Abstract
Urban water systems in industrialized countries have underpinned unprecedented improvements in urban living standards through effective drinking water supply, sanitation and drainage. However, conventional urban water systems are increasingly regarded as too rigid and not sufficiently resilient to confront growing social, technological and environmental complexity and uncertainty, manifested, for example, in the maladaptation to climate change, depletion of nonrenewable resources, and degrading urban livability. In response, a new urban water paradigm has emerged in the last two decades within the context of a broader societal change that promotes a more organic worldview over the classical mechanistic and technocratic understanding of reality. This article develops and applies an analytical framework to coherently describe the new paradigm and contrast it with the old urban water paradigm. The framework includes a philosophical foundation and set of methodological principles that shape the new paradigm’s approach to governance, management, and infrastructure.
Graphical Abstract
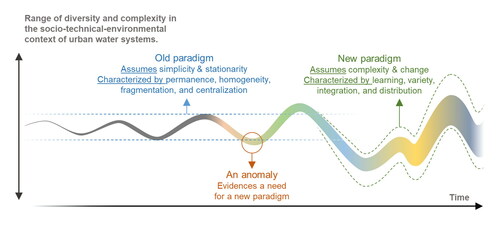
1. Introduction
The provision of water supply, sanitation and urban drainage services to households, businesses and communities has led to unprecedented improvements in life expectancy, economic growth, and quality of life in industrialized countries during the last 150 years. These services have relied on a system of social structures and material infrastructures—referred to in this paper as urban water systems (UWSs)—that have remained strikingly unchanged over the last century. In general terms society abstracts, cleans, transports, consumes and disposes water in the same ways it did at the end of the 19th century. However, the context in which these conventional UWSs operate has profoundly changed during recent decades. Western societies have grown increasingly complex due to accelerated technological development and faster exchange of information, where social, technical and biophysical elements have become more and more diversified and interdependent (Beck et al., Citation2003; Castells, Citation2010). This complexity has resulted in emerging problems—particularly climate change, rapid urbanization, and environmental degradation—and new societal needs, values and expectations—like social equity and urban livability—which conventional UWSs are poorly equipped to approach (Andoh et al., Citation2008; Bell, Citation2015; Daigger, Citation2009; Hering et al., Citation2013; Ludwig, Citation2001; Marlow et al., Citation2013; Pahl-Wostl et al., Citation2009). In other words, the ideas and assumptions that underpin our current UWSs are no longer fit for purpose.
A growing number of scholars have reported the gradual emergence of a new set of ideas and assumptions, a new mental framing or water paradigm (Gonzales & Ajami, Citation2017; Pahl-Wostl et al., Citation2011; Schoeman et al., Citation2014) that shapes new types of social structures and infrastructures capable of properly addressing current and anticipated needs and challenges. This new paradigm for UWSs can be seen as the local expression of a broader societal transformation that moves from a mechanistic to an organic worldview (Capra & Luisi, Citation2014; du Plessis & Brandon, Citation2015) which arguably started during the 1960s-70s (Franco-Torres, Citation2020) as an attempt to adapt to a more complex and dynamic reality. This broad paradigmatic transition had emerged in other sectors earlier (like urban planning (Jacobs, Citation1961), energy management (Lovins, Citation1976), or economic management (Schumacher, Citation1973)) and it is now increasingly recognizable in popular concepts like planetary boundaries (Rockström et al., Citation2009; Steffen et al., Citation2015) sustainability, resilience and green economy (UNEP, Citation2011), or the United Nations Sustainable Development Goals (UN, Citation2015).
While there is wide consensus about the existence of a new paradigm in the water sector, many authors have characterized the incumbent urban water paradigm as rigid and resistant to change, prone to continued operation under old beliefs and values despite evident problems of sustainability and increasingly complex societal needs (Brown & Farrelly, Citation2009; de Haan et al., Citation2015; Kiparsky et al., Citation2013; Roy et al., Citation2008). The incumbent paradigm has a distinct inertia as old ideas are entrenched within widespread technologies and infrastructures, management practices, rules, or organizational structures. This inertia is useful in providing stability and certainty, but also creates an impediment for adaptation to a changing reality. New ideas risk being discarded in favor of solutions that are firmly ingrained in the incumbent paradigm; they do not fit with established framings. See, for example, Sofoulis’ (Citation2015) description of the difficulty of introducing rainwater tanks—despite their obvious advantages—in the Australian water sector, Binz et al.’s (Citation2016) report of problems to legitimize potable water reuse in California, or Coombes et al.’s (Citation2016) analysis of engineering and economic assumptions belonging to the old paradigm impeding the adoption of governance policies toward water cycle management.
Despite this so-called lock-in, a growing number of scholars, policymakers and practitioners recognize the need for innovative approaches that derive from the new paradigm. Salient examples include Singapore’s integration of the whole water cycle (Jensen & Nair, Citation2019; Lee & Tan, Citation2016), urban design responses that are sensitive to water environments in Melbourne (Australia) (Brown et al., Citation2013; Ferguson, Brown, Frantzeskaki, et al., Citation2013), the use of stormwater to enhance urban livability in Copenhagen (Denmark) (Franco-Torres et al., Citation2020; Ziersen et al., Citation2017), and collaborative planning processes in Rotterdam (The Netherlands) (de Graaf & van der Brugge, Citation2010; Dunn et al., Citation2017).
Thorough analysis of these successful case studies often point to key factors that supported the local adoption of new solutions, like the work of champions, the creation of communities of practice, the diffusion of narratives, or the creation of pilot projects. We argue, however, that a broader enactment of the new urban water paradigm could be accelerated with a better understanding of the paradigm itself, and an integrated definition of its constituent elements, which so far remain dispersed and fragmented in the literature. A plethora of normative water management frameworks that implicitly reflect the new paradigm () has emerged (Esmail & Suleiman, Citation2020; Furlong et al., Citation2015; Schoeman et al., Citation2014), typically focusing on particular aspects of management, theories, and methods incorporated from other disciplines. These frameworks tend to be ambiguous (Biswas, Citation2004; Furlong et al., Citation2015; Molle, Citation2008) and “remain open to a multitude of interpretations which pose insurmountable obstacles in finding practical ways for their implementation” (Saurí & del Moral, Citation2001, p. 352). We argue that this coexistence of similar and ill-defined frameworks and terms means they tend to compete, hindering understanding and the development of the discipline and associated practices. The rampant diversity of partially overlapping terms used in the subfield of urban drainage management serves as a prime example of the reigning confusion (Chocat et al., Citation2001; Fletcher et al., Citation2015).
Table 1. Selection of management frameworks.
We therefore suggest that a transition to more sustainable and adaptive urban water management could be accelerated if scholars, policymakers and practitioners become conscious of their cognitive framings that may limit the consideration of alternative solutions, and of the existence of an alternative and coherent paradigm that can more effectively respond to present and future water-related needs (Abson et al., Citation2017; Meadows, Citation1999).
Certainly, there have been several insightful attempts to describe this new water paradigm (Capodaglio et al., Citation2016; Gleick, Citation2000; Grigg, Citation1998; Keath & Brown, Citation2009; Marlow et al., Citation2013; Ma et al., Citation2015; Mitchell, Citation2006; Novotny et al., Citation2010; Pahl-Wostl et al., Citation2011; Pinkham, Citation1999; Schoeman et al., Citation2014; Zandaryaa & Tejada-Guibert, Citation2009). However, these have not engaged with an in-depth explanation of what a paradigm is, tending to list characteristics that lack connection or a clear structure. They also tend to emphasize a particular water service—either drinking water provision, stormwater management, wastewater treatment, or water ecology—and have scarce reference to their common philosophical foundations.
This article therefore aims to describe a coherent framework that holistically connects the multiple ideas that underpin the new urban water paradigm and its derived social and technological structures in the water sector, across the different water services, and with particular attention to their shared philosophical foundations—the same foundations that underpin the broader social paradigm now emerging.
2. An analytical framework to describe urban water paradigms
Our paradigm framework encompasses three main categories: philosophical foundations, methodology, and operational articulations ().
Figure 1. Urban water paradigm framework, encompassing three main categories and seven themes. Philosophical foundations (grey) provide the basis for methodological principles (orange), which further supports the operational articulations of UWSs (blue).
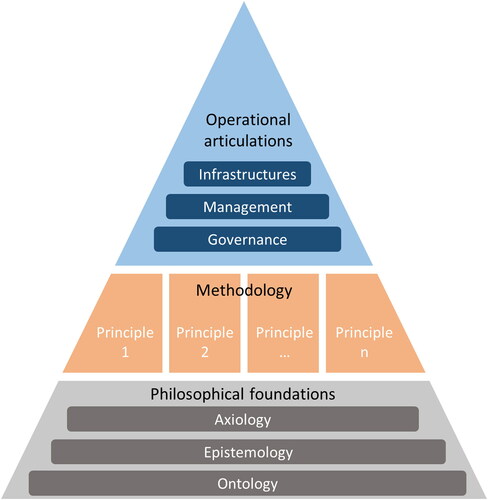
The first category, philosophical foundations, encompasses three themes that correspond with three nested branches of philosophy, which underpin both the broader social transformation and the sectorial transformation of the new urban water paradigm. These themes are rarely discussed explicitly in literature on UWSs. The first branch of philosophy, ontology, describes how the paradigm conceives the structure and nature of reality. The second, epistemology, expresses how knowledge about that reality is obtained. The third, axiology, describes the needs and values that guide actions. The description of these philosophical foundations builds on a theoretical argument that borrows elements from a wide range of disciplines, including science studies, philosophy, complexity studies, cybernetics or systems thinking.
The second category, methodology, encompasses a series of methodological principles that both reflect the paradigm’s foundational philosophies and shape or orient the design of water governance, management, and infrastructures. These three elements are therefore referred to as operational articulations of an UWS.
The first operational articulation, governance, includes the social structures and practices that allow actors to work together in order to achieve common goals. The second, management, defines how interventions on the available resources are understood, planned, implemented, monitored and evaluated —within the rules and policies demarked by governance—in order to fulfill societal needs. And third, the design, construction, and operation of infrastructure mediates society with its natural environment and makes the management of resources possible. Infrastructure, at the top of the framework, represents the most tangible signature of an urban water paradigm, the tip of the iceberg, and serves as the mechanism for delivering urban water services.
In the following, we elaborate these different aspects of our framework, gradually moving from the abstract theory of ontology to the most practical examples of infrastructure of urban water systems. The sources that support the framework have been identified through a nonsystematic literature screening that included 148 key books and articles. The methodology of this search and the selected sources can be found in the supplemental material that accompanies this article.
In addition, the analysis and examples presented below juxtapose the old and the new urban water paradigm, making clear that both are holistic understandings of the world with their corresponding governance, management and infrastructure.
3. Philosophical foundations
3.1. Ontology
The understanding of reality that lies behind the old urban water paradigm—its ontology—is deeply influence by classical Newtonian physics (Dunn et al., 2016) and more concretely by its ontological reductionism (Biswas, Citation2004). This perspective describes the world as an orderly place where the similarities among elements are highlighted—and their dissimilarities neglected—in order to create a limited number of discrete and homogeneous categories. These elements are assumed to be poorly interconnected. Their relationships are linear—i.e. propagate change proportionally—and governed by few, simple, well-defined, deterministic, and immutable laws that provide simplicity and regularity, creating subsystems that are independent of their context and eternally oscillate within well-defined boundaries (Guba, Citation1990; Mazzocchi, Citation2016). All these characteristics suggest the metaphor of the world as a deterministic clockwork machine (Capra & Luisi, Citation2014; Heylighen et al., Citation2007; Human & Cilliers, Citation2013; Morin, Citation2007).
In contrast, the ontology of the new urban water paradigm is as a complex system (Coombes & Kuczera, Citation2002; Voulvoulis et al., Citation2017). This emphasizes the heterogeneity of elements and their strong interdependence, recognizing a holistic system behavior rather than focusing on the study of the individual elements in isolation (Ackoff, Citation1991). There is not a universally accepted definition of complex system (Mikulecky, Citation2001; M. Mitchell, Citation2009), but most agree they are profusely interconnected systems that can generate emergent behaviors. Individual component elements typically have multiple, short-ranged, and dynamic connections with neighboring elements (von Foerster, Citation2002). As a result, while the number of elements grows linearly, the number of links among elements grows exponentially (Cilliers, Citation1998; Heylighen, Citation1999). High interconnectivity also means complex systems are typically open, exhibiting rich interactions with its environment and making it difficult to delimit a boundary between the system and its context (M. Mitchell, Citation2009). This high interconnectivity renders in practice a dense and continuous reality that is constantly modulated, a space that is experienced as a continuous heterogeneity—what in physics is known as a field—with unique local properties. All these characteristics of complex systems facilitates the metaphor of the world as a living organism (Waldrop, Citation1993), rather than as a machine.
Despite the short range of interactions between neighboring elements, their rich connectivity allows the propagation, modulation and amplification of signals through long ranges, producing multiple circular causations and positive (reinforcing) feedback loops (von Bertalanffy, Citation1968). This provokes non-linear behaviors; very small signals can get amplified, resulting in unpredictable system-wide change (Kofman & Senge, Citation1993; Waldrop, Citation1993). Feedback signals can also be negative, providing temporal order and stability to the system by counteracting perturbations. However, this stability is superficial because complex systems are in a permanent dynamic state, which guarantees its survival: “Equilibrium is another word for death” (Cilliers, Citation1998, p. 4). Complex systems have a history and continuously evolve.
Interestingly then, complex systems are self-organizing; they lack a central controller (Prigogine & Stengers, Citation1997; Waldrop, Citation1993). They create new structures and behaviors at the macro level that could not be inferred from the local rules that govern the relationships of the entities and their individual properties. This phenomenon, characteristic of complex systems, is called emergence (Heylighen et al., Citation2007; Kauffman, Citation1995; Prigogine & Stengers, Citation1997) and can be easily recognized in systems like ant colonies, DNA, or markets. The human brain is also a good example: the study of individual neurons does not provide much information about the emergence of human consciousness.
This transformation of ontology permeates the water sector, which is today being widely understood as complex, non-stationary and susceptible to emergent behaviors at physical and social levels (Larson et al., Citation2015; Milly et al., Citation2008; OECD, Citation2015).
3.2. Epistemology
As for ontology, the quest for knowledge about the world in the old urban water paradigm is heavily influenced by the classical Newtonian physics, from which it inherits an epistemological reductionism (Morin, Citation2007). In the same way that one can disassemble a clockwork to understand its mechanisms, (epistemological) reductionism attempts to explain the functioning of a well-defined system by analyzing its constituent elements and their relationships. It involves the isolation of a subsystem from its context, its fragmentation in smaller parts, and their classification in homogenous categories. Then, it defines the relationship among parts to finally infer the “regular” behavior of the whole system, and predict its future state (Kofman & Senge, Citation1993; Mazzocchi, Citation2016). Relying on reductionism, the “apparent” complexity is never a hindrance for the acquisition of knowledge, as it is assumed that all systems can be reduced to simpler ones in order to be easily understood.
However, this reduction to simplicity does not eliminate complexity, it just makes it invisible by neglecting the particularities of the constituent parts, their rich and dynamic relationships, and their dependence on the context (Morin, Citation2007). Whereas reductionism may be an acceptable explanatory approach to well-defined and isolated problems (like basic water services), its utility to understand and predict complex, open, and dynamic systems (such as the urban water services demanded by industrialized societies today) is limited (Cilliers, Citation1998; Kofman & Senge, Citation1993).
Unfortunately, the distinction between simple and complex is not always straightforward (Andersson et al., Citation2014; Kurtz & Snowden, Citation2003). From the point of view of an observer embedded in a complex system, everything may appear simple: its own properties, the short-range relationships with its neighboring elements, and the extension of the system are known. However, this same observer is usually unaware about the dependence on its context, the feedback effects of its own actions, and the emergent phenomena at the system level. A complex system is, therefore, incompressible (Richardson & Cilliers, Citation2001); any model that perfectly mimics its behavior must be at least as complex as the systems themselves, easily surpassing the human capacity of understanding. Then uncertainty is not about external randomness, but rather about the observer’s lack of knowledge (epistemic uncertainty) (di Baldassarre et al., Citation2016). This realization has influenced the epistemology of the new urban water paradigm, which has shifted from reductionism to holism, highlighting the contextual, dynamic, and always uncertain nature of knowledge.
In particular, the embracing of uncertainty is a key epistemological transformation. Relying on the power of reductionism and the deterministic nature of reality, the old paradigm is self-confident and predictive. It assumes that by carefully observing the past and accumulating knowledge about the mechanics of the system, it is possible to make accurate predictions and design optimal solutions, fostering the dream of a future without uncertainty. Contrarily, the new paradigm rejects simplicity, regularity, and the power attributed to reductionism. It focuses instead on open and dynamic systems, non-linear processes, emergent phenomena that are unpredictable, and the inability of the observer of acquiring the necessary knowledge (Allen et al., Citation2011; di Baldassarre et al., Citation2016; Heylighen et al., Citation2007; Morcol, Citation2001; Prigogine & Stengers, Citation1984).
Based on the perceived deterministic nature of reality and the power of reductionism, scientists and practitioners embedded in the old paradigm firmly believe they see the world “as it is”; that an objective reality exists “out there”, to which they have direct access through careful observation, quantification, and reason. In this view, humans are external and objective observers that search for the unique truth awaiting to be unpacked (Morin, Citation1977; Zwarteveen & Boelens, Citation2014). As there is just one possible (rational) interpretation of reality, this has to be revealed by experts that apply supposedly rigorous and value-free scientific methods, yielding a context-independent knowledge that will unambiguously settle all disputes and orient policy design (Sarewitz, Citation2004).
Contrarily, the new paradigm recognizes that knowledge in a complex system is always imperfect and subjective because there are no fixed points of reference or external points of view. The observer is inexorably embedded in the observed system and any of her interpretations are inevitably situated and contextual (Cilliers, Citation1998; Prigogine & Stengers, Citation1984). For example, a complex system like the Internet cannot possibly be objectively and comprehensibly described by one user, who can only aim at providing a description of his use of the network and contextualized experience. Narratives, then, are effective ways to describe a certain aspect of a complex system, to provide structure and meaning under particular circumstances from a partial view, while still being coherent with the underlying objective reality (Lyotard, Citation1984).
Cilliers (Citation1998) gives perhaps a better illustration of narrative knowledge by picturing a complex system as a dynamic network (). A narrative forms one of multiple possible paths through the network that rest on the objective truth. These paths are just temporal framings, subjective interpretations of a connection between an input and an output, defined in terms of particular and temporal points of view, needs, and constraints.
Figure 2. Alternative narratives (green and brown lines) provide situated explanations of a complex system (blue network) that do not necessarily contradict the underlying reality (the dots represent scientific “hard” facts). This figure is inspired by Cilliers (Citation1998, p. 130).
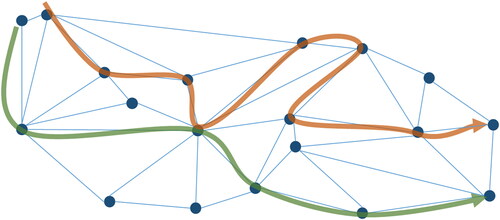
The new paradigm recognizes the impossibility of finding the absolute truth and that strictly scientific knowledge built from the point of view of a single discipline has limited value. It focuses instead on “pragmatic” or “useful” truths (Pierce, Citation2011) that “work” in a certain context or situation. They are bricolage narratives (Levi-Strauss, Citation1968) that integrate heterogeneous sources of information (Barbosa et al., Citation2012; Blanco-Gutiérrez et al., Citation2013; Croke et al., 2007) and have a practical relevance for concrete problems and needs. This “useful” truth is the only truth that will affect us, and the only truth that we may know (Bach et al., Citation2014; Gerlak, Citation2008; Harremoës, Citation2002).
The water management literature offers us multiple examples about the adoption of this new epistemology, advocating for participatory water management, multidisciplinary solutions, incorporation of uncertainty in planning, or continuous experimentation (Farrelly & Brown, Citation2011; Pan & Guo, Citation2019; Varady et al., Citation2016).
3.3. Axiology
In our review of ontology and epistemology we saw that the old urban water paradigm is essentially anthropocentric; the “external reality” is reduced to only those things that humans can observe or understand. Complexity and an ecological perspective are largely disregarded, largely due to the lack of the cognitive capacity (Simon, Citation1997) and analytical tools (Kellert, Citation1994) necessary to understand them. Unsurprisingly then, the fundamental values that steer behavior in the old paradigm (axiology) are also fundamentally anthropocentric; subsistence and (economic) growth. These are translated into a few universal, independent, and easily identifiable needs that typically include the provision of sufficient and safe drinking water, sanitation, and drainage (de Graaf et al., Citation2007; Gleick, Citation2000; Pahl-Wostl et al., Citation2011; Sofoulis, Citation2005), eclipsing any other “superfluous” needs.
This approach derives from an instrumental view of nature (Beck et al., Citation2003), which is regarded as a neutral context that lacks any intrinsic value. Nature is simultaneously seen as an unlimited source of resources, which generates a feeling of dominance for people, and as a constraining frame to fulfill human needs, which generates a feeling of fear related to the possibility of losing control of it (Wolfe & Brooks, Citation2017). The relationship with nature is, therefore, competitive. It is about nature’s benefit or humans’ benefit; a zero-sum game (Bernhardt et al., Citation2006). For example, there is a conflict between maintaining ecological flows of rivers and increasing abstractions of water to meet growing water demands.
The complex systems approach of the new urban water paradigm reveals that humans are not independent of their environmental context, but rather a part of it (Brooks & Brandes, Citation2011; Mebratu, Citation1998; Schmidt, Citation2013). Instead of competing with nature, humans must collaborate with it and design synergistic solutions that contribute to support human wellbeing (Bernhardt et al., Citation2006; Costanza & Daly, Citation1992; van Zeijl-Rozema et al., Citation2008; Zandaryaa & Tejada-Guibert, Citation2009). For example, during the last decade most industrialized countries have approved legislation that protect ecological flows in rivers in order to improve social welfare (EC, Citation2015; ICCATF, Citation2011). Therefore, the most salient values of the new paradigm are ecological sustainability and associated social welfare (sometimes referred as livability) (Garrote, Citation2017; Partzsch, Citation2009). This does not negate the importance of the values of the old paradigm, but expands them to include many others like physical and mental health, recreation, beauty, sense of community and social integration, equality, justice, or even cultural and spiritual values (de Haan et al., Citation2014; Ferguson, Brown, & Deletic, Citation2013; Marlow et al., Citation2013; Zwarteveen & Boelens, Citation2014).
The values considered in the new urban water paradigm are varied, ill-defined, subjective, interdependent (often conflicting) (Wong & Brown, Citation2009) and incommensurable. Therefore, it has become common praxis to lump them in the ambiguous concept of sustainability. This concept is not exclusive to the new paradigm; it has also been utilized in the old paradigm with a slightly different meaning. In line with the linear thinking of the old paradigm, sustainability has traditionally been understood as a static and objective goal or end-state, a point of optimal and static equilibrium in a perfect future where all needs are fulfilled in harmonic balance (Brown et al., Citation1987; Hardi, Citation1997). This is the so-called substantive sustainability (Truffer et al., Citation2010) and it is often reflected in sustainability indicators (UN, Citation2007; van der Steen & Howe, Citation2009; van Leeuwen, Citation2013) that provide a “deterministic single-criterion optimality” (Reed & Kasprzyk, Citation2009, p. 411).
In contrast, procedural sustainability (Truffer et al., Citation2010), which is more in line with the philosophical underpinnings of the new paradigm, acknowledges the dynamic nature of needs and values, and the complex system in general (e.g. Slocombe, Citation1990). In this interpretation, sustainability is an open-ended process—not a goal—that focuses on the available pathways to reach a moving target—a dynamic, socially constructed, unachievable ideal (Bagheri & Hjorth, Citation2007; Newman, Citation2005; Nonaka & Toyama, Citation2005; Voß & Kemp, Citation2006)—that must be constantly renegotiated within an evolving context (Robinson & Cole, Citation2015). Contrasting with the ambivalent feelings of dominance and fear that characterized the old paradigm, the new paradigm is associated with feelings of humbleness, hope and enthusiasm, guided by a utopic image of human welfare in perfect harmony with nature (Franco-Torres, Citation2020).
4. Methodology
Within a paradigm, a problem can be conceptualized as the factor that opens a gap between the present state and desired (optimal or sustainable) state where certain needs are effectively fulfilled. Building on the Merriam-Webster dictionary definition, this conceptualization leads to an understanding of methodology as “a body of methods, rules, and postulates employed by a discipline” to acquire knowledge or solve problems. Similarly, in the case of a paradigm, we interpret a methodology as a set of (methodological) principles, designed to modify or regulate the present state of things, solve concrete problems, and approximate to a desired state. These principles are shaped by the paradigm’s philosophical foundations and used as a guide to define a regulator. From the point of view of cybernetics, regulators are sub-systems that locally constrain the variation of a wider system in which it is embedded (its sociotechnical-environmental context) within certain bounds in order to fulfill a certain set of needs (Ackoff, Citation1991; Ashby, Citation1956).
4.1. UWS as regulators of their context
An UWS can be conceptualized as a regulator. Urban water services like drinking water provision, sanitation or drainage require an UWS that regulates certain natural processes (basically to retain, convey, or treat water) with physical infrastructures (like dams, pipes, pumps and water treatment plants) and regulates certain social behaviors with social rules (like policies, guidelines, contracts, prices, technical standards and roles). To do so, the UWS, and more concretely its operational articulations (governance, management, and infrastructures), follow a set of characteristic methodological principles associated with each paradigm.
A core theorem of cybernetics, states that "every good regulator of a system must be a model [a replica] of that system" (Conant & Ashby, Citation1970, p. 89). Accordingly, the old urban water paradigm’s methodology proposes an UWS that projects the stationarity and simplicity of its context and problems, while the new paradigm’s methodology promotes an UWS that mimics the complexity and dynamism of its context and problems.
4.2. Tame problems vs wicked problems
In order to explain the methodological principles of each paradigm, it is convenient to describe first what problems they aim to solve, which clearly align with their respective ontologies.
For the old urban water paradigm, the simplicity of the world and the well-defined needs and values yield what Rittel and Webber (Citation1973) call tame problems; simple, clearly structured, and static problems that are independent from other problems (Bagheri & Hjorth, Citation2007; Pahl-Wostl et al., Citation2011). Among several possible solutions there is always a unique optimal alternative—the “right solution”—an UWS configuration that has the capacity to solve the problem once and for all, which can be rationally inferred and that must be imposed as standard (R. R. Brown et al., Citation2006; Kreuter et al., Citation2004; Sarewitz, Citation2004).
The new paradigm focuses instead on wicked problems, which are complex, interdependent, unstructured, and pervasive (Rittel & Webber, Citation1973). There are infinite solutions to wicked problems but none of them are optimal or definitive—there are no silver bullets (Capodaglio et al., Citation2016; Ludwig et al., Citation1993). Whereas different solutions fulfill interlinked needs in variable degrees, being more or less attractive from different points of view (Kreuter et al., Citation2004), they also alter the system in a way that creates new problems elsewhere. Typical wicked problems in UWSs are the pervasive challenges that give rise to the need for a new paradigm, such as climate change, urbanization and non-point source pollution.
4.3. Methodological principles. From control to resilience
By focusing on a perceived existence of optimal and definitive solutions to tame problems, the old paradigm aims to build UWSs that function as rigid regulators based on prediction and control. These are able to withstand natural disruptions and change, keep homeostasis, and permanently fulfill a limited set of basic and independent needs in a de-contextualized environment (Capodaglio et al., Citation2016; de Bruijn et al., Citation2017; Pahl-Wostl, Citation2007).
From the perspective of the new paradigm this prediction and control approach is seen as a naïve delusion; it is considered not only ineffective, but also may result in unexpected and undesirable consequences (Holling & Meffe, Citation1996; Ludwig et al., Citation1993). For example, the straightening of rivers and construction of canals to facilitate urban development often results in greater flood risk (Castonguay, Citation2007; Wolsink, Citation2010). Instead, the new paradigm is inclined toward the development of resilience (Folke, Citation2006; Holling, Citation1973) as a regulative function to fulfill human and environmental needs.
Certainly, resilience has become a buzzword in academia and policy over the last decade, receiving varied—and sometimes contraposed—interpretations (Béné et al., Citation2014; Davoudi, Citation2012; Folke, Citation2006). For example, engineering resilience refers to the capacity of a system to quickly recover from a range of disturbances and maintain its ability to deliver its single intended function (de Bruijn et al., Citation2017; Holling & Meffe, Citation1996). This interpretation is more aligned with the old urban water paradigm, which aims to resist change by building up a threshold capacity to buffer contextual variations (Gleick, Citation2000), rigidly controlling the system and keeping it in homeostasis.
In contrast, the definition of resilience we attribute to the new paradigm, aligned with the concept of procedural sustainability, is the so-called evolutionary resilience (Davoudi, Citation2012). This resilience can be defined as the capacity of a regulatory system to continuously adapt to changes, identify synergies, and avoid conflicts with its environment in order to deliver a timely and convenient set of variable functions (Berkes et al., Citation2008; Simmie & Martin, Citation2010; Walker et al., Citation2004). This approach is radically opposed to the control methodology of the old paradigm and its engineering resilience, which seeks to force and dominate the environment to permanently yield a concrete output. Evolutionary resilience requires then relentless efforts of adjustment to ever changing values, knowledge and physical variables (Darnhofer et al., Citation2016; Takala, Citation2017), without losing fundamental structures that give continuity to the system (Herrfahrdt-Pahle & Pahl-Wostl, Citation2012). The design of flood-prone neighborhoods serves as a good illustrative example (Hale, Citation2016; Rode & Gralepois, Citation2017), where resilience is achieved through a range of measures (e.g. elevated buildings, flow-through neighborhoods, water storage, reduction of imperviousness) that reduce risks and simultaneous support new functions that improve urban livability.
We have identified four pairs of opposite principles that contrast the control methodology of the old paradigm and the resilience methodology of the new paradigm: stationarity vs learning, homogenization vs variety, fragmentation vs integration, and centralization vs distribution. Later we will explore how these four principles, shaped by the philosophical foundations of each paradigm, become reified as the operational articulations of the UWS.
4.3.1. Stationarity vs learning
To permanently dominate the environment and deliver a consistent service, the old urban water paradigm constrains the natural variability within predetermined bounds, forcing it to be stationary (Gleick, Citation2000; Schoeman et al., Citation2014). This is done in practice by, for example, constructing large hydraulic infrastructures like reservoirs for water storage, desalination plants, or dikes for flood retention. In this conservative approach, the reliability of infrastructures stands out as the main issue since a loss of control is potentially catastrophic. There is an aversion to uncertainty and risk, relying on only well-known, standard, and fail-safe methods that stifle innovation and experimentation (Brown & Farrelly, Citation2009; Farrelly & Brown, Citation2011; Harremoës, Citation2002).
Contrarily, the new paradigm sees stationarity in UWSs as a problem rather than a solution, since it promotes a non-responsive regulation, neglecting the emergence of new needs and the evolving nature of context (). Constant learning by doing—i.e. relentless experimentation (Allen et al., Citation2011; Farrelly & Brown, Citation2011; Kato & Ahern, Citation2008; Moberg & Galaz, Citation2005; Vreugdenhil et al., Citation2010)—is a preferred methodological principle that pragmatically reveals convenient ways to adapt to a dynamic and uncertain context. Small experiments purposefully create controlled instabilities and low-regret alternatives where it is safe to fail (Hashimoto et al., Citation1982; Holling, Citation1973), fostering innovation and anticipating emergent events, allowing the timely adaptation of an UWS to its environment (Conant & Ashby, Citation1970). However, learning not only requires proactive and persistent experimentation, but also the acceptance of uncertainty, tolerance of failure, constant monitoring, sensitivity to recognize change, trends and opportunities, reflexivity to continuously reconsider frames and goals, and the flexibility associated with the capacity to abandon old practices and structures and incorporate new ones (Burnham et al., Citation2016; Gunderson & Holling, Citation2002; Jiggins et al., Citation2007; Schelfaut et al., Citation2011; Wolsink, Citation2010).
Figure 3. UWSs operate under non-stationary conditions (green line) (Milly et al., Citation2008). The old paradigm assumes stationarity; based on past behavior predicts that the conditions will remain within a certain range (blue stripe). Due to emergent phenomena (unknown unknowns (di Baldassarre et al., Citation2016)) the conditions unexpectedly move out of the predefined stability threshold. Contrarily, the new paradigm does not assume a fixed stability threshold, but continuously experiments (brown dots) to temporarily adjust to new conditions (brown vertical bars).
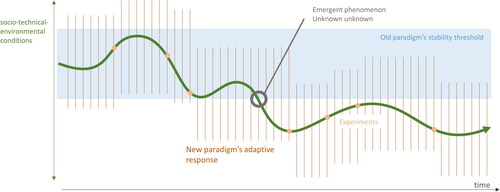
In contrast to the predictive approach of the old paradigm, the new paradigm turns to other type of learning that could be called abstract experimentation (also referred as possibilistic thinking (Clarke, Citation2008), what-if analysis (Brown et al., Citation2015), or counterfactual thought experiments (Klotz & Horman, Citation2010)). This type of experimentation consists of creating a range of hypothetical future scenarios (Ingram & Lejano, Citation2007; Novotny et al., Citation2010; Schoonenboom, Citation1995), typically narratives of success (dream scenarios) or narratives of failure (nightmare scenarios) that project backwards to the present, providing guidance for action.
4.3.2. Homogeneity vs variety
In cybernetics, the term variety refers to the total number of states in which a system can exist (Ashby, Citation1956). The law of requisite variety (Ashby, Citation1956, Citation1958) postulates that the greater variety of responses a regulator can perform (like policies, rules, management solutions, or infrastructures), the greater variety of disturbances the system is able to successfully adapt to.
The old urban water paradigm assumes the context to be simple and regular, making a large variety of regulatory responses a burden rather than a solution. Conversely, the new paradigm confronts a complex context and therefore fosters a larger variety in its constituent elements (Aerts et al., Citation2008; Wong & Brown, Citation2008) in order to enhance its capacity for local adaptation and innovation, efficiency or redundancy (R. Biggs et al., 2012; Keath & Brown, Citation2009).
The new paradigm promotes a many-to-many relationship between needs and solutions. A combination of interdependent interventions of different nature and scale (Marsalek & Schreier, Citation2009; Pahl-Wostl, Citation2007) provide a suboptimal and temporary accommodation of multiple, diffuse, ever-changing, and interdependent needs (Capodaglio et al., Citation2016; Gonzales & Ajami, Citation2015; Werbeloff & Brown, Citation2011), which also are deeply embedded in their unique local context (Coombes & Kuczera, Citation2002; Dunn et al., 2016; Liu et al., Citation2007).
4.3.3. Fragmentation vs integration
The old paradigm rests on the underlying assumption that both the regulatory system (the UWS) and its regulated context can be divided in isolated subsystems that perform easily identifiable functions. These individual elements can be locally optimized and reassembled to produce universal optimal solutions (Schoeman et al., Citation2014; Wong & Brown, Citation2009). Accordingly, the fragmentation principle (methodological reductionism) becomes a prerequisite for prediction and control (Capra & Luisi, Citation2014; Turton & Meissner, Citation2002).
However, during the last two decades it has become widely accepted in the water sector that fragmentation in governance, management and infrastructures is a serious barrier to sustainability (Mukhtarov, Citation2008). Fragmentation represents the negation of the systemic nature of reality and implies an artificial rupture of connections, generating confrontations, interferences, inefficiencies, and risks (Brown & Farrelly, Citation2009; GWP, Citation2000; Ioris, Citation2008; OECD, Citation2016)
Integration, on the other hand, reinforces the systems ontology of the new urban water paradigm. Focusing attention on the dynamic relationships among parts and with their context (being context-sensitive), it produces a holistic view that is more likely to produce (evolutionary) resilient outcomes than a fragmented one (Gonzales & Ajami, Citation2015; Hardy et al., Citation2005; Varady et al., Citation2016; Wong & Brown, Citation2009). It can, for example, facilitate the development of coordination and synergies (R. Biggs et al., 2012), reduce tradeoffs and conflicts (Pahl-Wostl et al., Citation2008; Wolsink, Citation2006), suppress vulnerabilities (Gober, Citation2010; Pahl-Wostl et al., Citation2007), allow auto-regulation, and foster serendipity (Darnhofer et al., Citation2016; Merton & Barber, Citation2011).
4.3.4. Centralization vs distribution
Despite its tendency toward fragmentation, the structures of the old paradigm are not completely disconnected. They exhibit centralized designs of control that excel at top-down integration where a central node concentrates resources. Still, centralized systems rely on fragmentation and homogenization. This is the typical scheme of networks of water distribution dependent on a single water treatment plant, or organizational schemes in hierarchical organizations dependent on a single leader.
Opposing centralization, many scholars argue that the new urban water paradigm supports decentralization (Daigger, Citation2009; Larsen et al., Citation2013; Leigh & Lee, Citation2019; Zhang et al., Citation2009), which implies that the whole system is not dependent on a central node, with the elements of the UWS geographically dispersed and often working in isolation. This claim is in line with the principle of variety, however, it opposes integration. Strictly speaking, decentralized sets of elements do not constitute a system because they may be disconnected—for example, a single household that exclusively relies on a private water well.
Instead, we argue that the varied and integrated regulatory systems of the new paradigm are actually distributed (Baran, Citation1964) (). Distribution, as decentralization, implies that the elements of the system are not exclusively dependent on a central node and that the nodes are geographically dispersed, but in addition it requires that the elements are connected to exchange resources and information to (ideally) all adjacent elements (Baran, Citation1964; Ryan, Citation2009). These “nodes” are semi-autonomous agents, meaning that they “work” at a range of scales; they both function autonomously, and they function as a part of a larger system generating emergent behaviors. Distributed systems are in general terms more resilient than both centralized and decentralized systems (C. Biggs et al., Citation2009; Chanan et al., Citation2009). They reduce risks, increase efficiency, and are more flexible and adaptable (Baran, Citation1964).
Figure 4. Types of system configurations. Adapted from (Baran, Citation1964). The points indicate units of production or consumption (differences in shape, size and color indicate their homogeneity), while the lines indicate their links to exchange resources and information.
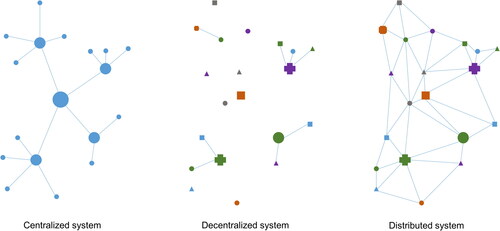
5. Operational articulations
Earlier, we conceptualized UWSs as regulators of their context that aim to solve water-related problems and fulfill water-related needs. They include aspects of governance, management, and infrastructures that reify some methodological principles, which in turn are shaped by the philosophical foundations of their corresponding paradigm.
In the old urban water paradigm, UWSs are meant to fulfill few, well-defined, immutable and non-contested needs (like drinking water provision, sanitation, and drainage) (Pahl-Wostl et al., Citation2011). This means that in order to achieve those well-defined goals, old management focuses on how to physically control nature and keep it within optimal bounds. The UWS of the old paradigm is therefore largely a material or technical issue (Saurí & del Moral, Citation2001; Swyngedouw, Citation1999).
In contrast, the new urban water paradigm sees water not only as a material issue, but also as a social issue (Zwarteveen & Boelens, Citation2014). It considers a variety of ill-defined and often conflicting needs in an ever-changing context that must be navigated and accommodated with help of good governance (Mguni et al., Citation2015). Indeed, recent literature widely acknowledges that water problems are mostly problems of governance (Bucknall et al., Citation2006; OECD, Citation2016; Pahl-Wostl, Citation2015; van Dijk, Citation2012).
5.1. Governance
The old paradigm assumes that it is possible to rationally design a simple and rigid institutional framework that provides guidance toward the optimal fulfillment of a few universal and undisputed water needs, including a small set of formal rules that keep human behavior in check—largely ignoring social or cultural variability (Bakker, Citation2010; Ioris, Citation2008; Pahl-Wostl, Citation2008).
The design of this rational system of rules and policies is the duty of a select group of actors with well-defined roles (the government) that are organized in rigid, centralized, hierarchical structures. The final decision-makers—usually politicians—are at the top, far from the resources that are being managed (Castonguay, Citation2007; Chandler, Citation2014), and carry the ultimate responsibility for water services (Turton & Meissner, Citation2002). They concentrate the authority, power, legitimacy, and information to rationally control the system by imposing formal coercive rules (Bakker, Citation2010). These decision-makers are supported by experts (Brown, Citation2005)—often engineers (Ingram & Schneider, Citation1998)—who have access to the “unique” truth. At the bottom of the hierarchy are the operators and consumers, whose participation in the policy design and rule-making is deemed as unnecessary or even detrimental (Bagheri & Hjorth, Citation2007; Schoeman et al., Citation2014; van Dijk, Citation2012), as the “right” technical decisions are already defined by experts: the beneficiaries of urban water services are mere rule-followers (Turton & Meissner, Citation2002).
However, when the old style of governance tries to engage with growing institutional complexity, where stakeholders have conflicting values, interests, agendas and horizons, sector-specific policies and rules become contradictory (Zandaryaa & Tejada-Guibert, Citation2009); governance becomes fragmented and multiple contestations and interferences emerge (Brown & Farrelly, Citation2009; Segrave et al., Citation2014). Governance problems become wicked.
The new paradigm fully recognizes that these problems transcend science and technology (Funtowicz & Ravetz, Citation1993; Weinberg, Citation1972) and cannot be optimally and permanently solved, fostering instead the coherence of local governance with its social context (Gonzales & Ajami, Citation2017; Neto, Citation2016; Wade, 2011) and the internal integration of policies and rules that affect the UWS. This integration requires wide participation of all actors (Brandes & Kriwoken, Citation2006; Carr et al., Citation2012; Zandaryaa & Tejada-Guibert, Citation2009), with active engagement on the definition of problems and the design of coherent and synergistic policies and rules across sectors (Ananda & Proctor, Citation2013; Everard & McInnes, Citation2013; Mitchell, Citation2006). More concretely, participation is deemed essential to: gather diverse resources, skills, knowledge, values, interests and needs (Allon & Sofoulis, Citation2006; Arnold, Citation2013; Jameson & Baud, Citation2016; Rijke et al., Citation2013; van der Brugge, Citation2009; van Dijk, Citation2012); harness enthusiasm and commitment (Patterson et al., Citation2013; Sofoulis, Citation2015); provide transparency, trust, and equity (Dietz et al., Citation2003; Domènech et al., Citation2013; Hahn et al., Citation2006; Wolsink, Citation2010); and confer legitimacy on the selected alternatives (Hering et al., Citation2013; Sofoulis, Citation2015).
This new governance (Osborne, Citation2010) is distributed in clusters (also referred to as network or polycentric governance). These clusters create partnerships between diverse actors through interactions to find synergies and negotiate conflicting interests (Bos et al., Citation2015; Pahl-Wostl et al., Citation2008; Torfing et al., Citation2012). For example, between public agencies specializing in different sectors (not only for water provision, sanitation, or flood prevention, but also other sectors like transport, energy, urbanism and recreation), private actors (like technology providers, consultants or land developers), research actors (like universities and research centers) and civil society organizations (like NGOs and neighborhood associations). These interactions are conducted not only through formal relationships, but also through informal (shadow) networks (Bos et al., Citation2015).
At the same time, there is a shift from the few rigid roles in the old paradigm to a wide variety of overlapping and flexible roles. For example, government agencies like water utilities are not only supply developers, but also resource custodians and information providers (Brown et al., Citation2009; Pires, Citation2004; Prasad Pandey & Kazama, Citation2014). For distributed infrastructures, consumers also become producers (prosumers) (Novotny et al., Citation2010; Sofoulis, Citation2015) of their own water supply or wastewater, and private competitors also become collaborators to achieve synergistic solutions. All those actors are dependent on each other to fulfill their duties and goals. For example, public water utilities are often dependent on private contractors or consultants to deliver the desired water service.
Hence, governance in the new paradigm is not the exclusive function of the government (Gleick, Citation2000; van de Meene et al., Citation2011; van Dijk, Citation2012); it is the collaborative effort of a group of actors with access to power, legitimacy, information, and knowledge in varying degrees, which aim to carry out enterprises that often involve conflicting interests (Costa et al., Citation2012)—water services become everybody’s responsibility (Turton & Meissner, Citation2002). The outcomes of this distributed governance are collaboratively created and emergent, instead of rationally planned by an elite (Bos & Brown, Citation2012). Therefore, pragmatic solutions arise from a learning approach that involves participation, continuous experimentation, monitoring, and revision of strategies, policies and rules (Bos & Brown, Citation2012; Hukka & Katko, Citation2015; Jameson & Baud, Citation2016; OECD, Citation2011). Policies are not fixed solutions, but instead “questions masquerading as answers” (Gunderson, Citation1999).
5.2. Management
The regulative function of management in the old urban water paradigm has a clear bias toward simplification and homogenization. For example, water is classified in binary: it is either fit or unfit for consumption, it is a resource or a waste (Bindra et al., Citation2003; Partzsch, Citation2009; Pinkham, Citation1999). Potable water, the highest water quality, is employed for all purposes (one-size-fits-all), including drinking, irrigation and toilet flushing. After its use, it is considered a waste and conveyed to the sewer, regardless of its quality or new characteristics. Compare this with the new urban water paradigm, which considers that all water is valuable, even when it is of low quality (Listowski et al., Citation2009; Wilcox et al., Citation2016). Here, water of the highest quality is used for human consumption, while lower quality water can be used for different non-consumptive purposes by matching it with their intended use (fit-for-purpose) (Gikas & Tchobanoglous, 2007; Lee & Tan, Citation2016; Makropoulos et al., Citation2018).
Another example is stormwater, which, in the old paradigm, is always considered a nuisance that must invariably be drained away by underground pipes—the only and standard structural solution. Conversely, in the new paradigm stormwater is seen as a valuable resource that contributes to improving urban amenity (Martin et al., Citation2007). Stormwater management tools are also manifold (Chocat et al., Citation2001; Hale, Citation2016; Marsalek & Schreier, Citation2009; Meinzen-Dick, Citation2007), including structural and technical solutions (like various green infrastructures or more traditional infrastructures), economic incentives and disincentives (like markets, insurances, innovative rate structures, taxes, rebates, or subsidies), or sociopolitical instruments (like benchmarking systems, educational and behavioral programs, water rights, changes in routines, or even organizational reforms).
The few, simple problems and solutions considered by the old paradigm are managed as if they were independent from other subsystems, while the new paradigm pays attention to the linkages between multiple problems and multiple solutions. For instance, while drinking water provision, sanitation, and urban drainage have traditionally been managed as independent subsystems in the water sector (Anderson & Iyaduri, Citation2003; Mukheibir et al., Citation2014), the new paradigm focuses on the coordinated management of these water services (Mitchell, Citation2006; Ross, Citation2018; Vairavamoorthy et al., Citation2015). Stormwater can be a source of drinking water (Campisano et al., Citation2017; Sharma et al., Citation2013; Sofoulis, Citation2015), leaky sewers can pose a pollution risk for water supply, and wastewater can be used to refill groundwater aquifers (Binz et al., Citation2016; Evans & Evans, Citation2012).
The fragmentation (methodological) principle of the old paradigm is also reflected operationally in other ways. For example, water management is usually approached through the individual lens offered by a particular discipline or functional silo (like hydraulics, hydrology, biology or economics) (Brown, Citation2005; Brown & Farrelly, Citation2009; Garrote, Citation2017; Saraswat et al., Citation2017). These predict the behavior of a few environmental variables and describe clear, linear paths of action to accomplish their objectives independently of other goals or constraints (Deng et al., Citation2013; Loorbach, Citation2014). Another example of fragmentation in management is the separation of UWSs from natural processes (its context), which must be understood, predicted, and tightly controlled. In practice, this means that natural processes not directly benefitting human interest must be disrupted or constrained, and substituted by rationally designed linear processes that permanently fulfill a fixed set of human needs (Bagheri & Hjorth, Citation2007; Brandes et al., Citation2005). For example, the natural water cycle is disrupted and converted to an artificial one-path-flow process (Daigger, Citation2009): raw water (the input) is abstracted from far locations where it is easily accessible (the “external” context), transported through long distances (often by interbasin transfers) (Domènech et al., Citation2013; Gleick, Citation2000; Saurí & del Moral, Citation2001), treated and distributed, consumed and polluted (the output), and discarded as waste back to nature (the “external” context) (Bindra et al., Citation2003; Everard & McInnes, Citation2013; Rojas et al., Citation2015; Takala, Citation2017). This linear flow creates an illusion of resource abundance (Stuart, Citation2007), in which higher demand urges increased raw water abstraction from the environment (Gleick, Citation2003; Saurí & del Moral, Citation2001).
In contrast, the integration (methodological) principle of the new paradigm invites a style of management that is context-sensitive and mimics or allies synergistically with natural processes of cyclical character (Byrnes, Citation2013; Zandaryaa & Tejada-Guibert, Citation2009), rather than a parallel linear process of environmental control (Hering et al., Citation2013; Niemczynowicz, Citation1999). For instance, it mimics circular natural processes where water—together with its associated energy and nutrients—is recovered or recycled to remain part of the system, as there is not an “outside” where it can be infinitely extracted or disposed (Anderson, Citation2003; Gondhalekar & Ramsauer, Citation2017; Haase, Citation2015; Hoff, Citation2011; Pennisi, Citation2012; WWAP, Citation2017). Following this logic, the concepts of waste (for wastewater) or nuisance (for stormwater) become obsolete because any element is eventually recycled and should be rather seen as a potential resource (Arden et al., Citation2019; Chocat et al., Citation2001; Grant et al., 2012; Ma et al., Citation2015; Novotny et al., Citation2010), use of which saves costs, prevents pollution and avoids the depletion of their sources (Chanan et al., Citation2013; Hemmes et al., Citation2011; van der Hoek et al., Citation2016; Wallace et al., Citation2017).
This type of management approaches also aligns with so-called nature-based (“green”) solutions for water (WWAP, Citation2018), which utilize ecosystems that can potentially deliver any water-related service that humans might require (MEA, Citation2005; Schuch et al., Citation2017)—for example, flood risk management and natural drainage (Pappalardo et al., Citation2017), water purification (Everard & McInnes, Citation2013), urban cooling (Norton et al., Citation2015; Schmidt, Citation2010), support of biodiversity (Filazzola et al., Citation2019), or even enhancement of physical and psychological health (Tzoulas et al., Citation2007)—often with lower costs and higher efficiencies than those of the “grey” solutions. Context-sensitive management requires then a local management style that benefits from intimate knowledge of local characteristics (like ecology, geomorphology, infrastructures, urban form, demographics, rules, standards and cultural characteristics) seen from an integrated perspective (Ferguson, Brown, & Deletic, Citation2013; Marlow et al., Citation2013; Mitchell, Citation2006; Rygaard et al., Citation2014).
Finally, management planning clearly reflects an epistemological transformation in shifting from the old to the new urban water paradigm. The old paradigm relies on isolated mathematical models that are regarded as prediction machines to find optimal solutions that unambiguously point toward the “right” course of action. Contrast this with the management planning of the new paradigm, which aims at producing pragmatic illustrations of reality (Bach et al., Citation2014; Deletic et al., Citation2018; Schmitt & Huber, Citation2006) and does not dismiss predictive models but combines them in a process of iterative and situated bricolage. It integrates their results (Brouwer & van Ek, Citation2004; Croke et al., 2007; Zhou, Citation2014) to produce hypothetical scenarios and narratives that improve the understanding of complex UWSs and support—but never settle—the decision making process (Bagheri & Hjorth, Citation2007; Rygaard et al., Citation2014; Westley et al., Citation2011).
5.3. Infrastructures
Infrastructures are the physical manifestation of urban water paradigms, reflecting their understanding of reality, relationship with nature and most important needs and values.
Considering that the old paradigm aims at physically forcing natural processes into certain linear processes to fulfill human needs, it is not surprising that in this frame, UWSs becomes a mechanical and technocratic issue (Capodaglio et al., Citation2016; de Bruijn, Citation2004; Wolsink, Citation2010), with focus on the construction of robust infrastructures (normally built from concrete, plastic or metal materials) (Pinkham, Citation1999).
Conversely, in the new paradigm, the concept of infrastructure acquires a wider meaning, merging technical and environmental elements that build synergies with its social and environmental context (Fletcher et al., Citation2015; Goonetilleke et al., Citation2005; Masi et al., 2017; Novotny, Citation2009). For example, infrastructures benefit from ecosystem services (Carlson et al., Citation2015; Fletcher et al., Citation2015) and strengthen popular values in our contemporary society like livability and sustainability, shaping and supporting certain social identities and social behaviors (A. Amin, Citation2014; Bell, Citation2015) ().
Figure 5. (a) In the old paradigm, hard infrastructures are the dominant factor, the socioeconomic environment is perceived as simple, and the natural environment is reduced to a container for the resources that need controlling and as a sink for residuals. Linear production (one-way flow) results in the depletion of resources and the proportional creation of pollution. The products and services produced are few and the capital costs high. Adapted from Sahely et al. (Citation2005). (b) In the new paradigm, infrastructures merge with the complex socioeconomic and natural environment, supporting circular flows of resources without residuals, and generating multiple products and services. The capital compromised is low as the system is more efficient and self-sustaining.
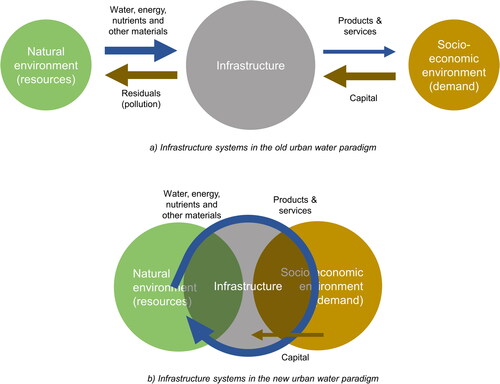
The old paradigm’s infrastructures are large and robust constructions with definite and long lifespans (Sharma et al., Citation2010). They respond to the need to withstand and dominate nature, create optimal economies of scale, and support professional management by technical experts. They exhibit a limited repertoire of standard, independent, and discrete elements that perform only one function—generally of hydraulic character—and are linearly connected in centralized schemes (Ashley et al., Citation2015; Everard & McInnes, Citation2013; Partzsch, Citation2009; Pinkham, Citation1999). Typical examples are large water treatment plants, urban channels, or dams.
On the other hand, infrastructures of the new urban water paradigm are distributed (Fane, Citation2005): varied, decentralized, and integrated (Chanan et al., Citation2009; Chocat et al., Citation2007; Makropoulos & Butler, Citation2010; Mitchell, Citation2006; Sharma et al., Citation2010). They form richly connected networks that continuously exchange resources and information (Yuan et al., Citation2019). These networks encompass locally adapted and semi-autonomous elements (Novotny, Citation2009; Rygaard et al., Citation2011) that have multiple forms and sizes (Fryd et al., Citation2010; Novotny et al., Citation2010; Saurí & Palau-Rof, Citation2017), are made with natural and artificial materials, perform and contribute to circular processes, and continuously fulfill multiple functions at multiple scales (Fletcher et al., Citation2015; Gill et al., Citation2007; Novotny et al., Citation2010; Pappalardo et al., Citation2017; Semadeni-Davies et al., Citation2008; Sharma et al., Citation2013). They conform organic systems in constant adaptation that can be regarded as ephemeral infrastructures with indefinite lifespans (Capodaglio et al., Citation2016; Chanan et al., Citation2010; Vieira et al., Citation2014).
While the infrastructure of the old paradigm is comprised of independent elements, invisible to the public (often buried, like pipes, or in distant locations, like treatment plants), and detached from its context, the new paradigm exhibits ubiquitous networks firmly embedded in the city fabric and environment, intentionally visible and representing a vital part of the public life (Bernhardt et al., Citation2006; Brandes & Brooks, Citation2007; Gleick, Citation2003; Pahl-Wostl et al., Citation2011; Saurí & del Moral, Citation2001). For example, stormwater managed at a catchment scale with a distributed network of green roofs, swales, or urban creeks that support biodiversity, provide an esthetic value, trap pollutants, act as temperature regulators for the city, or diminish the peak runoff under a storm (Andoh et al., Citation2008; Berardi et al., Citation2014; Schuch et al., Citation2017; Wong & Brown, Citation2009).
Advocates of distributed infrastructures argue that they are more resilient than centralized systems for several reasons. First, because they make possible a locally tailored management approach with solutions that efficiently adapt to multiple contexts, purposes or types of resources (Chanan et al., Citation2013; Díaz et al., Citation2016; Keath & Brown, Citation2009; Leigh & Lee, Citation2019; Wolsink, Citation2006). Second, because their modular nature gives them a sensitivity and scalability that efficiently allow the system to adapt to changing circumstances (Amin & Han, Citation2007; Gikas & Tchobanoglous, 2007; Marlow et al., Citation2013). Third, because they work on the basis of redundancy and complementarity of other solutions at multiple scales, minimizing risk and providing alternative functions (Andoh et al., Citation2008; Gonzales & Ajami, Citation2017; Marlow et al., Citation2013; Werbeloff & Brown, Citation2011; Wong & Brown, Citation2009).
A disadvantage of distributed systems is that they develop slowly (Baran, Citation1964). Therefore, in practice, distributed water infrastructures are most often implemented as a supplement to existing centralized systems, which serve as the backbone that connects all nodes (Ferguson, Brown, Frantzeskaki, et al., Citation2013; Lee et al., Citation2013; Porse, Citation2013). However, it is expected that, with time, the local stations turn to be the main centers of production and consumption. This is the case of urban drainage systems, for example, where local infrastructures for stormwater management are built today to support the traditional centralized system, but eventually will manage most of the stormwater locally in a distributed fashion (Saurí & Palau-Rof, Citation2017).
6. Discussion and conclusion
In this paper, we articulate a coherent and holistic set of ideas, values and assumptions that are shaping urban water innovations that aim to respond adaptively to the non-stationary nature, uncertainty and emergent needs of our current society. This description is intended to equip water scholars, policymakers, and practitioners with a frame of reference to understand and embrace the benefits of novel styles of governance (like participative approaches), management (like circular use of resources) and infrastructures (like solutions based on ecosystem services). The articulation of the new paradigm that we provide may also offer concrete guidance for action and decision making to these actors when defining the types of governance arrangements, management systems, and infrastructures needed to improve the sustainability of UWSs in complex contexts; namely, promoting variety, integration, distribution and constant learning. For instance, scholars could be encouraged to consider problems from the lens of different disciplines; policymakers could open decision processes for participation by multiple stakeholders and the creation of intersectoral policies; and practitioners could continuously experiment with distributed infrastructures that simultaneously deliver multiple functions, complement each other, and build synergies with nature.
In developing the urban water paradigm framework, we have aimed to be coherent but not necessarily comprehensive, as the depth of the paradigm cannot be fully encapsulated in a single article. The characterization of the new paradigm that we present in this article should be regarded then as a heuristic tool or an ideal type (Doty & Glick, Citation1994); an idealized model that does not exist exactly as described anywere in the world, but that serves as a benchmark to recognize and create innovative approaches that help to address emerging challenges in the water sector. Therefore, future studies that analyze the degree of implementation of these new styles of governance, management, and infrastructure could provide valuable insight into the key enablers and strategies that have helped enact the key methodological principles in practice.
Finally, we reflect on the parallel shifts being experienced in other parts of society as part of a broader paradigm change. According to recent literature, most sectors—like energy (Geels et al., Citation2017; Verbong & Geels, Citation2010), health (Johansen & van den Bosch, Citation2017), and education (Yarime et al., Citation2012)—are experiencing similar transitions toward more sustainable modes of production and consumption (Loorbach et al., Citation2017). These transitions reflect the same underlying changes in society that drive the transformation of the water sector, and share multiple aspects with the new urban water paradigm—like promotion of diversity, learning approaches, distributed structures, or greater citizen participation. The construction of a more solid definition of the new urban water paradigm would benefit from a deeper analysis of the roots of this broader societal change through further research.
Supplemental Material
Download MS Word (19.8 KB)Supplemental Material
Download MS Word (148.1 KB)Additional information
Funding
References
- Abson, D. J., Fischer, J., Leventon, J., Newig, J., Schomerus, T., Vilsmaier, U., von Wehrden, H., Abernethy, P., Ives, C. D., Jager, N. W., & Lang, D. J. (2017). Leverage points for sustainability transformation. Ambio, 46(1), 30–39. https://doi.org/10.1007/s13280-016-0800-y
- Ackoff, R. L. (1991). Science in the systems age: Beyond IE, OR, and MS BT - facets of systems science. In G. J. Klir (Ed.), Facets of systems science (pp. 325–335). Springer US. https://doi.org/10.1007/978-1-4899-0718-9_23
- Aerts, J. C. J. H., Botzen, W., Veen, A. v d., Krywkow, J., & Werners, S. E. (2008). Dealing with uncertainty in flood management through diversification. Ecology and Society, 13(1) https://doi.org/10.5751/ES-02348-130141
- Allen, C. R., Fontaine, J. J., Pope, K. L., & Garmestani, A. S. (2011). Adaptive management for a turbulent future. Journal of Environmental Management, 92(5), 1339–1345. https://doi.org/10.1016/j.jenvman.2010.11.019
- Allon, F., & Sofoulis, Z. (2006). Everyday water: Cultures in transition. Australian Geographer, 37(1), 45–55. https://doi.org/10.1080/00049180500511962
- Amin, A. (2014). Lively infrastructure. Theory, Culture & Society, 31(7-8), 137–161. https://doi.org/10.1177/0263276414548490
- Amin, M. T., & Han, M. (2007). Scope/need of soft path water resource management in developing countries. Water Supply, 7(5-6), 185–192. https://doi.org/10.2166/ws.2007.109
- Ananda, J., & Proctor, W. (2013). Collaborative approaches to water management and planning: An institutional perspective. Ecological Economics, 86, 97–106. https://doi.org/10.1016/j.ecolecon.2012.10.018
- Anderson, J. (2003). The environmental benefits of water recycling and reuse. Water Supply, 3(4), 1–10. https://doi.org/10.2166/ws.2003.0041
- Anderson, J., & Iyaduri, R. (2003). Integrated urban water planning: Big picture planning is good for the wallet and the environment. Water Science and Technology: A Journal of the International Association on Water Pollution Research, 47(7-8), 19–23. https://doi.org/10.2166/wst.2003.0666
- Andersson, C., Törnberg, A., & Törnberg, P. (2014). Societal systems - Complex or worse? Futures, 63, 145–167. https://doi.org/10.1016/j.futures.2014.07.003
- Andoh, R. Y. G., Faram, M. G., & Osei, K. (2008). Urban Water Management: Innovations and Paradigm Shifts to Address 21st Century Needs [Paper Presentation]. World Environmental & Water Resources Congress 2008; May 12-16, 2008, Honolulu, Hawaii, November 29). https://doi.org/10.1061/40976(316)419
- Arden, S., Ma, X., (Cissy), & Brown, M. (2019). Holistic analysis of urban water systems in the Greater Cincinnati region: (2) resource use profiles by emergy accounting approach. Water Research X, 2, 100012. https://doi.org/10.1016/j.wroa.2018.100012
- Arnold, T. R. (2013). Procedural knowledge for integrated modelling: Towards the Modelling Playground. Environmental Modelling & Software, 39, 135–148. https://doi.org/10.1016/j.envsoft.2012.04.015
- Ashby, W. R. (1956). An introduction to cybernetics. Chapman & Hall.
- Ashby, W. R. (1958). Requisite variety and its implications for the control of complex systems. Cybernetica, 1(2), 83–99. https://doi.org/10.1007/978-1-4899-0718-9_28
- Ashley, R. M., Lundy, L., Ward, S., Shaffer, P., Walker, A. L., Morgan, C., Saul, A., Wong, T., & Moore, S. (2013). Water-sensitive urban design: Opportunities for the UK. Proceedings of the Institution of Civil Engineers - Municipal Engineer, 166(2), 65–76. https://doi.org/10.1680/muen.12.00046
- Ashley, R. M., Woods-Ballard, B., Shaffer, P., Wilson, S., Illman, S., Walker, L., D’Arcy, B., & Chatfield, P. (2015). UK sustainable drainage systems: Past, present and future. Proceedings of the Institution of Civil Engineers - Civil Engineering, 168(3), 125–130. https://doi.org/10.1680/cien.15.00011
- Bach, P. M., Rauch, W., Mikkelsen, P. S., McCarthy, D. T., & Deletic, A. (2014). A critical review of integrated urban water modelling – Urban drainage and beyond. Environmental Modelling & Software, 54, 88–107. https://doi.org/10.1016/j.envsoft.2013.12.018
- Bagheri, A., & Hjorth, P. (2007). Planning for sustainable development: A paradigm shift towards a process-based approach. Sustainable Development, 15(2), 83–96. https://doi.org/10.1002/sd.310
- Bakker, K. (2010). Privatizing water: Governance failure and the world’s urban water crisis. Cornell University Press.
- Baran, P. (1964). On distributed communications networks. IEEE Transactions on Communications, 12(1), 1–9. https://doi.org/10.1109/TCOM.1964.1088883
- Barbosa, A. E., Fernandes, J. N., & David, L. M. (2012). Key issues for sustainable urban stormwater management. Water Research, 46(20), 6787–6798. https://doi.org/10.1016/j.watres.2012.05.029
- Beck, U., Bonss, W., & Lau, C. (2003). The theory of reflexive modernization: Problematic, hypotheses and research programme. Theory, Culture & Society, 20(2), 1–33. https://doi.org/10.1177/0263276403020002001
- Bell, S. (2015). Renegotiating urban water. Progress in Planning, 96, 1–28. https://doi.org/10.1016/j.progress.2013.09.001
- Béné, C., Cannon, T., Gupte, J., Mehta, L., Tanner, T. (2014). Exploring the potential and limits of the resilience agenda in rapidly urbanising contexts. https://opendocs.ids.ac.uk/opendocs/bitstream/handle/20.500.12413/3621/ER63 Exploring the Potential and Limits of the Resilience Agenda in Rapidly Urbanising Contexts.pdf?sequenc
- Berardi, U., GhaffarianHoseini, A., & GhaffarianHoseini, A. (2014). State-of-the-art analysis of the environmental benefits of green roofs. Applied Energy, 115, 411–428. https://doi.org/10.1016/j.apenergy.2013.10.047
- Berkes, F., Colding, J., & Folke, C. (2008). Navigating social-ecological systems: Building resilience for complexity and change. Cambridge University Press.
- Bernhardt, E., Bunn, S. E., Hart, D. D., Malmqvist, B., Muotka, T., Naiman, R. J., Pringle, C., Reuss, M., & Wilgen, B. v. (2006). Perspective: The challenge of ecologically sustainable water management. Water Policy, 8(5), 475–479. https://doi.org/10.2166/wp.2006.057
- Biggs, C., Ryan, C., Wiseman, J., Larsen, K. (2009). Distributed water systems: A networked and localised approach for sustainable water services. https://minerva-access.unimelb.edu.au/bitstream/handle/11343/162197/234_Distributed-Water-Systems.VEIL_.pdf?sequence=1&isAllowed=y
- Biggs, R., Schlüter, M., Biggs, D., Bohensky, E. L., BurnSilver, S., Cundill, G., Dakos, V., Daw, T. M., Evans, L. S., Kotschy, K., Leitch, A. M., Meek, C., Quinlan, A., Raudsepp-Hearne, C., Robards, M. D., Schoon, M. L., Schultz, L., & West, P. C. (2012). Toward principles for enhancing the resilience of ecosystem services. Annual Review of Environment and Resources, 37(1), 421–448. https://doi.org/10.1146/annurev-environ-051211-123836
- Bindra, S. P., Muntasser, M., el Khweldi, M., & el Khweldi, A. (2003). Water use efficiency for industrial development in Libya. Desalination, 158(1-3), 167–178. https://doi.org/10.1016/S0011-9164(03)00447-8 https://doi.org/10.1016/S0011-9164(03)00447-8
- Binz, C., Harris-Lovett, S., Kiparsky, M., Sedlak, D. L., & Truffer, B. (2016). The thorny road to technology legitimation — Institutional work for potable water reuse in California. Technological Forecasting and Social Change, 103, 249–263. https://doi.org/10.1016/j.techfore.2015.10.005
- Biswas, A. K. (2004). Integrated water resources management: A reassessment. Water International, 29(2), 248–256. https://doi.org/10.1080/02508060408691775
- Blanco-Gutiérrez, I., Varela-Ortega, C., & Purkey, D. R. (2013). Integrated assessment of policy interventions for promoting sustainable irrigation in semi-arid environments: A hydro-economic modeling approach. Journal of Environmental Management, 128, 144–160. https://doi.org/10.1016/j.jenvman.2013.04.037
- Bos, J. J., & Brown, R. R. (2012). Governance experimentation and factors of success in socio-technical transitions in the urban water sector. Technological Forecasting and Social Change, 79(7), 1340–1353. https://doi.org/10.1016/j.techfore.2012.04.006
- Bos, J. J., Brown, R. R., & Farrelly, M. A. (2015). Building networks and coalitions to promote transformational change: Insights from an Australian urban water planning case study. Environmental Innovation and Societal Transitions, 15, 11–25. https://doi.org/10.1016/j.eist.2014.10.002
- Brandes, O. M., & Kriwoken, L. (2006). Changing perspectives — Changing paradigms: Taking the “Soft Path” to water sustainability in the Okanagan Basin. Canadian Water Resources Journal, 31(2), 75–90. https://doi.org/10.4296/cwrj3102075
- Brandes, O. M., Brooks, D. B. (2007). The soft path for water in a nutshell. The Polis Project on Ecological Governance. http://poliswaterproject.org/publication/23
- Brandes, O. M., Ferguson, K., M’Gonigle, M., Sandborn, C. (2005). At a watershed: Ecological governance and sustainable water management in Canada. Polis Project on Ecological Governance, University of Victoria. http://dspace.library.uvic.ca/bitstream/handle/1828/7960/At a Watershed.pdf?sequence = 1&isAllowed = y
- Brooks, D. B., & Brandes, O. M. (2011). Why a water soft path, why now and what then?. International Journal of Water Resources Development, 27(2), 315–344. https://doi.org/10.1080/07900627.2011.571235
- Brouwer, R., & van Ek, R. (2004). Integrated ecological, economic and social impact assessment of alternative flood control policies in the Netherlands. Ecological Economics, 50(1-2), 1–21. https://doi.org/10.1016/j.ecolecon.2004.01.020
- Brown, B. J., Hanson, M. E., Liverman, D. M., & Merideth, R. W. (1987). Global sustainability: Toward definition. Environmental Management, 11(6), 713–719. https://doi.org/10.1007/BF01867238
- Brown, C. M., Lund, J. R., Cai, X., Reed, P. M., Zagona, E. A., Ostfeld, A., Hall, J., Characklis, G. W., Yu, W., & Brekke, L. (2015). The future of water resources systems analysis: Toward a scientific framework for sustainable water management. Water Resources Research, 51(8), 6110–6124. https://doi.org/10.1002/2015WR017114
- Brown, R. R. (2005). Impediments to integrated urban stormwater management: the need for institutional reform. Environmental Management, 36(3), 455–468. https://doi.org/10.1007/s00267-004-0217-4
- Brown, R. R., & Farrelly, M. A. (2009). Delivering sustainable urban water management: A review of the hurdles we face. Water Science and Technology : A Journal of the International Association on Water Pollution Research, 59(5), 839–846. https://doi.org/10.2166/wst.2009.028
- Brown, R. R., Farrelly, M. A., & Loorbach, D. A. (2013). Actors working the institutions in sustainability transitions: The case of Melbourne’s stormwater management. Global Environmental Change, 23(4), 701–718. https://doi.org/10.1016/j.gloenvcha.2013.02.013
- Brown, R. R., Keath, N., & Wong, T. (2009). Urban water management in cities: Historical, current and future regimes. Water Science and Technology: A Journal of the International Association on Water Pollution Research, 59(5), 847–855. https://doi.org/10.2166/wst.2009.029
- Brown, R. R., Sharp, L., & Ashley, R. M. (2006). Implementation impediments to institutionalising the practice of sustainable urban water management. Water Science and Technology : A Journal of the International Association on Water Pollution Research, 54(6-7), 415–422. https://doi.org/10.2166/wst.2006.585
- Bucknall, J., Damania, R., & Rao, H. (2006). Good governance for good water management. In World Bank Group (Ed.), Annual Review July 2005–June 2006 (pp. 20–23).
- Burnham, M., Ma, Z., Endter-Wada, J., & Bardsley, T. (2016). Water management decision making in the face of multiple forms of uncertainty and risk. JAWRA Journal of the American Water Resources Association, 52(6), 1366–1384. https://doi.org/10.1111/1752-1688.12459
- Byrnes, J. (2013). A short institutional and regulatory history of the Australian urban water sector. Utilities Policy, 24, 11–19. https://doi.org/10.1016/j.jup.2012.09.001
- Campisano, A., Butler, D., Ward, S., Burns, M. J., Friedler, E., DeBusk, K., Fisher-Jeffes, L. N., Ghisi, E., Rahman, A., Furumai, H., & Han, M. (2017). Urban rainwater harvesting systems: Research, implementation and future perspectives. Water Research, 115, 195–209. https://doi.org/10.1016/j.watres.2017.02.056
- Capodaglio, A. G., Ghilardi, P., & Boguniewicz-Zablocka, J. (2016). New paradigms in urban water management for conservation and sustainability. Water Practice and Technology, 11(1), 176–186. https://doi.org/10.2166/wpt.2016.022
- Capra, F., & Luisi, P. L. (2014). The systems view of life: A unifying vision. Cambridge University Press.
- Carlson, C., Barreteau, O., Kirshen, P., & Foltz, K. (2015). Storm water management as a public good provision problem: Survey to understand perspectives of low-impact development for urban storm water management practices under climate change. Journal of Water Resources Planning and Management, 141(6), 04014080. https://doi.org/10.1061/(ASCE)WR.1943-5452.0000476
- Carr, G., Blöschl, G., & Loucks, D. P. (2012). Evaluating participation in water resource management: A review. Water Resources Research, 48(11) https://doi.org/10.1029/2011WR011662
- Castells, M. (2010). The rise of the network society. Wiley-Blackwell.
- Castonguay, S. (2007). The production of flood as natural catastrophe: Extreme events and the construction of vulnerability in the drainage basin of the St. Francis River (Quebec), mid-nineteenth to mid-twentieth century. Environmental History, 12(4), 820–844. https://doi.org/10.1093/envhis/12.4.820
- Chanan, A. P., Kandasamy, J., Vigneswaran, S., & Sharma, A. K. (2009). A gradualist approach to address Australia’s urban water challenge. Desalination, 249(3), 1012–1016. https://doi.org/10.1016/j.desal.2009.09.012
- Chanan, A. P., Vigneswaran, S., & Kandasamy, J. (2010). Valuing stormwater, rainwater and wastewater in the soft path for water management: Australian case studies. Water Science and Technology : A Journal of the International Association on Water Pollution Research, 62(12), 2854–2861. https://doi.org/10.2166/wst.2010.544
- Chanan, A. P., Vigneswaran, S., Kandasamy, J., & Simmons, B. (2013). Wastewater Management Journey – From Indus Valley Civilisation to the Twenty-First Century BT - Wastewater Reuse and Management (S. K. Sharma & R. Sanghi, Eds.; pp. 3–18). Springer. https://doi.org/10.1007/978-94-007-4942-9_1
- Chandler, D. (2014). Beyond neoliberalism: Resilience, the new art of governing complexity. Resilience, 2(1), 47–63. https://doi.org/10.1080/21693293.2013.878544
- Chocat, B., Ashley, R. M., Marsalek, J., Matos, M. R., Rauch, W., Schilling, W., & Urbonas, B. (2007). Toward the sustainable management of urban storm-water. Indoor and Built Environment, 16(3), 273–285. https://doi.org/10.1177/1420326X07078854
- Chocat, B., Krebs, P., Marsalek, J., Rauch, W., & Schilling, W. (2001). Urban drainage redefined: From stormwater removal to integrated management. Water Science and Technology: A Journal of the International Association on Water Pollution Research, 43(5), 61–68. https://doi.org/10.2166/wst.2001.0251
- Cilliers, P. (1998). Complexity and post-modernism: Understanding complex systems. Routledge.
- Clarke, L. (2008). Possibilistic Thinking: A New Conceptual Tool for Thinking about Extreme Events. Social Research, 75(3), 669–690.
- Conant, R. C., & Ashby, W. R. (1970). Every good regulator of a system must be a model of that system. International Journal of Systems Science, 1(2), 89–97. https://doi.org/10.1080/00207727008920220
- Coombes, P. J., Kuczera, G. (2002). Integrated urban water cycle management: Moving towards systems understanding. Proceedings of the 2nd National Conference on Water Sensitive Urban Design, Brisbane, 2–4.
- Coombes, P. J., Smit, M., & MacDonald, G. (2016). Resolving boundary conditions in economic analysis of distributed solutions for water cycle management. Australian Journal of Water Resources, 20(1), 11–29. https://doi.org/10.1080/13241583.2016.1162762
- Costa, H., Costa, G., Nascimento, N., Dias, J., Welter, M., & Nunes, T. (2012). Urban water management and planning: Urban sanitation policies and an emerging institutional structure. International Journal of Water, 6(3/4), 250–269. https://doi.org/10.1504/IJW.2012.049499
- Costanza, R., & Daly, H. E. (1992). Natural capital and sustainable development. Conservation Biology, 6(1), 37–46. https://doi.org/10.1046/j.1523-1739.1992.610037.x
- Croke, B. F. W., Ticehurst, J. L., Letcher, R. A., Norton, J. P., Newham, L. T. H., & Jakeman, A. J. (2006). Integrated assessment of water resources: Australian experiences. Water Resources Management, 21(1), 351–373. https://doi.org/10.1007/s11269-006-9057-8
- Daigger, G. T. (2009). Evolving urban water and residuals management paradigms: Water reclamation and reuse, decentralization, and resource recovery. Water Environment Research: A Research Publication of the Water Environment Federation, 81(8), 809–823. https://doi.org/10.2175/106143009x425898
- Darnhofer, I., Lamine, C., Strauss, A., & Navarrete, M. (2016). The resilience of family farms: Towards a relational approach. Journal of Rural Studies, 44, 111–122. https://doi.org/10.1016/j.jrurstud.2016.01.013
- Davoudi, S., Shaw, K., Haider, L. J., Quinlan, A. E., Peterson, G. D., Wilkinson, C., Fünfgeld, H., McEvoy, D., Porter, L., & Davoudi, S. (2012). Resilience: A bridging concept or a dead end?. Planning Theory & Practice, 13(2), 299–333. https://doi.org/10.1080/14649357.2012.677124
- de Bruijn, K. M. (2004). Resilience indicators for flood risk management systems of lowland rivers. International Journal of River Basin Management, 2(3), 199–210. https://doi.org/10.1080/15715124.2004.9635232
- de Bruijn, K. M., Buurman, J., Mens, M., Dahm, R., & Klijn, F. (2017). Resilience in practice: Five principles to enable societies to cope with extreme weather events. Environmental Science & Policy, 70, 21–30. https://doi.org/10.1016/j.envsci.2017.02.001
- de Graaf, R., & van der Brugge, R. (2010). Transforming water infrastructure by linking water management and urban renewal in Rotterdam. Technological Forecasting and Social Change, 77(8), 1282–1291. https://doi.org/10.1016/j.techfore.2010.03.011
- de Graaf, R., van de Giesen, N. C., & van de Ven, F. H. M. (2007). The closed city as a strategy to reduce vulnerability of urban areas for climate change. Water Science and Technology : A Journal of the International Association on Water Pollution Research, 56(4), 165–173. https://doi.org/10.2166/wst.2007.548
- de Haan, F. J., Ferguson, B. C., Adamowicz, R. C., Johnstone, P., Brown, R. R., & Wong, T. (2014). The needs of society: A new understanding of transitions, sustainability and liveability. Technological Forecasting and Social Change, 85, 121–132. https://doi.org/10.1016/j.techfore.2013.09.005
- de Haan, F. J., Rogers, B. C., Frantzeskaki, N., & Brown, R. R. (2015). Transitions through a lens of urban water. Environmental Innovation and Societal Transitions, 15, 1–10. https://doi.org/10.1016/j.eist.2014.11.005
- Deletic, A., Zhang, K., Jamali, B., Charette-Castonguay, A., Kuller, M., Prodanovic, V., & Bach, P. M. (2018). Modelling to support the planning of sustainable urban water systems [Paper presentation]. International Conference on Urban Drainage Modelling, 10–19.
- Deng, Y., Cardin, M.-A., Babovic, V., Santhanakrishnan, D., Schmitter, P., & Meshgi, A. (2013). Valuing flexibilities in the design of urban water management systems. Water Research, 47(20), 7162–7174. https://doi.org/10.1016/j.watres.2013.09.064
- di Baldassarre, G., Brandimarte, L., & Beven, K. (2016). The seventh facet of uncertainty: Wrong assumptions, unknowns and surprises in the dynamics of human–water systems. Hydrological Sciences Journal, 61(9), 1748–1758. https://doi.org/10.1080/02626667.2015.1091460
- Díaz, P., Stanek, P., Frantzeskaki, N., & Yeh, D. H. (2016). Shifting paradigms, changing waters: Transitioning to integrated urban water management in the coastal city of Dunedin, USA. Sustainable Cities and Society, 26, 555–567. https://doi.org/10.1016/j.scs.2016.03.016
- Dietz, T., Ostrom, E., & Stern, P. C. (2003). The struggle to govern the commons. Science (New York, N.Y.).), 302(5652), 1907–1912. https://doi.org/10.1126/science.1091015
- Domènech, L., March, H., & Saurí, D. (2013). Degrowth initiatives in the urban water sector? A social multi-criteria evaluation of non-conventional water alternatives in Metropolitan Barcelona. Journal of Cleaner Production, 38, 44–55. https://doi.org/10.1016/j.jclepro.2011.09.020
- Doty, D. H., & Glick, W. H. (1994). Typologies as a unique form of theory building: Toward improved understanding and modeling. The Academy of Management Review, 19(2), 230–251. https://doi.org/10.2307/258704
- Du Plessis, C., & Brandon, P. (2015). An ecological worldview as basis for a regenerative sustainability paradigm for the built environment. Journal of Cleaner Production, 109, 53–61. https://doi.org/10.1016/j.jclepro.2014.09.098
- Dunn, G., Brown, R. R., Bos, J. J., & Bakker, K. (2017). Standing on the shoulders of giants: Understanding changes in urban water practice through the lens of complexity science. Urban Water Journal, 14(7), 758–767. https://doi.org/10.1080/1573062X.2016.1241284
- Dunn, G., Brown, R. R., Bos, J. J., & Bakker, K. (2017). The role of science-policy interface in sustainable urban water transitions: Lessons from Rotterdam. Environmental Science & Policy, 73, 71–79. https://doi.org/10.1016/j.envsci.2017.04.013
- Esmail, B. A., & Suleiman, L. (2020). Analyzing evidence of sustainable urban water management systems: A review through the lenses of sociotechnical transitions. Sustainability, 12(11), 4481. https://doi.org/10.3390/su12114481
- European Commission (EC). (2015). Ecological flows in the implementation of the Water Framework Directive. Technical Report - 2015 - 086.
- Evans, W. R., & Evans, R. (2012). Conjunctive use and management of groundwater and surface water. In Groundwater governance: A global framework for country action. Global Environment Facility (GEF).
- Everard, M., & McInnes, R. (2013). Systemic solutions for multi-benefit water and environmental management. The Science of the Total Environment, 461-462, 170–179. https://doi.org/10.1016/j.scitotenv.2013.05.010
- Fane, S. A. (2005). Planning for sustainable urban water: System approaches and distributed strategies [Doctoral dissertation].
- Farrelly, M. A., & Brown, R. R. (2011). Rethinking urban water management: Experimentation as a way forward. Global Environmental Change, 21(2), 721–732. ? https://doi.org/10.1016/j.gloenvcha.2011.01.007
- Ferguson, B. C., Brown, R. R., & Deletic, A. (2013). Diagnosing transformative change in urban water systems: Theories and frameworks. Global Environmental Change, 23(1), 264–280. https://doi.org/10.1016/j.gloenvcha.2012.07.008
- Ferguson, B. C., Brown, R. R., Frantzeskaki, N., de Haan, F. J., & Deletic, A. (2013). The enabling institutional context for integrated water management: Lessons from Melbourne. Water Research, 47(20), 7300–7314. https://doi.org/10.1016/j.watres.2013.09.045
- Filazzola, A., Shrestha, N., & MacIvor, J. S. (2019). The contribution of constructed green infrastructure to urban biodiversity: A synthesis and meta-analysis. Journal of Applied Ecology, 56(9), 2131–2143. https://doi.org/10.1111/1365-2664.13475
- Fletcher, T. D., Shuster, W., Hunt, W. F., Ashley, R. M., Butler, D., Arthur, S., Trowsdale, S., Barraud, S., Semadeni-Davies, A., Bertrand-Krajewski, J.-L., Mikkelsen, P. S., Rivard, G., Uhl, M., Dagenais, D., & Viklander, M. (2015). SUDS, LID, BMPs, WSUD and more – The evolution and application of terminology surrounding urban drainage. Urban Water Journal, 12(7), 525–542. https://doi.org/10.1080/1573062X.2014.916314
- Folke, C. (2006). Resilience: The emergence of a perspective for social-ecological systems analyses. Global Environmental Change, 16(3), 253–267. https://doi.org/10.1016/j.gloenvcha.2006.04.002
- Franco-Torres, M. (2020). The path to the new urban water paradigm - from modernity to metamodernism. Manuscript submitted for publication.
- Franco-Torres, M., Rogers, B., & Ugarelli, R. (2020). A framework to explain the role of boundary objects in sustainability transitions. Environmental Innovation and Societal Transitions, 36, 34–48. https://doi.org/10.1016/j.eist.2020.04.010Get https://doi.org/10.1016/j.eist.2020.04.010
- Fryd, O., Jensen, M. B., Ingvertsen, S. T., Jeppesen, J., & Magid, J. (2010). Doing the first loop of planning for sustainable urban drainage system retrofits: A case study from Odense. Urban Water Journal, 7(6), 367–378. https://doi.org/10.1080/1573062X.2010.527352
- Funtowicz, S. O., & Ravetz, J. R. (1993). The emergence of post-normal science. In R. von Schomberg (Ed.), Science, politics and morality: Scientific uncertainty and decision making (pp. 85–123). Springer. https://doi.org/10.1007/978-94-015-8143-1_6
- Furlong, C., Guthrie, L., de Silva, S., & Considine, R. (2015). Analysing the terminology of integration in the water management field. Water Policy, 17(1), 46–60. https://doi.org/10.2166/wp.2014.185
- Garrote, L. (2017). Managing water resources to adapt to climate change: facing uncertainty and scarcity in a changing context. Water Resources Management, 31(10), 2951–2963. https://doi.org/10.1007/s11269-017-1714-6
- Geels, F. W., Sovacool, B. K., Schwanen, T., & Sorrell, S. (2017). The socio-technical dynamics of low-carbon transitions. Joule, 1(3), 463–479. https://doi.org/10.1016/j.joule.2017.09.018
- Georgakakos, A. P., Yao, H., Kistenmacher, M., Georgakakos, K. P., Graham, N. E., Cheng, F.-Y., Spencer, C., & Shamir, E. (2012). Value of adaptive water resources management in Northern California under climatic variability and change: Reservoir management. Journal of Hydrology, 412-413, 34–46. https://doi.org/10.1016/j.jhydrol.2011.04.038
- Gerlak, A. K. (2008). Today’s pragmatic water policy: restoration, collaboration, and adaptive management along U.S. Rivers. Society & Natural Resources, 21(6), 538–545. https://doi.org/10.1080/08941920801907514
- Gikas, P., & Tchobanoglous, G. (2009). The role of satellite and decentralized strategies in water resources management. Journal of Environmental Management, 90(1), 144–152. https://doi.org/10.1016/j.jenvman.2007.08.016
- Gill, S. E., Handley, J. F., Ennos, A. R., & Pauleit, S. (2007). Adapting cities for climate change: The role of the green infrastructure. Built Environment, 33(1), 115–133. https://doi.org/10.2148/benv.33.1.115
- Gleick, P. H. (2000). The changing water paradigm: A look at twenty- first century water resources development. Water International, 25(1), 127–138. https://doi.org/10.1080/02508060008686804
- Gleick, P. H. (2003). Global freshwater resources: Soft-path solutions for the 21st century. Science (New York, N.Y.), 302(5650), 1524–1528. https://doi.org/10.1126/science.1089967
- Global Water Partnership (GWP). (2000). Integrated water resources management. TAC Background Paper No. 4.
- Global Water Partnership (GWP). (2012). Integrated urban water management. Technical Committee (TEC). Background Papers. No. 16.
- Gober, P. (2010). Desert urbanization and the challenges of water sustainability. Current Opinion in Environmental Sustainability, 2(3), 144–150. https://doi.org/10.1016/j.cosust.2010.06.006
- Gondhalekar, D., & Ramsauer, T. (2017). Nexus City: Operationalizing the urban Water-Energy-Food Nexus For Climate Change Adaptation In Munich, Germany. Urban Climate, 19, 28–40. https://doi.org/10.1016/j.uclim.2016.11.004
- Gonzales, P., & Ajami, N. K. (2015). Urban water sustainability: An integrative framework for regional water management. Hydrology and Earth System Sciences Discussions, 12(11), 11291–11329. https://doi.org/10.5194/hessd-12-11291-2015
- Gonzales, P., & Ajami, N. K. (2017). An integrative regional resilience framework for the changing urban water paradigm. Sustainable Cities and Society, 30(Supplement C), 128–138. https://doi.org/10.1016/j.scs.2017.01.012
- Goonetilleke, A., Thomas, E., Ginn, S., & Gilbert, D. (2005). Understanding the role of land use in urban stormwater quality management. Journal of Environmental Management, 74(1), 31–42. https://doi.org/10.1016/j.jenvman.2004.08.006
- Grant, S. B., Saphores, J.-D., Feldman, D. L., Hamilton, A. J., Fletcher, T. D., Cook, P. L. M., Stewardson, M., Sanders, B. F., Levin, L. A., Ambrose, R. F., Deletic, A., Brown, R., Jiang, S. C., Rosso, D., Cooper, W. J., & Marusic, I. (2012). Taking the “Waste” Out of “Wastewater” for Human Water Security and Ecosystem sustainability. Science (New York, N.Y.).), 337(6095), 681–686. https://doi.org/10.1126/science.1216852
- Grigg, N. S. (1998). A new paradigm for water management. Simpósio Internacional Sobre Gestão de Recursos Hídricos.
- Guba, E. G. (1990). The paradigm dialog. Sage publications.
- Gunderson, L. H. (1999). Resilience, flexibility and adaptive management – – Antidotes for spurious certitude? Conservation Ecology, 3(1) https://doi.org/10.5751/ES-00089-030107
- Gunderson, L. H., & Holling, C. S. (2002). Resilience and adaptive cycles. In L. H. Gunderson & C. S. Holling (Eds.), Panarchy, understanding transformations in human and natural systems (pp. 25–62). Island Press.
- Haase, D. (2015). Reflections about blue ecosystem services in cities. Sustainability of Water Quality and Ecology, 5, 77–83. https://doi.org/10.1016/j.swaqe.2015.02.003
- Hahn, T., Olsson, P., Folke, C., & Johansson, K. (2006). Trust-building, knowledge generation and organizational innovations: The role of a bridging organization for adaptive comanagement of a wetland landscape around Kristianstad, Sweden. Human Ecology, 34(4), 573–592. https://doi.org/10.1007/s10745-006-9035-z
- Hale, L. R. (2016). Spatial and temporal variation in local stormwater infrastructure use and stormwater management paradigms over the 20th century. Water, 8(7), 310. https://doi.org/10.3390/w8070310
- Hardi, P. (1997). Assessing sustainable development: Principles in practice. International Institute for Sustainable Development Winnipeg.
- Hardy, M. J., Kuczera, G., & Coombes, P. J. (2005). Integrated urban water cycle management: The UrbanCycle model. Water Science and Technology : A Journal of the International Association on Water Pollution Research, 52(9), 1–9. https://doi.org/10.2166/wst.2005.0276
- Harremoës, P. (2002). Integrated urban drainage, status and perspectives. Water Science & Technology, 45(3), 1 LP–10. https://doi.org/10.2166/wst.2002.0041
- Hashimoto, T., Stedinger, J. R., & Loucks, D. P. (1982). Reliability, resiliency, and vulnerability criteria for water resource system performance evaluation. Water Resources Research, 18(1), 14–20. https://doi.org/10.1029/WR018i001p00014
- Hellström, D., Jeppsson, U., & Kärrman, E. (2000). A framework for systems analysis of sustainable urban water management. Environmental Impact Assessment Review, 20(3), 311–321. https://doi.org/10.1016/S0195-9255(00)00043-3
- Hemmes, K., Luimes, P., Giesen, A., Hammenga, A., Aravind, P. v., & Spanjers, H. (2011). Ammonium and phosphate recovery from wastewater to produce energy in a fuel cell. Water Practice and Technology, 6(4) https://doi.org/10.2166/wpt.2011.0071
- Hering, J. G., Waite, T. D., Luthy, R. G., Drewes, J. E., & Sedlak, D. L. (2013). A changing framework for urban water systems. Environmental Science & Technology, 47(19), 10721–10726. https://doi.org/10.1021/es4007096
- Herrfahrdt-Pahle, E., & Pahl-Wostl, C. (2012). Continuity and change in social-ecological systems: The role of institutional resilience. Ecology and Society, 17(2) https://doi.org/10.5751/ES-04565-170208
- Heylighen, F. (1999). The growth of structural and functional complexity during evolution. In F. Heylighen, J. Bollen, & A. Riegler (Eds.), The evolution of complexity (pp. 17–44). Kluwer Academic.
- Heylighen, F., Cilliers, P., & Gershenson, C. (2007). Complexity and philosophy. In J. Bogg & E. Geyer (Eds.), Complexity, science and society (pp. 117–134). Radcliffe Publishing.
- Hoff, H. (2011). Understanding the Nexus. Background Paper for the Bonn 2011 Conference: The Water, Energy and Food Security Nexus. Stockholm Environment Institute.
- Holling, C. S. (1973). Resilience and stability of ecological systems. Annual Review of Ecology and Systematics, 4(1), 1–23. https://doi.org/10.1146/annurev.es.04.110173.000245
- Holling, C. S., & Meffe, G. K. (1996). Command and control and the pathology of natural resource management. Conservation Biology, 10(2), 328–337. https://doi.org/10.1046/j.1523-1739.1996.10020328.x
- Hukka, J. J., & Katko, T. S. (2015). Resilient asset management and governance for deteriorating water services infrastructure. Procedia Economics and Finance, 21, 112–119. https://doi.org/10.1016/S2212-5671(15)00157-4
- Human, O., & Cilliers, P. (2013). Towards an economy of complexity: Derrida, morin and bataille. Theory, Culture & Society, 30(5), 24–44. https://doi.org/10.1177/0263276413484070
- Ingram, H., & Lejano, R. (2007). Why institutions resist incorporating climate change scenarios into agency practice. International Symposium on New Directions in Urban Water Management, UNESCO, Paris, France, 12–14.
- Ingram, H., & Schneider, A. (1998). Science, democracy, and water policy. Water Resources Update, 113(1), 21–28.
- Interagency Climate Change Adaptation Task Force (ICCATF). (2011). National Action Plan: Priorities for Managing Freshwater Resources in a Changing Climate.
- Ioris, A. (2008). The limits of integrated water resources management: a case study of Brazil’s Paraíba do Sul River Basin. Sustainability: Science, Practice and Policy, 4(2), 4–11. https://doi.org/10.1080/15487733.2008.11908017
- Jacobs, J. (1961). The death and life of Great American Cities. Random House.
- Jameson, S., & Baud, I. (2016). Varieties of knowledge for assembling an urban flood management governance configuration in Chennai, India. Habitat International , 54, 112–123. https://doi.org/10.1016/j.habitatint.2015.12.015
- Jensen, O., & Nair, S. (2019). Integrated urban water management and water security: A Comparison of Singapore and Hong Kong. Water, 11(4), 785. https://doi.org/10.3390/w11040785
- Jiggins, J., van Slobbe, E., & Röling, N. (2007). The organisation of social learning in response to perceptions of crisis in the water sector of The Netherlands. Environmental Science & Policy, 10(6), 526–536. https://doi.org/10.1016/j.envsci.2006.12.006
- Johansen, F., & van den Bosch, S. (2017). The scaling-up of Neighbourhood Care: From experiment towards a transformative movement in healthcare. Futures, 89, 60–73. https://doi.org/10.1016/j.futures.2017.04.004
- Kato, S., & Ahern, J. (2008). Learning by doing’: Adaptive planning as a strategy to address uncertainty in planning. Journal of Environmental Planning and Management, 51(4), 543–559. https://doi.org/10.1080/09640560802117028
- Kauffman, S. (1995). At home in the universe: The search for the laws of self-organization and complexity. Oxford University Press.
- Keath, N. A., & Brown, R. R. (2009). Extreme events: Being prepared for the pitfalls with progressing sustainable urban water management. Water Science and Technology : A Journal of the International Association on Water Pollution Research, 59(7), 1271–1280. https://doi.org/10.2166/wst.2009.136
- Kellert, S. H. (1994). In the wake of chaos: Unpredictable order in dynamical systems. University of Chicago press.
- Kiparsky, M., Sedlak, D. L., Thompson, B. H., & Truffer, B. (2013). The innovation deficit in urban water: The need for an integrated perspective on institutions, organizations, and technology. Environmental Engineering Science, 30(8), 395–408. https://doi.org/10.1089/ees.2012.0427
- Klotz, L., & Horman, M. (2010). Counterfactual analysis of sustainable project delivery processes. Journal of Construction Engineering and Management, 136(5), 595–605. https://doi.org/10.1061/(ASCE)CO.1943-7862.0000148
- Kofman, F., & Senge, P. M. (1993). Communities of commitment: The heart of learning organizations. Organizational Dynamics, 22(2), 5–23. https://doi.org/10.1016/0090-2616(93)90050-B
- Kreuter, M. W., de Rosa, C., Howze, E. H., & Baldwin, G. T. (2004). Understanding Wicked Problems: A Key to Advancing Environmental Health Promotion. Health Education & Behavior: The Official Publication of the Society for Public Health Education, 31(4), 441–454. https://doi.org/10.1177/1090198104265597
- Kurtz, C. F., & Snowden, D. J. (2003). The new dynamics of strategy: Sense-making in a complex-complicated world. IBM Systems Journal, 42(3), 462–483. https://doi.org/10.1147/sj.423.0462
- Larsen, T. A., & Gujer, W. (1997). The concept of sustainable urban water management. Water Science and Technology, 35(9), 3–10. https://doi.org/10.2166/wst.1997.0326
- Larsen, T. A., Udert, K. M., & Lienert, J. (2013). Source separation and decentralization for wastewater management. IWA Publishing.
- Larson, K. L., White, D. D., Gober, P., & Wutich, A. (2015). Decision-making under uncertainty for water sustainability and urban climate change adaptation. Sustainability, 7(11), 14761–14784. https://doi.org/10.3390/su71114761
- Lee, E. J., Criddle, C. S., Bobel, P., & Freyberg, D. L. (2013). Assessing the scale of resource recovery for centralized and satellite wastewater treatment. Environmental Science & Technology, 47(19), 10762–10770. https://doi.org/10.1021/es401011k
- Lee, H., & Tan, T. P. (2016). Singapore’s experience with reclaimed water: NEWater. International Journal of Water Resources Development , 32(4), 611–621. https://doi.org/10.1080/07900627.2015.1120188
- Leigh, N. G., & Lee, H. (2019). Sustainable and resilient urban water systems: The role of decentralization and planning. Sustainability, 11(3), 918. https://doi.org/10.3390/su11030918
- Levi-Strauss, C. (1968). The Savage Mind. The University of Chicago Press.
- Listowski, A., Ngo, H. H., Guo, W. S., Vigneswaran, S., & Palmer, C. G. (2009). Concepts towards a novel integrated assessment methodology of urban water reuse. Desalination and Water Treatment, 11(1-3), 81–92. https://doi.org/10.5004/dwt.2009.846
- Liu, J., Dietz, T., Carpenter, S. R., Alberti, M., Folke, C., Moran, E., Pell, A. N., Deadman, P., Kratz, T., Lubchenco, J., Ostrom, E., Ouyang, Z., Provencher, W., Redman, C. L., Schneider, S. H., & Taylor, W. W. (2007). Complexity of coupled human and natural systems. Science (New York, N.Y.), 317(5844), 1513–1516. https://doi.org/10.1126/science.1144004
- Loorbach, D. A. (2014). To transition!. Governance penarchy in the new transformation. Erasmus University Rotterdam.
- Loorbach, D. A., Frantzeskaki, N., & Avelino, F. (2017). Sustainability transitions research: Transforming science and practice for societal change. Annual Review of Environment and Resources, 42(1), 599–626. https://doi.org/10.1146/annurev-environ-102014-021340
- Loucks, D. P. (2000). Sustainable water resources management. Water International, 25(1), 3–10. https://doi.org/10.1080/02508060008686793
- Lovins, A. B. (1976). Energy strategy: The road not taken? Foreign Affairs, 55(1), 65–96. https://doi.org/10.2307/20039628
- Ludwig, D. (2001). The era of management is over. Ecosystems, 4(8), 758–764. https://doi.org/10.1007/s10021-001-0044-x
- Ludwig, D., Hilborn, R., & Walters, C. (1993). Uncertainty, resource exploitation, and conservation: lessons from history. Ecological Applications : A Publication of the Ecological Society of America, 3(4), 548–549. https://doi.org/10.2307/1942074
- Lyotard, J.-F. (1984). The postmodern condition: A report on knowledge. University of Minnesota Press.
- Ma, X., Xue, X., González-Mejía, A., Garland, J., & Cashdollar, J. (2015). Sustainable water systems for the city of tomorrow—A conceptual framework. Sustainability, 7(9), 12071–12105. ( Issue https://doi.org/10.3390/su70912071
- Makropoulos, C. K., & Butler, D. (2010). Distributed water infrastructure for sustainable communities. Water Resources Management, 24(11), 2795–2816. https://doi.org/10.1007/s11269-010-9580-5
- Makropoulos, C. K., Rozos, E., Tsoukalas, I., Plevri, A., Karakatsanis, G., Karagiannidis, L., Makri, E., Lioumis, C., Noutsopoulos, C., Mamais, D., Rippis, C., & Lytras, E. (2018). Sewer-mining: A water reuse option supporting circular economy, public service provision and entrepreneurship. Journal of Environmental Management, 216, 285–298. https://doi.org/10.1016/j.jenvman.2017.07.026
- Marlow, D. R., Moglia, M., Cook, S., & Beale, D. J. (2013). Towards sustainable urban water management: A critical reassessment. Water Research, 47(20), 7150–7161. https://doi.org/10.1016/j.watres.2013.07.046
- Marsalek, J., & Schreier, H. (2009). Innovation in stormwater management in Canada: The way forward. Water Quality Research Journal, 44(1), v–x. https://doi.org/10.2166/wqrj.2009.001
- Martin, C., Ruperd, Y., & Legret, M. (2007). Urban stormwater drainage management: The development of a multicriteria decision aid approach for best management practices. European Journal of Operational Research, 181(1), 338–349. https://doi.org/10.1016/j.ejor.2006.06.019
- Masi, F., Rizzo, A., & Regelsberger, M. (2018). The role of constructed wetlands in a new circular economy, resource oriented, and ecosystem services paradigm. Journal of Environmental Management, 216, 275–284. https://doi.org/10.1016/j.jenvman.2017.11.086
- Mazzocchi, F. (2016). Complexity, network theory, and the epistemological issue. Kybernetes, 45(7), 1158–1170. https://doi.org/10.1108/K-05-2015-0125
- Meadows, D. H. (1999). Leverage points: Places to intervene in a system. The Sustainability Institute.
- Mebratu, D. (1998). Sustainability and sustainable development: Historical and conceptual review. Environmental Impact Assessment Review, 18(6), 493–520. https://doi.org/10.1016/S0195-9255(98)00019-5
- Meinzen-Dick, R. (2007). Beyond panaceas in water institutions. Proceedings of the National Academy of Sciences of the United States of America, 104(39), 15200–15205. https://doi.org/10.1073/pnas.0702296104
- Merton, R. K., & Barber, E. (2011). The travels and adventures of serendipity: A study in sociological semantics and the sociology of science. Princeton University Press.
- Mguni, P., Herslund, L., & Jensen, M. B. (2015). Green infrastructure for flood-risk management in Dar es Salaam and Copenhagen: Exploring the potential for transitions towards sustainable urban water management. Water Policy, 17(1), 126–142. https://doi.org/10.2166/wp.2014.047
- Mikulecky, D. C. (2001). The emergence of complexity: Science coming of age or science growing old. Computers & Chemistry, 25(4), 341–348. https://doi.org/10.1016/S0097-8485(01)00070-5
- Millennium Ecosystem Assessment (MEA). (2005). Ecosystems and human well-being. Vol.5. Island Press.
- Milly, P. C. D., Betancourt, J., Falkenmark, M., Hirsch, R. M., Kundzewicz, Z. W., Lettenmaier, D. P., & Stouffer, R. J. (2008). Climate change. Stationarity is dead: whither water management? Science (New York, N.Y.), 319(5863), 573–574. https://doi.org/10.1126/science.1151915
- Mitchell, M. (2009). Complexity: A guided tour. Oxford University Press.
- Mitchell, V. G. (2006). Applying integrated urban water management concepts: A review of Australian experience. Environmental Management, 37(5), 589–605. https://doi.org/10.1007/s00267-004-0252-1
- Moberg, F., Galaz, V. (2005). Resilience: Going from conventional to adaptive freshwater management for human and ecosystem compatibility. Swedish Water House Policy Brief Nr 3. http://swedishwaterhouse.se/wp-content/uploads/20050929133815resiliencepolicybrief.pdf
- Molle, F. (2008). Nirvana concepts, narratives and policy models: Insights from the water sector. Water Alternatives, 1(1), 131–156.
- Morcol, G. (2001). What is complexity science? Emergence, 3(1), 104–119. https://doi.org/10.1207/S15327000EM0301_07
- Morin, E. (1977). La Méthode (vol. 1) La nature de la nature. Seuil.
- Morin, E. (2007). Restricted complexity, general complexity. In C. Gershenson, D. Aerts, & B. Edmonds (Eds.), Worldviews, science and us: Philosophy and complexity (pp. 5–29). World Scientific Publishing.
- Mouritz, M. (1996). Sustainable urban water systems: Policy and professional praxis [Doctoral dissertation]. http://researchrepository.murdoch.edu.au/id/eprint/211/
- Mukheibir, P., Howe, C., & Gallet, D. (2014). What’s getting in the way of a One Water approach to water services planning and management? Water: Journal of the Australian Water Association, 41, 67–73.
- Mukhtarov, F. G. (2008). Intellectual history and current status of Integrated Water Resources Management: A global perspective. In C. Pahl-Wostl, P. Kabat, & J. Möltgen (Eds.), Adaptive and integrated water management (pp. 167–185). Springer. https://doi.org/10.1007/978-3-540-75941-6_1
- Neto, S. (2016). Water governance in an urban age. Utilities Policy, 43, 32–41. https://doi.org/10.1016/j.jup.2016.05.004
- Newman, L. (2005). Uncertainty, innovation, and dynamic sustainable development. Sustainability: Science, Practice and Policy, 1(2), 25–31. https://doi.org/10.1080/15487733.2005.11907970
- Niemczynowicz, J. (1999). Urban hydrology and water management – present and future challenges. Urban Water, 1(1), 1–14. https://doi.org/10.1016/S1462-0758(99)00009-6
- Nonaka, I., & Toyama, R. (2005). The theory of the knowledge-creating firm: Subjectivity, objectivity and synthesis. Industrial and Corporate Change, 14(3), 419–436. https://doi.org/10.1093/icc/dth058
- Norton, B. A., Coutts, A. M., Livesley, S. J., Harris, R. J., Hunter, A. M., & Williams, N. S. G. (2015). Planning for cooler cities: A framework to prioritise green infrastructure to mitigate high temperatures in urban landscapes. Landscape and Urban Planning, 134, 127–138. https://doi.org/10.1016/j.landurbplan.2014.10.018
- Novotny, V. (2009). Sustainable urban water management. In J. Feyen, K. Shannon, & M. Neville (Eds.), Water and urban development paradigms - Towards an integration of engineering, design and management approaches (pp. 19–33).
- Novotny, V., Ahern, J., & Brown, P. R. (2010). Water centric sustainable communities: Planning, retrofitting, and building the next urban environment. John Wiley & Sons.
- Organisation for Economic Co-operation and Development (OECD). (2016). Water governance in cities. OECD Publishing. https://doi.org/10.1787/9789264251090-en
- Organisation for Economic Co-operation and Development (OECD). (2011). Water governance in OECD countries: A multi-level approach. OECD Publishing. https://doi.org/10.1787/9789264119284-en
- Organisation for Economic Co-operation and Development (OECD). (2015). Water and cities: Ensuring sustainable futures. (OECD Studies on Water, Ed.). OECD Publishing. https://doi.org/10.1787/9789264230149-en
- Osborne, S. P. (2010). Introduction. The (New) Public Governance: a suitable case for treatment?. In S. P. Osborne (Ed.), The new public governance: Emerging perspectives on the theory and practice of public governance (pp. 1–16). Routledge.
- Pahl-Wostl, C. (2007). The implications of complexity for integrated resources management. Environmental Modelling & Software, 22(5), 561–569. https://doi.org/10.1016/j.envsoft.2005.12.024
- Pahl-Wostl, C. (2008). Requirements for adaptive water management. In C. Pahl-Wostl, P. Kabat, & J. Möltgen (Eds.), Adaptive and integrated water management: Coping with complexity and uncertainty (pp. 1–22). Springer. https://doi.org/10.1007/978-3-540-75941-6_1
- Pahl-Wostl, C. (2015). Water governance in the face of global change - From understanding to transformation. Springer. https://doi.org/10.1007/978-3-319-21855-7
- Pahl-Wostl, C., Jeffrey, P., Isendahl, N., & Brugnach, M. (2011). Maturing the new water management paradigm: Progressing from aspiration to practice. Water Resources Management, 25(3), 837–856. https://doi.org/10.1007/s11269-010-9729-2
- Pahl-Wostl, C., Sendzimir, J., & Jeffrey, P. (2009). Resources management in transition. Ecology and Society, 14(1) https://doi.org/10.5751/ES-02898-140146
- Pahl-Wostl, C., Sendzimir, J., Jeffrey, P., Aerts, J. C. J. H., Berkamp, G., & Cross, K. (2007). Managing change toward adaptive water management through social learning. Ecology and Society, 12(2) https://doi.org/10.5751/ES-02147-120230
- Pahl-Wostl, C., Tàbara, D., Bouwen, R., Craps, M., Dewulf, A., Mostert, E., Ridder, D., & Taillieu, T. (2008). The importance of social learning and culture for sustainable water management. Ecological Economics, 64(3), 484–495. https://doi.org/10.1016/j.ecolecon.2007.08.007
- Pan, H., & Guo, M. (2019). Can participatory water management improve residents’ subjective life quality?. Water Supply, 19(5), 1547–1554. https://doi.org/10.2166/ws.2019.023
- Pappalardo, V., la Rosa, D., Campisano, A., & la Greca, P. (2017). The potential of green infrastructure application in urban runoff control for land use planning: A preliminary evaluation from a southern Italy case study. Ecosystem Services, 26, 345–354. https://doi.org/10.1016/j.ecoser.2017.04.015
- Partzsch, L. (2009). Smart regulation for water innovation – the case of decentralized rainwater technology. Journal of Cleaner Production, 17(11), 985–991. https://doi.org/10.1016/j.jclepro.2009.01.009
- Patterson, J. J., Smith, C., & Bellamy, J. (2013). Understanding enabling capacities for managing the 'wicked problem' of nonpoint source water pollution in catchments: A conceptual framework. Journal of Environmental Management, 128, 441–452. https://doi.org/10.1016/j.jenvman.2013.05.033
- Pennisi, E. (2012). Water reclamation going green. Science (New York, N.Y.), 337(6095), 674–676. https://doi.org/10.1126/science.337.6095.674
- Pierce, C. S. (2011). Philosophical Writings of Peirce. (J. Buchler, Ed.). Dover Publications.
- Pinkham, R. (1999). 21st century water systems: Scenarios, visions, and drivers. EPA Workshop “Sustainable Urban Water Infrastructure: A vision of the future.”
- Pires, M. (2004). Watershed protection for a world city: The case of New York. Land Use Policy, 21(2), 161–175. https://doi.org/10.1016/j.landusepol.2003.08.001
- Porse, C. E. (2013). Stormwater governance and future cities. Water, 5(1), 29–52. https://doi.org/10.3390/w5010029
- Prasad Pandey, V., & Kazama, F. (2014). From an open-access to a state-controlled resource: The case of groundwater in the Kathmandu Valley. Water International, 39(1), 97–112. https://doi.org/10.1080/02508060.2014.863687
- Prigogine, I., & Stengers, I. (1984). Order out of chaos: Man’s new dialogue with nature. Bantam books.
- Prigogine, I., & Stengers, I. (1997). The end of certainty: Time, chaos and the new laws of nature. Free Press.
- Rahaman, M. M., & Varis, O. (2005). Integrated water resources management: Evolution, prospects and future challenges. Sustainability: Science, Practice and Policy, 1(1), 15–21. https://doi.org/10.1080/15487733.2005.11907961
- Reed, P. M., & Kasprzyk, J. (2009). Water resources management: The myth, the wicked, and the future. Journal of Water Resources Planning and Management, 135(6), 411–413. https://doi.org/10.1061/(ASCE)WR.1943-5452.0000047
- Richardson, K., & Cilliers, P. (2001). Special editors’ introduction: What is complexity science? A view from different directions. Emergence, 3(1), 5–23. https://doi.org/10.1207/S15327000EM0301_02
- Rijke, J., Farrelly, M., Brown, R. R., & Zevenbergen, C. (2013). Configuring transformative governance to enhance resilient urban water systems. Environmental Science & Policy, 25(ii), 62–72. https://doi.org/10.1016/j.envsci.2012.09.012
- Rittel, H. W. J., & Webber, M. M. (1973). Dilemmas in a general theory of planning. Policy Sciences, 4(2), 155–169. https://doi.org/10.1007/BF01405730
- Robinson, J., & Cole, R. J. (2015). Theoretical underpinnings of regenerative sustainability. Building Research & Information, 43(2), 133–143. https://doi.org/10.1080/09613218.2014.979082
- Rockström, J., Steffen, W., Noone, K., Persson, Å., Chapin, F. S. I. I., Lambin, E., Lenton, T. M., Scheffer, M., Folke, C., Schellnhuber, H. J., Nykvist, B., de Wit, C. A., Hughes, T., van der Leeuw, S., Rodhe, H., Sörlin, S., Snyder, P. K., Costanza, R., Svedin, U., … Foley, J. (2009). Planetary boundaries: Exploring the safe operating space for humanity. Ecology and Society, 14(2) https://doi.org/10.5751/ES-03180-140232
- Rode, S., & Gralepois, M. (2017). Towards an urban design adapted to flood risk?. In F. Vinet (Ed.), Floods. Volume 2. Risk management (pp. 365–380). Elsevier. https://doi.org/10.1016/B978-1-78548-269-4.50024-X
- Rojas, C., de Meulder, B., & Shannon, K. (2015). Water urbanism in Bogotá. Exploring the potentials of an interplay between settlement patterns and water management. Habitat International, 48, 177–187. https://doi.org/10.1016/j.habitatint.2015.03.017
- Ross, A. (2018). Speeding the transition towards integrated groundwater and surface water management in Australia. Journal of Hydrology, 567, e1–e10. https://doi.org/10.1016/j.jhydrol.2017.01.037
- Roy, A. H., Wenger, S. J., Fletcher, T. D., Walsh, C. J., Ladson, A. R., Shuster, W. D., Thurston, H. W., & Brown, R. R. (2008). Impediments and solutions to sustainable, watershed-scale urban stormwater management: Lessons from Australia and the United States. Environmental Management, 42(2), 344–359. https://doi.org/10.1007/s00267-008-9119-1
- Ryan, C. (2009). Climate change and ecodesign, Part II: Exploring distributed systems. Journal of Industrial Ecology, 13(3), 350–353. https://doi.org/10.1111/j.1530-9290.2009.00133.x
- Rygaard, M., Binning, P. J., & Albrechtsen, H.-J. (2011). Increasing urban water self-sufficiency: New era, new challenges. Journal of Environmental Management, 92(1), 185–194. https://doi.org/10.1016/j.jenvman.2010.09.009
- Rygaard, M., Godskesen, B., Jørgensen, C., & Hoffmann, B. (2014). Holistic assessment of a secondary water supply for a new development in Copenhagen, Denmark. The Science of the Total Environment, 497-498, 430–439. https://doi.org/10.1016/j.scitotenv.2014.07.078
- Sahely, H. R., Kennedy, C. A., & Adams, B. J. (2005). Developing sustainability criteria for urban infrastructure systems. Canadian Journal of Civil Engineering, 32(1), 72–85. https://doi.org/10.1139/l04-072
- Saraswat, C., Mishra, B. K., & Kumar, P. (2017). Integrated urban water management scenario modeling for sustainable water governance in Kathmandu Valley, Nepal. Sustainability Science, 12(6), 1037–1053. https://doi.org/10.1007/s11625-017-0471-z
- Sarewitz, D. (2004). How science makes environmental controversies worse. Environmental Science & Policy, 7(5), 385–403. https://doi.org/10.1016/j.envsci.2004.06.001
- Saurí, D., & del Moral, L. (2001). Recent developments in Spanish water policy. Alternatives and conflicts at the end of the hydraulic age. Geoforum, 32(3), 351–362. https://doi.org/10.1016/S0016-7185(00)00048-8
- Saurí, D., & Palau-Rof, L. (2017). Urban drainage in Barcelona: From hazard to resource?. Water Alternatives, 10(2), 475–492.
- Schelfaut, K., Pannemans, B., van der Craats, I., Krywkow, J., Mysiak, J., & Cools, J. (2011). Bringing flood resilience into practice: The FREEMAN project. Environmental Science & Policy, 14(7), 825–833. https://doi.org/10.1016/j.envsci.2011.02.009
- Schmidt, J. J. (2013). Integrating water management in the anthropocene. Society & Natural Resources, 26(1), 105–112. https://doi.org/10.1080/08941920.2012.685146
- Schmidt, M. (2010). Ecological design for climate mitigation in contemporary urban living. International Journal of Water, 5(4), 337–352. https://doi.org/10.1504/IJW.2010.038727
- Schmitt, T. G., & Huber, W. C. (2006). The scope of integrated modelling: System boundaries, sub-systems, scales and disciplines. Water Science and Technology: A Journal of the International Association on Water Pollution Research, 54(6-7), 405–413. https://doi.org/10.2166/wst.2006.595
- Schoeman, J., Allan, C., & Finlayson, C. M. (2014). A new paradigm for water? A comparative review of integrated, adaptive and ecosystem-based water management in the Anthropocene. International Journal of Water Resources Development, 30(3), 377–390. https://doi.org/10.1080/07900627.2014.907087
- Schoonenboom, I. J. (1995). Overview and state of the art of scenario studies for the rural environment. Scenario Studies for the Rural Environment: Selected and Edited Proceedings of the Symposium" Scenario Studies for the Rural Environment," Wageningen, The Netherlands, 12-15 September 1994, 15.
- Schuch, G., Serrao-Neumann, S., Morgan, E., & Low Choy, D. (2017). Water in the city: Green open spaces, land use planning and flood management – An Australian case study. Land Use Policy, 63, 539–550. https://doi.org/10.1016/j.landusepol.2017.01.042
- Schumacher, E. F. (1973). Small is beautiful. Harper & Row.
- Segrave, A. J., van der Zouwen, M. W., & van Vierssen, W. (2014). Water planning: From what Time Perspective?. Technological Forecasting and Social Change, 86(Supplement C), 157–167. https://doi.org/10.1016/j.techfore.2013.08.019
- Semadeni-Davies, A., Hernebring, C., Svensson, G., & Gustafsson, L.-G. (2008). The impacts of climate change and urbanisation on drainage in Helsingborg, Sweden: Combined sewer system. Journal of Hydrology, 350(1-2), 100–113. https://doi.org/10.1016/j.jhydrol.2007.05.028
- Sharma, A. K., Burn, S., Gardner, T., & Gregory, A. (2010). Role of decentralised systems in the transition of urban water systems. Water Supply, 10(4), 577–583. https://doi.org/10.2166/ws.2010.187
- Sharma, A. K., Tjandraatmadja, G., Cook, S., & Gardner, T. (2013). Decentralised systems - definition and drivers in the current context. Water Science and Technology: A Journal of the International Association on Water Pollution Research, 67(9), 2091–2101. https://doi.org/10.2166/wst.2013.093
- Simmie, J., & Martin, R. (2010). The economic resilience of regions: Towards an evolutionary approach. Cambridge Journal of Regions, Economy and Society, 3(1), 27–43. https://doi.org/10.1093/cjres/rsp029
- Simon, H. A. (1997). Administrative behavior. (4th ed.). Free Press.
- Slocombe, D. S. (1990). Assessing transformation and sustainability in the Great Lakes Basin. GeoJournal, 21(3), 251–272. https://doi.org/10.1007/BF02428510
- Sofoulis, Z. (2005). Big Water, Everyday Water: A Sociotechnical Perspective. Continuum, 19(4), 445–463. https://doi.org/10.1080/10304310500322685
- Sofoulis, Z. (2015). The trouble with tanks: Unsettling dominant Australian urban water management paradigms. Local Environment, 20(5), 529–547. https://doi.org/10.1080/13549839.2014.903912
- Steffen, W., Richardson, K., Rockström, J., Cornell, S. E., Fetzer, I., Bennett, E. M., Biggs, R., Carpenter, S. R., de Vries, W., de Wit, C. A., Folke, C., Gerten, D., Heinke, J., Mace, G. M., Persson, L. M., Ramanathan, V., Reyers, B., & Sörlin, S. (2015). Sustainability. Planetary boundaries: guiding human development on a changing planet. Science (New York, N.Y.).), 347(6223), 1259855. https://doi.org/10.1126/science.1259855
- Stuart, N. (2007). Technology and epistemology: Environmental mentalities and urban water usage. Environmental Values, 16(4), 417–431. https://doi.org/10.3197/096327107X243213
- Swyngedouw, E. (1999). Modernity and hybridity: Nature, regeneracionismo, and the production of the spanish waterscape, 1890–1930. Annals of the Association of American Geographers, 89(3), 443–465. https://doi.org/10.1111/0004-5608.00157
- Takala, A. (2017). Understanding sustainable development in Finnish water supply and sanitation services. International Journal of Sustainable Built Environment, 6(2), 501–512. https://doi.org/10.1016/j.ijsbe.2017.10.002
- Torfing, J., Peters, B. G., Pierre, J., & Sørensen, E. (2012). Interactive governance: Advancing the paradigm. Oxford University Press.
- Truffer, B., Störmer, E., Maurer, M., & Ruef, A. (2010). Local strategic planning processes and sustainability transitions in infrastructure sectors. Environmental Policy and Governance, 20(4), 258–269. https://doi.org/10.1002/eet.550
- Turton, A. R., & Meissner, R. (2002). The hydrosocial contract and its manifestation in society: A South African case study. In A. R. Turton & R. Henwood (Eds.), Hydropolitics in the developing world: A southern African perspective (pp. 37–60). African Water Issues Research Unit (AWIRU).
- Tzoulas, K., Korpela, K., Venn, S., Yli-Pelkonen, V., Kaźmierczak, A., Niemela, J., & James, P. (2007). Promoting ecosystem and human health in urban areas using Green Infrastructure: A literature review. Landscape and Urban Planning, 81(3), 167–178. https://doi.org/10.1016/j.landurbplan.2007.02.001
- United Nations (UN). (2007). Indicators of sustainable development: Guidelines and methodologies.
- United Nations (UN). (2015). Transforming our World: The 2030 Agenda for Sustainable Development.
- United Nations Environment Program (UNEP). (2011). Towards a green economy: Pathways to sustainable development and poverty eradication - A synthesis for policy makers. www.unep.org/greeneconomy
- United Nations World Water Assessment Programme (WWAP). (2017). The United Nations World Water Development Report 2017. Wastewater: The Untapped Resource. UNESCO. https://doi.org/10.29104/wins.d.0002.2018
- United Nations World Water Assessment Programme (WWAP)/UN-Water (2018). The United Nations World Water Development report 2018: Nature-based solutions for water. UNESCO. https://doi.org/10.18356/dc00fb4d-en
- Vairavamoorthy, K., Eckart, J., Tsegaye, S., Ghebremichael, K., & Khatri, K. (2015). A paradigm shift in urban water management: An imperative to achieve sustainability. In S. G. Setegn & M. C. Donoso (Eds.), Sustainability of integrated water resources management: Water governance, climate and ecohydrology (pp. 51–64). Springer International Publishing. https://doi.org/10.1007/978-3-319-12194-9_4
- van de Meene, S. J., Brown, R. R., & Farrelly, M. A. (2011). Towards understanding governance for sustainable urban water management. Global Environmental Change, 21(3), 1117–1127. https://doi.org/10.1016/j.gloenvcha.2011.04.003
- van der Brugge, R. (2009). Transition dynamics in social-ecological systems: The case of Dutch water management [Doctoral dissertation].
- van der Hoek, J. P., de Fooij, H., & Struker, A. (2016). Wastewater as a resource: Strategies to recover resources from Amsterdam’s wastewater. Resources, Conservation and Recycling, 113, 53–64. https://doi.org/10.1016/j.resconrec.2016.05.012
- van der Steen, P., & Howe, C. (2009). Managing water in the city of the future; strategic planning and science. Reviews in Environmental Science and Bio/Technology, 8(2), 115–120. https://doi.org/10.1007/s11157-009-9154-2
- van Dijk, M. P. (2012). Introduction. International Journal of Water, 6(3/4), 137–154. https://doi.org/10.1504/IJW.2012.049493
- van Leeuwen, C. J. (2013). City blueprints: Baseline assessments of sustainable water management in 11 cities of the future. Water Resources Management, 27(15), 5191–5206. https://doi.org/10.1007/s11269-013-0462-5
- van Zeijl-Rozema, A., Cörvers, R., Kemp, R., & Martens, P. (2008). Governance for sustainable development: a framework. Sustainable Development, 16(6), 410–421. https://doi.org/10.1002/sd.367
- Varady, R. G., Zuniga-Teran, A. A., Garfin, G. M., Martín, F., & Vicuña, S. (2016). Adaptive management and water security in a global context: Definitions, concepts, and examples. Current Opinion in Environmental Sustainability, 21, 70–77. https://doi.org/10.1016/j.cosust.2016.11.001
- Verbong, G. P. J., & Geels, F. W. (2010). Exploring sustainability transitions in the electricity sector with socio-technical pathways. Technological Forecasting and Social Change, 77(8), 1214–1221. https://doi.org/10.1016/j.techfore.2010.04.008
- Vieira, A. S., Beal, C. D., Ghisi, E., & Stewart, R. A. (2014). Energy intensity of rainwater harvesting systems: A review. Renewable and Sustainable Energy Reviews, 34, 225–242. https://doi.org/10.1016/j.rser.2014.03.012
- von Bertalanffy, L. (1968). General system theory. Foundations, development, applications. George Braziller.
- von Foerster, H. (2002). Understanding understanding: Essays on cybernetics and cognition. Springer.
- Voß, J.-P., & Kemp, R. (2006). Sustainability and reflexive governance: Introduction. In J.-P. Voß, D. Bauknecht, & R. Kemp (Eds.), Reflexive governance for sustainable development (pp. 3–30).: Edward Elgar. https://doi.org/10.4337/9781847200266.00009
- Voulvoulis, N., Arpon, K. D., & Giakoumis, T. (2017). The EU water framework directive: From great expectations to problems with implementation. The Science of the Total Environment, 575, 358–366. https://doi.org/10.1016/j.scitotenv.2016.09.228
- Vreugdenhil, H., Slinger, J., Thissen, W., & Rault, P. K. (2010). Pilot projects in water management. Ecology and Society, 15(3) https://doi.org/10.5751/ES-03357-150313
- Wade, J. S. (2012). The future of urban water services in Latin America. Bulletin of Latin American Research, 31(2), 207–221. https://doi.org/10.1111/j.1470-9856.2011.00603.x
- Waldrop, M. M. (1993). Complexity: The emerging science at the edge of order and chaos. Simon and Schuster.
- Walker, B., Holling, C. S., Carpenter, S. R., & Kinzig, A. (2004). Resilience, adaptability and transformability in social–ecological systems. Ecology and Society, 9(2), 5. https://doi.org/10.5751/ES-00650-090205
- Wallace, T., Gibbons, D., O'Dwyer, M., & Curran, T. P. (2017). International evolution of fat, oil and grease (FOG) waste management - A review. Journal of Environmental Management, 187, 424–435. https://doi.org/10.1016/j.jenvman.2016.11.003
- Weinberg, A. M. (1972). Science and trans-science. Minerva, 10(2), 209–222. https://doi.org/10.1007/BF01682418
- Werbeloff, L., & Brown, R. R. (2011). Working towards sustainable urban water management: The vulnerability blind spot. Water Science and Technology: A Journal of the International Association on Water Pollution Research, 64(12), 2362–2369. https://doi.org/10.2166/wst.2011.774
- Westley, F., Olsson, P., Folke, C., Homer-Dixon, T., Vredenburg, H., Loorbach, D. A., Thompson, J., Nilsson, M., Lambin, E., Sendzimir, J., Banerjee, B., Galaz, V., & van der Leeuw, S. (2011). Tipping toward sustainability: emerging pathways of transformation. Ambio, 40(7), 762–780. https://doi.org/10.1007/s13280-011-0186-9
- Wilcox, J., Nasiri, F., Bell, S., & Rahaman, M. S. (2016). Urban water reuse: A triple bottom line assessment framework and review. Sustainable Cities and Society, 27, 448–456. https://doi.org/10.1016/j.scs.2016.06.021
- Wolfe, S. E., & Brooks, D. B. (2017). Mortality awareness and water decisions: a social psychological analysis of supply-management, demand-management and soft-path paradigms. Water International, 42(1), 1–17. https://doi.org/10.1080/02508060.2016.1248093
- Wolsink, M. (2006). River basin approach and integrated water management: Governance pitfalls for the Dutch Space-Water-Adjustment Management Principle. Geoforum, 37(4), 473–487. https://doi.org/10.1016/j.geoforum.2005.07.001
- Wolsink, M. (2010). Contested environmental policy infrastructure: Socio-political acceptance of renewable energy, water, and waste facilities. Environmental Impact Assessment Review, 30(5), 302–311. https://doi.org/10.1016/j.eiar.2010.01.001
- Wong, T. (2006). An Overview of Water Sensitive Urban Design Practices in Australia. Water Practice and Technology, 1(1). https://doi.org/10.2166/wpt.2006.018
- Wong, T., & Brown, R. R. (2008). Transitioning to water sensitive cities: Ensuring resilience through a new hydro-social contract [Paper presentation]. 11th International Conference on Urban Drainage. September. Edinburgh,. 10p.
- Wong, T., & Brown, R. R. (2009). The water sensitive city: Principles for practice. Water Science and Technology : A Journal of the International Association on Water Pollution Research, 60(3), 673–682. https://doi.org/10.2166/wst.2009.436
- Yarime, M., Trencher, G., Mino, T., Scholz, R. W., Olsson, L., Ness, B., Frantzeskaki, N., & Rotmans, J. (2012). Establishing sustainability science in higher education institutions: Towards an integration of academic development, institutionalization, and stakeholder collaborations. Sustainability Science, 7(S1), 101–113. https://doi.org/10.1007/s11625-012-0157-5
- Yuan, Z., Olsson, G., Cardell-Oliver, R., van Schagen, K., Marchi, A., Deletic, A., Urich, C., Rauch, W., Liu, Y., & Jiang, G. (2019). Sweating the assets - The role of instrumentation, control and automation in urban water systems. Water Research, 155, 381–402. https://doi.org/10.1016/j.watres.2019.02.034
- Zandaryaa, S., & Tejada-Guibert, J. A. (2009). New directions in urban water management. In J. Feyen, K. Shannon, & M. Neville (Eds.), Water and urban development paradigms: Towards an integration of engineering, design and management approaches - Proceedings of the International Urban Water Conference. Heverlee, Belgium, 15-19 September, 2008 (pp. 513–518). Taylor & Francis Group. https://doi.org/10.1201/9780203884102.ch66
- Zhang, D., Gersberg, R. M., Wilhelm, C., & Voigt, M. (2009). Decentralized water management: Rainwater harvesting and greywater reuse in an urban area of Beijing. Urban Water Journal, 6(5), 375–385. https://doi.org/10.1080/15730620902934827
- Zhou, Q. (2014). A review of sustainable urban drainage systems considering the climate change and urbanization impacts. Water, 6(4), 976–992. https://doi.org/10.3390/w6040976
- Ziersen, J., Clauson-Kaas, J., & Rasmussen, J. (2017). The role of Greater Copenhagen Utility in implementing the city’s. Water Practice and Technology, 12(2), 338–343. https://doi.org/10.2166/wpt.2017.039
- Zwarteveen, M. Z., & Boelens, R. (2014). Defining, researching and struggling for water justice: Some conceptual building blocks for research and action. Water International, 39(2), 143–158. https://doi.org/10.1080/02508060.2014.891168