ABSTRACT
Objectives
The objective of this study was to detect success and failure factors for the implementation of passive exoskeletons in agriculture. Exoskeletons have been shown to reduce musculoskeletal loads during lab-based manual tasks, but long-term implementation experiences in agriculture are lacking.
Methods
We analyzed four intervention studies in agriculture focusing on methodological and contextual reasons why the trials were successful or unsuccessful. The study context, attempted intervention, and data collection of each field trial is compared. In the absence of long-term studies investigating the implementation and effectiveness of exoskeletons in agriculture, a set of multi-week pilot trials were initiated among German market vegetable farms and French vineyards from 2019 to 2022. Participant ratings, farm characteristics (e.g. employment duration and payment scheme) and intervention implementation characteristics (e.g. participation in implementation or language barriers) were analyzed using a mixed-methods approach to identify success and failure factors.
Results
The comparison of the studies showed that despite the organizational issues, there were several practical issues that limit the success of exoskeleton use in agriculture. We observed that participant rejection of the intervention is a major barrier to successfully conducting long-term field trials in agriculture. Factors like pain, discomfort, heat stress, or a lack of perceived benefits have been identified as failure factors but also the implementation process itself.
Conclusion
In addition to careful targeting of trial sites and inclusion of participatory elements in the implementation plan, successful implementation of exoskeletons in agriculture requires fundamental human factors development of the exoskeletons themselves. This will require better matching the physical needs of the workers, the production needs of the tasks, and compatibility with the environment.
Introduction
Agriculture is globally the second largest employerCitation1 and recognized as one of the most hazardous industries worldwide, with musculoskeletal disorders (MSDs) being among the most frequent workplace injuries.Citation2,Citation3 Exposure to physical risk factors such as awkward postures and lifting of heavy loads are part of the daily work routine of agricultural workers.Citation4 In the European Union in 2019, strain, sprain, and dislocation accounted for over 27% of non-fatal accidents in agriculture, forestry, and fishery.Citation5 These injuries have substantial personal and economic impacts for farmers.Citation6,Citation7 Although the hierarchy of controls calls for elimination or engineering solutions to address workplace hazards,Citation8 agriculture lags other industries in the development of technical solutions for musculoskeletal loading. Moreover, the prevalence of seasonal and temporary labor in agriculture makes work environment improvements challenging.Citation1 While there is a strong need for technical solutions that can reduce the risk of injury, effective and feasible solutions remain elusive.
Wearable exoskeletons that reduce loading on the trunk have been suggested as a potential exposure-reducing solution that could be portable across landscapes and across multiple tasks. Passive trunk-supporting exoskeletons have been found to reduce musculoskeletal loads during lab-based manual tasks,Citation9–13 and short-term quasi-experimental field trials over a range of agricultural tasks do show some reductions in muscular loading.Citation14 However, trials of a few hours in experimental and quasi-experimental conditions do not provide evidence for effectiveness over the long term under realistic use conditions. This is because some evaluation criteria such as perceived discomfort are better identified over a longer term.Citation15 Exoskeleton pilot studies in agriculture, where farm workers tested exoskeletons for minutes or hours and were then queried about usability and preferences, have identified several barriers to effective long-term use. These include both mismatch between the exoskeleton and a task’s movement requirements or constraints in the task environment, as well as concerns about hindering productivity.Citation16,Citation17 Most existing long-term evaluations of exoskeleton use have taken place in manufacturing contexts,Citation12,Citation18,Citation19 where the task conditions and timing are fairly consistent and controlled. However, these are not generalizable to agriculture, where the work tasks tend to be both dynamic and diverse, involving a variety of machinery and movements.Citation1 In a recent paper outlining a “roadmap” to exoskeleton adoption, Crea et al.Citation15 posit that one of the main barriers to widespread exoskeleton adoption is the lack of convincing evidence of their effectiveness. This study grew out of the identified need for long-term exoskeleton trials specific to agriculture, a context that requires the potential portability and multi-application possibilities promised by exoskeletons, but which had no reported evaluations of long-term exoskeleton trials to date.
In an effort to provide such evidence within agriculture and address the research gap, researchers from different countries (i.e., the authors of this paper) planned and initiated four long-term field trials. However, in two cases these interventions were cut short due to challenges surrounding the acceptance and integration of exoskeletons on farms. Despite enthusiasm on the part of participating farm management, half of the trials were discontinued due to objections from workers regarding the suitability of the exoskeletons for the tasks and working conditions. Historically, publication bias has limited the reports of such “failed” trials and negative results,Citation20 which can result in an evidence base that gives a skewed impression of intervention efficacy.Citation21 Sharing knowledge of what does not work can be just as valuable as sharing what does, and a better understanding of challenges and failures can help others to plan better studies and make better use of research resources towards the ultimate goal: improving workers’ health.
The objective of this paper is to describe the four trial protocols and context, as well as the participants’ objections and specific factors that contributed to discontinuation. The ultimate goal is to provide a deeper analysis and understanding of what can facilitate and hinder exoskeleton implementation to aid occupational safety and health practitioners querying the evidence base for exoskeletons, and also exoskeleton researchers, so they can apply the knowledge in improved study designs that avoid potential pitfalls.
Methods
Overview
This series of four case studies used a mixed-methods approach to synthesize results from four distinct exoskeleton intervention trials in the agricultural sector. Through close investigation of the highly challenging (and in some cases discontinued) field trials, we attempted to investigate the methodological and contextual reasons why the trials were unsuccessful. We approached this without the assumption that exoskeletons were the right solution in agriculture. Rather, we used an inductive perspective to assess broadly what the current limitations might be that are limiting efforts to provide evidence regarding the field effectiveness of exoskeletons in agriculture. In the methods section, the study context, attempted intervention, and data collection of each field trial is described. Via interviews with the farm contacts, several farm and workforce variables were collected for all cases: gender and age of workers, farm size, farm products, payment scheme, duration of work contract, intensity of work contract, and farm organization type. In all cases, convenience sampling was used to recruit participants based on targeted work tasks and willingness to participate.
Exoskeleton models tested
All four case studies used passive trunk-supporting exoskeletons. Three different models were tested: Hapo, Apex, and Liftsuit (see ). The Hapo (ErgoSanté, France) weighs 1.2 kg and includes three contact pads with the body: (1) the chest, (2) the low back, and (3) the upper legs. The Liftsuit (Auxivo AG, Switzerland) uses no rigid bars or poles for energy storage but rather two elastic bands that connect the upper body to the waist belt. The Apex from Herowear (USA) weighs approximately 1.5 kg, depending on the size and type of elastic band. It consists of a backpack-like module with a stringbox connecting to an elastic band, which is in turn connected to the thigh sleeves. Several different elastic bands allow us to adjust both the fit and the magnitude of the support.
Case study 1 – tree fruit and market vegetables
Study context
The first case study took place in an orchard in Potsdam, Germany, for 3 weeks in late May and June 2021. The orchard cultivates a large variety of organic fruit and vegetables on approximately 10 hectares. The work tasks in this orchard are very diverse depending on the season and throughout the day: tasks include weeding, harvesting, and maintenance of the fruit and vegetables. Many of these tasks are coupled with bending forward, lifting, and carrying of loads. The level of mechanization in this orchard is very low.
Procedures for attempted intervention
The implementation of the exoskeletons in the orchard was planned over several months, starting with a farm visit where the exoskeletons were presented to all potential users on the farm. The introduction included testing both Hapo and Apex exoskeletons, giving background information on the devices, planning the trial, and answering questions. A 3-week trial (Tuesday-Thursday) started in May/June 2021 with four participants (including one manager), two males and two females, focusing on acceptance and usability. Participants were instructed to wear the device for the whole working day, and alternated days wearing one of the two exoskeleton models or no exoskeleton. When necessary, participants were instructed they could disengage the support of the exoskeleton (for example, tasks required a greater range of motion than the exoskeletons allowed).
Data collection
Data collected from case 1 included a usability and acceptance questionnaire, and on site observations including photo and video documentation. Questions were the same after all exoskeleton conditions (model A, model B, or no exo) using a 5-point Likert scale to measure several views on comfort and usability. Open questions were used to described pain or discomfort. The questionnaires were completed after every day of the experiment. Informal interviews were conducted at the end of the trial. These were subsequently recorded as field notes and summarized qualitatively. Prior to the start, every participant completed a consent form and the standardized Nordic questionnaire quantifying muscular-skeletal-disorders.
Case study 2 – asparagus production
Study context
The second study took place on a large agricultural holding cultivating white asparagus and other cultures, located in greater Berlin. The trial started in May 2022, which had hot weather and temperatures above 25°C. The work process selected by farm managers for this trial included lifting and handling full boxes of harvested white asparagus weighing around 15 kg each (see , pictures 1–2). This process takes place before the asparagus is washed and sorted for sale. A pallet of boxes is placed next to a conveyor. One worker puts box by box on the conveyor, where a barcode is scanned, and the box is weighed. After that, the boxes are put back on a pallet and filled with water to keep the asparagus in the best condition. This work is done over a period of several hours. Based on an evaluation using the key item method for lifting and carrying loads, the workload can be characterized as heavy, and physical overload is likely. The work process includes bending forward and lifting heavy boxes as shown in .
Procedures for attempted intervention
This implementation was also planned over several months, starting with a farm visit where two farm managers were able to test the exoskeletons, and the trial was explained. The managers then presented the study to the workers. Three exoskeleton models (Hapo, Apex, Liftsuit) were used in the trial, and the three participating male workers tested the exoskeletons a day before the start. This field trial was intended to last for 3 weeks.
Data collection
A one-page questionnaire, translated into Polish, was designed to record a daily picture of the experienced work day, questioning satisfaction and exhaustion. Regardless of whether an exoskeleton was used or not, the workers had to answer the questions. Informal interviews were conducted with the farm manager by phone at the end of the trials. These were subsequently recorded as field notes and summarized qualitatively.
Case study 3 – endive farm
Study context
Similar to the asparagus farm, the selected tasks at the endive farm involved processing the harvested vegetables: Bags of about 3 endives (~500 g) fall onto a turntable, then 10 bags of endives are then put into a cardboard box, which are then loaded on a pallet. A pallet is filled by 80 boxes, and then the process repeats. This work process is illustrated in .
Procedures for attempted intervention
Following the cancellation of the trial in case study 2, an endive farm north of Berlin was recruited to replace the asparagus farm. As a result, there was no long-term planning and communication with the farm management to prepare for the intervention nor was there an advance presentation of the exoskeletons to managers or workers. Instead, the presentation took place the day before the trial was planned to start to adjust the fit, get accustomed to the function, and get first impressions. Three female workers participated in this session, and since none were willing to use the Hapo model, it was excluded from the trial
Data collection
Data collected from case 3 was intended to include a questionnaire with similar questions as in case 2. Also, similar to cases 1 and 2, one informal interview was conducted with the farm manager in person at the end of the trial. This was subsequently recorded as field notes and summarized qualitatively.
Case study 4 - vineyard
Study context
The fourth case study took place in a French vineyard (Château Calon-Segur, St-Estephe, France). Upon observation of the awkward trunk postures, and in consultation with the vineyard management and sick-leave records, three jobs and consequently three work processes were selected for the exoskeleton trial (): (1) vineyard workers performing shoot and bud removal, (2) cellar-workers performing cellar racking, and (3) workshop technician performing welding.
Procedures for attempted intervention
In this case study, two passive back support exoskeletons were tested: Hapo and LiftSuit. Eleven permanent workers (6 women/5 men) excluding managers (8 vineyard-workers, 2 cellar-workers, and 1 workshop technician) volunteered to participate (~50% of the total workforce). Each participant was asked to wear each exoskeleton, respectively, during 1 and 2 hours. Each condition was separated at least by a day. As in case study 1, the implementation of the exoskeletons on the vineyard was planned over several months, starting with a vineyard visit where the exoskeletons were presented to all potential users. The introduction included giving background information on the selected exoskeletons, planning the trial, and answering questions. This field trial lasted 6 weeks between April and May 2022.
Data collection
At the end of each condition using both exoskeletons, participants had to answer a series of questions categorized as ease of use, safety, work, comfort, mobility, productivity, and intention to use. Questionnaire items were adapted from previously published exoskeleton studies.Citation17,Citation22–24
Analysis and synthesis
The four field studies were planned independently and therefore collected different sets of data. Thus, the synthesis summarized and compared the characteristics of each participating farm, and the procedures attempted for each intervention trial. Characteristics and outcomes from the conceptual framework for participatory ergonomics proposed by Burgess-LimerickCitation25 were used to summarize and compare the four cases. In addition to farm and intervention characteristics, the resulting trial durations were quantified, and the feedback from each trial from both participants and management was summarized using qualitative content analysis.
A qualitative cost-benefit-risk analysis was used to bridge the characteristics according to Burgess-Limericks’ framework and the intervention outcomes. According to the methods described by Fischhoff,Citation26 a cost-benefit-risk analysis combines multiple evidence sources to understand sophisticated risk decision-making processes. While “benefit” in an exoskeleton means advantage of wearing exoskeleton (e.g., reduced injury or increased productivity), “cost” may indicate monetary investment and time required to learn and get used to the device. “Risk” refers to hazards and inconveniences that can be caused by wearing exoskeletons, for instance, discomfort, pains, limitation on movement, or insecurity. The cost-benefit-risk analysis is a qualitative estimation of the relative impact of these factors and not based on a monetary equation.
Results
Trial durations across cases
An overview of all the trial durations is presented schematically in , with the timescale normalized to the intervention start date. Two of the studies (case 1 and 4) took place as planned, with case 1 lasting 3 weeks and case 4 lasting 6 weeks. However, the other two (case 2 and 3) only ran for a short period without collecting all the planned end-of-trial data. The case 2 trial was cancelled after a few days. The workers refused to wear the exoskeletons, they did not swap exoskeleton models as planned, and they expressed unhappiness about wearing them. The average working hours on the days within the trial were 7.5 h (). presents a summary of participant ratings and feedback combined with questionnaires and interviews with workers and managers.
Figure 5. Schematic representation of the exoskeleton intervention timeline for each of the investigated cases.
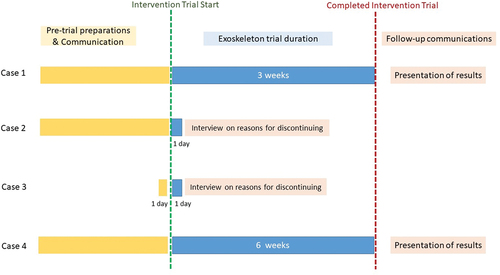
Table 1. Mixed-method summary of participant ratings after trialling exoskeletons for farm tasks from the four included cases.
Synthesizing the impact of farm and employment characteristics
The farm and employment characteristics of the four cases are presented in . In addition to variation in the size and products of participating farms, the cases differed in terms of payment type (hourly vs by-the-kilo “piecework” payment schemes); permanent vs seasonal employment durations, full-time and part-time work; and the organizational structure of the farm (contract farming vs independent owner-operator vs corporate). The workload in the case studies categorizes in either class 2 or 4, based on the OWAS (Ovako Working posture Assessment System).Citation26 Class 4 implies immediate action.
Table 2. Farm and employment characteristics from the four included cases.
Case study 2 included seasonal workers, which could be a characteristic for failure. None of the other farm characteristics presented in was confirmatory for the completion of the full trial. Therefore, there is a need to investigate further implementation characteristics.
Synthesizing the impact of intervention implementation characteristics
The intervention procedures and characteristics of the four cases are presented in . The included cases displayed a range of factors aligned with Burgess-Limerick’s conceptual framework: the locus of decision-making power (solely with management vs with participation of workers); the job title of participants who trialed the exoskeletons; the individual who filled the role of “ergonomist” (i.e., internal to the company vs consultant vs research scientist); exoskeleton model and duration of use; presence of a language barrier; and whether the farm management expressed a willingness to invest in exoskeletons as an occupational health and safety solution, should it prove successful.
Table 3. Intervention implementation characteristics from the four included cases.
The two cases that had no language barrier between the participants and the ergonomic specialists successfully completed the full trial, whereas the uncompleted studies 2 and 3 had characteristics of foreign workers in combination with a language barrier. Due to the small size of the fruit and vegetable farm (case 1), workers were included in the intervention process right from the beginning, and both workers and front-line staff were participants. Both characteristics may also be success factors. Participation in the trials was voluntary and unrewarded.
Despite the organizational issues, there are several practical issues that limited the success of exoskeleton use in agriculture. The first issue may be related to mobility, that is, how easy it is to move and do work with the exoskeleton. In the four case studies, many tasks included a wide variety of movements such as kneeling, squatting, or walking. In case studies 1, 3, and 4, the force produced by exoskeletons to support the back makes these movements almost impossible, especially reaching the ground or squatting. In case studies 3 and 4, this was a reason for the workers to decline wearing the Hapo and Liftsuit, respectively.
The second issue is fit. By the researcher's observation, the visual impression of the fit of the exoskeletons was suboptimal, especially with the Hapo. The fixation of the thigh cuffs was fairly lose. The shoulder straps can be fitted with inflatable pads, but the pads are bulky and might further limit mobility. The Apex is equipped with different shoulder straps (“male” and “female” versions), with rubber bands of different lengths and strength, and two sizes of thigh cuffs allowing a good adjustment for a range of users. The test subjects judged the shoulder straps of the Apex to be too narrow and not sufficiently padded. The Auxivo Liftsuit presents a one-size-fits-all model. However, the adjustment options in the upper and lower size ranges are associated with functional compromises. Poor fit may result in pain and discomfort. Participant feedback links the Liftsuit to chafing on lumbar vertebra, while the Hapo led to discomfort over the transition from chest to shoulders and thighs.
A third issue limiting exoskeleton acceptance may be related to productivity. Facilitation of productivity received an unfavorable opinion from participants in all case studies, suggesting that participants do not perceive exoskeletons help them achieve more in a shorter time. One possible explanation for the Hapo is that setting up engaging and disengaging the exoskeleton support takes time. The researchers observed that many participants struggled to engage the Hapo support mechanism. Interviews and observations have also highlighted the design of the exoskeletons was not yet matching agricultural use and may be considered as a second issue for exoskeleton acceptance. As an example, in case study 1 the workers were unable to carry their tools in the pockets of their trousers, because the thigh bands of the exoskeletons cover them.
The cost-benefit risk analysis, as shown in , does demonstrate benefits, such as the intervention decision-making power and early engagement of workers, but also costs and risks. Together, the evaluation of benefits vs costs and risks will be of perceived value and will influence the acceptance of an intervention ().
Discussion
Summary of results
In the absence of long-term studies investigating the implementation and effectiveness of exoskeletons in agriculture, a set of multi-week pilot trials was initiated among German market vegetable farms and French vineyards from 2019 to 2022. In synthesizing the findings from the four case studies, we noticed several similarities. For example, all the farm management teams were willing to invest in exoskeletons for their workers. In all studies, participation in the research was voluntary and unrewarded. There were also some differences between cases; only three of four studies organized meetings to present exoskeletons and the study to participants before the beginning of the trial. Decision-making power was located within the management in all cases except case 1. All the intervention participants were workers, except case 1 where the manager joined the trial as well. Participating farm workers had hourly contracts, except in case 2 and the case 4 vineyard workers. A language barrier between the workers and the ergonomic specialist was present in cases 2 and 3.
Together, these findings may be interpreted in light of the cost-benefit-risk framework (). It would seem seasonal employment, and piecework contracts in combination with a language barrier could hinder acceptance of an exoskeleton intervention. In contrast, including workers in the intervention decision-making process and the direct involvement of managers would seem to facilitate acceptance, and perhaps eventually adoption. Case studies 2 and 3 were lacking these named benefits, but perceived costs and risks were present. The discontinuation of the trials could be explained by a lack of worker participation.
Our findings suggest that, in agriculture, user exoskeleton acceptance is substantially affected by the work environment, which may limit feelings of comfort and safety. For instance, high temperatures in the workplace (cases 1 and 2) and misfit between exoskeleton and movement (cases 2 and 4) both increased perceived risks of exoskeleton by aggravating discomfort and pain. This confirms results reported in field studies implemented in other occupational sectors, such as the effects in the field (i.e., working environment) are less pronounced than in the laboratory setting.Citation27 For instance in the automotive sector, Hensel and KeilCitation19 have reported that after 4 weeks using a passive lower back exoskeleton among 30 male workers, a strong negative correlation developed between perceived discomfort and intention to use. Second, adverse impact on mobility reported in the present study in cases 1, 2, and 3 and the related negative evaluation on productivity in case 4 denote a low perceived benefit. This is in line with Upasani et al.,Citation16 who noted that first impressions with regard to productivity and pain relief were related to higher intention to use exoskeletons. Third, language barriers in the present study’s cases 2 and May 3, have increased the time investment required to adapt to the exoskeleton and raised the cost of adoption, given case 2 had piecework payment schemes that incentivize a high work pace and productivity, even temporary delays or productivity decrements would have substantially increased the cost of adoption and made it unappealing. Crea et al.Citation15 note that there are relatively few field studies compared to laboratory studies investigating exoskeletons, and we observe that participant rejection of the intervention is a major barrier to successfully conducting long-term field trials. These findings support the notion that participatory ergonomics programs are the most effective means of reducing the incidence of occupational musculoskeletal disorders.Citation25
In the hierarchy of hazard control, there are five levels: elimination, substitution, engineering controls, administrative controls, and finally personal protective equipment (PPE), which ranks last for reducing risk.Citation8 Exoskeletons designed to reduce MSD can be categorized as a PPE, and thus the challenges in implementing them can be viewed through the lens of widely accepted disadvantages of PPE. A large number of ergonomic intervention studies are dealing with technical improvements.Citation28 It is easier to measure the benefits of technical improvement in comparison to preventive measures. For example, a study on eye safety in citrus harvest shows the complexity of successful PPE implementation.Citation29 At the root of this program is the systematic use of community-based preventive marketing and the training of community health workers among citrus harvester using popular education. They mention piecework payment schemes and language barriers that need to be overcome among other factors. None of our case studies was as complex as this community-based intervention program. Consequently, we promote to intensify research on implementation processes for PPE in agriculture in those cases where alternative hazard control is impossible. A process to efficiently analyze risks and to develop and implement suggested controls appears to be an important component of successful participative ergonomic intervention,Citation30,Citation31 and the use of task-specific video footage appears to be a useful part of this process.Citation25,Citation32 Nevertheless, the issue of implementing PPE being the least effective measures may remain the biggest obstacle.
Strengths and limitations
This study gives unique insight into the challenges that can be expected when attempting to implement exoskeletons in agriculture. Rather than simply advocating for widespread adoption of exoskeletons, this study offers a critical re-thinking of the context and methods of implementation and provides descriptions of potential pitfalls so that other researchers may avoid them.
Since this was not initially intended to be a multi-national, multi-location study, the data collection from these four cases is not entirely consistent, and some assumptions have been made when comparing and summarizing the data. Recruitment targets were not met in any of the presented cases, resulting in a collection of small convenience samples. While this does suggest antipathy towards exoskeletons, the specific perceptions, challenges, and reasons to discontinue may not be representative of all agricultural workers or of other contexts, and caution is warranted before generalizing.
Conclusion
Challenges remain in implementing exoskeletons in agriculture. In addition to careful targeting of trial sites and inclusion of participatory elements in the implementation plan, successful implementation of exoskeletons in agriculture requires fundamental human factors development of the exoskeletons themselves. This will require better matching the physical needs of the workers, the production needs of the tasks, and compatibility with the environment. A human factors perspective is a vitally needed addition to the “road map to adoption” of any control attempting to limit MSD risk in agriculture.
Acknowledgments
The authors wish to sincerely thank all the Calon-Segur workers for their active participation and their patience in this study. The authors are also grateful to Marin Reyman and Oceane Tricard for their trust and their help during the implementation of this study. The authors also wish to thank the workers and management from Florahof in Potsdam as well as the other two cooperating farms.
Disclosure statement
No potential conflict of interest was reported by the authors.
Additional information
Funding
References
- Geneva ILO. Safety and Health in Agriculture. ILO Code of Practice. Geneva (CHE): International Labour Office; 2011.
- Fathallah FA. Musculoskeletal disorders in labor-intensive agriculture. Appl Ergon. 2010;41(6):738–743. doi:10.1016/j.apergo.2010.03.003.
- Niu S. Ergonomics and occupational safety and health: an ILO perspective. Appl Ergon. 2010;41(6):744–753. doi:10.1016/j.apergo.2010.03.004.
- Khan MI, Bath B, Kociolek A, Zeng X, Koehncke N, Trask C. Trunk posture exposure patterns among prairie ranch and grain farmers. J Agromedicine. 2020;25(2):210–220. doi:10.1080/1059924X.2019.1659200.
- Eurostat. Accidents at work by NACE rev. 2 activity and type of injury, HSW_N2_07. 2022; https://ec.europa.eu/eurostat/databrowser/view/HSW_N2_07__custom_4032586/default/table?lang=en.
- Bath B, Jaindl B, Dykes L, et al. Get ‘er done: experiences of Canadian farmers living with chronic low back disorders. Physiother Can. 2019;71(1):24–33. doi:10.3138/ptc.2017-65.
- Whelan S, Ruane DJ, McNamara J, Kinsella A, McNamara A. Disability on Irish farms–a real concern. J Agromedicine. 2009;14(2):157–163. doi:10.1080/10599240902813078.
- Dosman J, Hagel L, King N, et al. The hierarchy of control in the epidemic of farm injury. J Agromedicine. 2015;20(3):360–369. doi:10.1080/1059924X.2015.1048401.
- Bosch T, van Eck J, Knitel K, de Looze M. The effects of a passive exoskeleton on muscle activity, discomfort and endurance time in forward bending work. Appl Ergon. 2016;54:212–217. doi:10.1016/j.apergo.2015.12.003.
- Koopman AS, Kingma I, de Looze MP, van Dieen JH, de Looze MP. Effects of a passive back exoskeleton on the mechanical loading of the low-back during symmetric lifting. J Biomech. 2020;102:109486. doi:10.1016/j.jbiomech.2019.109486.
- Koopman AS, Naf M, Baltrusch SJ, et al. Biomechanical evaluation of a new passive back support exoskeleton. J Biomech. 2020;105:109795. doi:10.1016/j.jbiomech.2020.109795.
- Kim S, Nussbaum MA, Smets M. Usability, user acceptance, and health outcomes of arm-support exoskeleton use in automotive assembly: an 18-month field study. J Occup Environ Med. 2022;64(3):202–211. doi:10.1097/JOM.0000000000002438.
- Wei W, Zha S, Xia Y, Gu J, Lin X. A hip active assisted exoskeleton that assists the semi-squat lifting. Applied Sciences. 2020;10(7):2424. doi:10.3390/app10072424.
- Thamsuwan O, Milosavljevic S, Srinivasan D, Trask C. Potential exoskeleton uses for reducing low back muscular activity during farm tasks. Am J Ind Med. 2020;63(11):1017–1028. doi:10.1002/ajim.23180.
- Crea S, Beckerle P, De Looze M, et al. Occupational exoskeletons: A roadmap toward large-scale adoption. Methodology and challenges of bringing exoskeletons to workplaces. Wearable Technol. 2021;2. doi:10.1017/wtc.2021.11.
- Upasani S, Franco R, Niewolny K, Srinivasan D. The potential for exoskeletons to improve health and safety in agriculture—perspectives from service providers. IISE Transact Occupat Ergonom Human Fact. 2019;7(3–4):222–229. doi:10.1080/24725838.2019.1575930.
- Omoniyi A, Trask C, Milosavljevic S, Thamsuwan O. Farmers’ perceptions of exoskeleton use on farms: Finding the right tool for the work(er). Int J Ind Ergon. 2020;80:103036. doi:10.1016/j.ergon.2020.103036.
- Amandels S, Eyndt H, Daenen L, Hermans V. Introduction and testing of a passive exoskeleton in an industrial working environment. Proceedings of the 20th Congress of the International Ergonomics Association (IEA 2018). 2019;820:387–392.
- Hensel R, Keil M. Subjektive Evaluation industrieller Exoskelette im Rahmen von Feldstudien an ausgewählten Arbeitsplätzen. Zeitschrift für Arbeitswissenschaft. 2018;72(4):252–263. doi:10.1007/s41449-018-0122-y.
- DeVito NJ, Goldacre B. Catalogue of bias: publication bias. BMJ Evid Based Med. 2019;24(2):53–54. doi:10.1136/bmjebm-2018-111107.
- MJ P, JAC S, HME M, Egger M. Investigating and dealing with publication bias and other reporting biases in meta-analyses of health research: a review. Res Synth Methods. 2021;12(2):248–259. doi:10.1002/jrsm.1468.
- Huysamen K, Bosch T, de Looze M, Stadler KS, Graf E, O’Sullivan LW. Evaluation of a passive exoskeleton for static upper limb activities. Appl Ergon. 2018;70:148–155. doi:10.1016/j.apergo.2018.02.009.
- Luger T, Bär M, Seibt R, Rieger MA, Steinhilber B. Using a back exoskeleton during industrial and functional tasks—effects on muscle activity, posture, performance, usability, and wearer discomfort in a laboratory trial. Hum Factors. 2021;65(1):5–21. doi:10.1177/00187208211007267.
- Burgess-Limerick R. Participatory ergonomics: evidence and implementation lessons. Appl Ergon. 2018;68:289–293. doi:10.1016/j.apergo.2017.12.009.
- Fischhoff B. The realities of risk-cost-benefit analysis. Science. 2015;350(6260):aaa6516. doi:10.1126/science.aaa6516.
- Karhu O, Kansi P, Kuorinka I. Correcting working postures in industry: a practical method for analysis. Appl Ergon. 1977;8(4):199–201. doi:10.1016/0003-6870(77)90164-8.
- Moeller T, Krell-Roesch J, Woll A, Stein T. Effects of upper-limb exoskeletons designed for use in the working environment—a literature review. Front Robot AI. 2022;9:82. doi:10.3389/frobt.2022.858893.
- Naeini HS, Karuppiah K, Tamrin SB, Koustuv D. Ergonomics in agriculture: an approach in prevention of work-related musculoskeletal disorders (WMSDs). J Agricul Environ Sci. 2014;3:33–51.
- Tovar-Aguilar JA, Monaghan PF, Bryant CA, et al. Improving eye safety in citrus harvest crews through the acceptance of personal protective equipment, community-based participatory research, social marketing, and community health workers. J Agromed. 2014;19(2):107–116. doi:10.1080/1059924X.2014.884397.
- Carayon P, Haims MC, Hoonakker PLT, Swanson NG. Intervention research for reducing musculoskeletal injuries. Proceed Human Factor Ergonom Soc Ann Meet. 2000;44(10):169–172. doi:10.1177/154193120004401011.
- Laing AC, Frazer MB, Cole DC, Kerr MS, Wells RP, Norman RW. Study of the effectiveness of a participatory ergonomics intervention in reducing worker pain severity through physical exposure pathways. Ergonom. 2005;48(2):150–170. doi:10.1080/00140130512331325727.
- St-Vincent M, Lortie M, Chicoine D. Participatory ergonomics training in the manufacturing sector and ergonomic analysis tools. Relat Industrielles. 2002;56(3):491–515. doi:10.7202/000080ar.