Abstract
Tropomyosin 3 (TPM3) plays a significant role as a regulatory protein in muscle contraction, affecting the growth and development of skeletal muscles. Despite its importance, limited research has been conducted to investigate the influence of TPM3 on bovine skeletal muscle development. Therefore, this study revealed the role of TPM3 in bovine myoblast growth and development. This research involved conducting a thorough examination of the Qinchuan cattle TPM3 gene using bioinformatics tools to examine its sequence and structural characteristics. Furthermore, TPM3 expression was evaluated in various bovine tissues and cells using quantitative real-time polymerase chain reaction (qRT-PCR). The results showed that the coding region of TPM3 spans 855 bp, with the 161st base being the T base, encoding a protein with 284 amino acids and 19 phosphorylation sites. This protein demonstrated high conservation across species while displaying a predominant α-helix secondary structure despite being an unstable acidic protein. Notably, a noticeable increase in TPM3 expression was observed in the longissimus dorsi muscle and myocardium of calves and adult cattle. Expression patterns varied during different stages of myoblast differentiation. Functional studies that involved interference with TPM3 in Qinchuan cattle myoblasts revealed a very significantly decrease in S-phase cell numbers and EdU-positive staining (P < 0.01), and disrupted myotube morphology. Moreover, interference with TPM3 resulted in significantly (P < 0.05) or highly significantly (P < 0.01) decreased mRNA and protein levels of key proliferation and differentiation markers, indicating its role in the modulation of myoblast behavior. These findings suggest that TPM3 plays an essential role in bovine skeletal muscle growth by influencing myoblast proliferation and differentiation. This study provides a foundation for further exploration into the mechanisms underlying TPM3-mediated regulation of bovine muscle development and provides valuable insights that could guide future research directions as well as potential applications for livestock breeding and addressing muscle-related disorders.
Introduction
Skeletal muscle accounts for 40% of body weight,Citation1 and its growth and development greatly affect the meat production and meat quality of livestock.Citation2 The growth and development of skeletal muscle include the proliferation and fusion of myoblasts, the differentiation of muscle tubes, and eventually the formation of a large number of mature muscle fibers.Citation3 Thus, studying the specific regulatory mechanisms of myoblast proliferation and differentiation is very important. However, myoblast proliferation and differentiation are complex biological processes regulated by multiple genes, signaling pathways, and non-coding RNAs.Citation4,Citation5 Polygenes include the Pax protein family, MRFs (myogenic regulatory factors) family, MEF2 family, and so on. Numerous studies have confirmed that MRFs including Myf5, MyoD, myogenin, and MRF4, are involved in the regulation of myoblast proliferation and differentiation.Citation6 In recent years, myosin has been found to participate in the proliferation and differentiation of muscle cells. For example, interference with the MYH3 (myosin heavy chain 3) gene can promote the proliferation of bovine myoblasts and inhibit their differentiation.Citation7 In addition, myosin heavy chain transcription is also regulated by the MRF protein family.Citation8 The tropomyosin (TPM) family is widely distributed in eukaryotic cells and is an important part of microfilaments, mainly involved in the contraction of skeletal muscle and smooth muscle cells or the maintenance of cytoskeleton stability in non-muscle cells.Citation9 The TPM family consists of four genes: TPM1, TPM2, TPM3 and TPM4. TPM3 together with troponin and actin works to change calcium ion concentration and affects the activity of ATPase, thus affecting muscle contraction.Citation10 Eggert et al. found that the TPM3 gene affects the type and size of muscle fibers, resulting in differences in muscle fiber composition and distribution, thus affecting meat quality.Citation11 Other studies have verified that TPM3 might be related to skeletal muscle development in chicken embryos and postnatal muscle growth.Citation12 However, there are few reports on the effect of the TPM3 gene on bovine muscle growth and development.
Therefore, this study aimed to reveal the role of the TPM3 gene in the growth and development of bovine myoblasts and lay a theoretical foundation for improving the yield and quality of beef and molecular breeding in the future. In this study, the sequence and structural characteristics of the TPM3 gene in Qinchuan cattle were analyzed by bioinformatics, and its expression in bovine tissues and cells was detected by qRT-PCR. Finally, the effect of interference with the TPM3 gene on the proliferation and differentiation ability of bovine myoblast was investigated by siRNA technology.
Materials and methods
Experimental animals and tissue collection
Three healthy male calves (1 day old) and three male adult cattle (18 months old) from the experimental station of Northwest Agricultural & Forestry University (Yangling, Shaanxi) were slaughtered, and 10 tissues such as heart, liver, spleen, lung, and kidney were collected, and frozen with liquid nitrogen. They were stored in a refrigerator at −80 ° C for a long time.
Cloning and analysis of cattle TPM3 gene sequences
To obtain the CDS sequences of the Qinchuan cattle TPM3 gene, total RNA was extracted from the longissimus dorsi muscle of Qinchuan cattle using RNAiso Plus (TaKaRa, Dalian, China) and reverse transcribed into cDNA using reverse transcription reagents. Using the cDNA as a template, PCR amplification was performed using TPM3 CDS region-specific primers. PCR system: 20 µl volumes containing 10 µl 2X M5 HiPer plus Taq HiFi PCR mix (Mei5 Bio, China), 100 ng cDNA, 0.5 µl PCR forward primer, 0.5 µl PCR reverse primer, and 8 µl RNase-free H2O. After the PCR products were recovered by Gel Extraction Kit D 2500 (Omega Biotek, USA), T-Vector pMD 19 (TaKaRa, Dalian, China) was used to connect them, and sent to TsingkeBiotechnology Co., Ltd. (Beijing, China) for sequencing. Sequence blast analysis of the TPM3 gene was performed using the online software NCBI, and ORF Finder was used to identify open reading frames. ProtParam and ProtScale were used to analyze the physicochemical properties and hydrophilic properties of the TPM3 amino acid sequences. Utilizing NetPhos 3.1 server software to predict the phosphor site of TPM3 protein. The SignalP 5.0 and TMHMM 2.0 online tools were used to predict the signal peptide and transmembrane structure of TPM3. The secondary structures of proteins were predicted by SOPMA. In summary, shows the specific information of the online software used for bioinformatics analysis. The amino acid sequences about TPM3 used for the construction of the phylogenic tree were completed using MEGA 11 (version 11.0.13).
Table 1. Online software information for bioinformatics analysis.
Bovine myoblast culture and small interfering RNA transfection
Bovine myoblasts were derived from the primary skeletal muscle myoblasts of 1d male calves isolated and frozen in the laboratory. siRNA and Negative Control (NC) (Sangon Biotech, China) were transfected into the myoblasts according to the instructions of the Lipofectamine™ 3000 Transfection Reagent (Thermo Fisher Scientific, USA). si-TPM3 or NC was transfected into bovine myoblasts with a confluence of 70%. Interference efficiency was measured 24 hours after transfection.
qRT-PCR assay
The qRT-PCR analysis was completed with SYBR Green Premix Pro Taq HS qRT-PCR kit(Accurate Biotechnology(Hunan) Co., Ltd, China)and the CFX Connect Real-Time PCR Detection System (Bio-Rad, USA). Each group has three biological replicates, each containing three technical replicates. The expression levels of mRNAs were quantified using the 2−ΔΔCT method. GAPDH was used as the housekeeping gene, and all primer information is listed in .
Table 2. Primer information used in the experiment.
Flow cytometry assay
After 24 hours of si-TPM3 transfection, the myoblasts were digested and harvested with 0.25% pancreatic enzyme. According to the manufacturer’s instructions, the cells were incubated in a Cell Cycle Straining Solution (MultiSciences, China) for 30 minutes and subjected to Flow Cytometry (CytoFLEX, Beckman, China) detection with a sample size of 10,000 cells.
EdU staining assay
Myoblasts in the logarithmic growth phase were treated with si-TPM3 or NC. After 24 hours, according to the EdU Kit’s instructions (Ribio, China), the cells were treated with EdU solution, fixed with 4%Paraformaldehyde, and transparent with 0.5%Triton 1X-100. The nucleus was stained with DAPI (Thermo Fisher, China). EVOS™ Auto 2 (Thermo Fisher, China) and Image Pro Plus (version 6.0)are used for photography and data statistical analysis, respectively. Each group included 3 biological replicates, and two images were selected for statistical analysis for each biological replicate.
Western blotting analysis
To extract total protein, a protein lysis buffer supplemented with 1% PMSF (Solarbio, China) was added to the myoblasts and lysed on ice for 10 minutes. The lysate products were collected and centrifuged, the supernatant was taken, protein loading buffer was added and boiled for 10 minutes, BCA was used to detect the protein concentration, and the sample loading amount was adjusted. The proteins were then separated using a 12% Sodium Dodecyl Sulphate-Polyacrylamide Gel Electrophoresis (SDS-PAGE) and transferred to a Polyvinylidene Fluoride (PVDF) membrane. QuickBlock™ Western blocking solution (Beyotime, China) was used for blocking for 2 hours and incubated with primary antibodies overnight at 4 °C. The membrane was then incubated with the secondary antibody for 2 hours at room temperature in the dark. Finally, a DocTMXR system (Bio-Rad, USA) was utilized to expose the protein bands. This study used six primary antibodies: TPM3(1:1000, Proteintech), PCNA (1:2000, Abcam), CCNB1 (1:500, Proteintech), GAPDH (1:5000, Abcam), MYH3(1:1000, Proteintech) and MYF5(1:1000, Abcam) and secondary antibodies HRP-conjugated Rabbit anti-Goat IgG (1:5000, Sangon Biotech).
Statistical analysis
The data represent the mean ± standard deviation (SD). GraphPad Prism 8 (version 8.0.2, GraphPad Software, USA) is used for statistical analysis and image construction. Using Student’s t-test to analyse the significance of experimental results.Citation13 **P < 0.01 and *P < 0.05 indicate very significant or significant, respectively.
Results
Cloning and sequence analysis of the TPM3 gene
Sequence analysis found that the TPM3 gene was located on chromosome 3, with a total length of 27,550bp and 15 exons, encoding 284 amino acids (). Using the longissimus dorsal muscle of the Qinchuan cattle as a template, the CDS region of Qinchuan TPM3 gene was cloned with a length of 855 bp (). There was a degenerate base Y at position 161 of the TPM3 gene sequence in GenBank cattle (entry number NM: 000111674), and Sanger sequencing results indicated that the TPM3 sequence in Qinchuan cattle is a T base (). The protein has an isoelectric point of 4.6 and an instability coefficient of 43.92, indicating that it is an unstable acidic protein. The predicted results of protein hydrophobicity revealed that the protein was hydrophilic (). When the phosphorylation threshold is above the threshold line, it indicates that the amino acid is susceptible to phosphorylation. The TPM3 protein of Qinchuan cattle was susceptible to phosphorylation at 19 sites, among which the most phosphorylated site was serine (Ser) with 8 sites, followed by tyrosine (Tyr) with 6 sites, and the least was threonine (Thr) with 5 sites (). The transmembrane structure and potential signal peptide of the TPM3 protein were predicted using the online software TMHMM 2.0 and SignalP 5.0, and their results showed that there was no transmembrane structure and signal peptide for this protein. The results of protein secondary structure prediction indicated that the secondary structure of TPM3 protein mainly consisted of α-helix (98.59%), irregular coil (1.06%), and β-turn (0.35%) (). Evolutionary tree analysis showed that the amino acid sequence of the bovine TPM3 gene clustered with that of Bos indicus and was highly conserved among mammals ().
Figure 1. Sequence and structural characteristics of qinchuan cattle TPM3 gene. (A) TPM3 gene information. (B) PCR amplification of the TPM3 CDS region. (C) Sequence alignment analysis between TPM3 clone sequencing and bovine genome sequence. (D) Prediction of hydrophilicity and hydrophobicity of TPM3 sequence. © Phosphorylation site prediction of TPM3 sequence. (F) Secondary structure prediction of TPM3 protein sequence. (G) Phylogenetic tree of the amino acid sequences of TPM3.
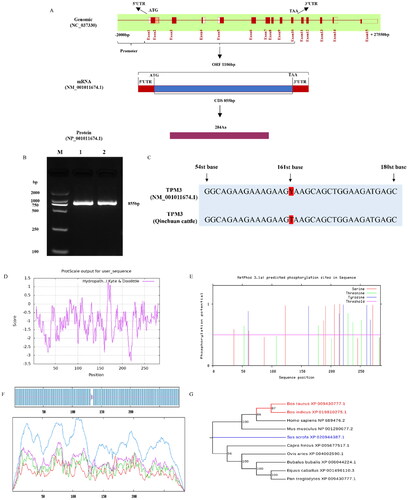
Expression patterns of TPM3 in qinchuan cattle tissues and myoblasts
qRT-PCR was used to detect the expression profile of the TPM3 gene in different tissues of newborn calves (1 day old) and adult cattle (24 months old). The expression of the TPM3 gene was highest in the longissimus dorsi muscle, followed by the heart and lung, and lower in other tissues of the newborn calf (). Interestingly, the expression pattern of the TPM3 gene in various tissues of adult cattle is similar to that of newborn calves, but the expression level of the TPM3 gene in tissues of adult cattle was higher than that of calf tissues (). The expression of TPM3 in myoblasts increased at first and then decreased, and the expression of TPM3 reached the highest level on the 3rd day and then decreased on the 4th day ().
Interference with TPM3 inhibits the proliferation of bovine myoblasts
To determine the effect of TPM3 on bovine muscle cell development, we synthesized the TPM3 siRNA sequence and transfected it into bovine myoblasts. After 24 hours, the expression level of TPM3 was detected by qRT-PCR and Western blot. The results showed that the mRNA and protein expression levels of TPM3 in myoblasts were very significantly reduced after si-TPM3 treatment (P < 0.01), indicating successful TPM3 interference (). Flow cytometry results exhibited that inhibition of TPM3 highly significantly decreased the proportion of cells in the S phase (P < 0.01) (). EdU staining analysis identified that inhibition of TPM3 highly significantly reduced the number of EdU-positive cells (P < 0.01) (). Through qRT-PCR, it was found that si-TPM3 extremely significantly or significantly inhibited the mRNA levels of CDK1, PCNA, CCNB1, CCNE1, CCNE2 (P < 0.01), and CCNA1 (P < 0.05) (). Protein levels of PCNA, and CCNB1 were very significantly reduced by Western blot analysis (P < 0.01) (). These results indicate that interference with TPM3 inhibits the proliferation of bovine myoblasts.
Figure 3. The effect of interfering with TPM3 on the proliferation of bovine myoblasts. (A) Detection of TPM3 interference efficiency (B) Cell cycle phase was measured by flow cytometry. (C) Cell proliferation detected by EdU. (D) a©©The expression of proliferation marker genes was detected by qRT-PCR and Western blots after transfection with si-TPM3. Abbreviations: *P < 0.05 and **P < 0.01 respectively indicate significant and highly significant differences between the two groups.
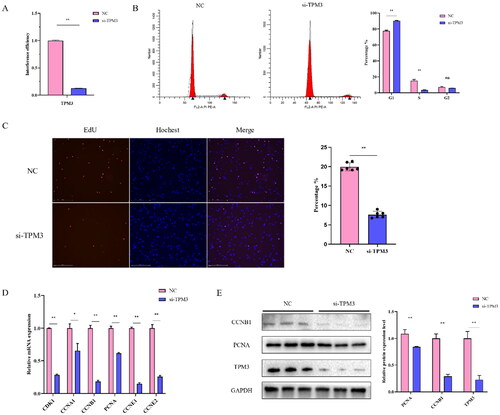
Interference with TPM3 suppresses the differentiation of bovine myoblasts
To confirm the role of TPM3 in the differentiation of bovine myoblasts. After 24 hours, the differentiation medium was changed to induce myotube formation, and the morphological changes of differentiated muscle cells were observed under an inverted microscope. We found that the myotubes of the si-TPM3 treated group began to change on day 2 of cell differentiation compared to the control group, and the changes were most pronounced on day 3, where atrophy and decreased integrity of the myotubes could be observed in the si-TPM3 treated group (). Therefore, we collected cell samples on day 3 of differentiation for qRT-PCR and Western blot analysis and found that si-TPM3 significantly reduced the abundance of MYOD, MYOG, MYH3, MEF2C, MYF5 (P < 0.01), and MYH6 (P < 0.05) expression at the mRNA level (). At the same time, protein levels of MYH3 (P < 0.05) and MYF5 (P < 0.01) were also reduced (). These results suggest that interference with TPM3 inhibits the normal differentiation of bovine myoblasts.
Figure 4. The effect of interfering with TPM3 on the differentiation of bovine myoblasts. (A) Phenotypic changes in induced differentiation of myoblasts after transfection with si-TPM3. (B) and (C) The expression of differentiation marker genes was detected by qRT-PCR and Western blots. Abbreviations: *P < 0.05 and **P < 0.01 respectively indicate significant and highly significant differences between the two groups.
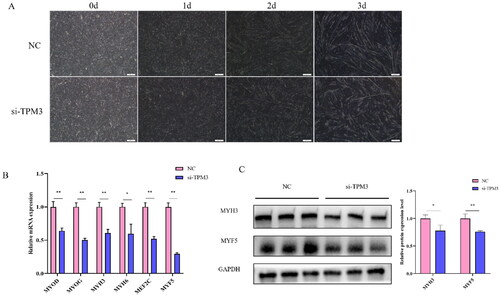
Discussion
Tropomyosin is an actin-binding protein, which plays an important role in regulating muscle contraction.Citation14 There are four tropomyosin genes in mammalian genomes, namely TPM1, TPM2, TPM3, and TPM4, each of which can produce a large number of isoforms by alternative splicing of mRNA.Citation15 High molecular weight TPMs(TPM1, TPM2, and TPM3) play an important role in stabilizing actin filaments and regulating cell growth and development.Citation16 Among them, TPM3 has been proven to participate in various biological processes, such as the growth and development of embryos, neurons, hearts, and skeletal muscles. For example, TPM3 may control changes in neuron size and neurite outgrowth.Citation17 The expression level of TPM3 was the highest in chicken embryonic skeletal muscle.Citation12 However, the role of TPM3 in the development of bovine skeletal muscle remains to be studied.
In this study, the 855 bp sequence of the Qinchuan cattle TPM3 CDS region was obtained by cloning. Bioinformatic analysis showed that it encoded 285 amino acids with 19 phosphorylation sites. The Sanger sequencing results confirmed that the 161st base of the TPM3 gene sequence in Qinchuan cattle is a T base, rather than the degenerate base Y predicted by the GenBank, which is beneficial for us to comprehensively understand all information of the TPM3 gene. It was an unstable acidic protein with a secondary structure composed mainly of α-helixes. Sequence homology analysis found that it was conserved in mammals and closer to zebu and humans, which was consistent with previous studies.Citation18 In this study, tissue expression profiles displayed that TPM3 was highly expressed in the longissimus dorsi muscle and myocardium of both adult and newborn calves, and the expression in the myocardium was lower than that in the longissimus dorsi muscle, which is consistent with its expression in adult muscle and myocardial tissue.Citation19 However, the results were inconsistent with the findings of Oe, M. et al. that TPM3 expression levels were similar in bovine heart and skeletal muscle.Citation20 Since we are not aware of the details of the bovine skeletal muscle samples in Oe, M.’s study, we hypothesize that the occurrence of such results may be related to skeletal muscle from different parts of the bovine origin. Another study also supports our hypothesis that the expression of TPM3 varies in different muscle tissues, including the masseter (MA), diaphragm (DP), tongue (TN), semispinalis (SS), deep chest (PP), biceps femoris (BF), and psoas major (PM).Citation18,Citation21 Previous studies have confirmed that the Met9Arg mutation in TPM3 is closely related to linear myopathy and skeletal muscle weakness.Citation22 LncWEE2-AS1 has been found to promote the expression of TPM3 through sponge miR-29b-2-5p, thereby promoting the proliferation and migration of glioma cells.Citation23 Chen et al. found that TPM3 helps promote the proliferation and migration ability of esophageal cancer cells by regulating the production of MMP2/9.Citation24 The above studies have confirmed that the TPM3 gene is closely related to muscle growth and development. Therefore, to further study the effect of TPM3 on muscle development, we established the Qinchuan bovine myoblast model in vitro and preliminarily explored the effect of TPM3 gene interference on the proliferation of bovine myoblasts. We found that down-regulation of TPM3 leads to abnormalities in normally proliferating myoblasts. Compared with the control group, the number of normal proliferating cells in the si-TPM3 group was significantly reduced, and the proportion of cells in the G0/1 phase was higher. At the same time, the mRNA and protein levels of the proliferation-related genes PCNA and CCNB1 were significantly decreased (P < 0.01). PCNA is a nuclear protein of cell DNA. As a kind of protein existing in the cell nucleus, PCNA can effectively reflect the proliferation activity of cells, and the expression of PCNA in proliferating cells is obviously enhanced.Citation25,Citation26 CCNB1, as a member of the cyclin family, is the key initiator of mitosis and participates in the process of the cell cycle.Citation27,Citation28 The results of our study suggest that the interference of TPM3 may affect the normal proliferation of bovine myoblasts by inhibiting the expression of marker genes related to proliferation and cycle.
Similarly, it has been reported that the TPM3 protein is expressed in the myofilaments and nuclei of human mesenchymal stem cells during their differentiation into cardiomyocytes.Citation29 The expression level of TPM3 increases with the active growth of cerebellar processes in rats.Citation30 In this study, we found that the expression of TPM3 in myoblasts at different differentiation stages showed different trends, showing a trend of increasing at first and then decreasing. Meanwhile, after TPM3 interference, the myotube morphology of myoblasts shrinks and narrows, and their integrity decreases. This is similar to the reported TPM3 mutation causing the production of very narrow fibers in the myotubes, which may be related to the failure of myotube fusion.Citation31 Moreover, the expression of MYOD, MYOG, MYH3, MYH6, MEF2C, and CKM was significantly decreased. Western blot results also showed that the expression of MYF5 and MYH3 was significantly decreased after TPM3 interference. MYOG plays an important role in the development of muscle and the differentiation of muscle cells. During the differentiation of muscle cells, the expression of MYOD depends on the expression of MYOG. When MYOG is absent or depleted, the expression of MYOD is inhibited.Citation32 MEF2C is the first member of the MEF2 family to be expressed, and it is targeted by MYOD to activate transcription through the E-box of its enhancer region in vivo, thus promoting skeletal muscle differentiation.Citation33 These results indicate that the interference of TPM3 can affect the differentiation of bovine myoblasts by inhibiting the expression of genes related to cell differentiation.
Skeletal muscle is composed of two main types of muscle fibers: type I (slow type) and types IIA and IIB (fast type) muscle fibers. Tropomyosin (TPM) can be used as a marker to distinguish the types of muscle fibers. In human and bovine skeletal muscle, TPM1 was found to be expressed in fast-type muscle fibers, and TPM3 was specifically expressed in slow-type muscle fibers.Citation18,Citation34 Muscle fiber type plays a significant role in the normal development of skeletal muscle. Congenital fiber type disorder (CFTD) is a rare subtype of congenital myopathy, which shows that type 1 (slow type) fiber is at least 50% smaller than type 2 (fast type) fiber. The main reason for its occurrence is the dominant missense mutation of slow α tropomyosin encoded by the TPM3 gene.Citation35,Citation36 The type of muscle fiber is not only related to the occurrence of muscle disease but also closely related to the meat quality traits of economic animals such as pigs and cattle.Citation11,Citation37 Schiaffino et al. discovered that myosin heavy chain (MYHC) is the main protein responsible for different muscle fiber types.Citation38 MYHC, MyHC-2a, and MyHC-2x are expressed in bovine skeletal muscle. In the skeletal muscle of Holstein cattle, the expression of TPM3 and MYHC is highly positively correlated (r = 0.92). MYHC and myofibrillar protein could affect muscle contraction after slaughter, thus affecting meat quality.Citation21 Gene hypermethylation is associated with the gene downregulated expression. Oe, M et al. found that in fast muscle fibers, the expression of slow-type muscle fiber-specific genes MYH7, TNNT1, and TPM3 is suppressed by hypermethylation, while in slow-type muscle fibers, fast-type muscle fiber-specific genes are also highly methylated and down-regulated, which indicates that after muscle fiber type commitment, the maintenance of muscle fiber type may be related to methylation.Citation39 Transcriptome analysis of different muscles of Dezhou donkeys showed that the tenderness of donkey meat may be related to muscle fiber type, which may benefit from differential genes MYH1, MYH7, TPM3, and so on.Citation40,Citation41 In addition, TPM3 was closely related to muscle development and lipid accumulation in different sexes of Korean cattle. The expression of TPM3 in the intramuscular adipose tissue of bulls was higher than that in the omental adipose tissue and positively correlated with marbling score and quality grade.Citation42 These studies suggest that TPM3 may play an important regulatory role in meat quality.
Collectively, our results revealed that the TPM3 gene was specifically highly expressed in the muscle tissue of Qinchuan cattle, and affected the proliferation and differentiation of myoblasts, but its mechanism still needs to be further explored.
Conclusions
Our study emphasizes the important role of TPM3 in the growth and maturation of skeletal muscle in Qinchuan cattle. Through cloning and sequence analysis, we found that the 161 base of the TPM3 gene sequence in the Genebank is a degenerate base Y, while the base at the same position in Qinchuan cattle is a T base. This gene consists of 15 exons and is responsible for encoding a protein with 285 amino acids. The strong conservatism of TPM3 proteins across mammalian species highlights the evolutionary importance of TPM3 in muscle physiology. Expression profiling analysis demonstrated prevalent TPM3 expression in multiple tissues of Qinchuan cattle, including prominent levels observed in the longissimus dorsi muscle, heart. The consistency in TPM3 expression patterns between newborn calves and adult cattle indicates a significant role throughout development, with elevated levels detected in mature animals. In vitro investigations revealed fluctuating TPM3 expression in myoblasts, reaching its peak on the third day before diminishing. Functional investigations, such as the disruption of TPM3, highlighted its crucial role in controlling the growth and development of bovine muscle cells. The notable decrease in cell proliferation, accompanied by a lower proportion of cells in the S phase and decreased expression of important genes related to proliferation, underlines the importance of TPM3 in regulating muscle cell behavior. Additionally, hindered myoblast differentiation following interference with TPM3 suggests its impact on the process of maturation. Future studies should focus on revealing the complex molecular processes involved in regulating muscle growth through TPM3. Understanding these mechanisms could lead to a better grasp of musculoskeletal biology and the identification of new targets for improving livestock breeding methods and meat quality. Our research not only highlights the significance of TPM3 in skeletal muscle formation but also opens up new possibilities for practical agricultural applications through innovative research paths.
Author contributions
Conceptualization, L.Z., J.G.; methodology, J.G., J.W., B.L.; software, K.Z., Y.P.; data curation, L.Z.; writing-original draft preparation, J.G,; Visualization, J.W., Z.Y.; writing-review and editing, K.Z., Z.Y.,Y.P., H.Y., S.Y., H.A.R, B.K.A.; supervision, L.Z.; project and funding acquisition, L.Z. All authors have read and agreed to the published version of the manuscript.
Disclosure statement
No potential conflict of interest was reported by the author(s).
Additional information
Funding
References
- Frontera WR, Ochala J. Skeletal muscle: a brief review of structure and function. Calcif Tissue Int. 2015;96(3):1–12.
- Gu X, Wang L, Liu S, Shan T. Adipose tissue adipokines and lipokines: Functions and regulatory mechanism in skeletal muscle development and homeostasis. Metabolism. 2023;139:155379.
- Bharathy N, Ling BM, Taneja R. Epigenetic regulation of skeletal muscle development and differentiation. Subcell Biochem. 2013;61:139–150.
- Zhang W, Sun B, Zhao Y, et al. Proliferation of bovine myoblast by LncPRRX1 via regulation of the miR-137/CDC42 axis. Int J Biol Macromol. 2022;220:33–42.
- Gao M, Li X, Yang Z, et al. circHIPK3 regulates proliferation and differentiation of myoblast through the miR-7/TCF12 pathway. J Cell Physiol. 2021;236(10):6793–6805.
- Zammit PS. Function of the myogenic regulatory factors Myf5, MyoD, Myogenin and MRF4 in skeletal muscle, satellite cells and regenerative myogenesis. Semin Cell Dev Biol. 2017;72:19–32.
- Tang J, Yang B, Song G, et al. Effect of bovine myosin heavy chain 3 on proliferation and differentiation of myoblast. Anim Biotechnol. 2022;34(9):4337–4346.
- Yun K, Wold B. Skeletal muscle determination and differentiation: story of a core regulatory network and its context. Curr Opin Cell Biol. 1996;8(6):877–889.
- Gunning P, O’Neill G, Hardeman E. Tropomyosin-based regulation of the actin cytoskeleton in time and space. Physiol Rev. 2008;88(1):1–35.
- Karpicheva OE, Avrova SV, Bogdanov AL, et al. Molecular mechanisms of deregulation of muscle contractility caused by the R168H mutation in TPM3 and its attenuation by therapeutic agents. Int J Mol Sci. 2023;139:155379.
- Eggert JM, Depreux FF, Schinckel AP, et al. Myosin heavy chain isoforms account for variation in pork quality. Meat Sci. 2002;61(2):117–126.
- Dube S, Abbott L, Randhawa S, et al. Sarcomeric TPM3 in developing chicken. Cytoskeleton (Hoboken). 2018;75(4):174–182.
- Ma X, Yang X, Zhang D, et al. RNA-seq analysis reveals the critical role of the novel lncRNA BIANCR in intramuscular adipogenesis through the ERK1/2 signaling pathway. J Anim Sci Biotechnol. 2023;14(1):21.
- Gonchar AD, Kopylova GV, Kochurova AM, et al. Effects of myopathy-causing mutations R91P and R245G in the TPM3 gene on structural and functional properties of slow skeletal muscle tropomyosin. Biochem Biophys Res Commun. 2021;534:8–13.
- Helfman DM, Cheley S, Kuismanen E, et al. Nonmuscle and muscle tropomyosin isoforms are expressed from a single gene by alternative RNA splicing and polyadenylation. Mol Cell Biol. 1986;6(11):3582–3595.
- Gimona M, Kazzaz JA, Helfman DM. Forced expression of tropomyosin 2 or 3 in v-Ki-ras-transformed fibroblasts results in distinct phenotypic effects. Proc Natl Acad Sci U S A. 1996;93(18):9618–9623.
- Tomanić T, Martin C, Stefen H, et al. Deletion of the Actin-Associated Tropomyosin Tpm3 Leads to Reduced Cell Complexity in Cultured Hippocampal Neurons-New Insights into the Role of the C-Terminal Region of Tpm3.1. Cells. 2021;10(3):715.
- Oe M, Ohnishi-Kameyama M, Nakajima I, et al. Muscle type specific expression of tropomyosin isoforms in bovine skeletal muscles. Meat Sci. 2007;75(4):558–563.
- Dube DK, Dube S, Abbott L, et al. Sarcomeric TPM3 expression in human heart and skeletal muscle. Cytoskeleton (Hoboken). 2020;77(8):313–328.
- Dube S, Abbott L, Randhawa S, et al. Identification of a novel TPM4 isoform transcript and comparison to the expression of other tropomyosin isoforms in bovine cardiac and skeletal muscles. International Journal of Biochemistry and Molecular Biology. 2021;12(1):17–34.
- Oe M, Nakajima I, Muroya S, et al. Relationships between tropomyosin and myosin heavy chain isoforms in bovine skeletal muscle. Anim Sci J. 2009;80(2):193–197.
- Laing NG, Wilton SD, Akkari PA, et al. A mutation in the alpha tropomyosin gene TPM3 associated with autosomal dominant nemaline myopathy. Nat Genet. 1995;9(1):75–79.
- Jia Z, Qian Z, Tang Y, et al. LncRNA WEE2-AS1 Knockdown Inhibits the Proliferation, Migration and 3 Invasion of Glioma Cells via Regulating miR-29b-2-5p/TPM3 Axis. Oncol Res. 2021;29(2):105–117.
- Chen S, Shen Z, Gao L, et al. TPM3 mediates epithelial-mesenchymal transition in esophageal cancer via MMP2/MMP9. Ann Transl Med. 2021;9(16):1338–1338.
- Naryzhny SN. Proliferating cell nuclear antigen: a proteomics view. Cell Mol Life Sci. 2008;65(23):3789–3808.
- Maga G, Hubscher U. Proliferating cell nuclear antigen (PCNA): a dancer with many partners. J Cell Sci. 2003;116(Pt 15):3051–3060.
- Petrachkova T, Wortinger LA, Bard AJ, et al. Lack of Cyclin B1 in zebrafish causes lengthening of G2 and M phases. Dev Biol. 2019;451(2):167–179.
- Xie B, Wang S, Jiang N, Li JJ. Cyclin B1/CDK1-regulated mitochondrial bioenergetics in cell cycle progression and tumor resistance. Cancer Lett. 2019;443:56–66.
- Asumda FZ, Chase PB. Nuclear cardiac troponin and tropomyosin are expressed early in cardiac differentiation of rat mesenchymal stem cells. Differentiation; Research in Biological Diversity. 2012;83(3):106–115.
- Weinberger RP, Henke RC, Tolhurst O, et al. Induction of neuron-specific tropomyosin mRNAs by nerve growth factor is dependent on morphological differentiation. The Journal of Cell Biology. 1993;120(1):205–215.
- Fidzianska A, Madej-Pilarczyk A, Hausmanowa-Petrusewicz I. Is mutation p.Arg168Gly in TPM3 gene responsible for Type 1 fiber hypoplasia and cap structure formation? Clin Neuropathol. 2014;33(1):61–64.
- Adhikari A, Kim W, Davie J. Myogenin is required for assembly of the transcription machinery on muscle genes during skeletal muscle differentiation. PLoS One. 2021;16(1):e0245618.
- Dodou E, Xu SM, Black BL. Mef2c is activated directly by myogenic basic helix-loop-helix proteins during skeletal muscle development in vivo. Mech Dev. 2003;120(9):1021–1032.
- Matyushenko AM, Shchepkin DV, Kopylova GV, et al. Unique functional properties of slow skeletal muscle tropomyosin. Biochimie. 2020;174:1–8.
- Clarke NF, Kolski H, Dye DE, et al. Mutations in TPM3 are a common cause of congenital fiber type disproportion. Ann Neurol. 2008;63(3):329–337.
- Moreno CAM, Estephan EP, Fappi A, et al. Congenital fiber type disproportion caused by TPM3 mutation: A report of two atypical cases. 2020;30:54–58.
- Ozawa S, Mitsuhashi T, Mitsumoto M, et al. The characteristics of muscle fiber types of longissimus thoracis muscle and their influences on the quantity and quality of meat from Japanese Black steers. Meat Sci. 2000;54(1):65–70.
- Schiaffino S, Reggiani C. Molecular diversity of myofibrillar proteins: gene regulation and functional significance. Physiol Rev. 1996;76(2):371–423.
- Oe M, Ojima K, Muroya S. Difference in potential DNA methylation impact on gene expression between fast- and slow-type myofibers. Physiol Genomics. 2021;53(2):69–83.
- Li Y, Ma QS, Shi XY, et al. Comparative transcriptome analysis of slow-twitch and fast-twitch muscles in dezhou donkeys. Genes (Basel). 2022;13(9):1610.
- Chai W, Qu H, Ma Q, et al. RNA-seq analysis identifies differentially expressed gene in different types of donkey skeletal muscles. Anim Biotechnol. 2022;34(5):1786–1795.
- Cho JH, Jeong JY, Lee RH, et al. Regional differences of proteins expressing in adipose depots isolated from cows, steers and bulls as identified by a proteomic approach. Asian-Australas J Anim Sci. 2016;29(8):1197–1206.
- Wilkins MR, Gasteiger E, Bairoch A, et al. Protein identification and analysis tools in the ExPASy server. Methods Mol Biol. 1999;112:531–552.
- Blom N, Gammeltoft S, Brunak S. Sequence and structure-based prediction of eukaryotic protein phosphorylation sites. J Mol Biol. 1999;294(5):1351–1362.
- Nielsen H, Tsirigos KD, Brunak S, et al. A Brief history of protein sorting prediction. Protein J. 2019;38(3):200–216.
- Hallgren J, Tsirigos KD, Pedersen MD, et al. DeepTMHMM predicts alpha and beta transmembrane proteins using deep neural networks. bioRxiv. 2022.
- Geourjon C, Deléage G. SOPMA: significant improvements in protein secondary structure prediction by consensus prediction from multiple alignments. Comput Appl Biosci. 1995;11(6):681–684.