ABSTRACT
Introduction: Female sex hormones have vasorelaxing effects in non-pregnant and pregnant women. We aimed to investigate the effect of controlled ovarian hyperstimulation (COH) for in vitro fertilization (IVF), and early pregnancy, on arterial stiffness as assessed by digital pulse wave analysis (DPA), hypothesizing reduced arterial stiffness as an effect of increased estrogen levels.
Material and methods: A total of 68 women undergoing IVF were examined with DPA before conception and during IVF treatment with COH and embryo transfer (ET), and in gestational week seven in 19 women who became pregnant. Heart rate (HR), mean arterial pressure (MAP) and the DPA variables cardiac ejection elasticity index (EEI), b/a, dicrotic index (DI), d/a and aging index (AI) were measured.
Results: HR was significantly increased at all measuring points (p ≤ 0.003) but MAP only at ET (p 0.007). DPA variables representing large arteries (EEI, b/a) and peripheral arteries (DI, but not d/a), and the global variable AI, indicated increased arterial stiffness at ET compared with baseline (p ≤ 0.035). No DPA variable was significantly changed at pregnancy measurements compared to baseline.
Conclusion: During COH for IVF treatment, DPA showed no changes in arterial stiffness during the follicular phase or in early pregnancy, but increased arterial stiffness in central and peripheral arteries in the early luteal phase. The result suggests a hormonal hemodynamic activation counteracting the effects of estrogen.
Introduction
There is both experimental and epidemiological evidence that female sex hormones have beneficial effects on the vascular system. Receptors for estrogen and progesterone are found in the vascular endothelium and smooth muscle cells (Citation1), through which vascular tone can be regulated (Citation2). Within 3 days of estrogen treatment, ovariectomized ewes show drops in mean arterial blood pressure (MAP) and total vascular resistance, and associated increases in heart rate (HR) and cardiac output (CO) (Citation3). Aortic compliance is greater in women compared to men of the same age, and the age-dependent degenerative changes of the vessel wall are postponed in women (Citation4). Estrogen, and possibly also progesterone, decreases vascular tone through nitric oxide mediated vasodilation (Citation1) as well as through a structural modification of the vessel wall matrix (Citation5), both of which can explain the gender differences with lower incidence of cardiovascular morbidity in women until menopause (Citation1,Citation2).
A person’s vascular condition can be expressed as arterial stiffness, which describes the buffer capacity of elastic arteries to ensure optimal blood flow and reduce pressure load on vessel walls and target organs. Arterial stiffness is determined by hemodynamic factors, including structural and functional properties, and is a strong independent risk factor of cardiovascular morbidity and mortality (Citation6,Citation7). Arterial stiffness is classically assessed by applanation tonometry and pulse wave analysis (PWA) of peripheral arteries, commonly expressed as pulse wave velocity (PWV) and augmentation index (AIx), which also provides information of endothelial function and arterial pressure wave reflections (Citation8). Digital pulse wave analysis (DPA) is more simple to perform than applanation tonometry and has been suggested as a quick and operator-independent alternative method for estimation of arterial stiffness and endothelial function. DPA variables have shown good correlations to age and cardiovascular pathology and morbidity (Citation9,Citation10). We have previously reported robust repeatability of DPA variables, as well as correlations to the tonometry variables PWV and AIx (Citation11).
Arterial vascular properties are affected by hormone replacement therapy (HRT) and endogenous hormonal fluctuations. HRT is associated with lower arterial stiffness (Citation12), which is in congruence with current evidence of a beneficial effect of HRT on cardiovascular risk, at least during the first decade after menopause (Citation13). In controlled ovarian hyperstimulation (COH) for in vitro fertilization (IVF), multiple follicles are developed by administration of injectable gonadotrophins (follicle-stimulating hormone [FSH] or human menopausal gonadotropin [hMG]), and follicle-derived estrogens then increase to supranormal levels (Citation14). To our knowledge, there are no previous studies investigating the effect of COH on arterial stiffness.
Pregnancy is a state with increased female sex hormone levels and a hyperkinetic low resistance circulation. Already in the first weeks of pregnancy, total vascular resistance (TVR) is reduced and arterial compliance increased with reduced pulse wave reflection, possibly due to the vasorelaxing effects of estrogen (Citation15–Citation18). A slight increase in TVR is then seen towards term (Citation15). In accordance with TVR changes, arterial stiffness parameters show a U-shaped relation to gestational age (Citation19–Citation22), but studies in very early gestation and studies using DPA in pregnancy are scarce.
In this longitudinal study we aimed to investigate the effect of COH and very early pregnancy on arterial stiffness assessed by DPA. We hypothesized that COH and early pregnancy would have vasodilatory effects and reduce arterial stiffness in central and peripheral arteries.
Methods
Patient selection and study protocol
Women seeking for infertility at the Nordic IVF Malmö clinic, Sweden, and whom were planned for IVF treatment, were asked to participate. The clinic is a private facility which treats women who are not entitled to publically financed IVF within the Swedish health care system. To be enrolled in the study, women had to speak and understand Swedish. Exclusion criteria were known cardiovascular disease or diabetes, or ongoing medical treatment with substances having cardiovascular effects. Women with inhalation treatment for asthma or levothyroxine were allowed in the study. The study was approved by the Regional Research Ethics Committee in Lund (Dnr 2014/648) and all women gave their oral and written informed consent. The women were not exposed to any deviation from the planned IVF treatment and no medical interventions or invasive procedures were performed because of the study.
Arterial stiffness measurements were performed at the first out-patient visit to the clinic (basal measurement), then at the first ultrasound scan during ovarian stimulation (day 7–8 of FSH or hMG treatment, stimulation measurement), and a third time after embryo transfer (ET) (ET measurement). In women who became pregnant, ultrasonography was booked in gestational week seven and DPA measurements were performed then.
Controlled ovarian hyperstimulation
In preparation for IVF treatment, two different protocols were used, a long gonadotropin-releasing hormone (GnRh) agonist or a short GnRH antagonist protocol. Women who received the long protocol were treated with the GnRH agonist nafarelin nasal spray 200 μg/dose (Synarela®, Pfizer AB, Sollentuna, Sweden). Nafarelin nasal spray was started on cycle day 21 in the menstrual cycle preceding ovarian IVF stimulation and given in a dose of 400 μg twice daily. In women following the short protocol, the GnRh antagonist ganirelix 0.25 mg (Orgalutran®, Merck Sharp & Dohme AB, Stockholm, Sweden) was injected subcutaneously daily from stimulation day 5 until the day of human chorionic gonadotropin (hCG) administration.
For ovarian stimulation, gonadotrophins were given, either as follitropin alfa (GONAL-f®, Merck Sharp & Dohme AB, Stockholm, Sweden; Bemfola®, Gedeon Richter Plc, Budapest, Hungary), follitropin beta (Puregon®, Merck Sharp & Dohme AB, Stockholm, Sweden), menotropin (Menopur®, Ferring Läkemedel AB, Malmö, Sweden), or urofollitropin (Fostimon®, HB Medical ApS, Hörsholm, Denmark). The follicular growth was followed by serial transvaginal ultrasound scans and oocyte maturation was induced with recombinant hCG (Ovitrelle®, 250 μg, Merck Sharp & Dohme AB, Stockholm, Sweden) when the mean diameters of leading follicles were 18–20 mm. Oocyte retrieval was then scheduled 36 h after the hCG injection. Oocyte retrieval was performed transvaginally under ultrasound guidance in conscious sedation with fentanyl 1 μg/kg IV (Fentanyl®,50 μg/mL, B. Braun Medical AB, Danderyd, Sweden). Propofol 20–40 mg IV (Propofol-®Lipuro, B. Braun Medical AB, Danderyd, Sweden) was given when needed. Embryos were cultured in SAGE 1-StepTM medium (ORIGIO a/s, Måløv, Denmark). Embryo transfer was performed with a Cook Sydney IVF embryo transfer catheter set (Cook Medical, Bloomington, Indiana, USA).
ET was performed on day 2, 3 or 5 after egg retrieval, depending on embryo development. Progesterone vaginal tablets 100 mg (Lutinus®, Ferring Läkemedel AB, Malmö, Sweden) were given for luteal support three times daily until time of pregnancy test. The pregnancy test was performed 20 days after the day of oocyte retrieval.
The ovarian responsiveness to gonadotropin stimulation was estimated with ovarian sensitivity index (OSI), as defined by Huber et al. (Citation23). OSI describes the ratio between oocyte yield and total dose of FSH: number of retrieved oocytes ×1000 divided by the cumulative FSH dose administered. The authors define a poor ovarian response as OSI < 1.697/IU, normal response as OSI 1.697–10.07/IU, and a high response as OSI >10.07/IU, corresponding to mean ± 1SD on the logarithmic curve after antilogarithmation. Thus, when OSI is used as a continuous variable the logarithmic value should be calculated (logOSI).
Digital pulse wave analysis
After resting for 10 min in the supine position in a single room with minimum disturbances, the blood pressure (BP) was measured using an automatic BP monitor (Omron M3, Omron Healthcare Europe, Hoofddorp, The Netherlands). MAP was defined as diastolic BP + (systolic BP − diastolic BP)/3.
When still in the supine position, two consecutive DPA recordings of 70 s each were performed using a Meridian™ DPA photoplethysmograph (Salcor AB, Uppsala, Sweden) connected to a laptop (HP 625, Hewlett Packard, Solna, Sweden). The mean value of the two recordings was used for further calculations. With a pulse oximetry finger clip placed distal on the left index finger, a photoelectric plethysmography (PPG) volume pulse wave curve is created. The compound waveform represents the forward-going pulse wave from the heart (percussion wave) and the reflected wave from peripheral arteries (tidal wave). The pulse wave contour is influenced by the elasticity of the arterial walls and can be mathematically analyzed. The Meridian™ DPA apparatus automatically generates HR and 16 different pulse wave indices of arterial function based on the PPG waveform contour and its second derivative (acceleration PPG, called APG), and can to some extent separate arterial stiffness in central and peripheral vessels (Citation10). The physiological background of PPG contour analysis has previously been described by Millasseau et al. (Citation24), and we have described the physiological and mathematical background of DPA variables and validated the Meridian™ DPA indices (Citation11). Based on our previous findings of repeatability and correlation to applanation tonometry variables (Citation11), we chose to restrict the analysis to five DPA indices: cardiac ejection elasticity index (EEI) and b/a for stiffness in central arteries and left ventricular capacity, dicrotic index (DI) and d/a for peripheral arteries, and aging index (AI) for systemic arterial stiffness. A physiological interpretation of the DPA variables is presented in .
Table 1. Description of digital pulse wave analysis (DPA) variables and effects of physiological conditions.
Since the DPA method cannot separate decreased arterial compliance caused by structural changes of the vessel wall from vasoconstriction or intravascular volume expansion, the term arterial stiffness is used interchangeably in this paper.
Statistical methods
Associations between continuous variables were tested with Kendall tau rank correlation, and paired longitudinal continuous variables compared with Wilcoxon matched-pairs signed-rank test. Comparisons between multiple groups of unpaired continuous variables were performed with the Kruskal–Wallis test, and in two-group comparisons with the Mann-Whitney U test. When significant associations between multiple continuous variables were found, first simple and then multiple linear regression analysis were performed to investigate variable independency. A two-sided p value <0.05 adjusted for ties was considered significant. The StatView version 5.0.1 (SAS Institute, Cary, NC), MedCalc (MedCalc Software, Mariakerke, Belgium) and IBM SPSS version 23.0 (IBM Corp., Armonk, NY) computer software programs were used for statistical calculations.
We have previously shown that some of the DPA variables used in the present study are HR dependent (Citation11). Hence, if a DPA variable showed a statistically significant association with HR at the basal measurement, and if the HR at the intervention measurement (i.e., at ovarian stimulation on day 7–8, at ET, or in pregnancy) had changed significantly, a simple linear regression analysis was performed; if then significant, the DPA variable in question was adjusted to a HR of 75 bpm (DPA@75) with the equation DPA@75 = DPA + C(75-HR). C denotes the slope constant.
Results
A total of 93 women were enrolled in the study. After excluding drop-outs due to not completing the FSH stimulation protocol, insufficient data collection, or data loss (the laptop computer was stolen), 68 women remained for analysis at baseline, 59 women at stimulation measurement, and 52 women who completed ET. shows the demographic characteristics.
Table 2. Demographic data of the study population.
DPA measurements during FSH stimulation were performed on average at day 7 (mean 7, median 7, interquartile range 7–8). A total of 25 women became pregnant, of whom DPA measurements were performed in 19. Pregnancy measurements were performed at 7 + 0 to 7 + 6 weeks of gestation, except in two women where measurements were made at 6, in one woman at 9 and one woman at 10 weeks, respectively.
Using the cut-off values of OSI, 9 women were classed as poor, 49 as normal, and 10 as high ovarian responders.
Baseline measurements
Associations between baseline DPA measurements and maternal age, HR and OSI are shown in . Since EEI and DI were significantly related to HR, and HR significantly increased at interventions (), EEI was adjusted to EEI@75 and DI to DI@75 at these statistical calculations.
Table 3. Associations at baseline measurements between DPA variables and maternal age, heart rate, logarithmic value of ovarian sensitivity index (logOSI) and grade of ovarian response at controlled ovarian hyperstimulation.
Table 4. Hemodynamic effects of oocyte stimulation and pregnancy, compared with basal measurements before conception. For details, see text. Digital pulse wave analysis values are indices without quantitative measures.
EEI and b/a indicated that older age was associated with stiffer large arteries, and DI and d/a indicated stiffer small arteries; the AI indicated a global arterial stiffness (). LogOSI was negatively associated with age (p < 0.0001, tau −0.35), indicating a lower ovarian sensitivity at higher age.
EEI, b/a, d/a and AI, but not DI, indicated a positive association between arterial elasticity and ovarian response. EEI, b/a and AI indicated higher arterial stiffness in poor responders. When including maternal age and logOSI in multiple regression analyses, significant associations with logOSI remained for AI (p = 0.032) and b/a (p = 0.008), but not for EEI (p = 0.14) and d/a (p = 0.61); maternal age remained being an independent determinant of arterial stiffness (p ≤ 0.0015).
There were at baseline measurements no significant differences in DPA variables between women receiving COH according to antagonist (n = 57) or agonist (n = 11) protocols (p ≥ 0.21).
The group who ultimately became pregnant did not differ significantly from the non-pregnant group in BMI (p 0.092), age, MAP, logOSI or baseline DPA measurements (p ≥ 0.25).
Measurements at interventions
and show the cardiovascular changes at measurement points. With exception for HR, which in comparison with the basal measurement increased at all interventions, variables changed significantly only at ET: an increase in MAP was paralleled by increases in arterial stiffness variables representing both peripheral (DI, but not d/a) and central arteries (EEI, b/a) compared to baseline. AI, representing global arterial stiffness, was also significantly changed toward stiffness at ET.
Figure 1. Boxplots showing cardiovascular changes after administration of follicle-stimulation hormone (Stim), at embryo transfer (ET) and in early pregnancy (Gravid) compared to baseline values (Basal). Gray boxes indicate significant changes (p < 0.05) compared with basal measurements, and star symbols denote values outside the panel boxes. HR, heart rate; MAP, mean arterial pressure; AI, aging index; EEI@75, cardiac ejection elasticity index adjusted to heart rate 75 bpm; DI@75, dicrotic index adjusted to heart rate 75 bpm.
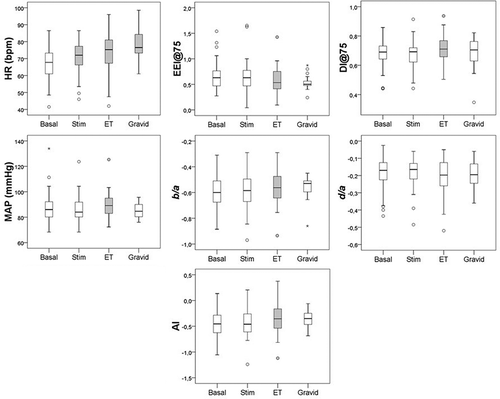
Calculated from baseline to ET (i.e. DPA value at ET minus DPA value at baseline), there were no significant differences in delta DPA values (for d/a p = 0.066, other p ≥ 0.27) between women in the agonist and antagonist protocols. Excluding the 11 women with agonist protocols from the analysis did not change the results at interventions.
There was no significant correlation (for b/a p = 0.07 and tau 0.17, other p ≥ 0.11) between the magnitude of change in DPA variables from baseline to ET (i.e. DPA value at ET minus DPA value at baseline) and logOSI. Including age in multiple regression analyses did not change significance levels, but b/a remained close to significant (logOSI p = 0.066; age p = 0.002).
In the group of women who became pregnant, no DPA variable changed significantly compared with the baseline value, but there was a significant increase in HR.
Discussion
Contrary to our hypotheses, we found an increased global arterial stiffness at ET in early luteal phase after COH for IVF, and no significant changes of DPA variables in early pregnancy. The effect of COH appears modest for all DPA variables, however. Since female sex hormones have vasodilatory effects (Citation25), we expected COH to reduce arterial stiffness at stimulation as well as at ET and early pregnancy.
The hormone levels are expected to be well above normal after a week of FSH stimulation, but the stimulation measurements showed no significant DPA variable changes. It might have been too early in the stimulation course to see any vascular effect. Furthermore, female reproductive hormones have diverse effects through known and unknown mechanisms on multiple organ systems (Citation26). The COH-induced hormonal situation is complex, and as for other steroid hormones, the effects of estrogen and progesterone may depend on individual responses and relative concentrations and bioactivity (Citation27). Manau et al. found significantly reduced TVR after hCG-induced ovulation in IVF cycles (Citation28). However, that study was small and had a proportionally high complication frequency (13%) of ovarian hyperstimulation syndrome, which might be hemodynamically different than uncomplicated COH. No previous studies on arterial stiffness during COH and IVF could enlighten us.
Previous studies of arterial elasticity in the normal menstrual cycle are inconclusive due to small study groups and different methodologies, showing either no changes (Citation29,Citation30) or increased arterial elasticity in the follicular phase, and decreased elasticity in the luteal phase (Citation31–Citation33). The only PPG study performed in the normal menstrual cycle confirmed the latter finding (Citation34), while the few studies using applanation tonometry for assessment of vascular elasticity have shown no changes or increased elasticity in the luteal phase (Citation18,Citation35). Researchers have speculated that an absent vasodilatory effect or increased stiffness in the luteal phase are due to increased levels of progesterone, which is a potential vasoconstrictor (Citation25), or activation of the renin-angiotensin-aldosterone-system (RAAS) or other hormones that counteract the estrogen effect (Citation30,Citation32). These explanations would support the results of increased arterial stiffness at ET in the present study.
The potential influence of RAAS deserves further consideration. Pro-renin exists in high concentrations in ovarian follicles and at hyperstimulation the plasma concentration after hCG is directly related to the number of ovarian follicles (Citation36). In women undergoing COH for IVF, the RAAS is markedly activated with high levels of pro-renin, renin and angiotensinogen in plasma and high urine aldosterone concentration, peaking in the early luteal phase after ovulation induction with hCG (Citation37,Citation38). Angiotensin II increases central and systemic arterial stiffness (Citation39), and we speculate that the increase in arterial stiffness found in our study could be an effect of angiotensin II, eclipsing a possible vasodilatory effect of estrogen. However, d/a, which previously has been shown to reflect the effect of angiotensin (Citation10), was not significantly changed.
Another reason for the increased arterial stiffness at ET could be a volume expansion causing a decrease in vascular compliance. Estrogen and progesterone increase the plasma volume (Citation40) and the DPA technique cannot separate increased stiffness based on structural changes in the arterial wall, vasoconstriction or increased volume load.
The majority of women responded normally to COH, as estimated by the OSI. Multiple factors influence the individual ovarian response to COH, but overall, estrogen levels increase tenfold in IVF compared to concentrations during the normal menstrual cycle, and continue to increase if pregnancy is achieved (Citation14). OSI was not significantly associated with the increase in arterial stiffness for any DPA variable. However, OSI was developed to be a standardized definition of ovarian response and associate to clinical outcome, but not to reflect hormone levels. It might then be a too blunt surrogate variable to use for estimation of endogenous hormone response.
The study confirmed that stiffer arteries relate to older age (Citation10), and also showed that with less elastic arteries the ovarian response was inferior. Age and OSI were both independently associated with arterial stiffness. These findings bring to mind trials to treat with low-dose aspirin to induce vasodilation and increased intraovarian circulation for improved folliculogenesis. A Cochrane meta-analysis from 2016 concludes that there is no evidence in favor of routine use of aspirin to improve pregnancy rates for a general IVF population (Citation41), and the women who became pregnant in the present study did not show significantly different DPA values at baseline. However, if our observations can be reproduced in other studies, it would be worthwhile to explore the selective use of low-dose aspirin in women with stiffer arteries. The myocardium and conduction system of the heart contain sex steroid hormone receptors, and stimulation causes increased HR and ventricular hypertrophy (Citation26). Consequently, HR increases in the first trimester of pregnancy (Citation15). This is in congruence with our results of increased HR during the IVF protocol.
Previous studies of normal hemodynamic adaptation to pregnancy have shown decreased TVR and increased arterial compliance, detectable already at five weeks of gestation (Citation17,Citation42). Previous research with PWA starting in the late first trimester, have shown a decrease in arterial stiffness, expressed as AIx or PPG indices, reaching a nadir in the second trimester, and then increasing toward the end of pregnancy (Citation18–Citation22). In a study with preconceptional comparisons in spontaneous conceptions, reduced AIx was found already at six gestational weeks (Citation43). However, we did not find any significant change of arterial stiffness at 7 weeks of gestation in those women who became pregnant. Although the series of 19 pregnant women is large enough for statistical comparisons with non-parametric statistics (Citation44), there is a possibility of type II error. Furthermore, the study population was a selected group and not necessarily representative of average women. It could also have been too early in pregnancy to detect changes in arterial stiffness with the modality of DPA.
A strength of the study is that we have performed longitudinal measurements from before conception, during the reproductive cycle, and in into early pregnancy. The women were then their own controls. The results should be reviewed in the context of COH, but would have been practically hard to achieve other than in an IVF setting.
A weakness of the study is that no plasma hormone levels were determined, since that is not routine in our standard IVF treatment. The study protocol followed the clinical visits during the IVF treatment, but measurements at oovum pick up were refrained because of perioperative sedative treatment and expected confounding stress.
In summary, we found no changes in arterial stiffness as detected by DPA during the follicular phase or in early pregnancy, but increased systemic arterial stiffness in early luteal phase during COH for IVF treatment. This could be the result of a hemodynamic activation counteracting the effects of estrogen, possibly via the RAAS. In pregnant women monitored at seven weeks, we found no difference in arterial stiffness compared with preconceptional measurements. As previously found in other studies, compared with preconceptional measurements HR showed an increase at all measurement points.
Acknowledgments
Staff at Nordic IVF Malmö are gratefully acknowledged for practical contribution.
Disclosure statement
The authors report no conflict of interest.
Additional information
Funding
References
- Orshal JM, Khalil RA, Gender, sex hormones, and vascular tone. Am J Physiol Regul Integr Comp Physiol. 2004 Feb;286(2):R233–49 doi:10.1152/ajpregu.00338.2003. PubMed PMID: 14707008; eng.
- Skafar DF, Xu R, Morales J, Ram J, Sowers JR. Clinical review 91: female sex hormones and cardiovascular disease in women. J Clin Endocrinol Metab. 1997 Dec;82(12):3913–18 doi:10.1210/jcem.82.12.4443. PubMed PMID: 9398686; eng.
- Magness RR, Parker CR Jr., Rosenfeld CR, Systemic and uterine responses to chronic infusion of estradiol-17 beta. Am J Physiol. 1993 Nov;265(5 Pt 1) :E690–8. PubMed PMID: 8238494; eng doi:10.1152/ajpendo.1993.265.5.E690.
- Sonesson B, Hansen F, Stale H, Lanne T. Compliance and diameter in the human abdominal aorta–the influence of age and sex. Eur J Vasc Surg. 1993 Nov;7(6):690–97. PubMed PMID: 8270073; eng.
- Natoli AK, Medley TL, Ahimastos AA, Drew BG, Thearle DJ, Dilley RJ, Kingwell BA. Sex steroids modulate human aortic smooth muscle cell matrix protein deposition and matrix metalloproteinase expression. Htn. 2005 Nov;46(5):1129–34 doi:10.1161/01.hyp.0000187016.06549.96. PubMed PMID: 16230520; eng.
- Vlachopoulos C, Aznaouridis K, O’Rourke Mf, Safar ME, Baou K, Stefanadis C. Prediction of cardiovascular events and all-cause mortality with central haemodynamics: a systematic review and meta-analysis. Eur Heart J. 2010 Aug;31(15):1865–71 doi:10.1093/eurheartj/ehq024. PubMed PMID: 20197424.
- Vlachopoulos C, Aznaouridis K, Stefanadis C, Prediction of cardiovascular events and all-cause mortality with arterial stiffness: a systematic review and meta-analysis. J Am Coll Cardiol. 2010 Mar 30;55(13):1318–27 doi:10.1016/j.jacc.2009.10.061. PubMed PMID: 20338492.
- Laurent S, Cockcroft J, Van Bortel L, Boutouyrie P, Giannattasio C, Hayoz D, Pannier B, Vlachopoulos C, Wilkinson I, Struijker-Boudier H. Expert consensus document on arterial stiffness: methodological issues and clinical applications. Eur Heart J. 2006 Nov;27(21):2588–605 doi:10.1093/eurheartj/ehl254. PubMed PMID: 17000623; eng.
- Elgendi M, On the analysis of fingertip photoplethysmogram signals. Curr Cardiol Rev. 2012 Feb;8(1):14–25. PubMed PMID: 22845812; PubMed Central PMCID: PMCPmc3394104. eng.
- Takazawa K, Tanaka N, Fujita M, Matsuoka O, Saiki T, Aikawa M, Tamura S, Ibukiyama C. Assessment of vasoactive agents and vascular aging by the second derivative of photoplethysmogram waveform. Htn. 1998;32(2):365–70 doi:10.1161/01.hyp.32.2.365.
- Von Wowern E, Ostling G, Nilsson PM, Olofsson P Digital photoplethysmography for assessment of arterial stiffness: repeatability and comparison with applanation tonometry. PLoS One. 2015;10(8):e0135659 doi:10.1371/journal.pone.0135659. PubMed PMID: 26291079; PubMed Central PMCID: PMCPmc4546304. eng.
- Hayward CS, Knight DC, Wren BG, Kelly R. P. Effect of hormone replacement therapy on non-invasive cardiovascular haemodynamics. J Hypertens. 1997 Sep;15(9):987–93. PubMed PMID: 9321746; eng.
- Boardman HM, Hartley L, Eisinga A, Main C, Roque i Figuls M, Bonfill Cosp X, Gabriel Sanchez R, Knight B. Hormone therapy for preventing cardiovascular disease in post-menopausal women. Cochrane Database Syst Rev. 2015 Mar 10;(3):Cd002229 doi:10.1002/14651858.CD002229.pub4. PubMed PMID: 25754617; eng.
- Joo BS, Park SH, An BM, Kim KS, Moon SE, Moon HS. Serum estradiol levels during controlled ovarian hyperstimulation influence the pregnancy outcome of in vitro fertilization in a concentration-dependent manner. Fertil Steril. 2010 Feb;93(2):442–46 doi:10.1016/j.fertnstert.2009.02.066. PubMed PMID: 19394001; eng.
- Melchiorre K, Sharma R, Thilaganathan B, Cardiac structure and function in normal pregnancy. Curr Opin Obstet Gynecol. 2012 Dec;24(6):413–21 doi:10.1097/GCO.0b013e328359826f. PubMed PMID: 23000697; eng.
- Poppas A, Shroff SG, Korcarz CE, Hibbard JU, Berger DS, Lindheimer MD, Lang RM. Serial assessment of the cardiovascular system in normal pregnancy. Role of arterial compliance and pulsatile arterial load. Circulation. 1997 May 20;95(10):2407–15. PubMed PMID: 9170404; eng.
- Spaanderman ME, Willekes C, Hoeks AP, Ekhart TH, Peeters LL. The effect of pregnancy on the compliance of large arteries and veins in healthy parous control subjects and women with a history of preeclampsia. Am J Obstet Gynecol. 2000 Nov;183(5):1278–86 doi:10.1067/mob.2000.106750. PubMed PMID: 11084578; Eng.
- Robb AO, Mills NL, Din JN, Smith IB, Paterson F, Newby DE, Denison FC. Influence of the menstrual cycle, pregnancy, and preeclampsia on arterial stiffness. Htn. 2009 Jun;53(6):952–58 doi:10.1161/hypertensionaha.109.130898. PubMed PMID: 19398652; eng.
- Macedo ML, Luminoso D, Savvidou MD, McEniery CM, Nicolaides KH. Maternal wave reflections and arterial stiffness in normal pregnancy as assessed by applanation tonometry. Htn. 2008 Apr;51(4):1047–51 doi:10.1161/hypertensionaha.107.106062. PubMed PMID: 18259025; eng.
- Khalil A, Jauniaux E, Cooper D, Harrington K. Pulse wave analysis in normal pregnancy: a prospective longitudinal study. PLoS One. 2009;4(7):e6134 doi:10.1371/journal.pone.0006134. PubMed PMID: 19578538; PubMed Central PMCID: PMCPMC2700961.
- Iacobaeus C, Andolf E, Thorsell M, Bremme K, Jorneskog G, Ostlund E, Kahan T. Longitudinal study of vascular structure and function during normal pregnancy. Ultrasound Obstet Gynecol. 2017 Jan;49(1):46–53 doi:10.1002/uog.17326. PubMed PMID: 27731532; eng.
- Su F, Li Z, Sun X, Han N, Wang L, Luo X. The pulse wave analysis of normal pregnancy: investigating the gestational effects on photoplethysmographic signals. Biomed Mater Eng. 2014;24(1):209–19 doi:10.3233/bme-130801. PubMed PMID: 24211900; eng.
- Huber M, Hadziosmanovic N, Berglund L, Holte J. Using the ovarian sensitivity index to define poor, normal, and high response after controlled ovarian hyperstimulation in the long gonadotropin-releasing hormone-agonist protocol: suggestions for a new principle to solve an old problem. Fertil Steril. 2013 Nov;100(5):1270–76 doi:10.1016/j.fertnstert.2013.06.049. PubMed PMID: 23931964; eng.
- Millasseau SC, Ritter JM, Takazawa K, Chowienczyk PJ. Contour analysis of the photoplethysmographic pulse measured at the finger. J Hypertens. 2006;24:1449–56 doi:10.1097/01.hjh.0000239277.05068.87.
- Dos Santos RL, Da Silva FB, Ribeiro RF, Jr., Stefanon I. Sex hormones in the cardiovascular system. Horm Mol Biol Clin Investig. 2014 May;18(2):89–103 doi:10.1515/hmbci-2013-0048. PubMed PMID: 25390005; eng.
- Turgeon JL, Carr MC, Maki PM, Mendelsohn ME, Wise PM. Complex actions of sex steroids in adipose tissue, the cardiovascular system, and brain: insights from basic science and clinical studies. Endocr Rev. 2006 Oct;27(6):575–605 doi:10.1210/er.2005-0020. PubMed PMID: 16763155; eng.
- Charkoudian N, Influences of female reproductive hormones on sympathetic control of the circulation in humans. Clin Auton Res. 2001 Oct;11(5):295–301. PubMed PMID: 11758795; eng.
- Manau D, Fabregues F, Arroyo V, Jimenez W, Vanrell JA, Balasch J. Hemodynamic changes induced by urinary human chorionic gonadotropin and recombinant luteinizing hormone used for inducing final follicular maturation and luteinization. Fertil Steril. 2002 Dec;78(6):1261–67. PubMed PMID: 12477522; eng.
- Willekes C, Hoogland HJ, Keizer HA, Hoeks AP, Reneman RS. Female sex hormones do not influence arterial wall properties during the normal menstrual cycle. Clin Sci (Lond). 1997 May;92(5):487–91. PubMed PMID: 9176022; eng.
- Ounis-Skali N, Mitchell GF, Solomon CG, Solomon SD, Seely EW. Changes in central arterial pressure waveforms during the normal menstrual cycle. J Investig Med. 2006 Sep;54(6):321–26 doi:10.2310/6650.2006.05055. PubMed PMID: 17134615; eng.
- Williams MR, Westerman RA, Kingwell BA, Paige J, Blombery P. A, Sudhir K, Komesaroff P. A. Variations in endothelial function and arterial compliance during the menstrual cycle. J Clin Endocrinol Metab. 2001 Nov;86(11):5389–95 doi:10.1210/jcem.86.11.8013. PubMed PMID: 11701712; eng.
- Giannattasio C, Failla M, Grappiolo A, Stella ML, Del Bo A, Colombo M, Mancia G. Fluctuations of radial artery distensibility throughout the menstrual cycle. Arterioscler Thromb Vasc Biol. 1999 Aug;19(8):1925–29. PubMed PMID: 10446072; eng.
- Hayashi K, Miyachi M, Seno N, Takahashi K, Yamazaki K, Sugawara J, Yokoi T, Onodera S, Mesaki N. Variations in carotid arterial compliance during the menstrual cycle in young women. Exp Physiol. 2006 Mar;91(2):465–72 doi:10.1113/expphysiol.2005.032011. PubMed PMID: 16407473; eng.
- Madhura M, Sandhya T, Effect of different phases of menstrual cycle on reflection index, stiffness index and pulse wave velocity in healthy subjects. J Clin Diagn Res JCDR. 2014 Sep;8(9):Bc01–4 doi:10.7860/jcdr/2014/7385.4778. PubMed PMID: 25386420; PubMed Central PMCID: PMCPMC4225872. eng.
- Papaioannou TG, Stamatelopoulos KS, Georgiopoulos G, Han N, Wang L, Luo X. Arterial wave reflections during the menstrual cycle of healthy women: a reproducibility study. Htn. 2009 Nov;54(5):1021–27 doi:10.1161/hypertensionaha.109.137703. PubMed PMID: 19738157; eng.
- Sealey JE, Glorioso N, Itskovitz J, Atlas SA, Pitarresi TM, Preibisz JJ, Troffa C, Laragh JH. Ovarian prorenin. Clin Exp Hypertens A. 1987;9(8–9):1435–54. PubMed PMID: 3308200; eng.
- Itskovitz J, Sealey JE, Glorioso N, Laragh J. H, Rosenwaks Z. The ovarian prorenin-angiotensin system. Lessons from IVF. Ann N Y Acad Sci. 1988;541:179–89. PubMed PMID: 3057994; eng.
- Sealey JE, Itskovitz-Eldor J, Rubattu S, James GD, August P, Thaler I, Levron J, Laragh JH. Estradiol- and progesterone-related increases in the renin-aldosterone system: studies during ovarian stimulation and early pregnancy. J Clin Endocrinol Metab. 1994 Jul;79(1):258–64 doi:10.1210/jcem.79.1.8027239. PubMed PMID: 8027239; eng.
- Wilkinson IB, MacCallum H, Hupperetz Pc, van Thoor CJ, Cockcroft JR, Webb DJ. Changes in the derived central pressure waveform and pulse pressure in response to angiotensin II and noradrenaline in man. J Physiol. 2001 Feb 01;530(Pt 3):541–50. PubMed PMID: 11158283; PubMed Central PMCID: PMCPMC2278423. eng.
- Stachenfeld NS, Taylor HS, Effects of estrogen and progesterone administration on extracellular fluid. J Appl physiol(Bethesda, Md: 1985). 2004 Mar;96(3):1011–18 doi:10.1152/japplphysiol.01032.2003. PubMed PMID: 14660504; eng.
- Siristatidis CS, Basios G, Pergialiotis V, Vogiatzi P. Aspirin for in vitro fertilisation. Cochrane Database Syst Rev. 2016 Nov 3;11:Cd004832 doi:10.1002/14651858.CD004832.pub4. PubMed PMID: 27807847; eng.
- Duvekot JJ, Cheriex EC, Pieters FA, Menheere PP, Peeters LH. Early pregnancy changes in hemodynamics and volume homeostasis are consecutive adjustments triggered by a primary fall in systemic vascular tone. Am J Obstet Gynecol. 1993 Dec;169(6):1382–92. PubMed PMID: 8267033; eng.
- Mahendru AA, Everett TR, Wilkinson IB, Lees CC, McEniery CM. Maternal cardiovascular changes from pre-pregnancy to very early pregnancy. J Hypertens. 2012 Nov;30(11):2168–72 doi:10.1097/HJH.0b013e3283588189. PubMed PMID: 22940682; eng.
- Mundry R, Fischer J, Use of statistical programs for nonparametric tests of small samples often leads to incorrect P values: examples fromAnimal behaviour. Anim Behav. 1998 Jul;56(1):256–59 doi:10.1006/anbe.1998.0756. PubMed PMID: 9710485; Eng.