ABSTRACT
Background
Endothelial dysfunction of the pulmonary artery contributes to hypoxia-induced pulmonary arterial hypertension (PAH). Omentin-1, as a novel adipocytokine, plays an important protective role against cardiovascular diseases. However, the effect and underlying mechanisms of omentin-1 against PAH remain unclear.
Methods
PAH was induced in SD (Sprague & Dawley) rats via a low-oxygen chamber for 4 weeks. Hemodynamic evaluation was undertaken using a PowerLab data acquisition system, and histopathological analysis was stained with hematoxylin and eosin (H&E). Endothelial function of pulmonary artery was assessed using wire myography.
Results
We found that omentin-1 significantly improved pulmonary endothelial function in rats exposed to hypoxia and attenuated PAH. Mechanistically, we found that omentin-1 increased phosphorylated 5’‑adenosine monophosphate‑activated protein kinase (p‑AMPK) level and reduced endoplasmic reticulum (ER) stress and increased NO production in pulmonary artery from rats exposed to hypoxia. However, the effect of omentin-1 was abolished by treatment with AMPK inhibitor (Compound C).
Conclusions
Our results reveal a protective effect of omentin-1 in PAH via inhibiting ER stress through AMPKα signaling and provide an agent with translational potential for the treatment of PAH.
Pulmonary arterial hypertension (PAH) is a serious pulmonary vascular disease caused by increased pulmonary vascular resistance, accompanied by persistent elevated pulmonary pressure, ultimately leading to respiratory and right-heart failure in patientsCitation1. PAH has a poor prognosis and limited treatment options, resulting in a high mortality rateCitation2. How to effectively improve the quality of life and prognosis of patients with PAH is the focus of current experimental and clinical research.
Hypoxia-induced PAH is the most common type of PAHCitation3. The mechanisms involved including abnormal contraction and relaxation of pulmonary artery, abnormal proliferation of pulmonary vascular smooth muscle cells, and activation of inflammation and oxidative stress, etcCitation4,Citation5. Vascular endothelial cells, smooth muscle cells, fibroblasts, and macrophages participate in the above processesCitation6. Studies have shown that impaired pulmonary vascular endothelial relaxation function is an early hallmark of PAH and improving pulmonary vascular endothelial relaxation function is an important strategy for the treatment of PAHCitation7. At present, clinically, there are drugs aimed at improving pulmonary vascular endothelial relaxation function, such as sildenafil, which can promote pulmonary vascular vasodilation by increasing the synthesis and release of NO, and effectively improve the clinical symptoms and quality of life of patients with PAHCitation8. However, such drugs also have some side effects. Therefore, the development of safe and effective drugs for the treatment of PAH, aiming at improving the pulmonary vascular endothelial relaxation function, is a major problem in the field of drug research and development.
Omentin-1 is a novel adipocytokine produced by visceral fat tissue, which plays an important protective role against cardiovascular diseases via inhibiting the proliferation of vascular smooth muscle cells, improving vascular endothelial relaxation, and inhibiting cardiomyocyte apoptosisCitation9. However, whether omentin-1 can protect PAH has not been reported. This study revealed that omentin-1 could inhibit ER stress by activating AMPK, improve pulmonary vasodilation function, and play a role in ameliorating PAH.
Materials and methods
Animal protocols
All animal experiments were approved by the Animal Use and Care Committee of Chongqing Medical University (No.2023104) and conformed to the guidelines of the National Institutes of Health (NIH) Guide for the Care and Use of Laboratory Animals. Male SD (Sprague & Dawley) rats (200–220 g, 10 weeks old) were provided by the Experimental Animal Center of Chongqing Medical University. Animals had free access to standard rodent chow and drinking water and were kept in a constant temperature at 21°C, 55 ± 10% relative humidity on a 12-h light cycle. The rats were randomly divided into the normoxia group, normoxia+omentin-1 group, hypoxia group, and hypoxia+omentin-1 group. Rats in the hypoxia group were exposed to hypoxia (10% O2 with balanced N2) in a low-oxygen chamber for 4 weeks to induce chronic PAH. Rats in the normoxia group were exposed to normal air for 4 weeks. During this period, the rats were subcutaneously injected with saline (0.14 mL/day) or omentin-1 (18 μg/kg/day, ProSpec-Tany TechnoGene Ltd., Rehovot, Israel) for 4 weeks. Before the rats were sacrificed, the concentration of omentin-1 in the rats’ plasma was measured by ELISA kit (Nanjing Jiancheng Bioengineering Institute, Nanjing, China).
Hemodynamic measurements
At the end of the treatment period, the rats were anesthetized with 2.5% pentobarbital sodium. An incision was made to expose the right external jugular vein, and a micromanometer-tipped pressure catheter was advanced into the right ventricle. Right ventricular systolic pressure (RVSP) was measured using a PowerLab data acquisition system (AD Instruments) and automatically calculated using the LabChart software.
Right ventricular mass measurements
To determine right ventricular hypertrophy, the right ventricle (RV), left ventricle (LV), and septum (S) were dissected, and each portion was weighed. The right ventricular hypertrophy index (RVHI) was calculated using the following equation: RVHI=RV/(LV+S).
Histopathological analysis
A histopathological examination was performed to visualize morphologic changes in pulmonary arteries. The lung tissue samples were immersed in 4% paraformaldehyde for 24 hours. The paraffin-embedded sections were stained with hematoxylin and eosin (H&E) (Beyotime, Shanghai, China) and analyzed under a light microscope.
Functional assay by wire myograph
After rats were sacrificed, pulmonary arteries were dissected, and arterial rings (~2 mm in length) were mounted on a myograph (Danish Myo Technology, Aarhus, Denmark) for isometric tension recording. The rings were equilibrated for 60 min and phenylephrine (PE; 3 µmol/L, Sigma-Aldrich) was added to the bath. Once a stable contraction had been reached, a concentration-response curve for acetycholine (Ach, 10−8.5-10−5M; Sigma-Aldrich) and sodium nitroprusside (SNP, 10−9-10−5M; Sigma-Aldrich) was constructed. To determine the role of NO in the EDR studies, the aortic rings were incubated with L-NAME (100 μM) for 30 min, before measuring the effects on Ach-induced relaxation.
Ex vivo studies of arterial rings
Arterial rings were incubated in Dulbecco’s Modified Eagle’s Media (DMEM, Gibco, MD, USA) with 10% fetal bovine serum (Gibco). Omentin-1 (0.6 μg/mL, ProSpec-Tany TechnoGene Ltd), Compound C (AMPK antagonist, 5 μmol/L; Sigma-Aldrich) was added into the culture medium with bathed the pulmonary artery rings in an incubator at 37°C for 16 h. After the incubation, the rings were transferred into fresh Krebs solution for functional studies and Western blot.
NADPH oxidase activity assay
Superoxide anions generated by NADPH oxidase were measured in lysates of arterial rings by the lucigenin-enhanced chemiluminescence method. Briefly, NADPH (100 μM, Sigma-Aldrich) and lucigenin (5 μM, Sigma-Aldrich) were added into 1 ml microcentrifuge tubes. Superoxide production was measured every 20 sec for 10 min until enzymatic activity reached a plateau. Using this method, the superoxide anion production also represents NADPH oxidase activity.
Measurement of NO metabolites
The pulmonary artery rings were stimulated with Ach (10−6 mol/L) for 5 min, and incubation solution was assayed for the products of NO using nitrate reductase method (Nanjing Jiancheng Bioengineering Institute).
Western blot analysis
Arterial rings were lysed in PIPA lysis buffer (Beyotime, Shanghai, China) and protein samples were separated by 10% SDS-PAGE and transferred to polyvinylidene fluoride (PVDF) membranes. Membranes were blocked and incubated with specific primary antibodies against p-AMPKα at Thr172, p-PERK, ATF6, p-eNOS at Ser1177, t-AMPKα, t-PERK, t-eNOS (1:300; Cell Signaling Technology, Danvers, MA, USA) and GAPDH (1:300, Proteintech Group, WA) overnight at 4°C. Immunoreactive bands were detected by incubating with secondary antibodies for 1 h at room temperature. The bands were visualized using the Odyssey Infrared Imaging System and the intensities were quantified by densitometry using Quantity-One software (Bio-Rad, Hercules, CA).
Statistical analysis
Results are expressed as means ± standard deviation (SD). The relaxation was presented as a percentage reduction of the PE-induced contraction. Comparisons among groups were made using ANOVA followed by Student’s Newman–Keuls test. The results were considered statistically significant with p values < 0.05.
Results
Omentin-1 attenuated hypoxia-induced PAH
To determine whether omentin-1 could attenuate PAH, the rats exposed to hypoxia were subcutaneously injected with omentin-1 for 4 weeks. First, we measured omentin-1 levels in plasma and found that omentin-1 was significantly reduced in rats exposed to hypoxia for 4 weeks (). Next, we observed that compared with the normoxia group, RVSP, and RVHI were significantly increased in rats exposed to hypoxia for 4 weeks, which were markedly reversed by the omentin-1 treatment (). We also observed that hypoxia induced pulmonary vascular remodeling, as indicated by increased pulmonary vascular remodeling. Similarly, omentin-1 ameliorated pulmonary vascular remodeling in rats exposed to hypoxia ().
Figure 1. Omentin-1 attenuated hypoxia-induced PAH. SD rats were exposed to a low-oxygen chamber (10% O2 with balanced N2) or normal air with saline or omentin-1 (18 μg/kg/day) treatment for 4 weeks. (a) omentin-1 levels were measured by ELISA kit. (b and c) right ventricular systolic pressure (RVSP) and right ventricular hypertrophy index (RVHI) in control (vehicle) and omentin-1 treated rats exposed to normoxia or hypoxia. (D1 and D2) Representative images of arteries and quantification of ratio of vascular medial thickness to total vessel size were shown. Data are expressed as the means ± SD (n=5/group). *p <.05 vs others. N+V, normoxia+Vehicle; N+O, normoxia+Omentin-1; H+V, hypoxia+Vehicle; H+O, hypoxia+ omentin-1.
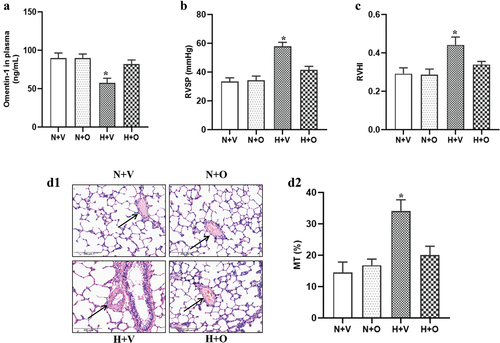
Omentin-1 improved endothelial function of pulmonary artery in rats exposed to hypoxia
Endothelial dysfunction is closely related to the occurrence and development of PAHCitation10. To determine the protective effect of omentin-1 on endothelial function in rats exposed to hypoxia, pulmonary-artery rings were treated with Ach or SNP. To assess the EDR of pulmonary-artery rings, after precontraction by PE stimulation, the artery rings were treated with Ach. We found that there was an impaired Ach-induced relaxation in rats exposed to hypoxia compared with the normoxia group, which was significantly reversed by omentin-1 treatment (). Pretreatment with L-NAME inhibited the Ach-induced relaxation in rats exposed to hypoxia and normoxia groups with or without omentin-1 treatment, indicating that the Ach-induced relaxation was no longer different among them (). Moreover, SNP induced-endothelium-independent relaxations were measured and there were no differences in all groups, indicating that the response of vascular smooth muscle to NO was not affected ().
Figure 2. Omentin-1 improved endothelial function of pulmonary artery in rats exposed to hypoxia. SD rats were exposed to a low-oxygen chamber (10% O2 with balanced N2) or normal air with saline or omentin-1 (18 μg/kg/day) treatment for 4 weeks. (a-c) ach-induced relaxation, ach-induced relaxation with L-NAME and SNP-induced relaxation in pulmonary artery from the rats exposed to hypoxia or normal air. Data are expressed as the means ± SD (n=5/group). *p <.05 vs others. N+V, normoxia+Vehicle; N+O, normoxia+Omentin-1; H+V, hypoxia+Vehicle; H+O, hypoxia+ omentin-1.
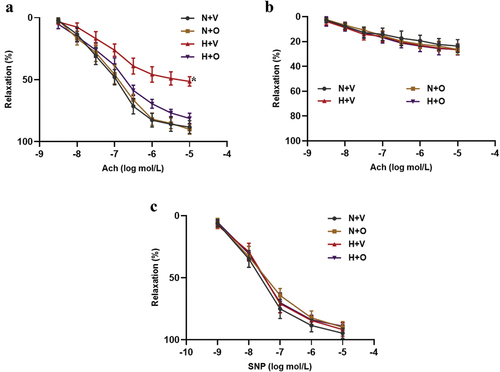
Omentin-1 increased NO production in pulmonary-artery rings from rats exposed to hypoxia
Endothelial dysfunction is mainly attributed to decreased endothelial NO production. We evaluated NO production and the activity of eNOS and found that compared to the normoxia group, NO production, and phosphorylation of eNOS at Ser1177 were decreased in rats exposed to hypoxia, which were reversed by omentin-1 treatment ().
Figure 3. Omentin-1 increased NO production in pulmonary-artery rings from rats exposed to hypoxia. SD rats were exposed to a low-oxygen chamber (10% O2 with balanced N2) or normal air with saline or omentin-1 (18 μg/kg/day) treatment for 4 weeks. (a and b) NO metabolites in endothelium-intact pulmonary arteries and phosphorylation and expression of eNOS at Ser1177 in pulmonary artery from the rats exposed to hypoxia or normal air. Data are expressed as the means ± SD (n=5/group). *p <.05 vs others. N+V, normoxia+Vehicle; N+O, normoxia+Omentin-1; H+V, hypoxia+Vehicle; H+O, hypoxia+ omentin-1.
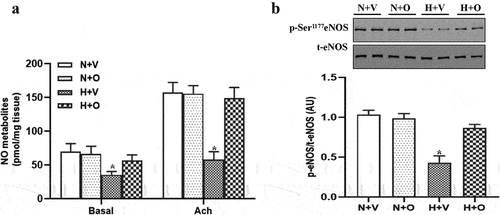
Omentin-1 attenuated ER stress in pulmonary-artery rings from rats exposed to hypoxia
Previous studies have shown that ER stress can lead to vascular endothelial dysfunction, which is an important pathogenesis of atherosclerosis and PAHCitation11,Citation12. Consistent with the previous studyCitation13, we found that compared with the normoxia group, ER stress was increased in pulmonary-artery rings from rats exposed to hypoxia. Omentin-1 treatment reversed increased ER stress in pulmonary-artery rings from rats exposed to hypoxia (). Moreover, treatment with omentin-1 normalized the increased oxidative stress in pulmonary-artery rings from rats exposed to hypoxia ().
Figure 4. Omentin-1 attenuated ER stress in pulmonary-artery rings from rats exposed to hypoxia. SD rats were exposed to a low-oxygen chamber (10% O2 with balanced N2) or normal air with saline or omentin-1 (18 μg/kg/day) treatment for 4 weeks. (a-c) the expressions of ER stress markers, such as p-PERK and ATF-6, and NADPH oxidase activity in lysates of pulmonary artery from the rats exposed to hypoxia or normal air. Data are expressed as the means ± SD (n=5/group). *p <.05 vs others. N+V, normoxia+Vehicle; N+O, normoxia+Omentin-1; H+V, hypoxia+Vehicle; H+O, hypoxia+ omentin-1.
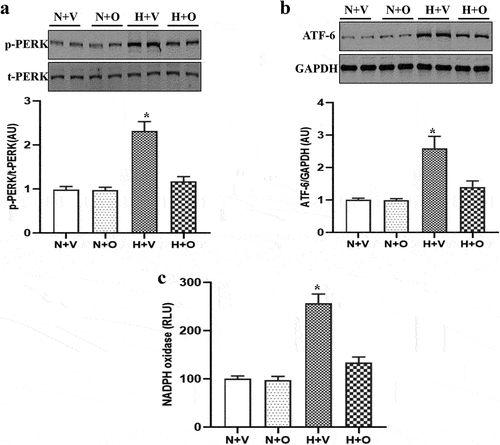
Omentin-1 alleviated ER stress via AMPKα signaling in rats exposed to hypoxia
Previous studies have shown that ER stress is regulated by AMPKα-dependent signaling pathwayCitation14. We first explored this pathway and found that the level of p-AMPKα at Thr172 was decreased in pulmonary-artery rings from rats exposed to hypoxia, compared with the normoxia group, which were reversed by omentin-1 treatment (). Next, we verified the effect of omentin-1 on the AMPKα signaling pathway in vitro. As expected, omentin-1 treatment ameliorated ER stress, increased phosphorylation of eNOS at Ser1177 and improved endothelial function in pulmonary-artery rings from rats exposed to hypoxia, which were inhibited by AMPK inhibitor (Compound C) ().
Figure 5. Omentin-1 alleviated ER stress dependent on AMPKα signaling in rats exposed to hypoxia. SD rats were exposed to a low-oxygen chamber (10% O2 with balanced N2) or normal air for 4 weeks. (a-d) phosphorylation and expression of AMPKα, expression of ATF-6, phosphorylation and expression of eNOS at Ser1177 and ach-induced relaxation in pulmonary artery from the rats incubated with omentin-1 alone or in the presence of compound C, AMPK inhibitor (5 μmol/L) for 16 h. Data are expressed as the means ± SD (n=5/group). *p <.05 vs N+V; #p <.05 vs H+O. N+V, normoxia+Vehicle; H+V, hypoxia+Vehicle; H+O, hypoxia+ omentin-1; H+O+C, hypoxia+ omentin-1+ compound C.
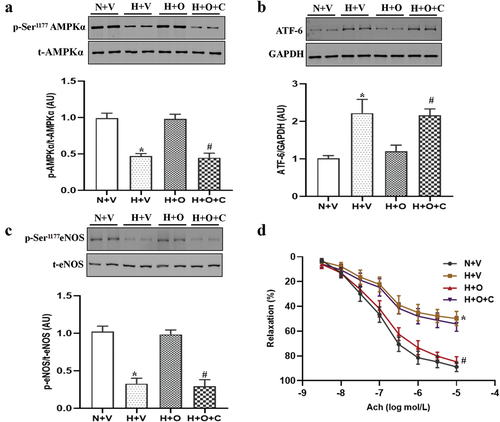
Discussion
Although there has been some progress in the prevention and treatment of PAH in recent years, treatment strategies are still limited and the mortality rate remains unsatisfactory. In the current study, we found that endothelial function was impaired in pulmonary artery of rats exposed to hypoxia and that omentin-1 treatment evidently reversed the hypoxia-induced endothelial dysfunction. We further determined that the mechanism by which omentin-1 improved endothelial function of pulmonary artery of rats exposed to hypoxia and found that inhibition of ER stress through activation of AMPK was an upstream regulatory signal for improving endothelial function. Thus, omention-1 may be considered as a novel strategy for the treatment of PAH.
The pathogenesis of PAH is complex and involves many factors, including endothelial dysfunction, abnormal proliferation of vascular smooth muscle cells and inflammatory cell infiltrationCitation15. Among them, endothelial dysfunction is the earliest and persistent phenotype of PAH, and it is also an important cause of abnormal proliferation of vascular smooth muscle cells in the later periodCitation16. At present, there are relevant clinical drugs for improving pulmonary artery endothelial dysfunction, but some drugs have certain side effects and fail to improve the prognosis of patients. Therefore, it is urgent to develop safer and more effective drugs for PAH targeting the improvement of endothelial function.
Previous studies have shown that omentin-1 has multiple beneficial effects against the disorders of the cardiovascular system or metabolismCitation17,Citation18. Serum omentin-1 levels are decreased in patients with hypertensionCitation19 and atherosclerosis, particularly in peripheral artery diseaseCitation20. Moreover, lower omentin-1 levels are related to obesity and insulin resistance, which are worse vascular outcomes in diabetes patientsCitation21,Citation22. Studies in animals and cells have shown that omentin-1 enhances the inhibitory effect of endothelial progenitor cells and suppresses the proliferation of vascular smooth muscle cells to attenuate neointimal hyperplasia by inhibiting the p38 MAPK/CREB pathway and AMPK-dependent mechanisms, respectivelyCitation23,Citation24. More importantly, omentin-1 protects against high glucose-induced endothelial dysfunction via the AMPK/PPARδ signaling pathwayCitation25. Whether omentin-1 could protect PAH and related protective mechanism has not been reported. In our present study, we found that hypoxia induced endothelial dysfunction and vascular remodeling of pulmonary artery, which could be significantly reversed by omentin-1 treatment, suggesting that omentin-1 may be a potential agent for the treatment of PAH.
Previous studies have shown that ER stress is involved in the occurrence and development of cardiovascular diseases, including hypertensionCitation26, atherosclerosisCitation27, and PAHCitation28. ER stress can lead to eNOS uncoupling and reduce endothelial NO production, thus driving endothelial dysfunction and hypertensionCitation29,Citation30. PAH is characterized by vasoconstriction and vascular remodeling of pulmonary artery, and reduction of ER stress by 4-phenylbutyric acid prevents the development of hypoxia-induced PAHCitation31. Furthermore, the upregulation of endothelin-1 inhibits endothelial nitric oxide synthase via ER stress response in endothelial cells, which could contribute to the occurrence and development of PAHCitation32. Consistent with previous findingsCitation31, our current study also found that the levels of ER stress were significantly increased in animal models of PAH, and omentin-1 treatment could reduce ER stress. However, how omentin-1 regulates ER stress needs to be further clarified.
AMPK signaling is an important regulator of energy metabolism and has been shown to play a protective role against cardiovascular diseaseCitation33. AMPK deficiency in smooth muscles causes persistent pulmonary hypertension of the new-born and premature deathCitation34. Activation of AMPK inhibits Galectin-3-induced pulmonary artery smooth muscle cells proliferation by upregulating hippo signaling effector YAPCitation35. Activation of AMPK ameliorates endothelial dysfunction in type 1 diabetic rats by suppressing ER stressCitation36. In our present study, we found that the level of p-AMPK was significantly down-regulated in pulmonary-artery rings from rats exposed to hypoxia. However, omentin-1 significantly increased AMPK levels. We found that AMPK inhibitor reduced the ability of omentin-1 to attenuate ER stress and the subsequent endothelial dysfunction in pulmonary-artery rings from rats exposed to hypoxia. Thus, our studies provide solid evidence that omentin-1 alleviates ER stress via AMPKα signaling in rats exposed to hypoxia.
In conclusion, this study demonstrated that omentin-1 ameliorated PAH by inhibiting ER stress through AMPKα signaling. Collectively, omentin-1 could be taken as a potential agent for the treatment of PAH.
Author contribution
XD conceived and designed the experiments and wrote the manuscript; HL performed the experiments and analyzed the data; JH and WD contributed reagents analysis tools; DX approved the final version of the manuscript.
Availability of data and materials
The data sets used and/or analyzed during the current study are available from the corresponding author on reasonable request
Disclosure statement
No potential conflict of interest was reported by the author(s).
Additional information
Funding
References
- Ruopp NF, Cockrill BA. Diagnosis and treatment of pulmonary arterial hypertension: a review. JAMA. 2022;327(14):1379–8. doi:10.1001/jama.2022.4402.
- Beshay S, Sahay S, Humbert M. Evaluation and management of pulmonary arterial hypertension. Respir Med. 2020;171:106099. doi:10.1016/j.rmed.2020.106099.
- Ghofrani HA, Voswinckel R, Reichenberger F, Weissmann N, Schermuly RT, Seeger W, Grimminger F. Hypoxia- and non-hypoxia-related pulmonary hypertension — established and new therapies. Cardiovasc Res. 2006;72(1):30–40. doi:10.1016/j.cardiores.2006.07.025.
- Naeije R, Richter MJ, Rubin LJ. The physiological basis of pulmonary arterial hypertension. Eur Respir J. 2022;59(6):2102334. doi:10.1183/13993003.02334-2021.
- Lan C, Fang G, Qiu C, Li X, Yang F, Yang Y. Inhibition of DYRK1A attenuates vascular remodeling in pulmonary arterial hypertension via suppressing STAT3/Pim-1/NFAT pathway. Clin Exp Hypertens. 2024;46:2297642. doi:10.1080/10641963.2023.2297642.
- Gorelova A, Berman M, Al Ghouleh I. Endothelial-to-mesenchymal transition in pulmonary arterial hypertension. Antioxid Redox Signal. 2021;34:891–914. doi:10.1089/ars.2020.8169.
- Klinger JR, Abman SH, Gladwin MT. Nitric oxide deficiency and endothelial dysfunction in pulmonary arterial hypertension. Am J Respir Crit Care Med. 2013;188(6):639–46. doi:10.1164/rccm.201304-0686PP.
- Bhogal S, Khraisha O, Al Madani M, Treece J, Baumrucker SJ, Paul TK. Sildenafil for pulmonary arterial hypertension. Am J Ther. 2019;26(4):e520–26. doi:10.1097/MJT.0000000000000766.
- Askin L, Duman H, Ozyildiz A, Tanriverdi O, Turkmen S. Association between omentin-1 and coronary artery disease: pathogenesis and clinical research. Curr Cardiol Rev. 2020;16(3):198–201. doi:10.2174/1573403X16666200511085304.
- Sun Y, Jiang R, Hu X, Gong S, Wang L, Wu W, Li J, Kang X, Xia S, Liu J, et al. CircGSAP alleviates pulmonary microvascular endothelial cells dysfunction in pulmonary hypertension via regulating miR-27a-3p/BMPR2 axis. Respir Res. 2022;23(1):322. doi:10.1186/s12931-022-02248-7.
- Abe JI, Ko KA, Kotla S, Wang Y, Paez-Mayorga J, Shin IJ, Imanishi M, Vu HT, Tao Y, Leiva-Juarez MM, et al. MAGI1 as a link between endothelial activation and ER stress drives atherosclerosis. JCI Insight. 2019;4(7). doi:10.1172/jci.insight.125570.
- Pan T, Zhang L, Miao K, Wang Y. A crucial role of endoplasmic reticulum stress in cellular responses during pulmonary arterial hypertension. Am J Transl Res. 2020;12(5):1481–90.
- Zhuan B, Wang X, Wang MD, Li ZC, Yuan Q, Xie J, Yang Z. Hypoxia induces pulmonary artery smooth muscle dysfunction through mitochondrial fragmentation-mediated endoplasmic reticulum stress. Aging (Albany NY). 2020;12(23):23684–97. doi:10.18632/aging.103892.
- Luo H, Lan C, Fan C, Gong X, Chen C, Yu C, Wang J, Luo X, Hu C, Jose PA, et al. Down-regulation of AMPK/PPARδ signalling promotes endoplasmic reticulum stress-induced endothelial dysfunction in adult rat offspring exposed to maternal diabetes. Cardiovasc Res. 2022;118(10):2304–16. doi:10.1093/cvr/cvab280.
- Correale M, Tricarico L, Grazioli D, Formica ES, Petrucci R, Persichella P, Di Biase M, Brunetti ND. Drug-induced pulmonary arterial hypertension: mechanisms and clinical management. Cardiovasc Drugs Ther. 2019;33(6):725–38. doi:10.1007/s10557-019-06920-x.
- Evans CE, Cober ND, Dai Z, Stewart DJ, Zhao YY. Endothelial cells in the pathogenesis of pulmonary arterial hypertension. Eur Respir J. 2021;58(3):58. doi:10.1183/13993003.03957-2020.
- Watanabe T, Watanabe-Kominato K, Takahashi Y, Kojima M, Watanabe R. Adipose tissue-derived omentin-1 function and regulation. Compr Physiol. 2017;7:765–81. doi:10.1002/cphy.c160043.
- Zhao A, Xiao H, Zhu Y, Liu S, Zhang S, Yang Z, Du L, Li X, Niu X, Wang C, et al. Omentin-1: a newly discovered warrior against metabolic related diseases. Expert Opin Ther Targets. 2022;26(3):275–89. doi:10.1080/14728222.2022.2037556.
- Celik M, Nar R, Nar G, Sokmen E, Gunver G. Serum omentin-1 levels in hypertensive patients. J Hum Hypertens. 2021;35(3):290–95. doi:10.1038/s41371-020-00420-4.
- Biscetti F, Nardella E, Bonadia N, Angelini F, Pitocco D, Santoliquido A, Filipponi M, Landolfi R, Flex A. Association between plasma omentin-1 levels in type 2 diabetic patients and peripheral artery disease. Cardiovasc Diabetol. 2019;18(1):74. doi:10.1186/s12933-019-0880-7.
- Biscetti F, Nardella E, Rando MM, Cecchini AL, Angelini F, Cina A, Iezzi R, Filipponi M, Santoliquido A, Pitocco D, et al. Association between omentin-1 and major cardiovascular events after lower extremity endovascular revascularization in diabetic patients: a prospective cohort study. Cardiovasc Diabetol. 2020;19(1):170. doi:10.1186/s12933-020-01151-z.
- Elsaid NH, Sadik NA, Ahmed NR, Fayez SE, Mohammed NAE. Serum omentin-1 levels in type 2 diabetic obese women in relation to glycemic control, insulin resistance and metabolic parameters. J Clin Transl Endocrinol. 2018;13:14–19. doi:10.1016/j.jcte.2018.05.003.
- Xiang Y, Zhou Z, Zhu L, Li C, Luo Y, Zhou J. Omentin-1 enhances the inhibitory effect of endothelial progenitor cells on neointimal hyperplasia by inhibiting the p38 MAPK/CREB pathway. Life Sci. 2023;331:122061. doi:10.1016/j.lfs.2023.122061.
- Uemura Y, Shibata R, Kanemura N, Ohashi K, Kambara T, Hiramatsu-Ito M, Enomoto T, Yuasa D, Joki Y, Matsuo K, Ito, M., et al. Adipose-derived protein omentin prevents neointimal formation after arterial injury. FASEB J. 2015;29(1):141–151. 10.1096/fj.14-258129
- Liu F, Fang S, Liu X, Li J, Wang X, Cui J, Chen T, Li Z, Yang F, Tian J, et al. Omentin-1 protects against high glucose-induced endothelial dysfunction via the AMPK/PPARδ signaling pathway. Biochem Pharmacol. 2020;174:113830.
- Zhu J, Shao A, Wang C, Zeng C, Wang H. Inhibition of endoplasmic reticulum stress restores the balance of renal RAS components and lowers blood pressure in the spontaneously hypertensive rats. Clin Exp Hypertens. 2023;45:2202367. doi:10.1080/10641963.2023.2202367.
- Ozcan L, Tabas I. Calcium signalling and ER stress in insulin resistance and atherosclerosis. J Intern Med. 2016;280(5):457–64. doi:10.1111/joim.12562.
- Katseff A, Alhawaj R, Wolin MS. Redox and inflammatory signaling, the unfolded protein response, and the pathogenesis of pulmonary hypertension. Adv Exp Med Biol. 2021;1304:333–73. doi:10.1007/978-3-030-68748-9_17.
- Zachariah M, Maamoun H, Milano L, Rayman MP, Meira LB, Agouni A. Endoplasmic reticulum stress and oxidative stress drive endothelial dysfunction induced by high selenium. J Cell Physiol. 2021;236:4348–59. doi:10.1002/jcp.30175.
- Liu H, Li Y, Li M, Xie L, Li F, Pan R, Pei F. Follistatin-like 1 protects endothelial function in the spontaneously hypertensive rat by inhibition of endoplasmic reticulum stress through AMPK-dependent mechanism. Clin Exp Hypertens. 2023;45(1):2277654. doi:10.1080/10641963.2023.2277654.
- Koyama M, Furuhashi M, Ishimura S, Mita T, Fuseya T, Okazaki Y, Yoshida H, Tsuchihashi K, Miura T. Reduction of endoplasmic reticulum stress by 4-phenylbutyric acid prevents the development of hypoxia-induced pulmonary arterial hypertension. Am J Physiol Heart Circ Physiol. 2014;306(9):H1314–23. doi:10.1152/ajpheart.00869.2013.
- Lenna S, Townsend DM, Tan FK, Kapanadze B, Markiewicz M, Trojanowska M, Scorza R. HLA-B35 upregulates endothelin-1 and downregulates endothelial nitric oxide synthase via endoplasmic reticulum stress response in endothelial cells. J Immunol. 2010;184(9):4654–61. doi:10.4049/jimmunol.0903188.
- Wu S, Zou MH. AMPK, Mitochondrial function, and cardiovascular disease. IJMS. 2020;21(14):4987. doi:10.3390/ijms21144987.
- Moral-Sanz J, Lewis SA, MacMillan S, Meloni M, McClafferty H, Viollet B, Foretz M, Del-Pozo J, Mark Evans A. AMPK deficiency in smooth muscles causes persistent pulmonary hypertension of the new-born and premature death. Nat Commun. 2022;13(1):5034. doi:10.1038/s41467-022-32568-7.
- Zhang Q, Li W, Zhu Y, Wang Q, Zhai C, Shi W, Feng W, Wang J, Yan X, Chai L, et al. Activation of AMPK inhibits galectin-3-induced pulmonary artery smooth muscle cells proliferation by upregulating hippo signaling effector YAP. Mol Cell Biochem. 2021;476(8):3037–49. doi:10.1007/s11010-021-04131-3.
- Yao W, Wang K, Wang X, Li X, Dong J, Zhang Y, Ding X. Icariin ameliorates endothelial dysfunction in type 1 diabetic rats by suppressing ER stress via the PPARα/Sirt1/AMPKα pathway. J Cell Physiol. 2021;236(3):1889–902. doi:10.1002/jcp.29972.