ABSTRACT
The anatomy of the carotid body (CB) and its nerve supply are important, because it is a potential therapeutic target for treatment of various clinical conditions. Visualization of the CB in situ in fixed human anatomical specimens is hampered by obscuring adipose and connective tissues. We developed a tissue clearing method to optimize identification of the CB. We used single sided carotid bifurcations of six human cadavers fixed long term. Visualization of the CB was accomplished by clearing tissue with xylene. Under incident light, carotid bifurcations exhibited a less transparent, darker colored CB; hematoxylin and eosin stained paraffin sections confirmed its identity. Our visualization of the CB in situ in human carotid bifurcations fixed long term enabled targeted resection and subsequent topographic and morphometric measurements of the CB. Our procedure does not interfere with immunohistochemical staining of sections prepared from such specimens.
The carotid body (CB) is a small, reddish brown ovoid chemoreceptive organ located at the carotid bifurcation. The CB is best known for its role in monitoring blood levels of O2, CO2 and pH by chemosensitive glomus (type I) cells (Richerson and Boron Citation2017). The CB is potentially a therapeutic target, because ablation of the CB, or denervation or stimulation of its afferent nerve, can improve hypertension (Paton et al. Citation2013; Narkiewicz et al. Citation2016), heart failure (Niewiński et al. Citation2013; Del Rio et al. Citation2015), chronic kidney disease (Porzionato et al. Citation2019), insulin resistance/glucose homeostasis (Joyner et al. Citation2018; Sacramento et al. Citation2018) and systemic inflammation (Santos-Almeida et al. Citation2017). Because all of these clinical conditions have been observed during the COVID-19 pandemic, the CB may be involved in this disease as well (Porzionato et al. Citation2020). Consequently, interest in the anatomy of the CB and its nerve supply has increased.
The CB is surrounded by adipose and connective tissues (Watkinson and Gleeson Citation2021), which hampers its visualization in situ in anatomical specimens; therefore, macroscopic recognition and isolation of the CB for further microscopic studies is challenging. To facilitate identification of the CB, we developed a xylene-based tissue clearing method. Our procedure simplifies macroscopic identification of the CB in situ, which facilitates CB resection and makes topographic and morphometric measurements possible. In addition, our procedure does not interfere with immunohistochemical staining for microscopic studies of CBs.
Material and methods
We used single side carotid bifurcations of six human cadavers, two males and four females, average age = 82.8 years. Cadavers were obtained through the body donation program of the Anatomy Department of the University Medical Center Utrecht. All donors provided informed consent prior to death.
Tissues were fixed using 3% (v/v) aqueous formaldehyde (Klinipath, Duiven, The Netherlands) by perfusion of the body through the femoral artery followed by immersion of the body for 4–6 months in the same fixative solution.
During dissection, skin of the neck was removed, ventral neck muscles were transected, carotid sheaths were opened and carotid bifurcations including 1 cm of the common carotid artery and 3 cm of the internal and external carotid arteries were resected. Specimens were approximately 4 × 2 x 1 cm. One specimen showed macroscopic calcification and was decalcified by immersion in 12% (w/v) EDTA disodium salt (Merck, Darmstadt, Germany) in distilled water at pH 7.4 for 13 days. All specimens were stored at 4 °C in 0.1 M phosphate buffer, pH 7.4, containing 15% (w/v) sucrose and 0.05% (w/v) sodium azide until subsequent investigation.
Visualization of the CB in situ was accomplished by clearing tissue with xylene. The specimens were passed through 70, 80, 90, 96 and 100% ethanol, each for 90 min. Specimens then were immersed in three 60 min baths of xylene. After the third xylene bath, specimens were placed in a petri dish, submerged in xylene and inspected macroscopically under incident illumination. Because xylene dissolves many plastics and its fumes are toxic, use of glass or metal equipment and a fume cabinet is required. All carotid bifurcations contained a less transparent, darker colored area that presumably was the CB. All bifurcations were photographed with a linear measure both prior to () and following tissue clearing ().
Figure 1. Carotid bifurcation from group A specimen II. A) Dorsal view of a right side carotid bifurcation prior to xylene treatment. The CB is obscured by the surrounding adipose and connective tissues. B) Dorsal view of a right side carotid bifurcation following xylene tissue clearing that shows the CB in situ. The CB is outlined with a white dotted line and the cranial and caudal margins indicated by continuous blue lines. Only sections within the CB site were cut. C) H & E stained transverse section of a carotid bifurcation that confirms the presence of CB tissue. ICA, internal carotid artery; ECA, external carotid artery.
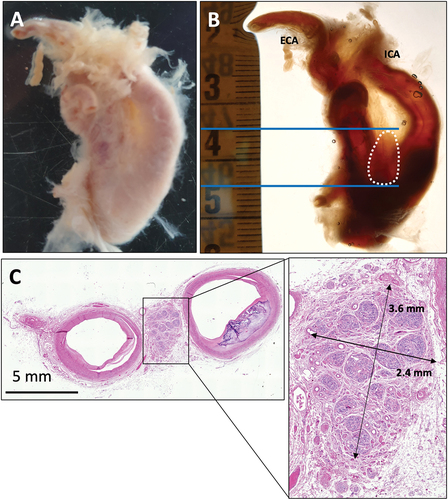
Figure 2. Carotid bifurcation from group B specimen VI. A) Dorsal view of a left side carotid bifurcation following xylene tissue clearing that shows the CB in situ. The CB is outlined with a white dotted line and the cranial and caudal margins are indicated by continuous blue lines. The CBs in group B were resected using a dissection microscope. B) Resected CB. C) H & E stained section of CB. ICA, internal carotid artery; ECA, external carotid artery.
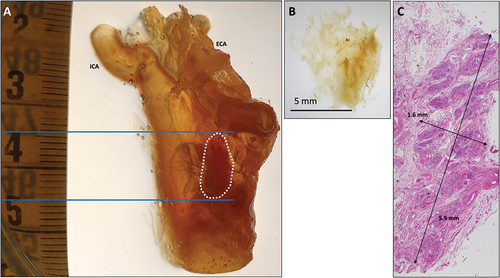
Three carotid bifurcations were embedded in paraffin in toto (group A), with the caudal edge of the common carotid artery toward the cutting plane to produce transverse sections through the CB. The location of the CB was marked on the outside of the paraffin block with reference measurements (). From the remaining three carotid bifurcations (group B), the presumed CBs were resected using an EZ4 dissection microscope (Leica, Nussloch, Germany) () and subsequently embedded in paraffin.
All specimens were sectioned using a 2050 Super Cut microtome (Leica) and serial sections of the presumed CBs were cut at 5 μm at intervals of 275 μm. Sections were placed on glass slides, air dried and heat fixed using a slide drying table (Medax, Kiel, Germany) at 60 °C for 2 h.
All sections were deparaffinized and rehydrated through decreasing alcohols. At every level, the first section was stained with hematoxylin and eosin (H & E) (Cleypool et al. Citation2021) to enable microscopic identification of the CB and for determination of topographic and morphometric CB characteristics.
Immunohistochemistry
Other sections were used for immunohistochemistry to evaluate its compatibility with our xylene based clearing technique. Sections were subjected to antigen retrieval in 0.01 M citrate buffer, pH 6.0, heated to 95 °C on a hot plate for 20 min. After washing several times with tris-buffered saline with 0.05% Tween 20 (Santa Cruz, Dallas, TX), sections were pre-incubated with 5% normal human serum or 5% normal goat serum in tris-buffered saline for 10 min. Sections then were incubated with primary antibodies in tris-buffered saline with 3% (w/v) bovine serum albumin. Immunohistochemical markers commonly used in our department were tested including protein gene product 9.5, S100, neurofilament, beta-III tubulin and tyrosine hydroxylase. contains technical details for these antibodies including dilution, incubation time and temperature. Sections were washed with tris-buffered saline with 0.05% Tween 20 several times and incubated for 30 min at room temperature with secondary antibodies (). Sections were washed with tris-buffered saline with 0.05% Tween 20 and incubated with liquid permanent red (Dako, Glostrup, Denmark) for 10 min. Sections were washed with distilled water, counterstained with hematoxylin, air dried at 60 °C for 90 min and coverslipped using Entellan (Merck, Darmstadt, Germany).
Table 1. Immunohistochemical staining procedures applied to human CB.
Human vagus nerve sections were included as negative and positive controls. Negative controls were obtained by incubation with Tris-buffered saline with 3% bovine serum albumin without primary antibodies.
Results
Macroscopic inspection
Prior to xylene treatment, the CB was obscured due to surrounding adipose and connective tissue (). After clearing with xylene, the CB could be identified in all carotid bifurcations. CBs were apparent as less transparent, darker colored regions positioned dorsally with respect to the carotid bifurcation (). The macroscopic length of the CB was 9–25 mm (mean = 13 mm). No differences in visualization of CBs were observed between decalcified and non-decalcified tissue.
Microscopy
H & E stained sections of groups A and B confirmed the presence of the CB in all cases. The CB contained many capillaries surrounded by large round glomus cells with cytoplasmic vesicles (). Glomus cells were surrounded by smaller type II/satellite or sustentacular cells with elongated nuclei. The CBs of group A were positioned at the macroscopically observed location. No glomus cells were observed in the sections at the extreme edges of the specimens, which indicated that the CB was entirely within the presumed CB site in group A (). Significant amounts of CB tissue were observed at the margins of all samples in group B, which indicated that the CB had not been totally resected ().
Figure 3. CB section from xylene cleared specimen II stained with H & E. Many vascular structures (*) are evident surrounded by clusters of large type I/glomus cells containing vesicles (arrow). The glomus cells in turn are surrounded by smaller type II/satellite or sustentacular cells with elongated nuclei (arrowhead).
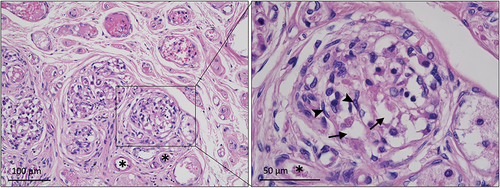
Topography and morphometry
All CBs were located dorsally at the level of the carotid bifurcation. The bifurcation in microscopy sections was defined as the first section that showed bifurcation of the common carotid artery into the internal and external carotid arteries. The center of the CB was used as a reference point to determine the position of the CB with respect to the carotid bifurcation. In two CBs, the center was positioned cranial to the bifurcation (3.6 mm and 11.9 mm), whereas the center of the third was positioned caudally (1.9 mm).
The microscopic average length of CBs in group A was 2.9 mm and the average width was 1.7 × 2.6 mm. In group B, the microscopic average length of the CB was 2.3 mm and the average width was 1.6 × 3.6 mm.
All immunohistochemical staining produced the expected staining patterns in CB tissue sections. Negative controls were unstained.
Discussion
We found that CBs can be visualized in situ in human carotid bifurcations fixed long term using a xylene based tissue clearing method. Our procedure permits macroscopic localization of the CB and enables study of its topography and morphometry. We also found that our method does not interfere with a variety of immunohistochemical stainings ().
Figure 4. Immunostained sections from a CB specimen subjected to xylene based tissue clearing. Pink staining indicates immunoreactivity to primary antibodies. Nuclei are stained purple by hematoxylin counterstain. Human vagus nerve sections were used for negative and positive controls. A) Staining of protein gene product 9.5, a general neural marker. B) Staining of S100, present in type II/satellite or sustentacular cells. C) Staining of low molecular weight neurofilament (70 kDa) present in all nerve fibers. D) Staining of beta-III tubulin IgG2A, a general neural marker. E) Staining of tyrosine hydroxylase, present in sympathetic nerves. Scale bar in panel A applies to all panels.
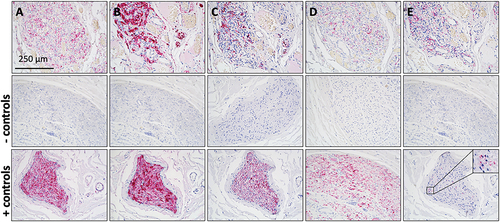
Various macroscopic tissue clearing methods have been reported (Geramizadeh and Razavi Citation2003; Yao et al. Citation2011; Richardson and Lichtman Citation2015). Earlier methods are limited by their incompatibility with tissues fixed long term, they are expensive, they are time-consuming or they require specialized skills and equipment. By contrast, the xylene based tissue clearing method described here is inexpensive, rapid and easy to integrate with standard immunohistochemical staining protocols. Our procedure is an useful alternative for examining tissue obtained from donated bodies that are fixed long term.
In group A specimens, all CBs were located well within the presumed CB sites. The CBs in group B appeared to be incomplete, because significant amounts of CB tissue were observed at the margins of all samples in group B. This might be due to the fact that excision of CBs in group B was complicated by the rigidity of tissue after xylene treatment. Consequently, we intend in the future to locate the CB in situ during xylene treatment as for group A and to discard tissue cranial and caudal to the CB rather than isolating the CB as for group B.
We investigated CB morphometry to illustrate the applicability of our visualization method for future investigation. The isolated CBs of group B, however, were incomplete and underestimate the actual size of the CB. Furthermore, tissue processing for paraffin embedding causes tissue shrinkage (Boonstra et al. Citation1983). Alterations due to tissue processing must be considered when interpreting results.
Because the number, order and duration of ethanol and xylene steps of this tissue clearing procedure are comparable to those used for processing tissues for paraffin embedment (Spencer and Bancroft Citation2013), no interference with immunohistochemical staining procedures was expected; our findings confirmed this for antigens including protein gene product 9.5, S100, neurofilament, beta-III tubulin and tyrosine hydroxylase ().
Disclosure statement
The authors declare no conflict of interest.
References
- Boonstra H, Oosterhuis JW, Oosterhuis AM, Fleuren GJ. 1983. Cervical tissue shrinkage by formaldehyde fixation, paraffin wax embedding, section cutting and mounting. Virchows Arch A Pathol Anat Histopathol. 402:195–201. doi:10.1007/BF00695061.
- Cleypool CGJ, Mackaaij C, Lotgerink Bruinenberg D, Schurink B, Bleys RLAW. 2021. Sympathetic nerve distribution in human lymph nodes. J Anat. 239:282–289. doi:10.1111/joa.13422.
- Del Rio R, Andrade DC, Marcus NJ, Schultz HD. 2015. Selective carotid body ablation in experimental heart failure: a new therapeutic tool to improve cardiorespiratory control. Exp Physiol. 100:136–142. doi:10.1113/expphysiol.2014.079566.
- Geramizadeh B, Razavi A. 2003. Lymph node revealing solution: a new useful method for isolation of minute axillary lymph nodes in breast cancer. Med J Islam Rep Iran. 17:11–14.
- Joyner MJ, Limberg JK, Wehrwein EA, Johnson BD. 2018. Role of the carotid body chemoreceptors in glucose homeostasis and thermoregulation in humans. J Physiol. 596:3079–3085. doi:10.1113/JP274354.
- Narkiewicz K, Ratcliffe LEK, Hart EC, Briant LJB, Chrostowska M, Wolf J, Szyndler A, Hering D, Abdala AP, Manghat N, Burchell AE, Durant C, Lobo MD, Sobotka PA, Patel NK, Leiter JC, Engelman ZJ, Nightingale AK, Paton JFR. 2016. Unilateral Carotid body resection in resistant hypertension: a safety and feasibility trial. J Am Coll Cardiol. 1:313–324. doi:10.1016/j.jacbts.2016.06.004.
- Niewiński P, Janczak D, Rucinski A, Jazwiec P, Sobotka PA, Engelman ZJ, Fudim M, Tubek S, Jankowska EA, Banasiak W, Hart ECJ, Paton JFR, Ponikowski P. 2013. Carotid body removal for treatment of chronic systolic heart failure. Int J Cardiol. 168:2506–2509. doi:10.1016/j.ijcard.2013.03.011.
- Paton JFR, Sobotka PA, Fudim M, Engelman ZJ, Hart ECJ, McBryde FD, Abdala AP, Marina N, Gourine AV, Lobo M, Patel N, Burchell A, Ratcliffe L, Nightingale A. 2013. The carotid body as a therapeutic target for the treatment of sympathetically mediated diseases. Hypertension. 61:5–13. doi:10.1161/HYPERTENSIONAHA.111.00064.
- Porzionato A, Emmi A, Stocco E, Barbon S, Boscolo-Berto R, Macchi V, De Caro R. 2020. The potential role of the carotid body in COVID-19. Am J Physiol Lung Cell Mol Physiol. 319:620–626. doi:10.1152/ajplung.00309.2020.
- Porzionato A, Macchi V, Stecco C, De Caro R. 2019. The carotid sinus nerve-structure, function, and clinical implications. Anat Rec. 302:575–587. doi:10.1002/ar.23829.
- Richardson DS, Lichtman JW. 2015. Clarifying tissue clearing. Cell. 162:246–257. doi:10.1016/j.cell.2015.06.067.
- Richerson GB, Boron WF. 2017. Chemical control of ventilation. In: Boron W, Boulpaep E, editors. Medical physiology. 3rd ed. Elsevier: Philadelphia; p. 709–713.
- Sacramento JF, Chew DJ, Melo BF, Donegá M, Dopson W, Guarino MP, Robinson A, Prieto-Lloret J, Patel S, Holinski BJ, Ramnarain N, Pikov V, Famm K, Conde SV. 2018. Bioelectronic modulation of carotid sinus nerve activity in the rat: a potential therapeutic approach for type 2 diabetes. Diabetologia. 61:700–710. doi:10.1007/s00125-017-4533-7.
- Santos-Almeida FM, Domingos-Souza G, Meschiari CA, Fávaro LC, Becari C, Castania JA, Lopes A, Cunha TM, Moraes DJA, Cunha FQ, Moraes DJA, Cunha FQ, Ulloa L, Kanashiro A, Tezini GCSV, Salgado HC. 2017. Carotid sinus nerve electrical stimulation in conscious rats attenuates systemic inflammation via chemoreceptor activation. Sci Rep. 7:6265. doi:10.1038/s41598-017-06703-0.
- Spencer LT, Bancroft JD. 2013. Principles of tissue processing. In: Suvarna S, Layton C, Bancroft J, editors. Bancroft’s theory and practice of histological techniques. 7th ed. Churchill Livingstone: London, UK; p. 105–109.
- Watkinson JC, Gleeson M. 2021. Neck. In: Standring S, editor. Gray’s anatomy: the anatomical basis of clinical practice. 42nd ed. Elsevier: Amsterdam; p. 587–588.
- Yao Y-F, Wang L, Liu Y-Q, Li J-Y, Gu J. 2011. Lymph node distribution and pattern of metastases in the mesorectum following total mesorectal excision using the modified fat clearing technique. J Clin Pathol. 64:1073–1077. doi:10.1136/jclinpath-2011-200190.