Abstract
In this study, we investigated the effects of supplemental Glycyrrhiza polysaccharide (GCP) on growth performance and intestinal health of weaned piglets. Ninety piglets weaned at 28 days of age were randomly allocated to three groups with five replicates per treatment. Piglets were fed the following diets for 28 days: (1) CON (control group), basal diet; (2) G500, CON + 500 mg/kg GCP; (3) G1000, CON + 1000 mg/kg GCP. The results showed that supplementation with 1000 mg/kg GCP increased the average daily gain (ADG) and decreased the feed-to-gain ratio (F/G) (P < 0.05). Serum diamine oxidase (DAO) and D-lactic acid (DL-A) levels were lower in the G1000 group (P < 0.05). Dietary GCP 1000 mg/kg improved mucosal trypsin activity in the duodenum, jejunum and ileum and increased lipase and amylase activity in the jejunum (P < 0.05). Moreover, in the G1000 group, ZO-1, claudin 1 and occludin levels were increased in the jejunum mucosa, whereas interleukin-1β (IL-1β) and IL-6 levels were decreased (P < 0.05). The 16S rRNA gene analysis indicated that dietary 1000 mg/kg GCP altered the jejunal microbial community, with increased relative abundances of beneficial bacteria. In conclusion, dietary GCP 1000 mg/kg can improve growth performance, digestive enzyme activity, intestinal immunity, barrier function and microbial community in weaned piglets.
Introduction
China is not only the largest consumer of pork in the world, but also the largest pork producer, providing nearly half of the world’s total production.Citation1 However, many challenges can affect performance in modern pig farming.Citation2 Among these problems, weaning is a stressful event that causes enormous changes in the gastrointestinal tract, resulting in inflammation and oxidative damage.Citation3 Intestinal health is increasingly considered to be closely related to the health, welfare and growth performance of pigs. Intestinal sickness or injury will lead to less efficient feed utilization, resulting in great economic losses in production.Citation4 Although antibiotics have been added to feed to improve the intestinal health of livestock, they have now been banned in many countries due to food safety concerns.Citation5 Hence, it is necessary to identify new feed additives to enhance the intestinal health of weaned piglets.
Plant polysaccharides, which are high molecular weight natural polymers that can be extracted, have been shown to have extensive pharmacological properties, including anti-inflammatory, antioxidant and antiviral activities.Citation6–9 As an example, Glycyrrhiza uralensis Fisch is an important traditional Chinese medicine and has been widely used in many fields such as food, medicine and cosmetics.Citation10 Glycyrrhiza polysaccharide, one of the main components of Glycyrrhiza uralensis Fisch, is mainly composed of rhamnose (Rha), arabinose (Ara), xylose (Xyl), mannose (Man), glucose (Glc), and galactose (Gal), and its molecular weight depends on the extraction method.Citation10 The application of Glycyrrhiza polysaccharide (GCP) has also been reported to have positive effects in animals. For example, dietary supplementation of GCP promotes the growth of broiler chickens.Citation11 Chen et al.Citation12 showed that GCP significantly improves growth performance and immunity in mice. Furthermore, GCP has been reported to inhibit tumor growth in a tumor-bearing mouse model by regulating intestinal microbiota.Citation13
We have recently shown that GCP improves the growth performance, immunity and antioxidant capacity of weaned piglets.Citation14 In this study, we explored the effects of dietary GCP supplementation improves growth performance, digestive enzyme activity, intestinal immunity, barrier function and microbiota in weaned piglets. The results of this study provide theoretical support for the use of GCP as a novel feed additive for weaned piglets.
Materials and methods
Ethics statement
All procedures were reviewed and approved by the Animal Care and Use Committee of Henan University of Science and Technology before the initiation of the study (Ethic Approval Code: HAUT20221115). All animals were treated humanely throughout the study.
Experimental design, animals and housing
A total of 90 crossed healthy piglets (Duroc × Landrace × Yorkshire; initial average body weight (BW) 9.36 ± 0.13 kg) weaned at 28 days of age were randomly allocated to three treatment groups [n = 30 per group consisting of five replicate pens of six piglets] as follows: (1) control group (basal diet supplemented with 0 mg/kg GCP), (2) G500 group (basal diet supplemented with 500 mg/kg GCP), (3) G1000 (basal diet supplemented with 1000 mg/kg GCP). The dose was based on the product basis. Piglets in each group were fed the experimental diet for 28 days. GCP (purity ≥ 24%; remainder mainly inactive substances such as ash, colloid and mineral salts) was obtained from Luoyang Lansealy Technology (Luoyang, China). The basal diet was formulated for piglets at the corresponding growth period according to the standards required by the National Research Council;Citation15 details of the ingredients and nutrient composition are presented in . Piglets were not exposed to solid feed before weaning. Piglets were housed in pens with fully slatted floors in an environmentally controlled room; the room temperature was maintained at 28 °C during the first week of the experiment, and then gradually dropped to 25 °C over the following three weeks. Each pen was equipped with a feeder and piglets had free access to water and feed throughout the experiment.
Table 1. Basal diet composition and nutrient levels.
Growth performance
All piglets were weighed individually after a 12-h fasting period, and the feed consumption in each pen was recorded daily. Based on the data we measured, the average daily weight gain (ADG), average daily feed intake (ADFI) and feed-to-gain ratio (F/G) were calculated.
Sample collection and preparation
At the end of the experiment, blood was collected via the anterior vena cava from two randomly selected piglets per replicate (n = 10 per group); samples (approximately 5 ml) were collected into separation gel coagulation-promoting vacuum tubes (Tianai Medical Instruments, Heze, China). After coagulation at room temperature for 1 h, samples were centrifuged at 3000×g for 30 min at 4 °C. The serum was collected and stored at −80 °C. The same piglets were euthanized humanely by sodium pentobarbital overdose. The gastrointestinal tract was immediately removed and the different regions distinguished. Samples of the contents (1–2 g) and the mucosa (300–500 mg) in the middle of the duodenum, jejunum, and ileum were collected and stored at −80 °C before measurement of indicators.
Serum DAO and D-LA determination
Serum levels of diamine oxidase (DAO) and D-lactic acid (D-LA) were assayed using commercial reagent kits purchased from Yubo Biotechnology (Guangzhou, China) using enzyme-linked immunosorbent assay (ELISA) and a multifunctional microplate reader (Tecan, Mannedorf, Switzerland) according to the manufacturer’s instructions.
Measurement of pH
Samples of the small intestine were collected into a sterile Petri dish. The pH of the contents was measured using an insertable microglass pH electrode (model 301, Hanna Instruments).
Digestive enzyme determination
The contents samples of duodenum, jejunum and ileum were homogenized with ice-cold physiologic saline (1:4, w/v) and vortexed and centrifuged at 3500×g for 15 min. The supernatant was collected and stored at −80 °C before assay. Lipase, protease and diastase activity in the supernatants of intestinal content was measured using commercial kits (Jiancheng Bioengineering Institute, Nanjing, China) according to the manufacturer’s instructions.
Total RNA extraction and quantitative real-time PCR analysis
Total RNA was extracted from intestinal mucosa using TRIzol reagent (Solarbio, Beijing, China) according to the manufacturer’s instructions. Subsequently, the integrity, purity (OD260/OD280 ratio 1.8–2.0), and concentration of RNA were evaluated by 1% agarose gel electrophoresis and a nucleic acid protein analyzer (Therom, Rockford, USA). Finally, RNA was reverse transcribed into cDNA using the HiScript® III RT SuperMix for qPCR (+gDNA eraser) kit (Vazyme, Nanjing, China).
Real-time quantitative PCR (qPCR) was used to evaluate the mRNA expression levels of the target genes: zonula occludens-1 (ZO-1), claudin-1, occludin, interleukin-1β (IL-1β), interleukin-6 (IL-6), and tumor necrosis factor-alpha (TNF-α). The qPCR was performed on the CFX Connect real-time PCR detection system (Bio-Rad). Details of the primers used in this study are shown in . The results were normalized to the expression of a housekeeping gene (β-actin) and relative gene expression was determined using the 2−ΔΔCt method.
Table 2. Primers used for qPCR.
16S rRNA gene sequencing
Based on the analysis of the above indicators, we chose the control group and an ideal-dose group for 16S rRNA gene sequencing. Five frozen samples of jejunum contents from each group were randomly selected and total genomic DNA was extracted with the E. Z.N.A.® soil DNA Kit (Omega Bio-tek, Norcross, GA, USA) according to the manufacturer’s instructions. The final DNA concentration and purity were measured by spectrophotometer (Thermo Scientific, Wilmington, USA), and DNA quality was determined by 1% agarose gel electrophoresis. The V3–V4 variable regions of the bacterial 16S rRNA gene were amplified using a forward primer (5′-ACTCCTACGGGAGGCAGCAG-3′) and a reverse primer (5′-GGACTACHVGGGTWTCTAAT-3′). The PCR products generated were extracted after separation by 2% agarose gel electrophoresis, purified using the AxyPrep DNA Gel Extraction Kit (Axygen Biosciences, Union City, CA, USA) and quantified using QuantiFluor™-ST (Promega, USA). Subsequently, purified amplicons were sequenced on the Illumina MiSeq platform (Illumina, San Diego, CA, USA) according to standard protocols (Majorbio, Shanghai, China).
The reads were quality-filtered and then subjected to quality control using UPARSE (version 7.1). Sequences with >97% similarity were considered as the same OTUs. A representative sequence for each OTU was screened for further annotation using RDP Classifier v.2.2 (http://rdp.cme.msu.edu/) trained on the Greengenes database by QIIME (v1.8.0), based on a 0.7 confidence threshold. OTU abundance information was normalized using a standard sequence number corresponding to the sample with the least sequences. Bioinformatics analysis (alpha diversity analysis, beta diversity and community composition) was carried out using the free online Majorbio Cloud Platform (www.majorbio.com).
Statistical analysis
Data were evaluated by one-way analysis of variance (ANOVA) using the SPSS17.0 software (IBM, Chicago, IL, USA). The animal pens were considered to be the experimental units for growth performance. Individual piglets were considered as the experimental units for other indicators. Differences in means among treatments were separated by Tukey’s multiple comparisons. The results were presented as the mean ± standard error (SEM). P < 0.05 was set as the threshold for statistical significance.
Results
The effect of GCP on the growth performance
As shown in , there was no significant effect on ADFI among the three treatment groups (P > 0.05). Compared to the control group, supplementation with 1000 mg/kg GCP significantly increased ADG and decreased F/G (P < 0.05).
Table 3. Effects of dietary supplementation with GCP on growth performance of weaned piglets.a
The effect of GCP on the serum DAO and D-LA levels
The serum DAO and DL-LA levels of piglets in the three groups are shown in . Dietary GCP supplementation at 500 and 1000 mg/kg decreased the serum levels of DAO and DL-LA compared to those in the controls, with lower levels detected in the GCP1000 group (P < 0.05).
Table 4. Effects of dietary supplementation with GCP on serum DAO and D-LA in weaned piglets.Table Footnotea
The effect of GCP on the pH of intestinal contents
As shown in , although the pH of the contents of the duodenum, jejunum and ileum was decreased by GCP, there were no significant differences in the pH of the contents of the duodenum, jejunum and ileum among the three groups (P > 0.05).
Table 5. Effects of dietary supplementation with GCP on intestinal pH of weaned piglets.Table Footnotea
The effect of GCP on digestive enzyme activities
The effect of dietary supplementation with GCP on intestinal digestive enzymes of weaned piglets is shown in . Compared with the control group, GCP treatments did not affect the amylase or lipase activity in the duodenum and ileum (P > 0.05), although increased activity of both enzymes was detected in the jejunum in the GCP1000 group. Although dietary supplementation with GCP at 500 mg/kg did not affect trypsin activity in intestinal contents compared with that in the control group (P > 0.05), the levels of trypsin activity detected in the duodenum, jejunum, and ileum of the GCP1000 group were higher than those in the control group.
Table 6. Effects of dietary supplementation with GCP on intestinal digestive enzymes of weaned piglets.Table Footnotea
The effect of GCP on the gene expression
As shown in . Compared with the control group, GCP1000 significantly upregulated mRNA expression of ZO-1 in all three regions of the small intestine (P < 0.05), although this effect was maintained only in the ileum when the dietary supplementation was reduced in the GCP500 group (P < 0.05). In the GCP1000 group, Claudin-1 was also upregulated in the duodenum and jejunum (P < 0.05), but this was maintained only in the duodenum when the dietary supplementation was reduced in the GCP500 group (P < 0.05). In the GCP1000 group, occludin was upregulated in the jejunum and ileum (P < 0.05), although this effect was not maintained in the ileum in the GCP500 group (P > 0.05). In contrast, mRNA expression of IL-1β was downregulated in the jejunum and ileum following supplementation with GCP at 1000 mg/kg (P < 0.05), while this effect was not maintained in the jejunum and ileum in the GCP500 group. In the GCP1000 group, IL-6 was downregulated in all three regions (P < 0.05), but this effect was not maintained in the jejunum in the GCP500 group (P > 0.05). No changes in the expression of TNF-α were detected in any of the regions of the small intestine following dietary supplementation with GCP, even at 1000 mg/kg (P > 0.05).
Figure 1. Effects of dietary GCP on relative gene expression in the small intestine mucosa of weaned piglets. Data represent means ± SEM. Means without a common letter differ significantly differ (P < 0.05). ZO-1, zonula occludens-1; IL-1β, interleukin-1β; IL-6, interleukin-6; TNF-α, tumor necrosis factor-α. CON, basal diet without GCP; G500, basal diet supplemented with 500 mg/kg GCP; G1000, basal diet supplemented with 1000 mg/kg GCP.
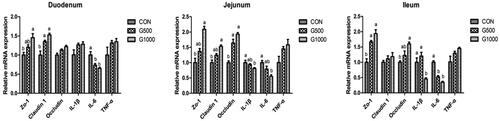
The effect of GCP on the gut microbiota
To explore the effect of GCP on the gut microbiota, we performed 16S rRNA gene sequencing. First, all the rarefaction curves tended to approach a plateau at the current sequencing depth for all samples (). At the OUT level, the Venn diagram then revealed 513 common core OTUs in both groups, and 101 and 131 unique OTUs distributed in the CON and G1000 groups, respectively (). Furthermore, the α diversity revealed that Shannon and Chao indexes were higher in the G1000 group (). In addition, for β diversity, principal coordinates analysis (PcoA) and non-metric multidimensional scaling (NMDS) showed significant separation between the two groups of sample points and clustering within a certain range (). In general, GCP changed the microbial composition in the jejunum.
Figure 2. Effects of dietary GCP on the diversity and composition of jejunal microbiota in weaned piglets. (a) Rarefaction curve based on OTUs; (b) Venn diagram of OTUs in the two groups; α diversity: (c) Shannon index and (d) Chao index; β diversity: (e) principal coordinates analysis (PcoA) and (f) non-metric multidimensional scaling (NMDS) based on OTUs; microbial composition at phylum level (g) and genus level (h). “others” represents species with relative abundance <1%. red circles denote significantly increased phyla and genera while blue circles represent a significant decrease. CON, a basal diet without GCP; G1000, a basal diet supplemented with 1000 mg/kg GCP.
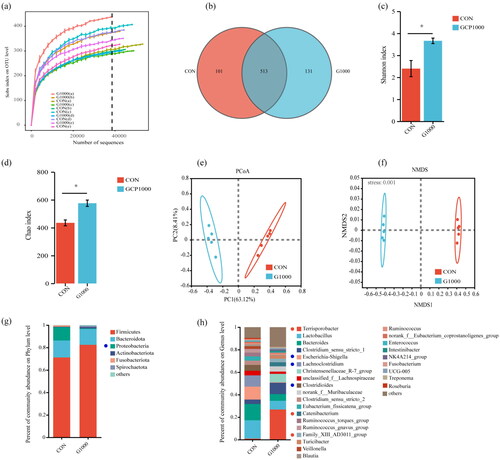
Discriminatory characteristics in the microbial relative abundance at the phylum and genus levels were observed between the CON and G1000 groups with an identical threshold that meant relative abundance in one group was >1%. At the phylum level, Firmicutes, Bacteroidota, Proteobacteria, Actinobacteriota, Fusobacteriota, and Spirochaetota were found to have a relative abundance >1% in at least one group (). The relative abundance of one phylum (Proteobacteria) exhibited a significant decrease in the G1000 group (P < 0.05). At the genus level, 28 genera showed a greater relative abundance (>1%), with 6 genera shifting significantly between the two groups (2 h). Compared with the CON group, the relative abundances of nine genera, including Terrisporobacter, Catenibacterium and Family_XIII_AD3011_group significantly increased, while the relative abundance of six genera, namely Escherichia-Shigella, Lachnoclostridium and Clostridioides significantly decreased in the G1000 group (P < 0.05).
Discussion
Many previous studies have demonstrated that plant polysaccharides can improve animal production performance. For example, Zhu et al.Citation16 reported that dietary supplementation with polysaccharides extracted from Astragalus Propinquus Schischkin improves the growth performance of Channa argus in terms of increasing body weight gain (BWG) and specific growth rate as well as decreasing F/G. Ao et al.Citation17 also found that dietary supplementation with Achyranthes bidentata polysaccharides increased BWG and reduced F/G in Pekin ducks. Extensive studies in pigs have also confirmed that supplementation of diets with plant polysaccharides improved growth parameters.Citation18–20 Similarly, in this study, we showed that dietary supplementation with 1000 mg/kg GCP significantly increased ADG and decreased F/G, indicating that dietary supplementation with GCP improved the growth performance and efficiency of weaned piglets.
Increased ADG and decreased F/G are closely related to digestion, with the small intestine playing a crucial role.Citation21 Hence, we further analyzed indicators that can affect the digestive and absorptive ability of the small intestine. The balance of intestinal microecology is crucial to animal health. It has been reported that beneficial bacteria exist in a low pH environment, while harmful bacteria are favored by a high pH.Citation22 The acidity of the gastrointestinal tract is also an important factor affecting digestion and absorption.Citation23 A previous study showed that dietary supplementation with Acanthopanax senticosus polysaccharides reduced the pH of the contents of the ileum and cecum in weaned piglets.Citation24 Another study showed that dietary supplementation with Gynostemma pentaphyllum polysaccharides effectively reduced the pH of the contents of the duodenum, jejunum and ileum.Citation25 In the current study, the pH of the contents of the duodenum, jejunum and ileum in the GCP1000 and GCP500 groups was lower than that in the control group. However, the mechanism by which GCP lowers the intestinal pH remains to be elucidated.
DAO is a highly active enzyme present mainly in intestinal epithelial cells.Citation26 DL-A, a metabolite secreted by intestinal bacteria, exists mainly in the intestinal cavity.Citation27 Damage to the intestinal mucosa and increased intestinal permeability leads to increased levels of DAO and DL-A in the blood; therefore, serum levels of DAO and DL-A indirectly reflect intestinal barrier function. In this study, dietary supplementation with GCP at both 500 and 1000 mg/kg decreased serum DAO and D-LA levels of weaned piglets compared to the levels detected in the control group. These observations suggest that GCP enhances the intestinal barrier function and decreases permeability, although this requires confirmation using direct methods of analysis.
Tight junctions, which consist of tight junction proteins such as occludin, claudins and ZO-1, are indispensable for the barrier function of the intestine and high levels of tight junction protein expression are very important for intestinal health.Citation28 Previous studies have shown plant polysaccharides have the potential to increase the expression of tight junction proteins. Atractylodis macrocephalae polysaccharides have been reported to increase the expression of tight junction proteins in intestinal epithelial cells challenged with dextran sulfate sodium.Citation29 In addition, Zou et al. (2021) reported that seaweed-derived polysaccharides elevated the mRNA levels of ZO-1, claudin-1 and occludin in the jejunum mucosa in weaned piglets. Similarly, we found that GCP increased the expression of ZO-1, claudin 1 and occludin, with the greatest effect achieved by dietary supplementation with GCP at 1000 mg/kg. Thus, these data indicate that GCP enhances intestinal barrier function by upregulating the expression of tight junction proteins.
Weaning stress causes intestinal inflammation in pigs and is one of the main causes of diarrhea.Citation30 Therefore, strategies to relieve intestinal inflammation in weaned piglets will be beneficial to pork production. Proinflammatory factors such as IL-1β, IL-6 and TNF-α reflect the severity of inflammation. IL-1β, a pleiotropic inflammatory cytokine, is produced by monocytes, macrophages and dendritic cells, and plays an important role in the inflammatory process.Citation31 IL-6 is a cytokine produced by activated T lymphocytes and has been confirmed to be involved in various inflammatory reactions.Citation32 TNF-α is also a key mediator of inflammatory reactions.Citation18 Generally, our findings showed that GCP downregulates the expression of IL-1β and IL-6 in the mucosa of the duodenum, jejunum and ileum, with a more marked effect achieved with 1000 mg/kg GCP. However, dietary supplementation with Radix pseudostellariae stem and leaf polysaccharides was shown to increase mRNA expression of IL-2 and IFN-γ in the duodenal mucosa of weaned piglets treated with cyclophosphamide.Citation33 The discrepancy between these results and those of our study may be related to the different types of polysaccharides used for dietary supplementation and animal treatment conditions. To date, there are few reports of the effects of plant polysaccharides as dietary supplements on the intestinal immunity of weaned piglets and further research is required for greater clarification.
By measuring the fundamental indices, it was determined that the GCP1000 group had a more advantageous impact on the intestinal health of weaned piglets. The jejunum, in particular, is the main site of nutrient digestion and absorption in the small intestine.Citation34 Hence, we chose the CON and G1000 groups to perform 16S sequencing and bioinformatics analyses of jejunum contents. Proteobacteria play an important role in the intestines by producing lipopolysaccharides, which can cause inflammation.Citation35 At the phylum level, we observed that G1000 had a lower relative abundance of Proteobacteria, indicating that GCP may reduce intestinal inflammation. We further evaluated the differences in the microbial composition at the genus level. Terrisporobacter is a beneficial intestinal bacteria that can play a probiotic role.Citation36 Catenibacterium can produce short-chain fatty acids to support energy demands.Citation37 The Family_XIII_AD3011_group has probiotic properties and has been shown to improve intestinal health.Citation38 Escherichia-Shigella, Lachnoclostridium and Clostridioides have been reported to be related to promoting inflammation and inflammatory diseases.Citation39–41 In the present study, we found an increase in the relative abundances of Terrisporobacter, Catenibacterium and Family_XIII_AD3011_group and a decrease in the relative abundances of Escherichia-Shigella, Lachnoclostridium and Clostridioides in the G1000 group, which reflects the ability to improve digestion and energy supply with a lower risk of inflammation and disease in weaned piglets.
Conclusion
In conclusion, our results showed that dietary supplementation with GCP at 1000 mg/kg can improve growth performance and intestinal health (immunity, barrier function, digestive enzyme activity, pH and jejunal microbial composition) of weaned piglets, thus indicating that GCP represents a potential alternative to antibiotics as a dietary supplement to improve growth performance.
Acknowledgments
This work was supported by the National Key R&D Program of China (No. 2022YFE0111100, No.2017YFE0129900) and Henan Provincial Science Popularization Fund Project (No. 183400410012).
Disclosure statement
No potential conflict of interest was reported by the authors.
References
- Zhao S, Lin H, Li S, et al. Modeling of chilled/supercooled pork storage quality based on the entropy weight method. Animals (Basel). 2022;12(11):1415.
- Jia G, Li W, Meng J, Tan H, Feng Y. Non-contact evaluation of pigs’ body temperature incorporating environmental factors. Sensors (Basel). 2020;20(15):4282.
- Upadhaya SD, Kim IH. The impact of weaning stress on gut health and the mechanistic aspects of several feed additives contributing to improved gut health function in weanling piglets: a review. Animals (Basel). 2021;11(8):2418.
- Wellington MO, Hamonic K, Krone JEC, et al. Effect of dietary fiber and threonine content on intestinal barrier function in pigs challenged with either systemic E. coli lipopolysaccharide or enteric Salmonella typhimurium. J Anim Sci Biotechnol. 2020;11(1):38.
- Liu Y, Espinosa CD, Abelilla JJ, et al. Non-antibiotic feed additives in diets for pigs: a review. Anim Nutr. 2018;4(2):113–125.
- Feng Y, Wassie T, Gan R, Wu X. Structural characteristics and immunomodulatory effects of sulfated polysaccharides derived from marine algae. Crit Rev Food Sci Nutr. 2023a;63(24):7180–7196.
- Feng Y, Wu Y, Duan R, Wang P, Zhong X, Wu X. Structural characterization and anti-inflammatory effects of Enteromorpha prolifera polysaccharide-Fe/Zn complexes. Int J Biol Macromol. 2023b;253(Pt 6):127166.
- Wassie T, Lu Z, Duan X, et al. Dietary enteromorpha polysaccharide enhances intestinal immune response, integrity, and caecal microbial activity of broiler chickens. Front Nutr. 2021;8:783819.
- Yarley OPN, Kojo AB, Zhou C, et al. Reviews on mechanisms of in vitro antioxidant, antibacterial and anticancer activities of water-soluble plant polysaccharides. Int J Biol Macromol. 2021;183:2262–2271.
- Simayi Z, Rozi P, Yang X, et al. Isolation, structural characterization, biological activity, and application of Glycyrrhiza polysaccharides: Systematic review. Int J Biol Macromol. 2021;183:387–398.
- Zhang C, Li CX, Shao Q, et al. Effects of Glycyrrhiza polysaccharide in diet on growth performance, serum antioxidant capacity, and biochemistry of broilers. Poult Sci. 2021;100(3):100927.
- Chen J, Zhu XQ, Yang L, et al. Effect of Glycyrrhiza uralensis Fisch polysaccharide on growth performance and immunologic function in mice in Ural City, Xinjiang. Asian Pac J Trop Med. 2016;9(11):1078–1083.
- Zhang X, Zhao S, Song X, et al. Inhibition effect of glycyrrhiza polysaccharide (GCP) on tumor growth through regulation of the gut microbiota composition. J Pharmacol Sci. 2018;137(4):324–332.
- Li C, Zhao P, Shao Q, et al. Effects of dietary Glycyrrhiza polysaccharide on growth performance, blood parameters and immunity in weaned piglets. J Anim Physiol Anim Nutr (Berl). 2023;107(1):136–146.
- National Research Council (NRC). 2012. Nutrient Requirements of Swine. 11th ed. Washington, DC: National Academic Press.
- Zhu XM, Liu XY, Xia CG, et al. Effects of dietary Astragalus Propinquus Schischkin polysaccharides on growth performance, immunological parameters, antioxidants responses and inflammation-related gene expression in Channa argus. Comp Biochem Physiol C Toxicol Pharmacol. 2021;249:109121.
- Ao X, Kim, IH, Effects of Achyranthes bidentata polysaccharides on performance, immunity, antioxidant capacity, and meat quality in Pekin ducks. Poult Sci. 2020;99(10):4884–4891.
- Chen J, Long L, Jiang Q, Kang B, Li Y, Yin J. Effects of dietary supplementation of Lycium barbarum polysaccharides on growth performance, immune status, antioxidant capacity and selected microbial populations of weaned piglets. J Anim Physiol Anim Nutr. 2020;104(4):1106–1115.
- Chen P, Lei J, Chen F, Zhou B. Ameliorative effect of urolithin A on d-gal-induced liver and kidney damage in aging mice via its antioxidative, anti-inflammatory and antiapoptotic properties. RSC Adv. 2020;10(14):8027–8038.
- Wang K, Zhang H, Han Q, et al. Effects of astragalus and ginseng polysaccharides on growth performance, immune function and intestinal barrier in weaned piglets challenged with lipopolysaccharide. J Anim Physiol Anim Nutr. 2020;104(4):1096–1105.
- Pu G, Li P, Du T, et al. Adding appropriate fiber in diet increases diversity and metabolic capacity of distal gut microbiota without altering fiber digestibility and growth rate of finishing pig frontiers in microbiology. Front Microbiol. 2020;11:533.
- Yanez-Lemus F, Moraga R, Smith CT, et al. Selenium nanoparticle-enriched and potential probiotic, Lactiplantibacillus plantarum S14 Strain, a diet supplement beneficial for rainbow trout. Biology (Basel). 2022;11(10):1523.
- Wang K, Yang A, Peng X, et al. Linkages of various calcium sources on immune performance, diarrhea rate, intestinal barrier, and post-gut microbial structure and function in piglets. Front Nutr. 2022;9:921773.
- Han J, Zhang F, Bian L. Effects of Acanthopanax senticosus polysaccharide on immune organ indexes, counts of fecal microflora and gastrointestinal pH of immune challenged weaner piglets. J Animal Nur. 2014;26(08):2314–2319.
- Fang L, Bi Y, Wang Z, Jia W. Effects of Gynostemma pentaphyllum polysaccharides on intestinal microflora in Weaned piglet. J Henan Agri Sci. 2017;46(12):122–125. 138.
- Sun X, Cui Y, Su Y, et al. Dietary fiber ameliorates lipopolysaccharide-induced intestinal barrier function damage in piglets by modulation of intestinal microbiome. mSystems. 2021;6(2):e01374-20.
- Chen Y, Zhang H, Li Y, Wang T. Pterostilbene confers protection against diquat-induced intestinal damage with potential regulation of redox status and ferroptosis in broiler chickens. Oxid Med Cell Longev. 2023;2023:8258354–8258315.
- Gao M, Wang J, Lv Z. Supplementing genistein for breeder hens alters the growth performance and intestinal health of offspring. Life (Basel). 2023;13(7):1468.
- Zong X, Xiao X, Kai L, et al. Atractylodis macrocephalae polysaccharides protect against DSS-induced intestinal injury through a novel lncRNA ITSN1-OT1. Int J Biol Macromol. 2021;167:76–84.
- Zhang R, Huang G, Ren Y, et al. Effects of dietary indole-3-carboxaldehyde supplementation on growth performance, intestinal epithelial function, and intestinal microbial composition in weaned piglets. Front Nutr. 2022;9:896815.
- Wu L, Yang B, Yuan X, et al. Regulation and evasion of host immune response by African Swine Fever virus. Front Microbiol. 2021;12:698001.
- Xu Q, Huang S, Xu ZM, et al. Promotion effects of DEHP on hepatocellular carcinoma models: up-regulation of PD-L1 by activating the JAK2/STAT3 pathway. Toxicol Res (Camb). 2021;10(3):376–388.
- Chen L, Cai X, Tan X, Lin G, Yufang MA, Huang Y. Effects of radix pseudostellariae stem and leaf polysaccharide on intestinal immune function, intestinal mucosal morphology and cecum contents flora of weaned piglets. J Anim Nutr. 2017;29(03):1012–1020.
- Tian Z, Ma X, Deng D, Cui Y, Chen W. Influence of nitrogen levels on nutrient transporters and regulators of protein synthesis in small intestinal enterocytes of piglets. J Agric Food Chem. 2019;67(10):2782–2793.
- Zhang S, Ru X, Zhang L, Gonçalves D, Yang H, Xu J. Effects of a high-fat diet on intestinal and gonadal metabolism in female and male sea cucumber Apostichopus japonicus. Biology (Basel). 2023;12(2):212.
- Lin C, Wei Z, Yi Z, Tingting T, Huamao D, Lichun F. Analysis of the effects of nanosilver on bacterial community in the intestinal fluid of silkworms using high-throughput sequencing. Bull Entomol Res. 2020;110(3):309–320.
- Kageyama A, Benno Y. Catenibacterium mitsuokai gen. nov., sp. nov., a gram-positive anaerobic bacterium isolated from human faeces. Int J Syst Evol Microbiol. 2000;50 Pt 4(4):1595–1599.
- Yu S, Zhang G, Liu Z, Wu P, Yu Z, Wang J. Repeated inoculation with fresh rumen fluid before or during weaning modulates the microbiota composition and co-occurrence of the rumen and colon of lambs. BMC Microbiol. 2020;20(1):29.
- Borisov VB, Forte E. Bioenergetics and reactive nitrogen species in bacteria. Int J Mol Sci. 2022;23(13):7321.
- Guo W, Mao B, Tang X, et al. Lactobacillus paracasei CCFM1223 protects against lipopolysaccharide-induced acute liver injury in mice by regulating the “gut-liver” axis. Microorganisms. 2022;10(7):1321.
- Liang T, Li D, Zunong J, et al. Interplay of lymphocytes with the intestinal microbiota in children with nonalcoholic fatty liver disease. Nutrients. 2022;14(21):4641.