs were identified using the MEME program,Citation30 with the number of motifs set to 10 and the maximum width revised to 200. Default parameters were used for the remaining settings. The GSDS2.0 programCitation31 was utilized to predict exons, while MG2CCitation32 extracted the chromosomal localization information of PcHsp70s from the annotation file of the P. chinensis genome. An evolutionary tree was constructed using HSP70 protein sequences from humans, mice, mudskippers, yellow croakers, zebrafish, swimming crabs, and the identified P. chinensis HSP70s. These sequences were obtained from NCBI, Ensembl, and UniProt, as listed by Song et al.Citation33 The sequences were aligned using MUSCLE. The phylogenetic tree was constructed with IQtreeCitation34 using 1,000 bootstrap replicates for maximum likelihood (ML) tree construction. The resulting tree was visualized using MEGA 11.Citation35
2.3. Gene duplications, selection pressure analysis and function annotation analysis
In our study, the Gene Ontology (GO) and Kyoto Encyclopedia of Genes and Genomics (KEGG) annotations for PcHSP70s were analyzed using eggnog-MAPPER (Powell, et al., 2014). The collinearity of P. chinensis and Portunus trituberculatus (P. trituberculatus) was analyzed using MCScan X.Citation36 Protein sequences from both species were aligned using the BlastP program, setting the e-value to 1e-10 and max_target_seqs of 5. The resulting alignment files, along with the merged genome annotation files of the two species (gff3), format, were then processed by MCScanX. This analysis helped in identifying segmental and tandem duplication genes. MEGA 11 was utilized to assess the non-synonymous substitution rate (Ka) and synonymous substitution rate (Ks), and the Ka/Ks ratio was calculated for the homologous gene pairs. The divergence times of P. chinensis were calculated using the following reference formula: T = Ks/(2 × 2 × 10−9) × 10−6,Citation37,Citation38 expressed in million years ago (Mya).
2.4. Hsp70 expression analysis
The expression profiles of PcHsp70 genes under biotic and abiotic stresses were determined using RNA-Seq data obtained from three distinct stress conditions: temperature stress (accession number: PRJNA683682), salt stress (accession number: PRJNA788096), and WSSV infection (accession number: PRJNA788096) from the Sequence Read Archive (SRA) database. These RNA-Seq data and the treatment details of P. chinensis under temperature stress, salt stress, and WSSV infection were reported by Zhang et al.,Citation39,Citation40 Shen et al.Citation41 and Meng et al.,Citation39,Citation40 respectively. Data quality control, generation of BAM files, and abundance calculations were performed using Trimmomatic, SAMtools, and StringTie, respectively. The (FPKM + 1) value normalized by log2 transformation was used to construct a heat map and visualized using Tbtools.Citation42 Additionally, the expression values were averaged within the same replicate experimental groups. A fold-change ≥ 2 between the stress and control groups was considered statistically significant for determining differential gene expression.
3. Results
3.1. Identification of Hsp70 genes
A total of 25 PcHsp70 genes of P. chinensis were obtained and named according to the order of their location on the chromosomes (). The PcHSP70 proteins ranged from 151 (PcHSP70.19, 17025.55KDa) to 1433 (PcHSP70.18, 158564.07KDa) amino acids (aa) in length. The predicted isoelectric point (PI) values of PcHSP70 protein ranged from 4.98 to 6.27. Two of the 25 PcHSP70 proteins (PcHSP70.4 and PcHSP70.6) were predicted to be unstable (cutoff > 40), and only PcHSP70.6 was a hydrophobic protein (grand average of hydropathicity index < 0). Most of the PcHSP70 proteins were predicted to be located in the cytoplasm. PcHSP70.10, PcHSP70.11, PcHSP70.12, and PcHSP70.21 were localized in the cytoplasm and nucleus. Additionally, two members (PcHSP70.9 and PcHSP70.22) were localized in the endoplasmic reticulum, while one member (PcHSP70.18) was exclusively found in the nucleus, and another member (PcHSP70.23) was specifically localized in the mitochondria.
Table 1. Basic information on Hsp70 family members in Penaeus chinensis.
3.2. Phylogenetic relationship analysis of PcHSP70 proteins
The HSP70 in swimming crabs exhibits the closest evolutionary relationship to PcHSP70 (). Unlike the vertebrate Hsp70 gene family, both P. chinensis and swimming crab belong to the Decapoda and contain Hsc70l, Hspa5, Hspa9, Hspa14, Hyou1, and Hsph1 members but lack Hsp70a1, Hspa2, Hspa4, Hspa8, Hspa12, and Hspa13 members. Additionally, there is a distinct branch consisting of five Hsp70 members (PcHsp70.5, PcHsp70.7, PcHsp70.8, PcHsp70.24, and PcHsp70.25) within the PcHsp70s that require further exploration to determine their specific functions.
Figure 1. Phylogenetic tree of 123 HSP70 proteins from seven species. The different colors of the dots represent different species. The varying colors in the outer ring region denote the six HSP70 categories (Hsc70l, Hspa5, Hspa9, Hspa14, Hyou1, and Hsph1) to which the 25 PcHSP70 members are classified.
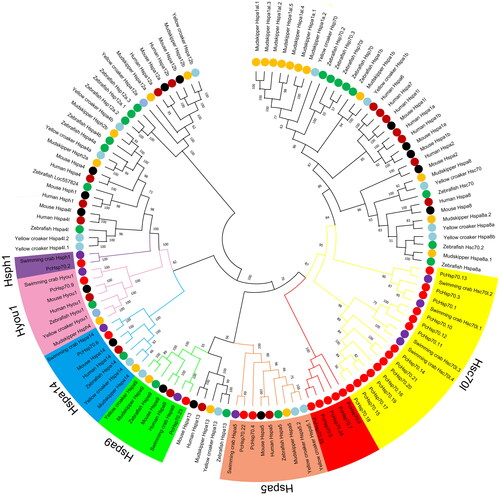
3.3. Conserved motifs, CDD domain, and gene structure of PcHsp genes
The 25 PcHsp70 proteins were grouped into one diagram with conserved motifs (), CDD domains (), and gene structures () to better understand the evolution conservation of the protein family. Fifteen motifs were identified in PcHSP70s; 12 of these motifs belonged to the HSP70 domain except for motifs 11, 12, and 13. Most members with closer phylogenetic relationships shared similar motifs. Among them, PcHSP70.3 contained the highest number of motifs (13), while PcHSP70.4 had only six. All 25 PcHSP70 members contained the conserved Hsp70 protein functional domain. PcHsp70.1, 3, 10, 11, 12, 13, 14, 15, 16, 18, 20, and 21 of the Hsc70l branch contained the HSPA1-2_6-8-like_NBD functional domain, while PcHsp70.17 and PcHsp70.19 did not. Additionally, PcHsp70.2, PcHsp70.9, PcHsp70.22, and PcHsp70.23 contained the HSPA4-like_NBD, HSPA9-like_NBD, HSPA5-like_NBD, and HSPA9-like_NBD functional domains, respectively, corresponding to branches of the evolutionary tree (). PcHsp4, 5, 7, 8, 10, 11, 12, 19, 24, and 25 did not contain introns, while PcHsp2 (16), 6 (10), 9 (18), and 23 (13) had a relatively higher number of introns. In addition, it should be noted that PcHsp70.18 displayed duplications across conserved motifs, CDD structural domains, and gene structures, suggesting the possible presence of two distinct genes, which is most likely caused by the relatively difficult gene assembly due to the complexity of the P. chinensis genome.Citation37
Figure 2. Schematic diagram of conserved motif (a), CDD domains (b), and gene structure (c) of PcHsp70s. For (a), the rectangles of different colors represent conservative base sequences. For (b), the colored bars signify conservative structural domains. For (c), green denotes 5’ and 3’ UTR regions; yellow signifies exons; black lines correspond to introns.
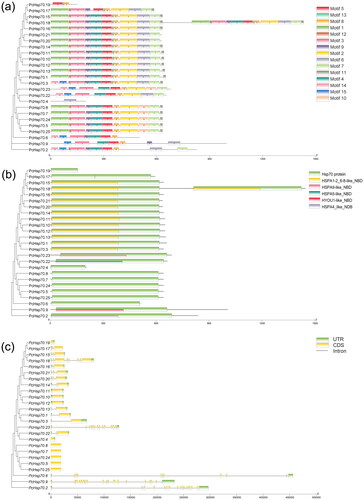
3.4. Chromosomal location and gene duplication analysis of PcHsp70 genes
Twenty-four PcHsp70 genes were irregularly distributed on 11 chromosomes, while PcHsp70.25 was located on the scaffold (). Most of these genes were scattered at both ends of the chromosome, except for PcHsp70.1, 9, 13, 22, and 23, which were concentrated in the middle of the chromosome. Chromosome 36 (Chr36) contained the highest number of PcHsp70s (8), followed by Chr19 and Chr25, each harboring three members.
Figure 3. Localization and tandem duplications of the PcHsp70 gene on chromosomes. Orange denotes chromosome or scaffold numbers, red represent the PcHsp70 genes, and red short lines connect between tandem duplication gene pairs.
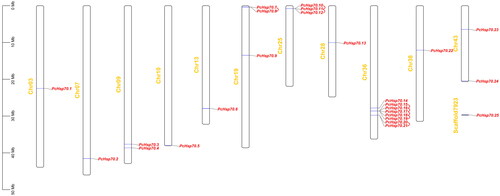
Collinearity relationships were analyzed to investigate the gene duplication events within the PcHsp70 family and between PcHsp70s and PtHsp70s. Eight pairs of tandem duplication genes were identified, with five pairs (PcHsp70.15/PcHsp70.16, PcHsp70.16/PcHsp70.17, PcHsp70.17/PcHsp70.18, PcHsp70.18/PcHsp70.19, and PcHsp70.20/PcHsp70.21) anchored on Chr36, and the remaining three pairs distributed on Chr19 (PcHsp70.7/PcHsp70.8) and Chr25 (PcHsp70.10/PcHsp70.11 and PcHsp70.11/PcHsp70.12) (, ). Only one pair of homologous genes (PcHsp70.22/PtHspa5) was found between P. chinensis and P. trituberculatus (, ). Ka and Ks values were calculated to obtain information about the selection pressure and evolutionary dates of these homologous gene pairs (). Except for PcHsp70.18/PcHsp70.19, which had a Ka/Ks value of 0.5432, all other homologous gene pairs had values below 0.5, indicating that PcHsp70 genes underwent strong purifying selection during the evolutionary process. The duplication events occurred approximately between 0.54 and 3.93 Mya for these PcHsp70 pair genes (). The divergence time for the PcHsp70.22/PtHspa5 pair was approximately 309 Mya.
Figure 4. Collinearity analysis of Hsp70 genes between Penaeus chinensis and Portunus trituberculatus. Chromosomes of Penaeus chinensis are depicted in orange, while those of Portunus trituberculatus are shown in green. The solitary pair of HSP70 homologous genes is linked by a red line.

Table 2. Ka/Ks Values and divergence date of Hsp70 duplicated genes in Penaeus chinensis and between Penaeus chinensis and Portunus trituberculatus.
3.5. Functional annotations of PcHsp70
To gain insights into the role of PcHsp70 in the life activities and metabolic processes of P. chinensis, we employed GO and KEGG enrichment analysis. The GO functional analysis indicated that 25 PcHsp70 genes were significantly enriched in 273 GO terms (p < 0.05), encompassing 222 biological processes (BP), 26 cellular components (CC), and 25 molecular functions (MF). illustrates the top 10 most significant terms from each of these categories. In the BP category, PcHSP70 primarily participated in processes such as DNA and RNA processing (GO:0006396) and protein folding (GO:0006457). Regarding the CC category, it predominantly participated in organelles, chromatin, and cytoplasmic components. In the MF category, PcHsp70 was primarily associated with unfolding protein or protein binding (GO:0051082 and GO:0005515) and chaperone binding (GO:0051087), along with regulating certain enzyme and ATP activities.
Figure 5. The GO enrichment analysis of PcHsp70 genes in Penaeus chinensis. Here, BP, CC, and MF are acronyms denoting biological processes, cellular components, and molecular functions, respectively. The color of the circles represents the magnitude of the p-value, with green indicating a larger value and red signifying a smaller value. The size of the circle is directly proportional to the enrichment count.
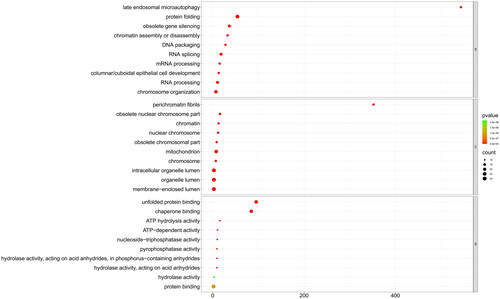
KEGG analysis further showed that these 25 PcHsp70 genes were significantly enriched in 41 KEGG pathways (p < 0.05), spanning six main classes: genetic information processing, environmental information processing, cellular processes, organismal systems, human diseases, and brite hierarchies (). These findings underscore the diverse biological roles of PcHsp70 genes in P. chinensis.
3.6. Expression patterns of PcHsp70 genes
We analyzed the PcHsp70 gene expression patterns to better understand the role of PcHSP70 gene family members in response to environmental stress in P. chinensis (temperature stress, salinity stress, and WSSV infection). PcHsp70s displayed the highest sensitivity to low-temperature stress among the three stress conditions (). Six out of 25 members were significantly upregulated (PcHsp70.1, 10, 11, 12, 20, and 21), wherein PcHsp70.10, PcHsp70.11, and PcHsp70.12 were significantly induced (). PcHsp70.11 expression was significantly elevated in a low-salt environment (), while the expression of the other members showed no significant differences. Low concentrations of WSSV resulted in significant suppression of PcHsp70.9 and PcHsp70.22 expression and significant upregulation of PcHsp70.10 and PcHsp70.11 expression (). Meanwhile, high concentrations of WSSV infection reduced the degree of upregulation of PcHsp70.10 and PcHsp70.11. Furthermore, PcHsp70.5, 7, 8, 24, and 25, which share relatively close phylogenetic relationships (), exhibited minimal expression levels under all examined conditions ().
Figure 7. The expression pattern of PcHsp70 genes under low-temperature (a), low-saline (b), and WSSV-infected (c) treatments. For the low-temperature treatment, ‘a_28 °C’ represents breeding post-larval samples subjected to a normal temperature treatment (28 °C), while ‘a_4 °C’ symbolizes breeding post-larval samples exposed to a low-temperature treatment (4 °C). ‘b_28 °C’ signifies wild post-larval samples under normal temperature conditions (28 °C), and ‘b_4 °C’ pertains to wild post-larval samples under low-temperature conditions (4 °C). For the low-saline treatment, ‘25 PPT’ denotes a salinity concentration of 25 parts per thousand and ‘10 PPT’ signifies a salinity concentration of 10 parts per thousand. In the context of the WSSV-infected treatment, ‘control’ represents the healthy group, ‘low’ corresponds to the low WSSV load group (3.04 × 10−4 ±1.20 × 10−4), and ‘high’ stands for the high WSSV load group (4.32 × 10−1±1.62 × 10−2). Color coding indicates expression levels, with red denoting high expression and blue representing low expression. The numerical values signify the normalized expression quantities.
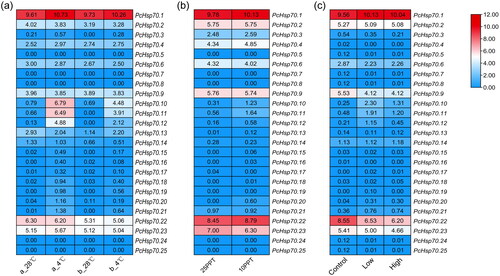
4. Discussion
HSP70 plays a crucial role in maintaining normal physiological and biochemical functions of cells during the adaptation of organisms to environmental changes. The Hsp70 gene family has been identified and characterized in various aquatic organisms but has not been systematically studied in shrimp. In this study, we identified the Hsp70 genes in P. chinensis, analyzed their evolutionary relationships and sequence features, and explored their functions and the physiological and biochemical pathways involved. Furthermore, we analyzed their expression patterns in response to cold stress, low-salt stress, and WSSV infection.
All eukaryotes contain multiple genes encoding different HSP70s.Citation43 For example, humans have 13 Hsp70 subfamilies, including Hspa1a, Hspa1b, Hspa2, Hspa4, Hspa5, Hspa6, Hspa7, Hspa8, Hspa9, Hspa12a, Hspa12b, Hspa13, and Hspa14.Citation44 In this study, the 25 Hsp70 genes identified from the P. chinensis genome were classified into six subfamilies (Hsc70l, Hspa5, Hspa9, Hspa14, Hyou1, and Hsph1); this is significantly fewer than that in fish, humans, and mice () and consistent with the number of Hsp70 subgroups in P. trituberculatus,Citation25 which is also a decapod. This result confirmed that the rate of gene family member loss in invertebrates during evolution is higher than that in vertebrates.Citation45 Moreover, there is a significant difference in the number of Hsp70 family members between different species. P. chinensis have more Hsp70 members than crabs (9)Citation25 and fish, such as Scophthalmus maximus (16)Citation46 and Lateolabrax maculatus (17),Citation23 but much fewer than bivalves, such as Mercenaria mercenaria (133)Citation47 and Crassostrea gigas (88).Citation48 HSP70 is one of the most ancient and conserved proteins during evolution.Citation49–51 The great disparity in the type and number of Hsp70 in different species indicates that these genes have undergone extensive loss or duplication events after species differentiation. These results suggest that the retention and number of gene subfamily members are closely related to the evolutionary history of the species. Changes in exon-intron distribution patterns promote gene evolution.Citation52 PcHsp70 members have either no introns, few and short introns, or many and long introns. The distribution pattern of the PcHsp70 gene structure was similar to that of P. trituberculatus.Citation25 This finding suggested that Hsp70 in shrimps and crabs probably underwent a similar evolutionary process, and the evolution of this distribution pattern was preserved before the divergence of shrimps and crabs. The sister members whose protein sequences are clustered on the same branch (except for PcHsp70.17/PcHsp70.19 and PcHsp70.4/PcHsp70.22/PcHsp70.23) have extremely similar exon-intron distribution patterns, similar protein motifs, and very similar or even consistent physicochemical constants, indicating a similar function and genetic evolutionary conservation among these sister members.Citation53
Gene duplication is an important driver of gene evolution because it provides the raw material and potential for creating new genes and functions.Citation54 In this study, nearly half of the 25 PcHsp70 members (12) involved eight tandem repeat events, with no other types of homologous gene pairs detected; this suggests that tandem duplication is the main driving force for the expansion of the PcHsp70 gene family. These homologous gene pairs have highly similar intron numbers and lengths and are highly concentrated on the chromosome. Therefore, PcHsp70s underwent a replication event at a relatively recent time in their evolution. We further analyzed the divergence time of PcHsp70, which revealed that the replication event occurred approximately 0.54-35.19 Mya, confirming that the expansion of the PcHSP70 gene family reflects a relatively recent duplication event. In addition, collinearity analysis on the Hsp70 genes of P. chinensis and P. trituberculatus identified one gene pair (PcHsp70.22/PtHspa5), and their homology occurred at 309.25 Mya. These two genes are localized in the endoplasmic reticulum and are the only homologous gene pair within the HSP70 gene family present in both species. This finding implies that these two genes have not undergone any duplication events since diverging from a common ancestor and also demonstrates their extreme conservation and important function during evolution. The results of selective pressure analysis also indicated that PcHsp70.22/PtHspa5 underwent a strong purifying selection (ka/ks value of 0.035) process during the evolutionary history that helped them maintain their gene functions.Citation55 Meanwhile, the ka/ks value was below 0.5 for all genes except for PcHsp70.18/PcHsp70.19, which had a ka/ks value slightly greater than 0.5; this indicated that the PcHsp70 gene family underwent strong negative selection during the evolutionary process and further illustrated the evolutionary conservation of Hsp70 function.
Biotic or abiotic stress can lead to misfolding of cellular proteins,Citation56,Citation57 and the excessive accumulation of these proteins can cause toxic damage to cells.Citation58 HSP70 plays a crucial role in how organisms respond to these environmental pressures. HSP70 functions through the regulation of the ATP-ADP cycle; the ADP that is produced when ATP is hydrolyzed binds to substrates such as newly synthesized polypeptides and misfolded proteins, resulting in conformational changes and stabilization in a foldable state. Meanwhile, regulation by co-chaperone proteins (such as nucleotide exchange factors and J proteins) results in the regeneration of ATP, with the release of substrate proteins and the beginning of standard folding, degradation, or proteins becoming targeted for transport;Citation59,Citation60 this process can effectively reduce the accumulation of misfolded proteins. Therefore, regulating the expression of Hsp70 family members can help maintain the normal function of cells to regulate the response of organisms under environmental stress. The results of transcriptome data indicate that six and four of the 25 PcHsp70 members are significantly upregulated at 10 and 40 days after larval development, respectively. Among these, PcHsp70.10, PcHsp70.11, and PcHsp70.12 were significantly upregulated, implying that these members may play a crucial role in the prawns’ response to low-temperature stress. PcHsp70.11 was significantly induced during low salt stress, while the expression levels of homologous genes PcHsp70.10 (log2FC:0.92) and PcHsp70.12 (log2FC:0.42) were also elevated but not significantly. The expression of PcHsp70.9 (Hyou1) and PcHsp70.22 (Hspa5) was significantly inhibited when shrimps were subjected to low concentrations of WSSV infection for 96 h, while the expression of PcHsp70.10 and PcHsp70.11 significantly increased. However, PcHsp70.10 and PcHsp70.11 were insignificantly upregulated when the shrimps were infected with a high concentration of WSSV.
Notably, PcHsp70.9 (Hyou1) and PcHsp70.22 (Hspa5) (located on the endoplasmic reticulum as chaperone proteins) showed an increase in expression during the infection process of some pathogens.Citation61,Citation62 Their expression levels significantly decreased after 96 h of WSSV infection in shrimps. Similarly, previous research pointed out that the expression levels of Hyou1 and Hspa5 in the gills of catfish infected with Flavobacterium columnare increased at 48 h but rapidly decreased at 72 h;Citation33 the reason for this may be that a large number of misfolded proteins are produced as the stimulation time of pathogen on cells extends and exceeds the ability of the degradation system to remove the misfolded proteins. This induces excessive endoplasmic reticulum stress, which elicits and amplifies tissue pathology and reduces protein expression within the endoplasmic reticulum.Citation63 The significant downregulation of PcHsp70.9 (Hyou1) and PcHsp70.22 (Hspa5) expression in this study may be related to the longer infection time. Additionally, PcHsp70.10, PcHsp70.11, and PcHsp70.12 are three homologous genes that may be induced to varying degrees under stress conditions. In particular, PcHsp70.11 expression was significantly upregulated under all three stress conditions in the transcriptome analysis, which indicated that this gene is more sensitive to environmental change and that it may play a vital role in the prawn’s response to environmental stress. However, this inference requires further empirical validation through specific experiments.
5. Conclusion
In this study, we identified 25 Hsp70 genes on 11 chromosomes and one scaffold of the shrimp genome. They were divided into six subgroups based on their phylogenetic relationships. Members clustered on the same evolutionary branch had similar base sequences and gene structures and exhibited similar expression patterns when faced with environmental stress. Furthermore, PcHsp70.11 was significantly induced in P. chinensis under low temperature, low salinity, and WSSV infection stress, making it a promising target for subsequent PcHsp70 functional studies. In addition, those located in the endoplasmic reticulum also exhibited significant differences when P. chinensis was subjected to WSSV infection. This finding indicated that they were involved in the P. chinensis-WSSV interaction. This study provides important information on the Hsp70 gene family in P. chinensis and helps understand the role of these genes under environmental stress, thus laying the groundwork for further functional studies.
Disclosure statement
No potential conflict of interest was reported by the author(s).
Additional information
Funding
References
- Zuiderweg ER, Hightower LE, Gestwicki JE. The remarkable multivalency of the Hsp70 chaperones. Cell Stress Chaperones. 2017;22(2):173–189.
- Yu EM, Yoshinaga T, Jalufka FL, Ehsan H, Mark Welch DB, Kaneko G. The complex evolution of the metazoan HSP70 gene family. Sci Rep. 2021a;11(1):17794.
- Chong KY, Lai CC, Su CY. Inducible and constitutive HSP70s confer synergistic resistance against metabolic challenges. Biochem Biophys Res Commun. 2013;430(2):774–779.
- Rosenzweig R, Nillegoda NB, Mayer MP, Bukau B. The Hsp70 chaperone network. Nat Rev Mol Cell Biol. 2019;20(11):665–680.
- Lyu D, Sun S, Shan X, et al. Genetic diversity monitoring of Fenneropenaeus chinensis in the Bohai Sea in the past decade: a study on the effect of release on the natural population. Reg. Stud. Mar. Sci. 2023;61:102823.
- Xie B, Zhang M, Yang H, Jiang W. Effects of conventional versus organic production systems on amino acid profiles and heavy metal concentrations in the Chinese shrimp Penaeus chinensis. Fish Sci. 2011;77(5):839–845.
- Kim YS, Kim EK, Ryu BI, et al. Antioxidant activity of extract from the cephalothorax of Fenneropenaeus chinensis. Adv. Exp. Med. Biol. 2017;975:1153–1163.
- Zhu Y, Li P, Meng R, et al. Lipid profiles of the heads of four shrimp species by UPLC-Q-Exactive Orbitrap/MS and their cardiovascular activities. Molecules. 2022;27(2):350.
- Millard RS, Ellis RP, Bateman KS, et al. How do abiotic environmental conditions influence shrimp susceptibility to disease? A critical analysis focussed on white spot disease. J Invertebr Pathol. 2021;186:107369.
- Ren X, Wang Q, Shao H, Xu Y, Liu P, Li J. Effects of low temperature on shrimp and crab physiology, behavior, and growth: a review. Front Mar Sci. 2021;8:746177.
- Jeyachandran S, Chellapandian H, Park K, Kwak I-S. A review on the involvement of heat shock proteins (extrinsic chaperones) in response to stress conditions in aquatic organisms. Antioxidants . 2023;12(7):1444.
- Xue C, Xu K, Jin Y, Bian C, Sun S. Transcriptome analysis to study the molecular response in the gill and hepatopancreas tissues of Macrobrachium nipponense to salinity acclimation. Front Physiol. 2022;13:926885.
- Ye Y, Zhu B, Zhang J, et al. Comparison of growth performance and biochemical components between low-salinity-tolerant hybrid and normal variety of pacific white shrimp (Penaeus vannamei). Animals . 2023;13(18):2837.
- Ou H, Liang J, Liu J. Effects of acute ammonia exposure on oxidative stress, endoplasmic reticulum stress and apoptosis in the kuruma shrimp (Marsupenaeus japonicus). Aquacult. Rep. 2022;27:101383.
- Ren X, Lv J, Liu M, et al. A chromosome-level genome of the kuruma shrimp (Marsupenaeus japonicus) provides insights into its evolution and cold-resistance mechanism. Genomics. 2022;114(3):110373.
- Mengal K, Kor G, Kozák P, Niksirat H. Heat shock proteins adaptive responses to environmental stressors and implications in health management of decapods. Aquacult. Rep. 2023;30:101564.
- Junprung W, Supungul P, Tassanakajon A. Litopenaeus vannamei heat shock protein 70 (LvHSP70) enhances resistance to a strain of Vibrio parahaemolyticus, which can cause acute hepatopancreatic necrosis disease (AHPND), by activating shrimp immunity. Dev Comp Immunol. 2019;90:138–146.
- Junprung W, Supungul P, Sangklai N, Tassanakajon A. Heat shock protein 70 is a damage-associated molecular pattern that by binding to lipopolysaccharide and β-1,3-glucan-binding protein activates the prophenoloxidase system in shrimp. J Immunol. 2022;209(3):582–592.
- Deng Z, Zhang Z, Zhao R, et al. Effects of high-salinity on the expression of aquaporins and ion transport-related genes in Chinese shrimp (Fenneropenaeus chinensis). Aquacult. Rep. 2023;30:101577.
- Cui C, Liang Q, Tang X, Xing J, Sheng X, Zhan W. Differential apoptotic responses of hemocyte subpopulations to white spot syndrome virus infection in Fenneropenaeus chinensis. Front Immunol. 2020;11:594390.
- He Q, Zhang X, He M, et al. Genome-wide characterization of RsHSP70 gene family reveals positive role of RsHSP70-20 gene in heat stress response in radish (Raphanus sativus L.). Plant Physiol Biochem. 2023;199:107710.
- Tripathy K, Sodhi M, Kataria RS, Chopra M, Mukesh M. In silico analysis of Hsp70 gene family in bovine genome. Biochem Genet. 2021;59(1):134–158.
- Sun Y, Wen H, Tian Y, et al. HSP90 and HSP70 families in Lateolabrax maculatus: genome-wide identification, molecular characterization, and expression profiles in response to various environmental stressors. Front Physiol. 2021;12:784803.
- Gao Y, Li JN, Pu JJ, Tao KX, Zhao XX, Yang QQ. Genome-wide identification and characterization of the HSP gene superfamily in apple snails (Gastropoda: Ampullariidae) and expression analysis under temperature stress. Int J Biol Macromol. 2022;222(Pt B):2545–2555.
- Jin S, Deng Z, Xu S, Zhang H, Han Z. Genome-wide identification and low-salinity stress analysis of the Hsp70 gene family in swimming crab (Portunus trituberculatus). Int J Biol Macromol. 2022;208:126–135.
- Wang J, Chitsaz F, Derbyshire MK, et al. The conserved domain database in 2023. Nucleic Acids Res. 2023;51(D1):D384–D388.
- Letunic I, Khedkar S, Bork P. SMART: recent updates, new developments and status in 2020. Nucleic Acids Res. 2021;49(D1):D458–D460.
- Wilkins MR, Gasteiger E, Bairoch A, et al. Protein identification and analysis tools in the Expasy server. Methods Mol Biol. 1999;112:531–552.
- Horton P, Park KJ, Obayashi T, et al. WoLF PSORT: protein localization predictor. Nucleic Acids Res. 2007;35(Web Server issue):W585–W587.
- Bailey TL, Johnson J, Grant CE, Noble WS. The MEME suite. Nucleic Acids Res. 2015;43(W1):W39–W49.
- Hu B, Jin JP, Guo AY, Zhang H, Luo J, Gao G. GSDS 2.0: an upgraded gene feature visualization server. Bioinformatics. 2015;31(8):1296–1297.
- Chao J, Li Z, Sun Y, et al. MG2C: a user-friendly online tool for drawing genetic maps. Mol Horticulture. 2021;1(1):16.
- Song L, Li C, Xie Y, et al. Genome-wide identification of Hsp70 genes in channel catfish and their regulated expression after bacterial infection. Fish Shellfish Immunol. 2016;49:154–162.
- Minh BQ, Schmidt HA, Chernomor O, et al. Corrigendum to: IQ-TREE 2: new models and efficient methods for phylogenetic inference in the genomic era. Mol Biol Evol. 2020;37(8):2461–2461.
- Tamura K, Stecher G, Kumar S. MEGA11: molecular evolutionary genetics analysis version 11. Mol Biol Evol. 2021;38(7):3022–3027.
- Wang Y, Tang H, Debarry JD, et al. MCScanX: a toolkit for detection and evolutionary analysis of gene synteny and collinearity. Nucleic Acids Res. 2012;40(7):e49–e49.
- Yuan J, Zhang X, Li F, Xiang J. Genome sequencing and assembly strategies and a comparative analysis of the genomic characteristics in penaeid shrimp species. Front Genet. 2021b;12:658619.
- Yuan J, Zhang X, Wang M, et al. Simple sequence repeats drive genome plasticity and promote adaptive evolution in penaeid shrimp. Commun Biol. 2021;4(1):186.
- Li XP, Luan S, Luo K, et al. Comparative transcriptomic analysis of Chinese shrimp Fenneropenaeus chinensis infected with white spot syndrome virus. Aquacult. Rep. 2022a;22:100986.
- Li Y, Jin Y, Wang J, Ji G, Zhang X. Significant genes in response to low temperature in Fenneropenaeus chinensis screened through multiple transcriptome group comparisons. J Therm Biol. 2022b;107:103198.
- Liu J, Zhang D, Zhang L, Wang Z, Shen J. New insight on vitality differences for the penaeid shrimp, Fenneropenaeus chinensis, in low salinity environment through transcriptomics. Front Ecol Evol. 2022;10:716018.
- Chen C, Chen H, Zhang Y, et al. Tbtools: an integrative toolkit developed for interactive analyses of big biological data. Mol Plant. 2020;13(8):1194–1202.
- Stricher F, Macri C, Ruff M, Muller S. HSPA8/HSC70 chaperone protein: structure, function, and chemical targeting. Autophagy. 2013;9(12):1937–1954.
- Kampinga HH, Hageman J, Vos MJ, et al. Guidelines for the nomenclature of the human heat shock proteins. Cell Stress Chaperones. 2009;14(1):105–111.
- Hughes AL, Friedman R. Differential loss of ancestral gene families as a source of genomic divergence in animals. Proc Biol Sci. 2004;271 Suppl 3(Suppl 3):S107–S109.
- Zheng W, Xu X, Chen Y, et al. Genome-wide identification, molecular characterization, and involvement in response to abiotic and biotic stresses of the hsp70 gene family in turbot (Scophthalmus maximus). Int J Mol Sci. 2023;24(7):6025.
- Hu Z, Song H, Feng J, et al. Massive heat shock protein 70 genes expansion and transcriptional signatures uncover hard clam adaptations to heat and hypoxia. Front Mar Sci. 2022;9:898669.
- Zhang G, Fang X, Guo X, et al. The oyster genome reveals stress adaptation and complexity of shell formation. Nature. 2012;490(7418):49–54.
- Chen S, Qiu G. Overexpression of Zostera japonica heat shock protein gene ZjHsp70 enhances the thermotolerance of transgenic Arabidopsis. Mol Biol Rep. 2022;49(7):6189–6197.
- Gupta RS, Golding GB. Evolution of HSP70 gene and its implications regarding relationships between archaebacteria, eubacteria, and eukaryotes. J Mol Evol. 1993;37(6):573–582.
- Yang J, Zhang H, Gong W, et al. S-Glutathionylation of human inducible Hsp70 reveals a regulatory mechanism involving the C-terminal α-helical lid. J Biol Chem. 2020;295(24):8302–8324.
- Long M, VanKuren NW, Chen S, Vibranovski MD. New gene evolution: little did we know. Annu Rev Genet. 2013;47(1):307–333.
- Roy SW, Gilbert W. The evolution of spliceosomal introns: patterns, puzzles and progress. Nat Rev Genet. 2006;7(3):211–221.
- Magadum S, Banerjee U, Murugan P, Gangapur D, Ravikesavan R. Gene duplication as a major force in evolution. J Genet. 2013;92(1):155–161.
- Tanaka KM, Takahasi KR, Takano-Shimizu T. Enhanced fixation and preservation of a newly arisen duplicate gene by masking deleterious loss-of-function mutations. Genet Res . 2009;91(4):267–280.
- Devi S, Chaturvedi M, Fatima S, Priya S. Environmental factors modulating protein conformations and their role in protein aggregation diseases. Toxicology. 2022;465:153049.
- Walker AC, Bhargava R, Dove AS, Brust AS, Owji AA, Czyż DM. Bacteria-derived protein aggregates contribute to the disruption of host proteostasis. Int J Mol Sci. 2022;23(9):4807.
- Gonzalez-Garcia M, Fusco G, De Simone A. Membrane interactions and toxicity by misfolded protein oligomers. Front Cell Dev Biol. 2021;9:642623.
- Aragonès Pedrola JP, Rüdiger SGD. Double J-domain piloting of an Hsp70 substrate. J Biol Chem. 2021;296:100717.
- Clerico EM, Tilitsky JM, Meng W, Gierasch LM. How hsp70 molecular machines interact with their substrates to mediate diverse physiological functions. J Mol Biol. 2015;427(7):1575–1588.
- Causey DR, Pohl MAN, Stead DA, Martin SAM, Secombes CJ, Macqueen DJ. High-throughput proteomic profiling of the fish liver following bacterial infection. BMC Genomics. 2018;19(1):719.
- Zhu J, Fu Q, Ao Q, et al. Transcriptomic profiling analysis of tilapia (Oreochromis niloticus) following Streptococcus agalactiae challenge. Fish Shellfish Immunol. 2017;62:202–212.
- You K, Wang L, Chou CH, et al. QRICH1 dictates the outcome of ER stress through transcriptional control of proteostasis. Science. 2021;371(6524):eabb6896.