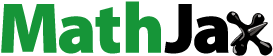
ABSTRACT
The comparative sensory, nutritional, and chemical quality properties of protein hydrolysates produced from mackerel and salmon backbones, heads, and viscera were evaluated. All hydrolysates had high essential amino acid and low biogenic amine levels, implying good raw material quality. The mackerel head-based hydrolysate was rich in ash, influencing the salty taste of the product. Hydrolysates based on viscera were significantly more taste intense and bitter compared with hydrolysates based on backbones and heads. There were only small differences in sensory intensity scores of hydrolysates based on either salmon or mackerel, with no significant difference in bitter taste.
Introduction
In 2018, the Norwegian aquaculture and fisheries industries generated almost 1,000,000 tons of side stream materials, such as heads, backbones, and viscera (Richardsen et al. Citation2019). More than 80% of this was utilized; however, it was mostly as low-cost feed ingredients, and there is a great potential for upgrading this material for human consumption (Stevens et al. Citation2018). All side streams from the fish filleting industry are food grade after the primary processing and represent raw material with a high protein content, including all essential amino acids, vitamins, and minerals (Liaset and Espe Citation2008; Stevens et al. Citation2018).
A promising approach for utilizing marine side stream materials is the production of enzymatic protein hydrolysates using commercial enzymes. Enzymatic protein hydrolysis is a mild processing technology that decreases the molecular weight and increases the water-solubility of the peptides, thus facilitating their recovery as a protein hydrolysate (Panyam and Kilara Citation1996). A major challenge in the production of enzymatic protein hydrolysates is the formation of bitter taste and unpalatable flavors. Bitter taste is related to the liberation of small hydrophobic peptides in the hydrolysis process (Fu et al. Citation2019; Kim and Li-Chan Citation2006). Bitterness may be reduced by proper choice of enzyme and processing conditions (Aspevik et al. Citation2016; Steinsholm et al. Citation2020). In addition to bitter taste, a protein hydrolysate will contain several other tastes and flavors, which are more dependent on the raw material. Water-soluble molecules and metabolites present in the raw material will follow the aqueous hydrolysate phase and may influence the overall product sensory properties (Steinsholm et al. Citation2020).
Fish is highly perishable, and the formation of various metabolites associated with spoilage begins right after harvesting (Prabhakar et al. Citation2020). Thus, proper handling and processing of as-fresh-as-possible raw materials is imperative in products destined for human consumption. Many fish contain significant amounts of trimethylamine-oxide (TMAO) that can be bacterially reduced to trimethylamine (TMA), which has a distinctive stale and unpleasant “fishy” flavor (Wu and Bechtel Citation2008). Moreover, several fish species may develop biogenic amines during storage and processing that can cause food poisoning if present in high amounts. Biogenic amines are formed by bacterial decarboxylation of amino acids; particularly, scombroid fish such as mackerel, are highly susceptible to the formation of histamine during storage (Biji et al. Citation2016; Sone et al. Citation2019). These amines are water-soluble and thus may follow the hydrolysate fraction. Today, mackerel is mostly sold as round frozen in Norway, but the share that is filleted is increasing, generating large amounts of side stream materials available for utilization in novel food applications. Side stream materials from farmed salmon, on the other hand, are highly utilized today; however, the visceral fraction is mostly used for low-value silage production (Richardsen et al. Citation2019). The visceral fraction is highly perishable and susceptible to the formation of TMA and biogenic amines during storage, due to high proteolytic and bacterial activity in the digestive tract.
Several studies have addressed flavor development during protein hydrolysis of marine substrates (Aspevik et al. Citation2016; Dauksas et al. Citation2004; Steinsholm et al. Citation2020). However, to our knowledge, no studies have compared the sensory attributes and chemical properties of hydrolysates based on different side stream fractions from mackerel and salmon at similar hydrolysis conditions. Such studies may improve the understanding of sensory attribute development depending on raw material and hydrolysis parameters. Furthermore, understanding of the chemical composition and quality of the final products is of utmost importance for their use towards human consumption. The objectives of this study were to produce enzymatic protein hydrolysates based on salmon and mackerel heads, backbones, and viscera and compare their chemical composition, nutritional quality, and sensory properties.
Materials and methods
Materials
Salmon (Salmo salar) heads, backbones, and viscera were collected fresh from the filleting factory at Sotra Seafood (Øygarden, Norway), stored cold (4°C), and processed within 48 hours. Mackerel (Scomber scombrus) heads, backbones, and viscera were collected directly from the filleting line at Pelagia (Selje, Norway) in October 2019 and stored frozen (- 22°C) until processing (1 month). On the day of hydrolysis, the raw materials were milled on a Comitrol 1700 processor (Urchel Laboratories Inc., Valparaiso, IN) using a 3K030120U cutting head (3 mm horizontal and 5 mm vertical gaps). The protease used was FoodPro PNL (EC 3.4.24.28, DuPont, Wilmington, DE, USA). All other chemicals used were of analytical grade.
Methods
Chemical analyses
Nitrogen was analyzed by the Kjeldahl method (ISO Citation5983-2 2009), and crude protein was estimated based on N × 6.25. Ash was determined by combustion of raw material at 550°C (ISO Citation5984-2 2002). Dry matter was determined by drying at 103°C (ISO Citation6496-2 1999). Fat content was determined by chloroform-methanol extraction (Bligh and Dyer Citation1959). Peptide size distribution was measured by high-performance liquid chromatography (HPLC) size exclusion chromatography (SEC) (1260 series HPLC Agilent Technologies, Santa Clara, CA, USA) using a Superdex Peptide 10/300GL column (GE Healthcare, Uppsala, Sweden), acetonitrile with TFA as eluent, and UV detection at 190–600 nm. Total amino acid composition was quantified by fluorescence detection with excitation/emission at 250/395 nm. Proteins were hydrolyzed to free amino acids with 6 N HCl and derivatized with 6-aminoquinolyl-N-hydroxysuccinimidyl carbamate before HPLC (column: Waters Accq Tag 3.9 × 150 mm (Cohen and Michaud Citation1993). Free amino acids were measured by HPLC using Waters Pico-Tag method and UV-detection at 254 nm (Bidlingmeyer et al. Citation1987). TMAO and TMA were determined based on the micro-diffusion technique described by Conway and Byrne (Citation1933). The biogenic amines cadaverine, histamine, and putrescine were measured by HPLC according to Mietz and Karmas (Citation1978).
Enzymatic protein hydrolysis
All raw materials, except mackerel viscera, were combined with tap water 1:1 by weight in a 200 L jacketed reactor equipped with overhead stirring. The mixtures were stirred vigorously while raising the temperature to 55°C before adding the enzyme. The enzyme was added at concentrations between 0.17 and 0.22 wt % () based on initial tests to reach similar enzyme activity of 10 U/g protein, and the hydrolysis was run for 50 min at 55°C. The temperature was then raised to 90°C, over approx. 15 minutes, and held at that temperature for 10 minutes to inactivate the enzyme. The temperature was then lowered to 60°C. The coarse bone particles were removed (where applicable) by filtration through a 2 mm sieve before pumping the remaining hydrolysate into a 3-phase separating centrifuge (Flottweg Tricanter Z-23-3). Due to small raw material volume, the mackerel viscera were hydrolyzed on a laboratory scale in a modified R10Bear Varimixer (A/S Wodschow & Co., Brøndby, Denmark). The reaction was stirred (20 rpm) for 60 min at 55°C, and enzyme activity was terminated by heating to 90°C in a microwave oven. The hydrolysis slurry was then centrifuged (20 000 × g, 10 min; Sorvall LYNX 6000, Thermo Scientific, Waltham, MA, USA). The liquid phase was decanted from the solid phase, and the oil and aqueous phases were separated in a separatory funnel. The remaining downstream processing was the same for all test raw materials.
Table 1. Overview of the hydrolysates and enzyme dose used
The aqueous phase was further purified by crossflow filtration using a ceramic filter (0.1 µM ceramic ZrO2/TiO2 “Kleansep” membranes; Orelis Environment, SAS, Salindres, France) to remove small particles and residual lipids. The filtrate was concentrated on a 4-stage falling film evaporator before spray-drying (inlet temperature 220 to 225°C, outlet temperature 94 to 100°C). The purified hydrolysates were isolated as hygroscopic, white to off-white, fluffy powders.
Hydrolysis yield was calculated from the mass of dry powder divided by the mass of raw material:
Hydrolysis yield =
Sensory evaluation
Spray-dried hydrolysates were dissolved in tap water at 1.0% dry matter concentration for sensory evaluation. A highly trained panel of 10 assessors (10 women; aged, 37–64 years) performed a sensory descriptive analysis according to the “Generic Descriptive Analysis” as described by Lawless and Heymann (Citation2010) and the ISO 13299 (Citation2016). The assessors are regularly tested and trained according to ISO 8586 (Citation2012), and the sensory laboratory follows the practice of ISO 8589 (Citation2007). The assessors agreed upon 15 attributes describing the hydrolysate samples (). Samples were served in plastic glasses (20 ml) with a lid at room temperature of 18°C ± 2°C. All attributes were evaluated on an unstructured 15 cm line scale with labeled end points ranging from “no intensity” (1) to “high intensity” (9). Each assessor evaluated all samples at individual speed on a computer system for direct recording of data (EyeQuestion, Software Logic8 BV, Utrecht, The Netherlands).
Table 2. Sensory attributes determined in the hydrolysates and their respective descriptions
In a pretest session before the main test, the assessors were calibrated on samples that were considered the most different on the selected attributes typical for the hydrolysate samples to be tested. All samples were served to the panel coded with a three-digit number in a full balanced design. Tap water and unsalted crackers was available for palate cleansing, and a red light was used in the sensory laboratory to masque differences in appearance between samples.
Statistical analysis
Analysis of variance (ANOVA) of the sensory profiling data was performed using Minitab (v19.2, Pennsylvania State University, PA, USA). First, a two-way mixed effects ANOVA model was conducted to assess differences between products for all sensory attributes. The product was set as a fixed variable, whereas assessor and interaction effects were set as random variables (Næs and Langsrud Citation1998). Mixed effects ANOVA was used to evaluate the individual fixed effects of species and fraction on sensory attributes, still treating assessor as a random variable. Tukey’s pairwise comparison was applied where significant (p < .05) differences were found.
Results and discussion
Chemical composition of raw material
The raw materials contained high levels of protein and fat (). The mackerel heads contained a significant level of ash, indicating high proportion of bones in this raw material. Both salmon and mackerel contain high levels of polyunsaturated fats, so proper handling and processing is imperative to avoid lipid oxidation (Prabhakar et al. Citation2020; Shumilina et al. Citation2016). In this study, the raw materials were collected directly from the processing lines and either frozen immediately (mackerel) or stored cold (4°C; salmon) until processing (within 48 hours) to avoid quality degradation before enzymatic hydrolysis. The raw materials contained high levels of essential amino acids, and especially mackerel backbones were rich in lysine and leucine (). Furthermore, the heads and backbones from both mackerel and salmon contained notable levels of the connective tissue amino acids glycine, proline, and hydroxyproline. The discrepancy between protein levels () and sum of amino acids () is mainly ascribed to the commonly used N-to protein factor of 6.25, which is inaccurate for fish substrates (about 5.2) (Steinsholm et al. Citation2020).
Table 3. Chemical composition (g/100 g) of mackerel and salmon backbones, heads, and viscera
Table 4. Amino acid composition (g/100 g sample) of backbones, heads, and viscera from mackerel and salmon
Chemical composition of protein hydrolysates
Protein hydrolysates were produced based on the different side stream fractions from salmon and mackerel. Products based on mackerel contained higher levels of ash compared with products based on salmon (). The hydrolysate based on mackerel heads had ash levels above 38%, which indicates that further processing, such as nanofiltration, should be performed on these products to reduce the salt content. The amino acid profiles of the hydrolysates () were comparable, with high levels of essential amino acids. The viscera products contained the highest levels of essential amino acids, followed by hydrolysates based on backbones and heads for both species. Furthermore, the products based on heads contained the highest levels of glycine, proline, and hydroxyproline, indicating higher release of connective tissue proteins from this raw material.
Table 5. Chemical composition (g/100 g) of protein hydrolysates based on mackerel (M) and salmon (S) backbones (B), heads (H), and viscera (V)
Table 6. Amino acid composition (g/100 g crude protein) of protein hydrolysates based on mackerel (M) and salmon (S) backbones (B), heads (H), and viscera (V)
All products consisted mainly of peptides smaller than 2000 Da (). The products based on viscera contained significantly higher levels of peptides <200 Da, i.e. mostly free amino acids (), accounting for almost 40% of the total protein content in the viscera products. Peptides <200 Da were less than 10% of the total protein content in head and backbone hydrolysates (). This indicates significant endogenous enzyme activity in the viscera, augmenting the exogenous enzyme hydrolysis process and causing higher levels of free amino acids. Furthermore, all products contained more than 2.4%, on protein basis, non-protein amino acids, which may add to the nutritional properties of the products (Wu Citation2020).
Table 7. Molecular weight distribution (wt%) of hydrolysates based on mackerel (M) and salmon (S) backbones (B), heads (H), and viscera (V)
Table 8. Levels of free amino acids (g/100 g protein) in hydrolysates based on mackerel (M) and salmon (S) backbones (B), heads (H), and viscera (V)
The estimation of biogenic amines and TMA in protein hydrolysates is of great importance, not only from a toxicological point of view, but also as an indication of degree of freshness in the raw material used. Histamine, putrescine, and cadaverine are three common biogenic amines found in seafood, formed by decarboxylation of histidine, ornithine, and lysine, respectively (Biji et al. Citation2016). TMA is formed by bacterial reduction of TMAO (Wu and Bechtel Citation2008). For both salmon and mackerel, the backbone-hydrolysates had highest levels of TMAO, but rather low levels of TMA, indicating fresh raw-material (). On the other hand, no TMAO was detected in the viscera hydrolysates, which suggests that all TMAO had been converted to TMA.
Table 9. Levels of trimethylamine (TMA), trimethylamine-oxide (TMAO) (mg N/100 g), and biogenic amines (mg/kg) in spray-dried protein hydrolysates based on mackerel (M) and salmon (S) backbones (B), heads (H), and viscera (V)
Due to high content of free histidine (), mackerel is highly susceptible to the formation and accumulation of histamine (Lehane and Olley Citation2000). However, there were only negligible differences in histamine levels between the hydrolysates based on salmon and mackerel, and all were below the acceptable toxicity threshold limit of 90 mg/dose (FAO/WHO Citation2012). Furthermore, the average content of biogenic amines was slightly lower in the mackerel hydrolysates compared with the salmon hydrolysates. The mackerel raw materials were only partially thawed before grinding and hydrolysis, whereas the salmon raw materials were stored cold (4°C) up to 48 hours before hydrolysis, possibly explaining this observation. The visceral fractions were not heat-treated prior to the hydrolysis process, and endogenous bacteria, mainly from the digestive tracts, were active in the raw materials during the hydrolysis process. This could explain why the SV hydrolysate contained the highest levels of biogenic amines of all the hydrolysates () and was the only product with detectable cadaverine levels (). High cadaverine levels indicate substantial microbial decarboxylation of free lysine, and formation of cadaverine is one of the main spoilage processes during salmon visceral storage (Shumilina et al. Citation2016). Cadaverine and putrescine are considered to be histamine potentiators; however, the minimum level that potentiates toxicity is unknown (FAO/WHO Citation2012).
Sensory evaluation
Generic descriptive analysis was performed on the hydrolysates to compare the effect of fish species and side stream fraction on sensory attributes. Except for sweet and acidic tastes and shellfish and rancid flavors, all tested attributes varied significantly between the products (). Salt taste demonstrated the largest variance in intensity between products, with MH having the highest value. This may be attributed to the extreme levels of ash in this product (). Furthermore, total flavor intensity and bitter taste demonstrated a large variance between products, with SV and SH having highest and lowest intensity values, respectively, for both attributes. The intensity of rancid flavor was very low, indicating a negligible level of lipid oxidation in the raw material and hydrolysis process.
Table 10. Mean sensory values of protein hydrolysates based on mackerel (M) and salmon (S) backbones (B), heads (H), and viscera (V). Different letters indicate statistical difference (p < 0.05) among the hydrolysates by two-way ANOVA and Tukey’s multiple comparison test
The hydrolysate based on mackerel heads was perceived as the most intense in TMA and fish flavor. This does not coincide with the chemical analysis (), where levels of TMA in the MH-product were low and similar to other product, except SV. The SV product had high levels of TMA () and did also show high-intensity score for TMA flavor, whereas the intensity score for fish flavor was average (). This hydrolysate was also perceived as the most bitter, astringent and taste intense product, possibly overshadowing the fishy flavors thus demonstrating that the formation of fish flavor is complex and not fully understood, also discussed by Steinsholm et al. (Citation2020).
The effects of fish species and side stream fraction on the sensory attributes were evaluated using mixed model ANOVA (). Products based on viscera were significantly more flavor intense and displayed significantly stronger bitter, burned, and astringent attributes compared with products based on heads and backbones (). The strong bitter taste of viscera-based products may be explained by the additional endogenous proteolytic activity, as previously mentioned, adding to the formation of small, bitter peptides. Furthermore, the viscera contained bile that may add to the bitter taste sensation (Dauksas et al. Citation2004). The high contents of free amino acids in the viscera hydrolysates () may also influence the sensory profiles by contributing to bitter, umami, sweet, and acidic tastes (Kirimura et al. Citation1969). The visceral fractions were also associated with the highest intensity of the non-pleasant flavor cloying and astringent mouthfeel (). The products based on heads were significantly saltier compared with backbones and viscera hydrolysates, which can be explained by the high ash contents of the head hydrolysates as discussed above ().
Figure 1. Mean intensity of sensory attributes based on (a) backbones, heads, and viscera and (b) mackerel and salmon. Different letters indicate statistical difference (p < 0.05) based on mixed model ANOVA and Tukey’s multiple comparison test
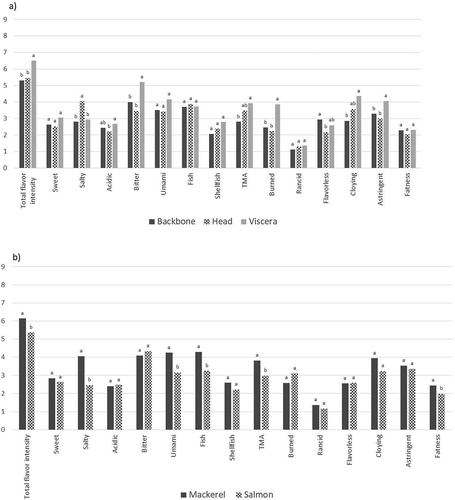
Hydrolysates based on mackerel were in general perceived as more flavor intense, salt, umami, and fish tasting and with a stronger mouthfeel of fatness, compared with hydrolysates based on salmon (), indicating substrate-inherent metabolites influencing the overall taste sensation. On the other hand, there were no significant differences between salmon and mackerel with regards to bitter taste, reflecting similar hydrolysis conditions and substrate-independent release of bitter-peptides, in accordance with previous findings by Steinsholm et al. (Citation2020).
Conclusion
For both salmon and mackerel, hydrolysates based on viscera were the most taste intense and more bitter compared with hydrolysates based on heads and backbones. Furthermore, hydrolysates based on viscera were rich in small peptides and free amino acids, as well as biogenic amines and TMA. Still, the levels of biogenic amines were low for all products, suggesting good quality of the raw materials and appropriateness of the processing methods used towards novel food applications. Hydrolysates based on mackerel were slightly more taste intense and had higher scores for umami, salty, and fish taste as compared to those based on salmon side streams. This may partly be explained by the high levels of ash in the mackerel hydrolysates, especially in the product based on heads and indicate the need for salt removal for human consumption purposes.
Acknowledgments
We want to thank the skillful and accurate laboratory personnel at Nofima BioLab and the technical staff in the sensory panel at Nofima.
Additional information
Funding
References
- Aspevik T, Totland C, Lea P, Oterhals Å. 2016. Sensory and surface-active properties of protein hydrolysates based on Atlantic salmon (Salmo salar) by-products. Process Biochem. 51(8):1006–14.
- Bidlingmeyer BA, Cohen SA, Tarvin TL, Frost B. 1987. A new, rapid, high-sensitivityanalysis of amino acids in food type samples. J Assoc Off Anal Chem. 70(2):241–47.
- Biji KB, Ravishankar CN, Venkateswarlu R, Mohan CO, Gopal TKS. 2016. Biogenic amines in seafood: A review. J Food Sci Technol. 53(5):2210–18.
- Bligh EG, Dyer WJ. 1959. A rapid method for total lipid extraction and purification. Can J Biochem Phys. 37:911–17.
- Cohen SA, Michaud DP. 1993. Synthesis of a fluorescent derivatization reagent, 6-aminoquinolyl-N-hydroxysuccinimidyl carbamate, and its application for the analysis of hydrolysate amino-acids via high-performance liquid-chromatography. Anal Biochem. 211(2):279–87.
- Conway EJ, Byrne A. 1933. An absorption apparatus for the micro-determination of certain volatile substances: the micro-determination of ammonia. Biochem J. 27(2):419–29.
- Dauksas E, Slizyte R, Rustad T, Storrø I. 2004. Bitterness in fish protein hydrolysates and methods for removal. J Aquat Food Prod Technol. 13(2):101–14.
- FAO/WHO. 2012. Public health risks of histamine and other biogenic amines from fish and fishery products. Joint FAO/WHO expert meeting report. Rome (Italy)
- Fu Y, Chen JR, Bak KH, Lametsch R. 2019. Valorisation of protein hydrolysates from animal by-products - perspectives on bitter taste and debittering methods: A review. Int J Food Sci Tech. 54(4):978–86.
- ISO 13299. 2016. Sensory analysis - Methodology - General guidance for establishing a sensory profile. International Organization for Standardization, Geneva (Switzerland)
- ISO 5983-2. 2009. Animal feeding stuffs - Determination of nitrogen content and calculation of crude protein content. Part 2: block digestion and steam distillation method. International Organization for Standardization, Geneva (Switzerland).
- ISO 5984-2. 2002. Animal feeding stuffs - Determination of crude ash. International Organization for Standardization, Geneva (Switzerland).
- ISO 6496-2. 1999. Animal feeding stuffs - Determination of moisture and other volatile matter content. International Organization for Standardization, Geneva (Switzerland).
- ISO 8586. 2012. Sensory Analysis - General guidelines for the selection, training and monitoring of selected assessors and expert sensory assessors. International Organization for Standardization, Geneva (Switzerland).
- ISO 8589. 2007. Sensory analysis - General guidance for the design of test rooms. International Organization for Standardization, Geneva (Switzerland).
- Kim H-O, Li-Chan ECY. 2006. Quantitative structure-activity relationship study of bitter peptides. J Agric Food Chem. 54(26):10102–11.
- Kirimura J, Shimizu A, Kimizuka A, Ninomiya T, Katsuya N. 1969. Contribution of peptides and amino acids to the taste of foods. J Agric Food Chem. 17(4):689–95.
- Lawless HT, Heymann H. 2010. Sensory Evaluation of Food. 2nd ed. New York (NY): Springer; p. 596.
- Lehane L, Olley J. 2000. Histamine fish poisoning revisited. Int J Food Microbiol. 58(1):1–37.
- Liaset B, Espe M. 2008. Nutritional composition of soluble and insoluble fractions obtained By enzymatic hydrolysis of fish-raw materials. Process Biochem. 43(1):42–48.
- Mietz JL, Karmas E. 1978. Polyamine and histamine content of rockfish, salmon, lobster, and shrimp as an indicator of decomposition. J Assoc Off Anal Chem. 61(1):139–45.
- Næs T, Langsrud O. 1998. Fixed or random assessors in sensory profiling? Food Qual Prefer. 9(3):145–52.
- Panyam D, Kilara A. 1996. Enhancing the functionality of food proteins by enzymatic modification. Trends Food Sci Technol. 7(4):120–25.
- Prabhakar PK, Vatsa S, Srivastac PP, Pathak SS. 2020. A comprehensive review on freshness of fish and assessment: analytical methods and recent innovations. Food Res Int. 133:109157.
- Richardsen R, Myhre M, Nystøyl R, Strandheim G, Marthinussen A 2019. Analyse av marint restråstoff 2018, SINTEF 2019:00475. Trondheim (Norway). ISBN: 978-82-14-06352-3.
- Shumilina E, Slizyte R, Mozuraityte R, Dykyy A, Stein TA, Dikiy A. 2016. Quality changes of salmon by-products during storage: assessment and quantification by NMR. Food Chem. 211:803–11.
- Sone I, Skara T, Olsen SH. 2019. Factors influencing post-mortem quality, safety and storage stability of mackerel species: A review. Eur Food Res Technol. 245(4):775–91.
- Steinsholm S, Å O, Underhaug J, Måge I, Malmendal A, Aspevik T. 2020. Sensory assessment of fish and chicken protein hydrolysates. Evaluation of NMR metabolomics profiling as a new prediction tool. J Agric Food Chem. 68(12):3881–90.
- Stevens JR, Newton RW, Tlusty M, Little DC. 2018. The rise of aquaculture by-products: increasing food production, value, and sustainability through strategic utilisation. Mar Policy. 90:115–24.
- Wu G. 2020. Important roles of dietary taurine, creatine, carnosine, anserine and 4-hydroxyproline in human nutrition and health. Amino Acids. 52(3):329–60.
- Wu TH, Bechtel PJ. 2008. Ammonia, dimethylamine, trimethylamine and trimethylamine oxide from raw and processed fish by-products. J Aquat Food Prod Technol. 17(1):27–38.