ABSTRACT
Although hematoxylin and eosin (H & E) staining of sectioned embryonic insect material is widely used, it is time-consuming and may not provide sufficient information. We evaluated new staining procedures for embryonic whole mounts of the greater wax moth, Galleria mellonella. We compared a combination of toluidine blue and rhodamine B (TB-RB) to H & E; we also investigated calcofluor white (CFW) staining. TB-RB staining produced staining similar to H & E. TB-RB staining was less time-consuming and improved visualization of the blastoderm and its differentiation into the germ disk and serosa membrane. CFW enhanced details of mitosis in nuclei post-fertilization and stained the primary serosal membrane. Staining of whole mounts with TB-RB and CFW enabled embryonic staging that was more rapid, convenient and effective than the routine approach using H & E and fluorescent probes.
There currently is considerable interest in developing biological control programs for various insect pests. Cold storage of eggs and embryos is a promising strategy for avoiding the cost of the continuous rearing of new organisms while preventing genetic drift. Development of new approaches requires accurate determination of embryonic stages, because different stages respond differently to cryoprotectants and cooling (Roversi et al. Citation2008; Rajamohan et al. Citation2015). One insect pest of interest is the greater wax moth, Galleria mellonella.
Information concerning the embryonic development of G. mellonella is sparse. One difficulty has been a lack of suitable histotechnical methods. If specimens are embedded and sectioned, it often is difficult to obtain suitable planes of section through the embryo. One approach has been to use a whole mount procedure (Riemann Citation1965; Zucker Citation2006), which eliminates the plane of section problem, but the high optical density of yolk granules in the egg severely hinders microscopic analysis. Consequently, yolk granules must be removed manually from the embryo (Wigand et al. Citation1998).
Other issues concern the stain used. Routine hematoxylin and eosin (H & E) staining, which differentiates nuclei and cytoplasm, is not ideal for whole mount specimens, because microscopic digital images suffer from non-uniform illumination (Kuru Citation2014). Hematoxylin is not suitable when fluorescence microscopy is required and disposal of fluorescent DNA dyes is problematic (Jež et al. Citation2013).
We devised a new and simple method for staining whole mount specimens for studying embryonic development. We used toluidine blue (TB)-rhodamine B (RB) staining as a substitute for H & E. TB-RB also permits additional staining with calcofluor white (CFW) if desired. We compared our method to H & E for studying early embryogenesis in whole mount specimens of G. mellonella. Our procedure is generally applicable to insect embryo studies.
TB has been used earlier for studies of morphogenesis and development of insect embryos (Dorn et al. Citation1987; Bilinski et al. Citation1993; Tanigawa et al. Citation2009). RB has been used to study permeability of dechorionated eggs (Mazur et al. Citation1992; Berkebile et al. Citation2000; Rand et al. Citation2010).
We also investigated the use of calcofluor white (CFW), a nontoxic fluorescent (optical) brightener (Herth and Schnepf Citation1980; Harrington and Hageage Citation2003). CFW binds with high affinity to structural polysaccharides, β-1,3 and β-1,4 polysaccharides (cellulose) and chitin (Pavenstädt-Grupp and Ruthmann Citation1989; Rasconi et al. Citation2009). CFW has been used to visualize chitin development in D. melanogaster embryos (Moussian et al. Citation2005), nematodes (Fanelli et al. Citation2005) and yeast (Roncero et al. Citation1988). We considered CFW to be useful for G. mellonella embryos, because chitin-rich cuticles may influence permeabilization during cryopreservation steps.
Material and methods
Egg collection and staging
G. mellonella eggs were obtained from females reared for 6 months at 26 ± 2 °C and 70 ± 10% relative humidity. Eggs were collected on oviposition paper placed on the top of cages containing 100–150 adults with a sex ratio of about 1:1. For precise staging, the oviposition period for the colony females was limited to 30 min. Egg batches then were placed in 10 × 20 cm aerated cages and incubated in a humidified brood chamber with near normal brood nest conditions, i.e., darkness, 30 ± 0.5 °C and 85 ± 2% relative humidity. Time zero was defined as when the fertilized eggs were placed in the brood chamber. Embryogenesis until hatching of G. mellonella requires 6 days (144 h) under these conditions and the age of an embryo is defined as post-oviposition hours (h PO). A total of 360 embryos were obtained for observation of developmental stages. Embryos were studied until hatching; 10−15 embryos were observed at 3 h intervals. Using this procedure, 40–50% of the embryos exhibited the same embryonic characteristics for each interval studied.
Preparation of whole mounts
The chorion layer of eggs was removed chemically using 3% (w/v) sodium hypochlorite for 4–5 min. Dechorionated eggs then were permeabilized briefly by isopropanol, which also removed residual water. Heptane was applied for 10–20 sec to remove the wax layer. Eggs then were washed in phosphate-buffered saline (PBS) and fixed in Carnoy’s fixative (6:3:1 (v/v) methanol:chloroform:acetic acid) for 3–4 h at room temperature (24 °C).
Chemicals and dyes
All chemicals and dyes were purchased from Sigma-Aldrich (Milan, Italy) and used without further purification.
TB-RB staining
The staining solution of TB consisted of 1% (w/v) dye in 70% ethanol. The staining solution of RB consisted of 0.1% (w/v) dye in distilled water. Staining was carried out at 24 °C as follows:
Immerse specimen in TB solution for one of two staining times depending on the complexity of embryonic structure. Stain zygote to germ disk (GD) stages for 3–5 min, or gastrulation to stages of germ band formation for 15–20 min.
Wash vigorously with PBS.
Dip into RB solution for 1 sec; increase time to several seconds for older embryos.
Wash vigorously with PBS.
Dehydrate through 35, 50, 70, 80 and 95% alcohols for 3 min each.
Store dehydrated specimens for up to two days in 70% ethanol at 4 °C for fixation.
Transfer to xylene and agitate the slide until the sample is clear (about 2 sec).
Mount on coverslips with Canada balsam.
H & E staining
Harris’ alum hematoxylin was prepared according to Llewellyn (Citation2005). All staining was carried out at room temperature (24 °C) as follows:
Stain in Harris’ hematoxylin for 6 min.
Wash in distilled water for 5 min.
Differentiate in acid ethanol for 1–3 sec.
Wash in distilled water for 5 min.
Stain with 1% (w/v) eosin for 1–2 min.
Wash in running tap water.
Dehydrate through the alcohols and clear in xylene, as described in the TB-RB protocol.
Mount in Canada balsam.
CFW staining
CFW was dissolved in dimethylsulfoxide (DMSO) to give a 0.5% (w/v) yellow solution, and stored at 4 °C in the dark in a refrigerator. Staining was carried out at room temperature (24 °C) as follows.
Stain specimens separately in CFW, checking for optimal staining time by staining for 18 min, 4 h and overnight. Stain in the dark following the procedure of Rezende et al. (Citation2008).
Wash three times with PBS, pH 6.2.
Re-suspend in 20% (v/v) aqueous glycerol.
Microscopy
We used a Nikon A1 R+ confocal laser scanning microscope with an ALSI system (Nikon, Tokyo, Japan) equipped with an argon 457–514 nm ion laser and a diode-pumped solid-state laser emitting at 561 nm. Emissions at 457 and 514 nm were captured by frame grabber software (NIS Elements; Nikon) and subsequently transferred to Photoshop (Adobe, Tokyo, Japan) for further processing. Confocal recordings were performed with CFW, RB, eosin and TB dyes. Pairs of single images were superimposed for visualization of co-localization. All micrographs shown are sections from serial Z-stack μm apart. CFW and eosin were excited at 514 nm. RB was excited sequentially, first with a laser at 514 nm, then with a xenon lamp laser at 540–525 nm.
Figure 1. Early embryonic development in G. mellonella. a) TB-RB staining showing the structures involved in early embryonic development in G. mellonella at 12–22 h PO. TB staining time influenced the quality of visualization of the structures during early embryonic development: compare TB staining for 3 min (a, a’, a’’) and 20 min (b, b’, b’’). b) Similarity of results from TB-RB (a, b, c) and H & E (d, e, f) staining. TB-RB staining was invisible by confocal alone unless observed first with DIC (TB staining time = 3 min). Panels show fluorescence (fluo) or confocal (conf) alone, or combined with DIC. Scale bars = 100 µm.
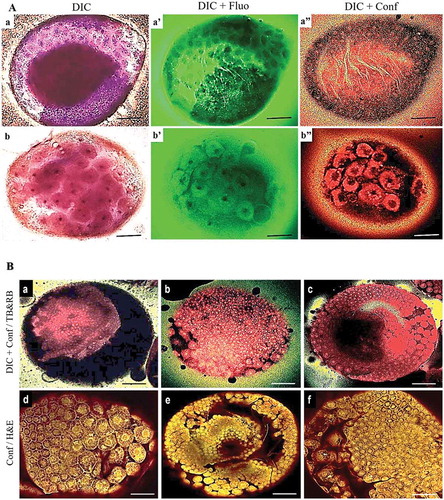
Differential interference contrast (DIC) images were acquired using a 20 × 0.45 NA plan fluor ELWD (Nikon) and Leitz fluor 40 × 0.60 NA PL objective lenses (Leica Lasertechnik GmbH, Heidelberg, Germany).
Fluorescence microscopy images of specimens stained with CFW were recorded using a DIC microscope equipped with an Intensilight C-HGFI/C-HGFIE fiber illuminator with a xenon arc lamp (Nikon) using filters with excitation wavelengths 340–380 nm, 465–495 nm and 540–525 nm. RB was excited sequentially, first with a laser at 514 nm, then with a xenon lamp laser at 540–525 nm.
CWF has a broad excitation/emission spectrum and can be used in combination with broadband filters and most UV or blue light sources (Londershausen et al. Citation1988). A polarizing filter always was used when capturing images and Adobe Photoshop was used to adjust images for better identification of morphological markers, sometimes even changing the color of the embryos. For CFW staining, we integrated the output of two types of microscopy in one photomicrograph (DIC + fluorescence) using the method described by Rezende et al. (Citation2008).
Results
TB-RB vs. H & E staining
The first protocol for TB-RB combination staining for three phases of early embryonic development (0–4.5 h PO, 5–11 h PO, 12–22 h PO) are shown in −. With this procedure, TB stained the nuclei in the germ disk, serosa and vitellophages, while RB stained the cytoplasm. Staining solution was influenced by required exposure time, pH and tissue structure; the time required for TB staining must be increased when working with more developed embryos. A staining time of 3 min was required for pre- and post-blastoderm formation, while 20 min was required for GD formation and its immersion in the yolk. Because TB-RB staining was performed at room temperature, the best staining time depended only on the pH of the staining solution and the tissue structure being stained.
The intensity of cellular staining was similar for both TB-RB and H & E using DIC microscopy (). The embryos stained by the TB-RB method were clearly visible internally and the periplasm nuclei of the surficial layer were visible undergoing synchronous mitotic division with their spindles parallel to the egg surface (). TB-RB staining revealed a change in the cross sectional appearance of extra-embryonic cells from hexagonal during cellular blastoderm () to pentagonal during the final serosa cells formation (). The TB-RB method produced more intense staining than H & E staining. TB-RB clearly distinguished the formation of the cell membrane between each nucleus of the surficial layer and primary yolk cells. After 6 h PO, TB-RB showed the development of the cellular blastoderm with most of nuclei located at the periphery of the egg within the vitelline membrane (pre-cellularization stage) (). TB-RB staining confirmed this specific syncytial type of blastoderm formation in G. mellonella embryos.
Figure 2. DIC of G. mellonella embryos at 2nd development stage (5–11 h PO) stained by TB-RB (a, b, c) and H & E (d, e, f). a) Energid nuclei during the mitosis prior to cellular blastoderm formation. b) Final cellular blastoderm cells appear hexagonal. c) Blastoderm cells are uniform with darker staining than for H & E. d) Cellular blastoderm embryos stained with H & E. e) Blastoderm cells differentiating to the embryonic area (small cells) as GD and extra-embryonic area (larger and more separated cells that will later form the serosa cuticle (Se)) stained with H & E. f) Blastoderm cells differentiating into a smaller embryonic region and a larger extra-embryonic region stained with H & E. Scale bars = 100 µm (a, b, d, e), 50 µm (c, f).
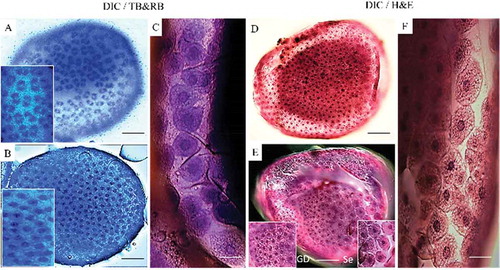
Figure 3. DIC of embryos of G. mellonella during the 3rd developmental stage (12–22.5 h PO) stained with TB-RB. a) GD (white arrowhead) is beginning to separate from serosa cells (black arrows). b) GD (white arrow) after splitting from serosa membrane cells, and vitellophages (white arrowheads) with 1–2 stained nuclei. c) GD sinks and covers vitellophage cells in the center of embryo. Serosal cells have a five-sided appearance. d) Serosal cells were removed manually and blue tissue of GD is surrounding the vitellophage cells. Scale bars = 100 µm.
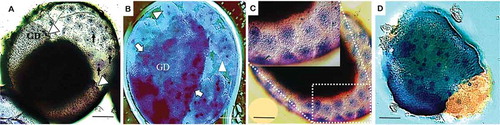
TB stained the nuclei well at early embryonic stages and enabled their visualization in DIC photomicrographs. RB was useful for all microscopic observations, i.e., DIC, DIC + fluorescence, DIC + confocal (). H & E staining was better for observations using the confocal microscope ().
Compared to other methods using molecular probe dyes, the whole mount method was less time-consuming for observing embryonic events in G. mellonella and this advantage would be true for other insect groups. The TB-RB procedure requires less specimen preparation and the staining procedure is faster than H & E. Moreover, TB-RB is more suited to yolk-filled specimens. Because the TB-RB stain also distinguished the main morphological markers at each early embryonic stage, our method may provide sufficient information for finding the optimal stage for cold resistance for developing protocols for cryopreservation of embryos of other insects.
CFW staining
For G. mellonella, the stages of GD formation and its sinking into the yolk provided CFW stained structures (, ʼ). TB staining following CFW did not change this staining pattern (, ′, , ′). CFW stained chitin in the serosa cuticle, especially in combination with TB, using confocal microscopy (). In specimens exposed to light transmitted by a violet 340–380 nm filter for a few minutes, then observed under a Nomarski DIC microscope, visualization of the mitotic spindle during fertilization was achieved (, ′).
Figure 4. Early embryonic development in G. mellonella stained with CFW. a, a′) At 0–4.5 h PO, embryos exhibit CFW staining of mitotic spindle after fertilization. Image was obtained following staining overnight in CFW. b, b′) Embryos at germ disk stage (12–22.5 h PO) with likely CFW stained chitin in germ disk and serosa. Image was obtained following staining with CFW for 4 h. c, c′, d, d′) Embryos at 12–22.5 h PO stained by CFW (4 h) followed by TB (5 min). Fluorescence + DIC (a′, b′, c′, d′), fluorescence + confocal (C, D), or DIC alone (A, B). Scale bars = 100 µm.
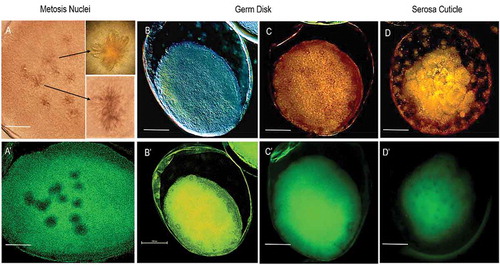
Discussion
TB-RB stained the cleavage nuclei of permeated eggs clearly at pH 2.0–2.5, but they were stained somewhat less well at pH 2.8–3.6. The pH dependent staining of TB was particularly marked in the germ disk and germ band (). TB at pH 7.6–9.0 stained neurons in the insect ganglia (Altman and Tyrer Citation1980). Therefore, the optimum pH may be tissue- and/or stage-specific in insect embryos. Our staining of nuclei in serosa, germ disk and vitellophages of G. mellonella embryos by TB contrasted with unsuccessful attempts to observe serosa nuclei of Aedes aegypti embryos using the fluorescent DAPI dye (Rezende et al. Citation2008). The difficulty with the earlier work possibly was due to either embryo autofluorescence that produces high background staining or an unsuitable fixative. Although the TB-RB protocol showed that TB staining of syncytial blastoderm division interferes with visualization of the regularly spaced extraembryonic nuclei, the serosa nuclei were clearly visible due to embryonic autofluorescence after fixation.
A 20 x objective lens provided the best laser penetration with less light scattering and fewer laser attenuation artifacts than other lenses for confocal microscopy () (Zucker Citation2006). At the early embryonic stages studied, exposure to RB for 4 min was sufficient to visualize cytoplasm and extracellular matrix. Hematoxylin stained nucleic acids and eosin stained proteins nonspecifically.
shows that staining of blastoderm and GD structures was similar with both stains when examined by confocal microscopy, except that the TB-RB stained specimens were observed with DIC light, which is the same procedure used by Rezende et al. (Citation2008). The TB-RB stain also was useful for observing both anatrepsis movement and changes in GD structure during immersion of these structures into yolk and subsequent formation of the germ band. During the GD immersion into the yolk, shortening the staining time of TB to 4–5 min produced similar observations during the anatrepsis movement by fluorescence alone or with DIC (Abidalla Citation2018). Earlier observations of early mitosis of embryonic development in B. mori using H & E staining (Kawamura Citation1978) produced a pattern similar to that presented here using TB-RB.
Our objective for staining with CFW was to visualize primary formation of cuticle membranes based on findings in other species such as the embryo of lepidopteran Manduca sexta (Dorn et al. Citation1987). We found that the CFW protocol was particularly interesting for cryopreservation, because the amnion and serosa membranes may limit the permeability of cryoprotectants (Roversi et al. Citation2008). Confirmation that staining was due to chitin requires molecular studies. CFW is compatible with fluorochromes specific for other cellular components, such as lipid and nuclei (Butt Citation1997).
Acknowledgments
We thank Drs. Pio F. Roversi and Elena Cosi, Centro de Ricerca per l’Agrobiologia e la Pedologia, Florence, Italy for his support of this work and Prof. Christian Sturmbauer, Karl Franzens University, Graz, Austria, for his revision.
Disclosure statement
No potential conflict of interest was reported by the authors.
Additional information
Funding
References
- Abidalla M. 2018. Morphogenesis of early embryonic development in the greater wax moth, Galleria mellonella (Lepidoptera: Pyralidae). J Entomol. 15:1–12.
- Altman JS, Tyrer NM. 1980. Filling selected neurons with cobalt through cut axons. In: Strausfeld NJ, Miller TA, editors. Neuroanatomical techniques. New York: Springer-Verlag; p. 373–402.
- Berkebile D, Chirico J, Leopold RA. 2000. Permeabilization of Cochliomyia hominivorax (Diptera: Calliphoridae) embryos. J Med Entomol. 37:968–972.
- Bilinski SM, Klag J, Kubrakiewicz J. 1993. Morphogenesis of accessory nuclei during final stages of oogenesis in Cosmoconus meridionator (Hymenoptera: Ichneumonidae). Roux’s Arch Dev Biol. 203:100–103.
- Butt TM. 1997. Complementary techniques: fluorescence microscopy. In: Lacey LA, editor. Manual of techniques in insect pathology. Academic Press, New York; p. 355–365.
- Dorn A, Bishoff ST, Gilbert LI. 1987. An incremental analysis of the embryonic development of the tobacco hornworm. Manduca Sexta Int J Invertebr Repr Dev. 11:137–157.
- Fanelli E, Di Vito M, Jones JT, De Giorgi C. 2005. Analysis of chitin synthase function in a plant parasitic nematode, Meloidogyne artiellia, using RNAi. Gene. 349:87–95.
- Harrington BJ, Hageage GJ. 2003. Calcofluor white: a review of its uses and applications in clinical mycology and parasitology. Lab Med. 5:361–367.
- Herth W, Schnepf E. 1980. The fluorochrome, calcofluor white, binds oriented to structural polysaccharide fibrils. Protoplasma. 105:129–133.
- Jež M, Bas T, Veber M, Košir A, Dominko T, Page R, Rožman P. 2013. The hazards of DAPI photoconversion: effects of dye, mounting media and fixative, and how to minimize the problem. Histochem Cell Biol. 139:195–204.
- Kawamura N. 1978. The early embryonic mitosis in normal and cooled eggs of the silkworm, Bombyx mori. J. Morphol. 158:57–71.
- Kuru K. 2014. Optimization and enhancement of H & E stained microscopical images by applying bilinear interpolation method on lab color mode. Theor Biol Med Mod. 11:9.
- Llewellyn BD. 2005. Harris’ alum hematoxylin. Stains File. [accessed 2018 Oct 4]. http://stainsfile.info/StainsFile/stain/hematoxylin/aluminum/harris.htm.
- Londershausen M, Kammann V, Spindler-Barth M, Spindler KD, Thomas H. 1988. Chitin synthesis in insect cell lines. Insect Biochem Mol Biol. 18:631–636.
- Mazur P, Cole KW, Mahowald AP. 1992. Critical factors affecting the permeabilization of Drosophila embryos by alkanes. Cryobiology. 29:210–239.
- Moussian B, Heinz Schwarz M, Bartoszewski S, Nusslein–Volhard C. 2005. Involvement of chitin in exoskeleton morphogenesis in Drosophila melanogaster. J. Morphol. 264:117–130.
- Pavenstädt-Grupp I, Ruthmann A. 1989. Microscoporidian infection in Pimpla turionellae (Ichneumonidae, Hymenoptera): characteristics and reaction with calcofluor white. Parasitol Res. 76:74–79.
- Rajamohan A, Rinehart JP, Leopold RA. 2015. Stage selection and restricted oviposition period improves cryopreservation of dipteran embryos. Cryobiol. 70:143–149.
- Rand MD, Kearney AL, Daoand J, Clason T. 2010. Permeabilization of Drosophila embryos for introduction of small molecules. Insect Biochem Mol Biol. 40:792–804.
- Rasconi S, Jobard M, Jouve L, Sime–Ngando T. 2009. Use of calcofluor white for detection, identification, and quantification of phytoplanktonic fungal parasites. Appl Env Microbiol. 75:2545–2553.
- Rezende GL, Martins JM, Gentile C, Farnesi LC, Pelajo–Machado M, Peixoto AA, Valle D. 2008. Embryonic desiccation resistance in Aedes aegypti: presumptive role of the chitinized serosal cuticle. BMC Dev Biol. 8:182.
- Riemann JG. 1965. The development of eggs of the screw-worm fly Cochliomyia hominivorax (Coquerel) (Diptera: Calliphoridae) to the blastoderm stage as seen in whole-mount preparations. Biol Bull. 129:329–339.
- Roncero C, Valdivieso MH, Ribas JC, Duran A. 1988. Isolation and characterization of Saccharomyces cerevisiae mutants resistant to calcofluor white. J Bacteriol. 170:1950–1954.
- Roversi PF, Cosi E, Irdani T. 2008. Chill sensitivity and cryopreservation of eggs of the greater wax moth Galleria mellonella (Lepidoptera: Pyralidae). Cryobiol. 56:1–7.
- Tanigawa N, Matsumoto K, Yasuyama K, Numata H, Shiga S. 2009. Early embryonic development and diapause stage in the band-legged ground cricket. Dianemobius nigrofasciatus. Dev Genes Evol. 219:589–596.
- Wigand B, Bucher G, Klingler M. 1998. A simple whole mount technique for looking at Tribolium embryos. Techn Inf. 38:281.
- Zucker RM. 2006. Whole insect and mammalian embryo imaging with confocal microscopy: morphology and apoptosis. Cytometry A. 69:1143–1152.