ABSTRACT
Intramyocellular lipid (IMCL) and extramyocellular lipid (EMCL) of ectopic fat in muscles are associated with arterial stiffness in normal-weight individuals. Furthermore, aerobic exercise training-induced changes in IMCL or EMCL content are related to a decrease in arterial stiffness in elderly people. Though arterial stiffness is strongly related with obesity, but the effects of aerobic exercise training on IMCL or EMCL content, with a particular focus on arterial stiffness, in obese individuals remains unclear. Here, we investigated the effects of aerobic exercise training on IMCL or EMCL content and arterial stiffness in obese individuals. First, in a cross-sectional study, we examined the relationship between arterial stiffness and IMCL or EMCL content in 24 overweight and obese men. Secondly, we investigated the effects of aerobic exercise intervention on arterial stiffness and IMCL or EMCL content in 21 overweight and obese men. In the cross-sectional study, EMCL content was positively correlated with baPWV and β-stiffness index, whereas IMCL content was negatively correlated with baPWV. In the intervention study, there were no significant changes in baPWV, β-stiffness index, and IMCL and EMCL contents after aerobic exercise training. However, exercise-induced change in baPWV and β-stiffness index were positively correlated with changes in EMCL content. Moreover, the group of improvements in baPWV was only correlated significantly with reduced EMCL content. These results suggest that IMCL and EMCL contents may affect arterial stiffness in overweight and obese men.
Introduction
Obesity is a major global health problem, and it has been reported by the World Health Organization that worldwide obesity has nearly tripled since 1975 (Citation1). Obesity is an independent predictor of cardiovascular disease (Citation2). Moreover, obesity is intimately related with increases in arterial stiffness (Citation3,Citation4), which is a primary risk factor of cardiovascular disease.
Obesity is defined as an abnormal or excessive fat accumulation following an energy imbalance between the energy consumed and expended. In general, excessive fat is stored in subcutaneous and abdominal visceral fat. Furthermore, excessive fat can be stored in the liver, heart, muscle or pancreas (Citation5), which are referred to ectopic fat.
Recently, there has been increasing interest in ectopic fat as a risk factor of cardiovascular diseases. The intrahepatic triglyceride content in the liver, compared to visceral fat, is a better marker of the metabolic disorders associated with obesity (Citation6). Moreover, an independent relationship was demonstrated between epicardial fat thickness and arterial stiffness (Citation7). These findings suggest that the distribution of fat, not the absolute amount of fat, can play a more important role as a risk factor for cardiovascular disease.
Among the ectopic fat, fat accumulation in skeletal muscle can be divided into intramyocellular lipid (IMCL) and extramyocellular lipid (EMCL). IMCL is stored in lipid droplets within muscle cells, while EMCL is stored in interstitial adipose tissue. These ectopic lipid storage in skeletal muscle also prevalent in overweight and obese individuals and may lead to increased cardiovascular risk (Citation8). Recently, a cross-sectional study showed that IMCL and EMCL contents may be a risk factor for arterial stiffness in normal-weight individuals (Citation9). Furthermore, aerobic exercise training induced-changes in arterial stiffness was correlated with changes in IMCL or EMCL content in elderly individuals of normal weight (Citation10). However, the relationship with arterial stiffness and IMCL or EMCL content in overweight and obese individuals remain unclear. Furthermore, although a habitual aerobic exercise has been recommended to improve the lifestyle in these individuals, the effects of aerobic exercise training on arterial stiffness and IMCL or EMCL content have not been verified.
The purpose of this study was to determine the effects of aerobic exercise training on IMCL or EMCL content and arterial stiffness. First, in a cross-sectional study, we examined the relationship between arterial stiffness and IMCL or EMCL content in overweight and obese men. Secondly, we investigated the effects of aerobic exercise intervention on arterial stiffness and IMCL or EMCL content in overweight and obese men.
Materials and methods
Subjects
Subjects were recruited through the local newspaper advertisement, and the inclusion criteria were as follows: (a) men aged 30–64 years, and (b) body mass index (BMI) ≥ 25 kg/m2. For experiment 1, a total of 24 overweight and obese men (mean age, 49 ± 2 years; BMI, 27.8 ± 0.5 kg/m2) were recruited in a cross-sectional study. And subsequently, all subjects participated aerobic exercise training program (experiment 2). A total of 21 overweight and obese men (mean age, 49 ± 2 years; BMI, 27.7 ± 0.5 kg/m2) completed a 12-week aerobic exercise training program. 3 subjects withdrew from the study due to time constraints. None of the subjects had apparent cardiovascular or cerebrovascular disease, as determined by reviewing the medical history and physical examination results. This study included 2 current smokers, and 7 subjects were taking anti-hypertensive, anti-diabetic, or anti-hyperlipidemic medication. This study was approved by the Institutional Review Board of the University of Tsukuba and was registered in the UMIN (University Hospital Medical Information Network) clinical trials registry (UMIN000027711). All subjects provided their written informed consent before participation.
Study design
Before they were tested, subjects abstained from caffeine and fasted for at least 12 h. In experiments 1 and 2, the subjects had rested in the supine position for a minimum of 20 minutes in a quiet and temperature-controlled room (25°C).
In experiment 1, the body weight, height, visceral fat, VO2peak, resting arterial blood pressure, resting heart rate (HR), and blood biochemistry were measured in all subjects. Also, brachial-ankle pulse wave velocity (baPWV) and β-stiffness index were examined for evaluation of arterial stiffness. IMCL and EMCL contents were measured as ectopic fats of muscles. In experiment 2, the obese men completed the 12-weeks aerobic exercise training intervention. The subjects were tested 48 h after their last exercise training session to avoid the acute effects. Body weight, height, visceral fat, VO2peak, resting arterial blood pressure, resting HR, blood biochemistry, baPWV, β-stiffness index, and IMCL and EMCL contents were measured before and after the intervention. Dietary intake was assessed using a questionnaire before and after the intervention.
Exercise intervention
In experiment 2, all subjects performed aerobic exercise training program for 12 weeks (3 days per week). The exercise program included a 15-minute warm-up session followed by 40–60 minutes of walking and/or light jogging, and concluded with a 15-minute cool-down session (Citation11). The exercise intensity was gradually increased between 60% and 85% of the subject’s maximum heart rate during the intervention period. Moreover, the subjects were encouraged to keep performing aerobic exercise in their homes on other days. The subjects were instructed to maintain current eating behaviors during the intervention period.
Anthropometric measurements
Anthropometric measurements were taken when the participants were barefoot and wearing only light clothing. Body mass was measured once to the nearest 0.1 kg on a calibrated digital scale (InBody 770, InBody Japan, Tokyo, Japan), and height was measured once to the nearest 0.1 cm by using a wall-mounted stadiometer (Digital height meter AD-6227; A&D, Tokyo, Japan). BMI values were calculated by dividing the weight (kg) measurement by the square of the height (m). Total body fat was measured by using bioelectrical impedance (InBody 770). Waist circumference was directly measured on the skin at the level of the umbilicus in a standing position. Duplicate waist circumference measurements were recorded to the nearest 0.1 cm. Abdominal visceral fat area was measured by the dual-impedance analysis method (HDS-2000; Omron Healthcare, Kyoto, Japan). This method is useful for a simple visceral fat accumulation measurement, and there were good correlations between abdominal visceral fat area determined using HDS-2000 and CT (r = 0.888, p < 0.001) (Citation12).
Blood pressure and heart rate
Systolic blood pressure (SBP), diastolic blood pressure (DBP), and heart rate (HR) were measured using a non-invasive and automatic vascular profiling system (form PWV/ABI, Colin Medical Technology, Komaki, Japan) in the supine position.
Brachial-ankle pulse wave velocity (bapwv)
Brachial-ankle pulse wave velocity (baPWV) is a simple method for measuring the systemic arterial stiffness. The baPWV is automatically measured using separated cuffs for each of the 4 limbs by an oscillometric pressure-sensor method (form PWV/ABI) (Citation13). Bilateral brachial and post-tibial arterial pressure waveforms were stored for 10 s by each cuff connected to an oscillometric pressure sensor wrapped on arms and ankles (Citation12). The pulse wave velocity was calculated from the arterial length (estimated from the participant’s height) divided by the measured transit time. All subjects were examined 2 or 3 times, and the mean value of the right and left baPWV were obtained for the analysis.
β-stiffness index
The β-stiffness index is a parameter of central arterial stiffness to independence from blood pressure (Citation14). The arterial compliance was obtained through a combination of ultrasound imaging of the pulsatile carotid artery and simultaneous applanation of tonometrically obtained arterial pressure from the contralateral carotid artery (Citation15). The right common carotid artery diameter was measured from images obtained using an ultrasound device (En Visor; Koninklijke Philips Electronics, Eindhoven, the Netherlands) equipped with a high-resolution linear array transducer. A longitudinal image of the cephalic portion of the common carotid artery was acquired 1–2 cm proximal to the carotid bulb. All image analyses were performed by one investigator and were analyzed using image analysis software (Image J, NIH, Bethesda, Md, United States). The arterial lumen diameter at minimal and maximal was measured at 3 points each frame, and mean value was calculated. Brachial blood pressure was measured from form PWV/ABI.
The carotid β-stiffness index was calculated with the following equation: ln (Pmax/Pmin)/[Dmax-Dmin]/Dmin], where P and D corresponded to the pressure and diameter, respectively, and Max and Min referred to the maximum (systolic) and minimum (diastolic) values for diameters and pressures during the cardiac cycle (Citation16).
Measurement of IMCL and EMCL contents
The IMCL and EMCL contents were determined using non-invasive localized 1H-MR spectroscopy (1H-MRS) measurements in the right vastus lateralis. We used a 3T MR scanner Philips Achieva (Philips, Amsterdam, Nederland) with a six-channel Torso coil. All subjects laid in a supine position with the lower body extended fully. The 1H-MRS data was acquired using point-resolved spatially localized spectroscopy (PRESS) sequence with a repetition time of 3000 ms, echo time of 40 ms, bandwidth of 2000 Hz, 1024 sampling points, and 96 repetitions. In all cases, the volume of interest (VOI) was a single voxel of 15 mm×15 mm×30 mm. It was measured at the midpoint between the greater trochanter and the suprapatellar, and by avoiding visible interstitial tissue, fat, or vessels in the muscle cross-sectional area. The voxel was carefully placed at the same position for repeated measurements following the intervention period. Optimization of gradient shimming and water suppression were performed through automated procedures. The data were analyzed with the LCModel ver 6.3 software (LCMODEL Inc., Oakville, ON, Canada) (Citation17). In the analysis results, a standard deviation was less than 20%, which is considered as reliable of EMCL or IMCL content on the LCModel (Citation17,Citation18).
Measurement of VO2peak
The peak oxygen consumption (VO2peak) was determined during a graded exercise test using a cycling ergometer before and after exercise intervention. We used an online computer-assisted circuit spirometry (AE300S; Minato Medical Science, Osaka, Japan) for measuring VO2peak. After a 2-minute warm-up at 20 W, the workload was increased by 15 W each minute until the subject felt exhausted (Borg’s scale > 19) or reached 95% of the age-predicted maximal heart rate.
Survey of dietary intake
Daily dietary intake was recorded using the food frequency questionnaire (FFQg 5.0; Kenpaku-sha, Tokyo, Japan) based on food groups and established available for clinical investigation (Citation19). The FFQg is composed of item on 29 food groups and 10 cooking methods and assesses the average of daily intake frequency and total intake per week for each food or food groups in commonly used units or portion sizes (Citation20). The nutrient content was analyzed using a computer system with software (Excel-Eiyokun version 8.0; Kenpaku Co., Ltd., Tokyo, Japan).
Blood biochemistry
Blood samples were collected from each participant in the morning after a 12-hour overnight fast. Serum concentrations of triglycerides, total cholesterol, high-density lipoprotein cholesterol, and low-density lipoprotein cholesterol were determined using standard enzymatic techniques.
Data analysis
Values were presented as means ± SE. In experiment 1, the correlations between the IMCL or EMCL content with baPWV, β-stiffness index, or blood pressure (SBP and DBP) were determined using the Pearson’s partial correlation coefficient. The partial correlation coefficient was adjusted for age, blood pressure, medication, and smoking. In experiment 2, the paired student’s t-test was used to compare differences before and after the 12-week aerobic exercise training intervention. The relationships between changes in baPWV, β-stiffness index, and SBP with the IMCL or EMCL content after the intervention were determined using the Pearson’s correlation coefficient. Two-way (group × time) repeated analysis of variance was used to identify the effects of group, time, and the interaction between two groups (improvement and non-improvement of baPWV). The unpaired student’s t-test was used to compare the group differences between the characteristics at baseline. All statistical analyses were performed using SPSS 23.0 (IBM, Armonk, NY, United States), with statistical significance set at P < 0.05.
Results
Cross-sectional study (experiment 1)
The characteristics of all subject are presented in . In all subjects, baPWV was positively correlated with EMCL (r = 0.56, p < 0.01), whereas it was negatively correlated with IMCL (r = −0.46, p < 0.05), and IMCL/EMCL ratio (r = −0.41, p < 0.05) adjusted for age (). Furthermore, after controlling for age, blood pressure, smoking, and medication, there remained a significant association between arterial stiffness and EMCL (r = 0.56, p < 0.05) and IMCL (r = −0.51, p < 0.05). The β-stiffness index or SBP was also positively correlated with EMCL (r = 0.45, p < 0.05; r = 0.45, p < 0.05; respectively) adjusted for age. However, there was no significant correlation between the β-stiffness index or SBP and IMCL (r = −0.24, p = 0.28; r = − 0.40, p = 0.06; respectively).
Table 1. Characteristics of all subjects.
Table 2. Correlations between IMCL or EMCL and baPWV, β-stiffness index, SBP and DBP.
Intervention study (experiment 2)
After the exercise intervention, weight, BMI, total body fat, abdominal visceral fat area, heart rate, and triglyceride were significantly decreased, and VO2peak was significantly elevated after the intervention. There were no significant differences in SBP, DBP, total cholesterol, HDL cholesterol, LDL cholesterol, and energy intake before and after the intervention. Moreover, there were no significant changes in baPWV, β-stiffness index, and IMCL and EMCL contents after the exercise intervention ().
Table 3. Change in values after exercise intervention.
The changes in VO2peak by aerobic exercise training were not associated with the changes in IMCL content (r = −0.236, p = 0.30) or EMCL content (r = −0.283, p = 0.21). However, the changes in baPWV were positively correlated with the changes in EMCL content, whether the comparison was unadjusted (r = 0.71, p < 0.001) or adjusted for changes in SBP (r = 0.60, p < 0.01). In contrast, there was no significant correlation between changes in baPWV and changes in IMCL content (r = −0.12, p = 0.39) (). Furthermore, the changes in the β-stiffness index were positively correlated with the changes in EMCL content (r = 0.48, p < 0.05), whereas it was negatively correlated with the changes in IMCL content (r = −0.44, p < 0.05). The changes in EMCL content were positively correlated with changes in SBP (r = 0.49, p < 0.05), whereas it was negatively correlated with the changes in IMCL content (r = −0.47, p < 0.05).
Figure 1. Association between the changes in IMCL or EMCL content and baPWV (A, D), β-stiffness index (B, E), and SBP (C, F) following a 12 weeks of aerobic exercise intervention.
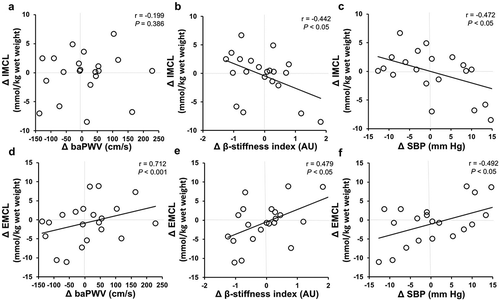
Additionally, we divided two groups (improvement and non-improvement of baPWV) and compared the effect on arterial stiffness and IMCL or EMCL content after exercise intervention (). Interestingly, the EMCL content after exercise intervention for the group of improvements in baPWV were significantly decreased compared with the group of non-improvements in baPWV (p < 0.05).
Table 4. Groups of baPWV.
Discussion
This cross-sectional study showed that IMCL content negatively correlated with arterial stiffness, while EMCL content positively correlated with arterial stiffness in overweight and obese men. Furthermore, we found that aerobic exercise training induced-changes in arterial stiffness were correlated with changes in IMCL or EMCL content. Additionally, the group of improvements in baPWV was correlated only significantly with reduced EMCL content. Therefore, we proposed that IMCL and EMCL contents were associated with arterial stiffness, and aerobic exercise training-induced changes in EMCL content may have affected the changes in arterial stiffness in overweight and obese men.
In this study, the EMCL content in overweight and obese men was positively correlated with arterial stiffness (i.e., baPWV and β-stiffness index). These results indicated that more EMCL content could be a bad influence on arterial stiffness in the obese population and was consistent with a previous study demonstrating that EMCL content was associated with arterial stiffness in normal-weight subjects (Citation9). EMCL is stored in adipose tissue such as subcutaneous fat or abdominal visceral fat. Adipose tissue is a metabolically active organ that controls plasma-free fatty acid levels and contributes to systemic metabolic homeostasis by secreting adipokines (Citation21). However, the adipose tissue enlarges with obesity, and they release a large number of inflammatory cytokines, such as leptin, interleukin-6 (IL-6), and tumor necrosis factor-α (TNF-α) while decreasing the secretion of adiponectin. These processes influence not only body weight homeostasis, insulin resistance, diabetes, fibrinolysis, and inflammation (Citation22), but could also contribute to an increase in arterial stiffness, endothelial dysfunction, and atherosclerosis (Citation23). Therefore, it is possible that an accumulation of EMCL content elevates the risk of increased arterial stiffness.
On the other hand, the IMCL content in overweight and obese men was negatively correlated with arterial stiffness (i.e., baPWV), and our results were consistent with a recent study in normal-weight adults, especially in older adults with low cardiorespiratory fitness levels (Citation10). The IMCL content was increased in obese individuals and individuals type 2 diabetes (T2D), and the accumulations of IMCL was strongly correlated with insulin resistance (Citation24,Citation25). However, the IMCL content was also correlated with cardiorespiratory fitness levels (Citation26), and exercise training increased the IMCL content (Citation10,Citation27–Citation29). Moreover, endurance athletes have increased IMCL content, although they have high insulin sensitivity (Citation30), a phenomenon called “the athlete’s paradox”. Recently, the IMCL content in overweight individuals without T2D has been shown to be lower than that in overweight individuals with T2D or endurance athletes, and is at similar levels with sedentary normal-weight individuals (Citation31). In the present study, the overweight and obese individuals had normal levels of insulin resistance or insulin sensitivity, and their IMCL content was lower than that in overweight or obese individuals in a previous study (Citation32). Therefore, the obese individuals with normal insulin sensitivity, particularly those with lower IMCL content, as well as normal-weight adults with lower IMCL content may have a higher risk of arterial stiffness.
In this study, the subjects with decreased baPWV after exercise intervention had a significant reduction in EMCL content. Additionally, our intervention study showed that aerobic exercise training-induced changes in arterial stiffness was correlated with changes in EMCL content. In the present study, a significant negative correlation was also found between the amount of changes in the IMCL and EMCL contents, suggesting that aerobic exercise training may redistribute these lipids in skeletal muscles. During aerobic exercise, adipose tissue lipolysis was also increased (Citation33), and thus the total amount of adipose tissue was decreased, resulting in continuous aerobic exercise training. Such a reduction in the EMCL content of skeletal muscle adipose tissue in response to the effects of aerobic exercise training may be beneficial to the arterial stiffness. In our study, the subcutaneous and visceral fats were significantly decreased after exercise intervention, but no relationship was observed with changes in these fats and changes in arterial stiffness or blood pressure. Therefore, EMCL in adipose tissue in skeletal muscle may be more important effects on arterial stiffness than subcutaneous or visceral fat in overweight and obese population, suggesting that the EMCL content may be a risk factor for arterial stiffness in overweight and obese individuals. However, further studies are needed to explicate the mechanism underlying the effects of EMCL on arterial stiffness in overweight and obese individuals.
The present study has certain limitations. First, there were no statistically significant changes in the parameters of arterial stiffness after exercise intervention. One meta-analysis review reported that the effects on improvements of arterial stiffness following aerobic exercise training were more representative of the longer frequency period and the higher exercise intensity, or the higher level of the subject’s arterial stiffness (Citation34). Furthermore, the effects of exercise training have individual variations (Citation35). Thus, these multiple factors would have affected the results of this intervention study. Secondly, the number of subjects was small, and we have no comparable control groups. It is not clear if other factors (e.g., diets, amount of physical activities and so on) may have influenced results. Future randomized-control trials need to establish the effectiveness of aerobic exercise training.
In conclusion, aerobic exercise training did not alter both arterial stiffness and IMCL or EMCL content. However, we confirmed that relationship between arterial stiffness and IMCL or EMCL content through both cross-sectional and intervention studies. These results suggest that IMCL or EMCL contents may affect arterial stiffness in overweight and obese men. Further research is warranted to better understand these relationships and the mechanisms responsible for the observed changes in overweight and obese individuals.
Conflict of interest statement
Yoshiyuki Sankai is a professor of University of Tsukuba, a founder, a shareholder, and the chief executive officer of University venture company ‘‘CYBERDYNE Inc.”, Ibaraki, Japan. He is managed according to the University rules and guidelines. CYBERDYNE is the manufacturer of the HAL. The present study was proposed by the authors. CYBERDYNE was not directly involved in the study design; collection, analysis, or interpretation of data; writing the report; or the decision to submit the paper for publication.
References
- World Health Organization. (2018). Obesity and overweight. Retrieved from http://www.who.int/news-room/fact-sheets/detail/obesity-and-overweight (accessed June 1, 2018)
- Jia G, Aroor AR, DeMarco VG, Martinez-Lemus LA, Meininger GA, Sowers JR. Vascular stiffness in insulin resistance and obesity. Front Physiol. 2015;6:1–8. doi:10.3389/fphys.2015.00098.
- Zebekakis PE, Nawrot T, Thijs L, Balkestein EJ, van der Heijden-Spek J, Van Bortel LM, Struijker-Boudier HA, Safar ME, Staessen JA. Obesity is associated with increased arterial stiffness from adolescence until old age. J Hypertens. 2005;23:1839–46. doi:10.1097/01.hjh.0000179511.93889.e9.
- Safar ME, Obesity A. Stiffness, and Cardiovascular Risk. J Am Soc Nephrol. 2006;17:S109–S111. doi:10.1681/ASN.2005121321.
- Hannah WN, Harrison SA. Lifestyle and dietary interventions in the management of nonalcoholic fatty liver disease. Dig Dis Sci. 2016;61:1365–74. doi:10.1007/s10620-016-4153-y.
- Fabbrini E, Magkos F, Mohammed BS, Pietka T, Abumrad NA, Patterson BW, Okunade A, Klein S. Intrahepatic fat, not visceral fat, is linked with metabolic complications of obesity. Proc Natl Acad Sci. 2009;106:15430–35. doi:10.1073/pnas.0904944106.
- Kim BJ, Kim BS, Kang JH. Echocardiographic epicardial fat thickness is associated with arterial stiffness. Int J Cardiol. 2013;167:2234–38. doi:10.1016/j.ijcard.2012.06.013.
- Zacharewicz E, Hesselink MKC, Schrauwen P. Exercise counteracts lipotoxicity by improving lipid turnover and lipid droplet quality. J Intern Med. 2018;284(5):505–18. doi:10.1111/joim.2018.284.issue-5.
- Hasegawa N, Kurihara T, Sato K, Homma T, Fujie S, Fujita S, Sanada K, Hamaoka T, Iemitsu M. Intramyocellular and extramyocellular lipids are associated with arterial stiffness. Am J Hypertens. 2015;28:1473–79. doi:10.1093/ajh/hpv041.
- Hasegawa N, Fujie S, Kurihara T, Homma T, Sanada K, Sato K, Hamaoka T, Iemitsu M. Effects of habitual aerobic exercise on the relationship between intramyocellular or extramyocellular lipid content and arterial stiffness. J Hum Hypertens. 2016;30:606–12. doi:10.1038/jhh.2016.28.
- Kumagai H, Yoshikawa T, Zempo-miyaki A, Myoenzono K, Tsujimoto T, Tanaka K, Maeda S. Vigorous physical activity is associated with regular aerobic exercise-induced increased serum testosterone levels in overweight/obese men. Horm Metab Res. 2018;500:73–79.
- Shiga T, Hamaguchi T, Oshima Y, Kanai H, Hirata M, Hosoda K, Nako K. A new simple measurement system of visceral fat accumulation by bioelectrical impedance analysis. World Congress on Medical Physics and Biomedical Engineering, September 7-12, 2009, Munich, Germany. IFMBE Proceedings, 25/7, 2009; 338–41.
- Sugawara J, Hayashi K, Yokoi T, Cortez-Cooper MY, DeVan AE, Anton MA, Tanaka H. Brachial–ankle pulse wave velocity: an index of central arterial stiffness? J Hum Hypertens. 2005;19:401–06. doi:10.1038/sj.jhh.1001838.
- Laurent S, Cockcroft J, Van BL, Boutouyrie P, Giannattasio C, Hayoz D, Pannier B, Vlachopoulos C, Wilkinson I, Struijker-Boudier H, et al. Expert consensus document on arterial stiffness: methodological issues and clinical applications. Eur Heart J. 2006;27(21):2588–605. doi:10.1093/eurheartj/ehl254.
- Tanaka H, Dinenno FA, Monahan KD, Clevenger CM, DeSouza CA, Seals DR. Aging, habitual exercise, and dynamic arterial compliance. Circulation. 2000;102:1270–75. doi:10.1161/01.CIR.102.11.1270.
- Miyachi M, Kawano H, Sugawara J, Takahashi K, Hayashi K, Yamazaki K, Tabata I, Tanaka H. Unfavorable effects of resistance training on central arterial compliance: A randomized intervention study. Circulation. 2004;110(18):2853–63. doi:10.1161/01.CIR.0000146380.08401.99.
- Isobe T, Okamoto Y, Hirano Y, Ando H, Takada K, Sato E, Shinoda K, Kamizawa S, Mori Y, Suzuki H, et al. Effect of biological factors on successful measurements with skeletal-muscle 1H-MRS</sup>. Ther Clin Risk Manag. 2016;12:1133–37. doi:10.2147/TCRM.
- Provencher SW LCModel and LCMgui user’s manual 2019. http://s-provencher.com/pub/LCModel/manual/manual.pdf (accessed 5 June, 2019)..
- Sone H, Yoshimura Y, Tanaka S, Iimuro S, Ohashi Y, Ito H, Seino H, Ishibashi S, Akanuma Y, Yamada N. Japan Diabetes Complications Study (JDCS) Group: Cross-sectional association between BMI, glycemic control and energy intake in Japanese patients with type 2 diabetes. Diabetes Res Clin Pract. 2007;1:s23-29. doi:10.1016/j.diabres.2007.01.029.
- Takahashi K, Yoshimura Y, Kaimoto T, Kunji D, Komatsu T, Yamamoto S. Validation of a food frequency questionnaire based on food groups for estimating individual nutrient intake. Jpn J Nutr 2001; 59: 221-232. doi:10.5264/ejyogakuzashi.59.221.
- Gutierrez DA, Puglisi MJ, Hasty AH. Impact of increased adipose tissue mass on inflammation, insulin resistance, and dyslipidemia. Curr Diab Rep. 2009;9.1:26–32. doi:10.1007/s11892-009-0006-9.
- Van Gaal LF, Mertens IL, De Block CE. Mechanisms linking obesity with cardiovascular disease. Nature. 2006;444:875. doi:10.1038/nature05376.
- JS Y, CD S, JJ E, SW C. C-reactive protein in healthy subjects: associations with obesity, insulin resistance, and endothelial dysfunction: a potential role for cytokines originating from adipose tissue? Arterioscler Thromb Vasc Biol. 1999;19:972–78. doi:10.1161/01.ATV.19.4.972.
- Virkamaki A, Korsheninnikova E, Seppala-Lindroos A, Vehkavaara S, Goto T, Halavaara J, Hakkinen A-M, Yki-Jarvinen H. Intramyocellular lipid is associated with resistance to in vivo insulin actions on glucose uptake, antilipolysis, and early insulin signaling pathways in human skeletal muscle. Diabetes. 2001;50:2337–43. doi:10.2337/diabetes.50.10.2337.
- Gemmink A, BH G, Schrauwen P, MKC H. BBA - Molecular and Cell Biology of Lipids Intramyocellular lipid droplets and insulin sensitivity, the human. BBA - Mol Cell Biol Lipids. 2017;1862:1242–49. doi:10.1016/j.bbalip.2017.07.010.
- McCormack SE, McCarthy MA, Harrington SG, Farilla L, Hrovat MI, Systrom DM, Thomas BJ, Torriani M, McInnis K, Grinspoon SK, et al. Effects of exercise and lifestyle modification on fitness, insulin resistance, skeletal muscle oxidative phosphorylation and intramyocellular lipid content in obese children and adolescents. Pediatr Obes. 2014;9:281–91. doi:10.1111/ijpo.2014.9.issue-4.
- Phillips SM, Green HJ, Tarnopolsky MA, Heigenhauser GJ, Grant SM. Progressive effect of endurance training on metabolic adaptations in working skeletal muscle. Am J Physiol. 1996;270:E265–E272.
- Schrauwen-Hinderling VB, Schrauwen P, Hesselink MK, vanEngelshoven JM, Nicolay K, Saris WH, Kessels AGH, Kooi ME. The increase in intramyocellular lipid content is a very early response to training. J Clin Endocrinol Metab. 2003;88:1610–16. doi:10.1210/jc.2002-021464.
- Shepherd SO, Cocks M, Tipton KD, Ranasinghe AM, Barker TA, Burniston JG, Wagenmakers AJ, Shaw CS. Sprint interval and traditional endurance training increase net intramuscular triglyceride breakdown and expression of perilipin 2 and 5. J Physiol. 2013;591:657–75. doi:10.1113/jphysiol.2012.247577.
- Goodpaster BH, He J, Watkins S, Kelley DE. Skeletal muscle lipid content and insulin resistance: evidence for a paradox in endurance- trained athletes. J Clin Endocriol Metab. 2001;86(12):5755–61. doi:10.1210/jcem.86.12.8075.
- Gemmink A, Daemen S, Brouwers B, Huntjens PR, Schaart G, Moonen-Kornips E, Jörgensen J, Hoeks J, Schrauwen P, Hesselink MKC. Dissociation of intramyocellular lipid storage and insulin resistance in trained athletes and type 2 diabetes patients; involvement of perilipin 5? J Physiol. 2018;596:857–68.
- Shida T, Akiyama K, Oh S, Sawai A, Isobe T, Okamoto Y, Ishige K, Mizokami Y, Yamagata K, Onizawa K, et al. Skeletal muscle mass to visceral fat area ratio is an important determinant affecting hepatic conditions of non-alcoholic fatty liver disease. J Gastroenterol. 2018;53:535–47.
- Stich V, de Glisezinski I, Berlan M, Bulow J, Galitzky J, Harant I, Suljkovicova H, Lafontan M, Rivière D, Crampes F. Adipose tissue lipolysis is increased during a repeated bout of aerobic exercise. J Appl Physiol. 2000;88(4):1277–83. doi:10.1152/jappl.2000.88.4.1277.
- Ashor AW, Lara J, Siervo M, Celis-morales C, Mathers JC. Effects of exercise modalities on arterial stiffness and wave reflection : a systematic review and meta-analysis of randomized controlled trials. PLoS One. 2014;9(10):1–15. doi:10.1371/journal.pone.0110034.
- Hecksteden XA, Kraushaar J, Scharhag-rosenberger F, Theisen D, Senn S, Meyer T. Individual response to exercise training - a statistical perspective. J Appl Physiol. 2015;118:1450–59. doi:10.1152/japplphysiol.00714.2014.