Abstract
Tumor suppressor genes are highly affected during the development of leukemia, including circadian clock genes. Circadian rhythms constitute an evolutionary molecular machinery involving many genes, such as BMAL1, CLOCK, CRY1, CRY2, PER1, PER2, REV-ERBa, and RORA, for tracking time and optimizing daily life during day-night cycles and seasonal changes. For circulating blood cells many of these genes coordinate their proliferation, output, and function, and therein lies their importance for the development of leukemia. Recent findings suggest that environmental pollutants may affect circadian rhythms and thus affect cancer development and treatment. Such environmental pollutants are often found in mixtures and include benzene, tobacco smoke, pesticides and microplastics. Our understanding of the molecular basis for the interaction mechanisms within complex mixtures is also growing, confirming the plausible occurrence of synergistic (superadditive effect) and antagonistic (cancellation effect) actions of pollutant cocktails. In this work, we discuss the relationship of environmental pollutants and the alteration of circadian rhythms that potentially may cause leukemia. We highlight the need of additional dimensions and perhaps a paradigm shift for future studies in relation to continuously growing magnitude of environmental pollution using multitude of disciplines such as development of high throughput reporter cell lines, other cell screening methods, contaminant measurements in leukemia patients, advanced pharmacology and toxicological measurement of mixtures and highly efficient computer analysis including artificial intelligence, among others.
Graphical Abstract
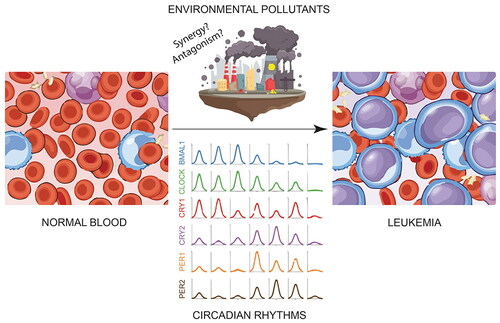
Keywords:
HANDLING EDITORS:
1. Introduction
Leukemia is the common name for a group of heterogeneous malignant disorders that are caused by rapid production of abnormal cells in blood-forming tissues such as the bone marrow and lymphatic system (Lagunas-Rangel & Chávez-Valencia, Citation2017). There are several types of leukemia, which are mainly divided according to whether the leukemia is acute (fast growing) or chronic (slower growing) and whether it begins in myeloid cells (cells that give rise to erythrocytes, platelets, monocytes, neutrophils, basophils, and eosinophils) or lymphoid cells (cells that give rise to T lymphocytes, B lymphocytes and NK cells) (Lagunas-Rangel et al., Citation2017). Acute leukemias are characterized by a rapid increase in abnormal and immature white blood cells, crowding out normal blood cells from the bone marrow, while chronic leukemias are characterized by a relatively slow accumulation of abnormal, mostly mature blood cells (Arber et al., Citation2016; Swerdlow et al., Citation2016).
Approximately 350,000 people worldwide are diagnosed with leukemia each year and 250,000 people die from it. Acute forms of leukemia represent 44% of cases, while chronic forms represent 43%, and with 13% as indeterminate forms (). Myeloid leukemias correspond to 46% of the total, while lymphocytic and lymphoblastic leukemias represent 47%, and 7% are nonspecific (). Of the total leukemias, 13% are chronic myeloid leukemias (CMLs), 28% are acute myeloid leukemias (AMLs), 30% are chronic lymphocytic leukemias (CLLs), 13% are acute lymphoblastic leukemias (ALLs) and 16% remaining are other types of leukemia () (Elert, Citation2013; Sung et al., Citation2021). Leukemias can occur at all life ages, from a newborn to an older person, but the different forms have very different age distributions (). Notably, leukemia is the most common type of childhood cancer, representing approximately one third of pediatric cancers (children younger than 15 years). ALL is common in early childhood and rare in adults, accounting for nearly a quarter of all childhood cancers, while AML is less common in children, but it is common in older adults. Indeed, ALL occurs five times more frequently in children than AML, with B-acute lymphoblastic leukemia (B-ALL) being the most prevalent and having high mortality rates (Whitehead et al., Citation2016). CML and CLL are rare in young children, but their incidence increases in adulthood, peaking between the ages of 75 and 79. Overall, adult leukemias tend to have a worse prognosis than childhood leukemias (Elert, Citation2013; Sung et al., Citation2021).
Figure 1. Distribution of the different types of leukemias. The percentages of the main types of leukemia are shown according to their acute or chronic character (A), the predominant cell type (B), their classification (C) and according to the ages at which they occur (D). The graphs are based on data taken from Elert (Citation2013).
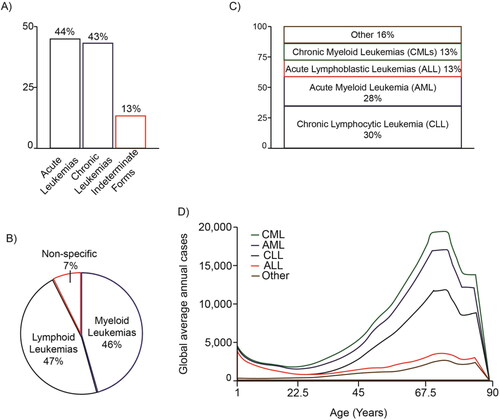
Leukemia has a multifactorial origin, where different genetic, nutritional and environmental conditions called risk factors act together to promote its development. Several risk factors of different etiologies have been associated with the development of leukemia and each type of leukemia has a predilection for certain types of factors. These include genetic syndromes, including Down syndrome, Bloom syndrome, Fanconi anemia, ataxia telangiectasia and neurofibromatosis, all of which increase the risk of childhood ALL and AML (Belson et al., Citation2007; Davis et al., Citation2014). People exposed to ionizing radiation, either accidentally such as survivors of an atomic bomb explosion, or those exposed through work-related causes, are at increased risk of developing ALL, AML, and CML (Belson et al., Citation2007; Buffler et al., Citation2005; Davis et al., Citation2014). A history of hematological malignancies is also considered a risk factor since these can progress or lead to the subsequent development of leukemias (Belson et al., Citation2007). Occupational and environmental exposure to benzene (a chemical used in the manufacture of paints and plastics, as well as being released by incomplete combustion of fossil fuels) is an established risk factor for leukemia, particularly adult AML (Belson et al., Citation2007; Buffler et al., Citation2005; Davis et al., Citation2014; Vinceti et al., Citation2012). There are contrasting reports on the role of parental smoking in the development of childhood leukemia, but there is growing evidence of a relationship between smoking and susceptibility to developing AML, although it is not yet conclusive (Belson et al., Citation2007; Buffler et al., Citation2005). Exposure to pesticides in the early stages of development, such as in utero and in the first three years of life, has been associated with an increased risk of childhood ALL. Likewise, an increased risk of developing childhood leukemia has been linked to greater exposure to air pollutants, particularly particulate matter with diameters of 10 microns (PM10) and 1,3-butadiene (Filippini et al., Citation2019; Vinceti et al., Citation2012). Meanwhile, Epstein-Barr virus positive mothers were associated with a significantly higher risk of ALL in their children (Belson et al., Citation2007; Buffler et al., Citation2005; Davis et al., Citation2014). Obesity has also been linked to an increased risk for developing leukemia and with circadian desynchrony (Davis et al., Citation2014; Laermans & Depoortere, Citation2016).
Environmental pollutants have been shown to actively participate in the development of some types of cancer (Boffetta, Citation2006; Koual et al., Citation2020), and so far, their activity has mainly been investigated individually, although reports have begun to appear where the effects of pollutant cocktails are analyzed (Hansen et al., Citation2019; Ngamwong et al., Citation2015; Q. Wang et al., Citation2020). There is increasing awareness in the modern societies that living beings are simultaneously exposed to an increasing number of environmental pollutants and their transformation products and metabolites. Most often the levels of these pollutants are below the acute toxicity reference values but there is growing concern that these compounds act and interact within the human body especially if considering chronic and multigeneration approach (Cedergreen, Citation2014). Synergy occurs when the interaction between two or more factors makes the total effect greater than the sum of the individual effects of each, while additivity can describe a situation in which the overall effect caused by a combination of compounds is the sum of the effects of each individual agent. In contrast, antagonism occurs between two or more compounds that have opposite effects and, therefore, their effects are weaker than those of each individual agent (Geary, Citation2013; Niu et al., Citation2019; Tang et al., Citation2015). Synergistic effects, or true over-additive effects, are often observed in experimental and clinical studies of a wide variety of compounds (Cedergreen, Citation2014; Jatkowska et al., Citation2021; Kudłak et al., Citation2019; Oliver et al., Citation2020). These complex interactions may occur through a wide variety of mechanisms, for example, the two compounds could act in the same key nodes or in different nodes in the same mechanistic pathway. Moreover, the presence of one could influence the ability to mitigate the action of the other, or the presence of one could influence the available dose of another in the cells targeted. Furthermore, the keto-enol balance of one substance could be affected by presence of the other thus affecting its availability. Such underestimated mechanisms may lead to both decrease and increase of the summarized toxicity of cocktails of xenobiotics of organisms exposed (Lupu et al., Citation2020; Mauderly & Samet, Citation2009). Antecedents of these types of synergies have been seen promoting the development of some types of cancer and there are numerous studies confirming increased activity of various cancer cells exposed to these mixtures of environmental pollutants (Burke & Wei, Citation2009; Hansen et al., Citation2019; Lu et al., Citation2018; Ngamwong et al., Citation2015; Wieczerzak et al., Citation2021). There is thus growing interest to systematically investigate the putative impacts of these associations in the development of leukemia and for its treatment.
The purpose of this work is to discuss the current evidence on how environmental pollutants may participate in the development of the main types of leukemias, with a special emphasis on the role of circadian rhythms in relationship to the growing number of studies linking circadian regulation with cancer and the impact of dysregulated sleep patterns within the modern society.
2. Circadian rhythms
Circadian clocks constitute the evolutionary molecular machinery for keeping track of time and optimizing daily life during day-night cycles and seasonal changes in day length. These coordinate multiple physiological processes by establishing oscillations of biological variables with a 24-hour periodicity, which are called circadian rhythms (Sulli et al., Citation2019). Suprachiasmatic nuclei (SCN) are the master circadian pacemakers of mammals, they receive photic information from retinal ganglion cells (RGCs) and synchronize peripheral clocks with external light/dark cycles through neural and humoral pathways (Shostak, Citation2017). The mammalian circadian clock at the molecular level is made up of a time-delayed transcription-translation feedback loop (TTFL) (). Two enhancer box (E-box) specific transcription factors, brain and muscle ARNT-like 1 (BMAL1) and circadian locomotor output cycles kaput (CLOCK) (or its paralog neuronal PAS domain-containing protein 2 (NPAS2)), form the activating arm of the TTFL, while the cryptochrome (CRY1 and CRY2) and period (PER1, PER2 and probably PER3) proteins constitute the repressor arm. This primary TTFL is consolidated by a secondary loop formed by the nuclear receptors reverse strand of ERB alpha (REV-ERBa) and the retinoic acid receptor-related orphan receptor alpha (RORA) (Sancar & Van Gelder, Citation2021).
Figure 2. Mammalian circadian clock controls transcription and circadian oscillations of blood cell components. A) The mammalian circadian clock mechanism is a time-delayed transcription-translation feedback loop (TTFL). BMAL1-CLOCK constitute the positive arm and cryptochrome (CRY1 and CRY2) - period (PER1 and PER2) constitute the repressive arm. REV-ERB inhibitor and RORA activator form a secondary feedback loop that consolidates the primary loop. It also shows which phases occur in the day (sun) and night (moon). B) Circadian oscillations of different cell types present in the blood and bone marrow. The graphs are based on data taken from Haus et al. (Citation1983), Oh et al. (Citation2019) and Paatela et al. (Citation2019). CCG: Clock controlled gene, WBC: White blood cell, HSPC: Hematopoietic stem and progenitor cells, CT: Circadian time.
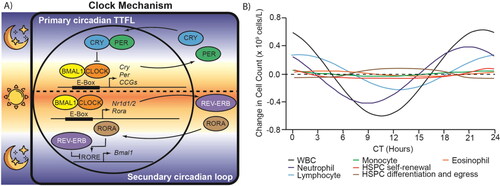
The circadian timing system controls numerous biological functions in mammals, including immune functions, cell cycle events, apoptosis, angiogenesis, xenobiotic metabolism, and detoxification. Components of the circadian clock directly or indirectly regulate the expression of even thousands of clock-controlled genes (CCGs) in different cell types, leading to daily rhythms in many cellular processes including body temperature, endocrine input, sleep-wake cycle, rhythm of fasting-feeding, nutrient metabolism, redox regulation, autophagy, DNA damage repair, protein folding and cellular secretion. Desynchronization of circadian rhythmicity was implicated in several pathologic conditions, including tumorigenesis and progression of cancer (Gachon et al., Citation2004; Shafi & Knudsen, Citation2019). Reinforcing this idea, oncogenic processes directly weaken circadian rhythms (Sulli et al., Citation2019).
The normal functioning of circadian clocks can be altered by genetic, environmental, and internal factors. Indeed, there are interesting recent findings that demonstrate the interaction of the environment, particularly some environmental pollutants, and circadian rhythms (Liu et al., Citation2021; Ndikung et al., Citation2020; Prokkola & Nikinmaa, Citation2018). It has previously been described that cells that follow a circadian synchrony have greater responses to the effects of environmental chemicals (Ndikung et al., Citation2020). For example, dibutyl phthalate (DBP), a common plasticizer in our lives, disrupts the circadian rhythm and promotes the proliferation and migration of mammary cells (Liu et al., Citation2021). However, it is worth mentioning that so far there is very little information that relates the alteration of circadian rhythms with the development, evolution, prognosis and/or treatment of any type of leukemia, as well as whether any environmental pollutant or mixture of them has an important role in altering the genes that control the circadian clock in these cancers.
3. Leukemia and circadian clock genes
Cells present in the bone marrow and blood follow circadian cycles for their proliferation, egression, and function () (Haus et al., Citation1983; Paatela et al., Citation2019; Smaaland et al., Citation2002). Indeed, circadian rhythms are an important long-term regulatory component of hematopoiesis (Méndez-Ferrer et al., Citation2008). In this regard, disruption of circadian rhythms and alterations in circadian clock genes has been reported in the four main types of leukemia (AML, ALL, CML, and CLL) () (Puram et al., Citation2016; Rana et al., Citation2014; Sun et al., Citation2010; Taniguchi et al., Citation2009). There is evidence that peripheral blood mononuclear cells (PBMC) from patients with AML, ALL and CLL retain partially the oscillations of circadian genes, but are absent in cells from CML patients (Rahman et al., Citation2017).
Table 1. Expression of core clock genes in patients with different types of leukemia.
3.1. AML patients
Samples collected from AML patients showed downregulation of BMAL1, PER1, PER2, PER3, CRY1 and CRY2 () that were negatively correlated with the amount of blast cells in the bone marrow and, therefore, indicate that in this type of cancer these proteins act as tumor suppressors (M.-Y. Yang et al., Citation2015). The downregulation of circadian clock genes in patients with AML was maintained until the end of treatment and those who relapsed showed an increase in CRY2 expression (Rahman et al., Citation2017). Related to this, increased DNA methylation of the PER3 promoter, an epigenetic mechanism of gene silencing, was observed in patients with AML and, as treatment with decitabine progressed, this methylation decreased and the expression levels of PER3 increased in patients with complete remission, while in those who relapsed, levels remained low (Y. Wang et al., Citation2011). The same pattern was observed in patients with complete remission and in those who relapsed for PER1 expression (M.-Y. Yang et al., Citation2015). In myeloid leukemic cells that possess the MLL-AF9 fusion gene, a marker associated with an intermediate to good prognosis, it was observed that down-regulation of the CLOCK and BMAL1 genes decreases their cell proliferation, induces their differentiation, and depletes the number of leukemic stem cells (LSCs) (Puram et al., Citation2016; Winters & Bernt, Citation2017). Contrastingly, although normal hematopoietic stem and progenitor cells (HSPCs) are also influenced by the circadian cycle, BMAL1 was not shown to be necessary for their self-renewal, differentiation, or multilineage engagement (Puram et al., Citation2016). To explain these differences, we must mention that oscillating levels of NAD + have been reported to control acetylation of histone methyltransferase mixed-lineage leukemia 1 (MLL1) (also known as histone-lysine N-methyltransferase 2 A (KMT2A)) via NAD+-dependent deacetylase SIRT1 (also called sirtuin 1) and, in turn, CLOCK-BMAL1-dependent transcriptional activation. When NAD + levels are low (e.g. nutrients high), MLL1 is acetylated and its activity increases causing high levels of H3K4me3 and high expression of CCGs, but when NAD + levels increase (e.g. nutrients low), SIRT1 deacetylates MLL1 and reduces its enzymatic activity leading to a decrease in H3K4me3 levels and in the expression of CCGs (Aguilar-Arnal et al., Citation2015). Then, MLL1 controls the levels of H3K4me3 and influences the levels of H3K9ac and H3K14ac in such a way that these have circadian oscillations in the CCG promoters. Also, MLL1 interacts, synergizes and is required for the proper recruitment of the CLOCK-BMAL1 dimer and the activation of CCG transcription (Katada & Sassone-Corsi, Citation2010). Thus, although low levels of BMAL1 are produced in normal cells, the presence of the normal and functional MLL1 protein that synergizes its transcriptional activation functions in CCGs promotes compensation against loss, while in leukemic cells, which express the MLL-AF9 fusion protein, where MLL1 loses several domains, including transactivation and histone methyltranferase SET domains, there is no way to compensate for the low levels of BMAL1.
3.2. ALL patients
In a small cohort analysis, it was found that 33% of ALL patients had decreased expression of BMAL1 due to hypermethylation of its promoter (Taniguchi et al., Citation2009). In RPMI8402 cells, the demethylation of hypermethylated CpG islands restored BMAL1 levels and, consequently, the circadian oscillation of the PER2 gene and its target genes (Tomita et al., Citation2017). Reinforcing these observations, by inducing a decrease in the expression of BMAL1 in MOLT-4 cells, which do not exhibit hypermethylation of CpG islands, there was an increase in their cell growth (Taniguchi et al., Citation2009). In patients newly diagnosed with ALL, there was also a decrease in the expression of CLOCK, PER2, PER3, CRY1, CRY2 and REV-ERBa (), which with the exception of CLOCK remained low until the end of treatment (Rahman et al., Citation2017; M.-Y. Yang et al., Citation2015). In pediatric patients with ALL, altered sleep-rest circadian activity patterns are well described, and less intense sleep-wake rhythms have been associated with increased cancer-related fatigue (Berger et al., Citation2010; Rogers et al., Citation2014). These alterations were stronger when high-dose dexamethasone was used in induction therapy and during the intensive phase of therapy with 6-mercaptopurine and methotrexate (Rogers et al., Citation2014; Steur et al., Citation2020). Furthermore, a long-term follow-up study of survival showed that ALL patients who received maintenance treatment with 6-mercaptopurine at night had a greater chance of complete remission than those who received it in the morning (Rivard et al., Citation1993). The same happened with the combined treatment of 6-mercaptopurine and methotrexate (Clemmensen et al., Citation2014). This could be linked to a higher number of circulating CD10+ lymphocytes at night (Canon et al., Citation1985) and lower levels of DNA repair proteins, particularly those of the nucleotide excision repair (NER) pathway (Sancar & Van Gelder, Citation2021). For ALL patients who achieved remission, BMAL1, PER3, and CRY2 were found at higher levels than patients whose disease relapsed after treatment (M.-Y. Yang et al., Citation2015). The evidence presented above suggests that circadian rhythm may play a role in the development of childhood ALL, as well as affect chemotherapy treatments.
3.3. CML Patients
CML patients showed a significant reduction in the expression of CLOCK, BMAL1, PER2, and CRY1 compared to the expression levels in healthy controls () (Rahman et al., Citation2017). In CML KCL22 cells, it was observed that the overexpression of the PER2 gene prevented its proliferation by modifying the number of cells in each phase of the cell cycle, increasing cells in G1 and decreasing in S/G2 (N. Wang et al., Citation2020). Meanwhile, in CML K562 cells, the overexpression of PER2 caused an arrest in the G2 phase of the cell cycle that later led to apoptosis as p53 levels increased and MYC and Cyclin B1 decreased. Thus, the tumors caused by these cells were smaller and with less infiltration in murine models (Sun et al., Citation2010). The PER2 promoter contains several potential binding sites for the CCAAT/enhancer-binding protein (C/EBP) transcription factors, and at least C/EBP alpha (C/EBPA) and C/EBP epsilon (C/EBPE) are known to bind directly at these sites and stimulate their expression, and where C/EBPA was notably the strongest transcriptional modulator. Likewise, the expression of C/EBP proteins could be regulated by circadian genes, which would represent a feedback loop that could be involved in the development of myeloid leukemias by inhibition of the granulocytic differentiation (Gery et al., Citation2005). Although BMAL1 oscillations are lost in CML cells, inhibition of SIRT1 has been shown to restore them, which also tells us that this protein plays an important role in "fine-tuning" the circadian clock (Rahman et al., Citation2017).
3.4. CLL Patients
At the time of diagnosis, patients with CLL showed a dysregulation of the core clock genes, with high levels of CLOCK and decreased levels of BMAL1, PER1, PER2, CRY1 and REV-ERBa (), and consequently their target genes MYC and Cyclin D1 increased while WEE1 decreased (Rahman et al., Citation2017; Rana et al., Citation2014). Transcriptional silencing of CRY1 by promoter hypermethylation was associated with a favorable prognosis for these patients, particularly when the PER2:CRY1 ratio is low (Eisele et al., Citation2009; Hanoun et al., Citation2012). Indeed, this could explain, in part, why night shift workers are at higher risk of developing this disease (Costas et al., Citation2016).
4. Environmental pollutants and circadian clock genes
Environmental pollutants are compounds found in the environment as a result of human activities and that are hazardous to health. The genes of circadian rhythms are affected by several chemical environmental pollutants that have been associated with the development of leukemias such as benzene, cigarette smoke, some pesticides and microplastics, although for some of them there is still not enough data to be considered as risk factors (). Given that leukemia is a disease that is favored by several factors for its development (multifactorial) (Belson et al., Citation2007; Buffler et al., Citation2005; Davis et al., Citation2014), it is possible that the presence of environmental pollutants that affect circadian rhythms can contribute, to some extent, in people to make them more susceptible to developing leukemia (Scharf et al., Citation2020). In addition, it is not yet known whether the effects of environmental pollutants, or their mixtures (synergistic effects), on leukemic cells and circadian rhythms, also affect the efficacy of the therapeutic drugs used against this disease.
Table 2. Changes in the expression of circadian clock genes after exposure to chemical environmental pollutants.
Exposure to benzene has toxic effects on the blood and bone marrow cells leading to leukopenia, pancytopenia, aplastic anemia, and leukemia (Snyder & Hedli, Citation1996). The hematotoxic effects of benzene and its metabolites, such as phenol, catechol, hydroquinone, 1,2,4-benzenetriol, transtrans-muconic acid and L-phenylmercapturic acid, are due to the fact that these can covalently bind to DNA and other macromolecules and induce damage, cause the overproduction of reactive oxygen species (ROS), as well as other mechanisms that are not yet fully understood (Park et al., Citation2008; Snyder & Hedli, Citation1996). Notably, among the genes most affected by exposure to benzene are those of the circadian rhythms, possibly due to the activation of the aryl hydrocarbon receptor (AhR) that has direct effects on the HSPCs () (Gasiewicz, Singh, & Casado et al., Citation2010; Park et al., Citation2008). AhR is expressed in hematopoietic stem cells (HSCs), in which it is a crucial mediator for their growth and differentiation by regulating c-MYC, OCT4, HIF-1α, NOTCH, and HES-1 signaling. AhR expression leads mainly to the myeloid line, being particularly important in the generation of erythrocytes and monocytes, although it is also important for the differentiation of naïve lymphocytes (Angelos & Kaufman, Citation2018; Casado et al., Citation2010; Lindsey & Papoutsakis, Citation2012). AhR forms a heterodimer with BMAL1 and, in turn, prevents its heterodimerization with CLOCK, thus acting as a transcriptional repressor for CCGs. Difficulty expressing PER1 reduces the robustness of the rhythm and slows down the clock progression (Tischkau, Citation2011; Xu et al., Citation2010).
Figure 3. Impact of aryl hydrocarbon receptor (AhR) activation on circadian rhythms and hematopoiesis. A) The inactive AhR is localized in the cytosol in complex with 90 KDa heat shock protein (HSP90), AhR-interacting protein (AIP), 23 KDa prostaglandin (p23) and cellular-SRC (c-SRC). This can be activated by both endogenous and exogenous (xenobiotic) ligands or agonists. Upon interaction with an agonist, the conformational changes result in the translocation of the complex to the nucleus and the interaction of AhR with BMAL1 after dissociation of the cytoplasmic complex. AhR can compete with CLOCK to form heterodimers with BMAL1 and unlike the CLOCK/BMAL1 dimer that acts as an activator in the promoter E boxes, the AhR/BMAL1 dimer acts as a repressor. B) This causes proteins such as PER1, PER2, CRY1 and CRY2 to not be expressed and, therefore, the amplitude of the circadian oscillations decreases. C) Deregulated AhR signaling in hematopoietic stem and progenitor cells (HSPCs) can cause hematological diseases through a mechanism that is not yet fully understood. AhR overactivation alters bone marrow microenvironment signaling and disrupts quiescence in hematopoietic stem cells (HSCs). Cell proliferation is promoted, and differentiation is altered, favoring mainly the myeloid lineage at the expense of cells of the lymphoid lineage, although AhR overactivation has also been reported in some lymphoid leukemias. LT-HSC: Long-term hematopoietic stem cell, ST-HSC: Short-term hematopoietic stem cell, CMP: Common myeloid progenitor, CLP: Common lymphoid progenitor cell.
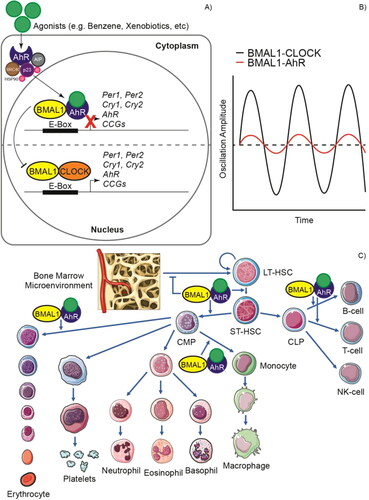
Burnt tobacco smoke is estimated to contain more than 3,800 chemicals of which more than half are considered potential toxins or carcinogens (including benzene) (IARC, Citation2004). Chronic exposure to cigarette smoke damages the cells of the hematopoietic niche, resulting in abnormal hematopoiesis, where proliferative signals increase and stimulate the expansion of HSPCs (Siggins et al., Citation2014). Chronic exposure to cigarette smoke caused a dysregulation of circadian clock genes, with nuclear receptor subfamily 1 group D member 1 (NR1D1) and nuclear receptor subfamily 1 group D member 2 (NR1D2) (genes that code for REV-ERBa), PER3, and PER2 up-regulated, while BMAL1 and RORC were down-regulated, resulting in increased proliferation but also a greater susceptibility to oxidative stress, inflammation, and cell damage (Hwang et al., Citation2014; Tsutsumi et al., Citation2020). This countercyclical change with respect to BMAL1 of several CCGs has been suggested to be due to the alteration in the redox potential in cells and tissues after exposure to cigarette smoke, which consequently alters DNA-binding activity and, in turn, the transcriptional activity of BMAL1-containing transcription factors (Gebel et al., Citation2006). In addition to these changes, a reduction in SIRT1 and an increase in the levels of acetylated BMAL1 were also found facilitating the recruitment of CRY1 to CLOCK-BMAL1, and thus promoting transcriptional repression (Hirayama et al., Citation2007; Hwang et al., Citation2014).
Pesticide is defined as any substance or mixture of substances that is used to prevent, destroy or control pests and includes insecticides, herbicides, fungicides and rodenticides (Abubakar et al., Citation2020). Exposure to household pesticides during pregnancy and their excessive use has been linked to an increased risk of developing childhood leukemia. In these cases, both ALL and AML could develop and in many cases fusion proteins were found as a result of translocations of the MLL gene, probably by inhibition of topoisomerase II (Hernández & Menéndez, Citation2016). Exposure of zebrafish to thifluzamide, a fungicide from the carboxamide family, induced pronounced changes in genes related to circadian rhythms. Among these, the CRY1ba and CLOCK1 transcripts were extremely correlated with the exposure concentrations (Y. Yang et al., Citation2019a). The same was observed with flutolanil, a fungicide of the anilide family, only in the opposite direction, while thifluzamide increases the expression of circadian core genes, flutolanil decreases its expression (Y. Yang et al., Citation2019b). Meanwhile, 2,3,7,8-tetrachlorodibenzo-p-dioxin (TCDD) is an organochloride from the dioxin family that was a contaminant in the herbicide Agent Orange. Like benzene, this is an AhR agonist, albeit with a higher potency, and has been closely linked to the development of leukemia as it causes alterations in the ability of HSCs to respond appropriately to signals within the medullary microenvironment and disrupts their quiescence (Garrett & Gasiewicz, Citation2006; Singh et al., Citation2009). TCDD affected in a dose-dependent manner the rhythmicity of core clock genes by reducing the amplitude or causing the complete loss of their oscillations and, consequently, also of a large proportion of the CCGs (Fader et al., Citation2019). Polychlorinated biphenyls (PCBs) are persistent organic pollutants that are widely distributed in the environment and have also been considered a probable risk factor for childhood leukemia (Ward et al., Citation2009). Of these, PCB153 was shown to positively regulate several genes involved in the circadian rhythm of zebrafish embryos (Aluru et al., Citation2020).
Plasticizers, produced for nearly 100 years, have become ubiquitous in society and are found in a variety of everyday products. Many of these have been shown to participate in the development of diseases when they are introduced into the body by inhalation, ingestion and dermal absorption (Zarus et al., Citation2021). Some of these such as bisphenol A (BPA), phthalates and perfluorinated chemicals can act as endocrine disrupting compounds (EDCs) with significant consequences including infertility, polycystic ovary syndrome (PCOS), precocious puberty, hormone-dependent tumors, such as breast and prostate cancer, and several metabolic disorders linked to cancer (Braun et al., Citation2013; Dematteo et al., Citation2012; Diamanti-Kandarakis et al., Citation2009; Loganathan et al., Citation2019). BPA is widely used in plastics intended for direct contact with food, including plastic packaging, coatings of cans and jar caps, as well as kitchenware. BPA at nanomolar concentrations promotes the proliferation of acute promyelocytic leukemia HL-60 and histiocytic lymphoma U937 cells and reduces their sensitivity to daunorubicin and cytarabine by causing an increase in IL-4 levels through NFAT1, and increasing IL-6 levels through the NF‐κB pathway (Zhang et al., Citation2020). However, leukemic cells were also shown to be more sensitive to the cytotoxic effects of BPA than other types of cancer cells, with apoptosis cascades being activated more rapidly in leukemic cells (Terasaka et al., Citation2005). NB4 cells that possess the PML-RARA fusion protein were the most sensitive to BPA, which induced their differentiation and activated apoptosis pathways by causing an increase in the levels of p21, p27, p16 and RB, while decreasing those of cyclin D1 (Bontempo et al., Citation2009). Interestingly, in mouse pro-opiomelanocortin (POMC) neurons, BPA has altered the circadian clock, mainly in the BMAL1, PER2 and REV-ERBa genes, and also caused an increase in the binding of BMAL1 to its target promoters that they are reflected in deleterious effects on the expression of neuropeptides (Loganathan et al., Citation2019).
Phthalates are commonly used in medical devices and in personal care products such as soaps, lubricating oils, hairsprays, perfumes, cosmetics, and shampoos. The L5178Y mouse lymphoma mammalian cell mutation assay demonstrated mutagenic activity of dimethyl phthalate (DMP) and DBP, but only in the presence of hepatic S9 pools that are a rich source of drug metabolizing enzymes, including those of the P-450 family, suggesting that the damage is caused primarily by its metabolites. This was not the case for butyl benzyl phthalate (BBP), di-{n hexyl, n-octyl, n-decyl} phthalate (610 P), di-isononyl phthalate (DINP), di-{heptyl, nonyl, undecyl} phthalate (711 P), diisodecyl phthalate (DIDP) and di-undecyl phthalate (DUP) (Barber et al., Citation2000). On the other hand, exposure to DBP at a concentration of 100 μg/mL for four days was shown to selectively affect the viability of acute myeloid leukemic blast progenitor cells (AML-CFU), but not of normal granulocyte/macrophage progenitor cells (CFU-GM) (Wu et al., Citation1995). Apoptosis was also caused in acute megakaryoblastic leukemia cells M-O7e and histiocytic lymphoma U-937 using DBP at a concentration of 100 μg/mL, as well as in cells of promyelocytic leukemia HL-60 with a concentration of 50 μg/mL for nine days (Chu-Tse et al., Citation1993; L.-S. Wang et al., Citation2002). In a clinical trial in patients with AML, bone marrow samples were collected for autotransplantation. For this, bone marrow collection bags containing a culture medium with DBP were used and after 6 days the medium was replaced with medium without DBP. In this manner, a significant reduction in the number of leukemic cells was observed, but an adequate number of normal cells was maintained to repopulate the bone marrow of the patients. Thus, DBP was considered as a purging agent (Lisheng et al., Citation1996). Meanwhile, di(2-ethylhexyl) phthalate (DEHP) was shown to cause apoptosis in HL-60 promyelocytic leukemia cells, mainly at high doses (over 100 μg/mL), and also reduce cell migration at low doses (10 μg/mL). Its metabolite, MEHP, required lower doses to cause apoptosis (50 μg/mL), but higher doses to affect cell migration (25 μg/mL) (Manz et al., Citation2014). Regarding the circadian clock genes, it was shown that DEHP significantly alters their levels, increasing the expression of CLOCK, CRY1 and NR1D1, but decreasing the expression of PER2 and PER3 () (Currie et al., Citation2005).
5. Conclusions and future perspective
Circadian rhythms are strongly linked to cancer, including breast, ovarian, prostate, brain, kidney, gastric, lung, liver, and colorectal cancer, among others (Masri & Sassone-Corsi, Citation2018; Sancar & Van Gelder, Citation2021; Sulli et al., Citation2019; Verlande & Masri, Citation2019). Notably, both circulating blood cells and those in the bone marrow present circadian rhythms in their proliferation, function, differentiation, and discharge. Thus, when these rhythms are altered, there is a possibility that they facilitate the development of leukemia. For many years, an accepted model of leukemogenesis was the "two-hit hypothesis", which suggested that two different types of alterations were required for malignant transformation. From the data we describe here, we suggest that altering genes that control circadian rhythms, whether by altered gene expression, mutations, epigenetic changes, or other mechanisms, are unlikely to cause leukemia alone, but may play an important role to predispose for its development. This could be a "first hit" and would subsequently only require a "second hit", possibly of lesser magnitude, affecting some tumor suppressor gene, DNA repair gene, or activating an oncogene to induce cancer.
It can be concluded that more experiments are necessary to confirm and validate the magnitude of the putative impact that environmental pollutants and circadian rhythm disruption have on various forms of leukemia. New models could be developed where circadian genes, such as CLOCK or BMAL1, are silenced to investigate if these are more susceptible to developing leukemia when exposed to agents, such as benzene. Likewise, these models can be combined with models where mutations are generated in genes that drive leukemogenesis. Clearly, this is now easier with improvements in gene editors, for example the CRISPR/Cas9 system (Fellmann et al., Citation2017). Furthermore, some of these genes, after being confirmed in larger clinical trials, can also be considered as markers for the diagnosis, prognosis and/or evaluation of the efficacy of the treatment. This can be illustrated with PER1 and PER3 in AML patients and BMAL1, PER3 and CRY2 in ALL patients, which increase their expression in patients with complete remission (Y. Wang et al., Citation2011; M.-Y. Yang et al., Citation2015). It is also important to better decipher the exact molecular pathways involved in the dysregulation of the expression of such genes for each type of leukemia using genome wide RNA sequencing.
It is important to identify the environmental factors and their combinations that can lead to the alteration of circadian clock genes and subsequently quantify this impact. It is also valuable to put this in the perspective of which pollutants we are most exposed to during our routine habits. It would be very valuable to determine the actual presence of pollutants in the blood or urine of patients (Lee et al., Citation2011). Therefore, more tools must be created to make this easier and more frequent. More experiments are required to determine which single compounds can cross the blood-brain barrier and how co-present admixtured xenobiotics affect this process. Experiments to determine the specific effects these have on bone marrow cells, both individually and in the form of pollutants cocktails, must also be performed, in order to uncover possible synergies and antagonisms. The effects of mixtures are notoriously difficult to study for several reasons, the most obvious being the number of possible combinations is immense. Moreover, the problem is compounded by the fact that synergistic or antagonistic effects are most often dose dependent, vary widely in different systems and experimental organisms and can be endpoint dependent. Antagonistic effects of some compounds can be hidden in experimental models where synergistically active ingredients are co-present. In addition, other factors that influence the results obtained should also be considered such as artificial light-at-night (ALAN), which has been related to an increased risk of cancers in breast and colorectum, as well as the alteration of circadian rhythms (Al-Naggar & Anil, Citation2016; Fonken & Nelson, Citation2014). Therefore, more consistent and high-performance studies with models that allow the analysis of this type of interaction have become a necessity.
While we are making great strides in cancer drug development, for example genetically targeted therapies (Huang et al., Citation2020), soluble ligands (Attwood et al., Citation2020; Lagunas-Rangel & Bermúdez-Cruz, Citation2020) and kinases (Attwood et al., Citation2021), there is a need to better understand how drug treatments are affected by pollutants and circadian rhythms, and if this can be controlled in such a way as to provide the best benefit for patients. Certainly, as computer and artificial intelligence systems improve, we will be able to explore the dynamics of tumor progression in such a way that we simulate a variety of scenarios and generate "virtual tumors" with mutational patterns that mimic patient data. In addition, possible scenarios could be predicted of how complex cocktails of environmental pollutants are absorbed and metabolized, if they cross the blood-brain barrier and if they affect circadian rhythms and/or interact with therapeutic drugs, among many other considerations (). In this way, it will be easier for medical personnel to model and predict the effects of compound mixtures and to describe/control their role in the generation and treatment of leukemia (or other cancer). Finally, based on the experimental results obtained, improvements could be made in regulations related to exposure to environmental pollutants, as well as in the strategies and technologies to reduce or eliminate their presence.
Figure 4. Schematic presentation of possible future experimental developments. We consider four levels: determining pollutants and drug intake, their metabolism/transformation, their arrival at the target organs and tissues, and the eventual development/treatment of leukemia in relation to alterations in circadian cycles.
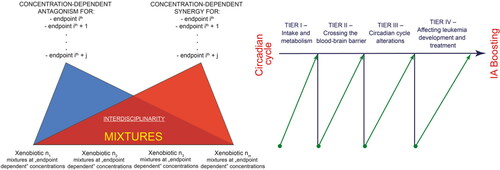
Disclosure statement
No potential conflict of interest was reported by the authors.
Additional information
Funding
References
- Abubakar, Y., Tijjani, H., Egbuna, C., Adetunji, C. O., Kala, S., Kryeziu, T. L., … Patrick-Iwuanyanwu, K. C. (2020). Pesticides, history, and classification. In Natural remedies for pest, disease and weed control (pp. 29–42). Elsevier. https://doi.org/10.1016/B978-0-12-819304-4.00003-8
- Aguilar-Arnal, L., Katada, S., Orozco-Solis, R., & Sassone-Corsi, P. (2015). NAD(+)-SIRT1 control of H3K4 trimethylation through circadian deacetylation of MLL1. Nature Structural & Molecular Biology, 22(4), 312–318. https://doi.org/10.1038/nsmb.2990
- Al-Naggar, R. A., & Anil, S. (2016). Artificial Light at Night and Cancer: Global Study. Asian Pacific Journal of Cancer Prevention: APJCP, 17(10), 4661–4664. https://doi.org/10.22034/apjcp.2016.17.10.4661
- Aluru, N., Krick, K. S., McDonald, A. M., & Karchner, S. I. (2020). Developmental exposure to PCB153 (2,2',4,4',5,5'-Hexachlorobiphenyl) alters circadian rhythms and the expression of clock and metabolic genes. Toxicological Sciences: An Official Journal of the Society of Toxicology, 173(1), 41–52. https://doi.org/10.1093/toxsci/kfz217
- Angelos, M. G., & Kaufman, D. S. (2018). Advances in the role of the aryl hydrocarbon receptor to regulate early hematopoietic development. Current Opinion in Hematology, 25(4), 273–278. https://doi.org/10.1097/MOH.0000000000000432
- Arber, D. A., Orazi, A., Hasserjian, R., Thiele, J., Borowitz, M. J., Le Beau, M. M., Bloomfield, C. D., Cazzola, M., & Vardiman, J. W. (2016). The 2016 revision to the World Health Organization classification of myeloid neoplasms and acute leukemia. Blood, 127(20), 2391–2405. https://doi.org/10.1182/blood-2016-03-643544
- Attwood, M. M.,Fabbro, D.,Sokolov, A. V.,Knapp, S., &Schiöth, H. B. (2021). Trends in kinase drug discovery: targets, indications and inhibitor design. Nature Reviews Drug Discovery. https://doi.org/10.1038/s41573-021-00252-y
- Attwood, M. M., Jonsson, J., Rask-Andersen, M., & Schiöth, H. B. (2020). Soluble ligands as drug targets. Nature Reviews. Drug Discovery, 19(10), 695–710. https://doi.org/10.1038/s41573-020-0078-4
- Barber, E. D., Cifone, M., Rundell, J., Przygoda, R., Astill, B. D., Moran, E., Mulholland, A., Robinson, E., & Schneider, B. (2000). Results of the L5178Y mouse lymphoma assay and the Balb/3T3 cell in vitro transformation assay for eight phthalate esters. Journal of Applied Toxicology, 20(1), 69–80. https://doi.org/10.1002/(SICI)1099-1263(200001/02)20:1 < 69::AID-JAT630 > 3.0.CO;2-2
- Belson, M., Kingsley, B., & Holmes, A. (2007). Risk factors for acute leukemia in children: A review. Environmental Health Perspectives, 115(1), 138–145. https://doi.org/10.1289/ehp.9023
- Berger, A. M., Wielgus, K., Hertzog, M., Fischer, P., & Farr, L. (2010). Patterns of circadian activity rhythms and their relationships with fatigue and anxiety/depression in women treated with breast cancer adjuvant chemotherapy. Supportive Care in Cancer: Official Journal of the Multinational Association of Supportive Care in Cancer, 18(1), 105–114. https://doi.org/10.1007/s00520-009-0636-0
- Boffetta, P. (2006). Human cancer from environmental pollutants: The epidemiological evidence. Mutation Research, 608(2), 157–162. https://doi.org/10.1016/j.mrgentox.2006.02.015
- Bontempo, P., Mita, L., Doto, A., Miceli, M., Nebbioso, A., Lepore, I., Franci, G., Menafra, R., Carafa, V., Conte, M., De Bellis, F., Manzo, F., Di Cerbo, V., Benedetti, R., D'Amato, L., Marino, M., Bolli, A., Del Pozzo, G., Diano, N., … Altucci, L. (2009). Molecular analysis of the apoptotic effects of BPA in acute myeloid leukemia cells. Journal of Translational Medicine, 7(1), 48. https://doi.org/10.1186/1479-5876-7-48
- Braun, J. M., Sathyanarayana, S., & Hauser, R. (2013). Phthalate exposure and children's health. Current Opinion in Pediatrics, 25(2), 247–254. https://doi.org/10.1097/MOP.0b013e32835e1eb6
- Buffler, P., Kwan, M., Reynolds, P., & Urayama, K. (2005). Environmental and genetic risk factors for childhood leukemia: Appraising the evidence. Cancer Investigation, 23(1), 60–75. https://doi.org/10.1081/CNV-200046402
- Burke, K., & Wei, H. (2009). Synergistic damage by UVA radiation and pollutants. Toxicology and Industrial Health, 25(4–5), 219–224. https://doi.org/10.1177/0748233709106067
- Canon, C., Lévi, F., Reinberg, A., & Mathe, G. (1985). Circulating CALLA-positive lymphocytes exhibit circadian rhythms in man. Leukemia Research, 9(12), 1539–1546. https://doi.org/10.1016/0145-2126(85)90047-5
- Casado, F. L., Singh, K. P., & Gasiewicz, T. A. (2010). The aryl hydrocarbon receptor: Regulation of hematopoiesis and involvement in the progression of blood diseases. Blood Cells, Molecules & Diseases, 44(4), 199–206. https://doi.org/10.1016/j.bcmd.2010.01.005
- Cedergreen, N. (2014). Quantifying synergy: A systematic review of mixture toxicity studies within environmental toxicology. PLoS One, 9(5), e96580. https://doi.org/10.1371/journal.pone.0096580
- Chu-Tse, W., Xue-Tao, P., Jun-Rong, C., & Hui-Hua, X. (1993). A new pharmacological activity of dibutyl phthalate (DBP) on selective elimination of tumor cells from bone marrow. Leukemia Research, 17(7), 557–560. https://doi.org/10.1016/0145-2126(93)90084-X
- Clemmensen, K. K. B., Christensen, R. H., Shabaneh, D. N., Harila-Saari, A., Heyman, M., Jonsson, O. G., Wesenberg, F., Rosthøj, S., & Schmiegelow, K., On behalf of the Nordic Society of Pediatric Hematology, Oncology (NOPHO). (2014). The circadian schedule for childhood acute lymphoblastic leukemia maintenance therapy does not influence event-free survival in the NOPHO ALL92 protocol. Pediatric Blood & Cancer, 61(4), 653–658. https://doi.org/10.1002/pbc.24867
- Costas, L., Benavente, Y., Olmedo-Requena, R., Casabonne, D., Robles, C., Gonzalez-Barca, E.-M., de la Banda, E., Alonso, E., Aymerich, M., Tardón, A., Marcos-Gragera, R., Gimeno-Vázquez, E., Gómez-Acebo, I., Papantoniou, K., Castaño-Vinyals, G., Aragonés, N., Pollán, M., Kogevinas, M., & de Sanjosé, S. (2016). Night shift work and chronic lymphocytic leukemia in the MCC-Spain case-control study. International Journal of Cancer, 139(9), 1994–2000. https://doi.org/10.1002/ijc.30272
- Currie, R. A., Bombail, V., Oliver, J. D., Moore, D. J., Lim, F. L., Gwilliam, V., Kimber, I., Chipman, K., Moggs, J. G., & Orphanides, G. (2005). Gene ontology mapping as an unbiased method for identifying molecular pathways and processes affected by toxicant exposure: Application to acute effects caused by the rodent non-genotoxic carcinogen diethylhexylphthalate. Toxicological Sciences: An Official Journal of the Society of Toxicology, 86(2), 453–469. https://doi.org/10.1093/toxsci/kfi207
- Davis, A. S., Viera, A. J., & Mead, M. D. (2014). Leukemia: An overview for primary care. American Family Physician, 89(9), 731–738. Retrieved from http://www.ncbi.nlm.nih.gov/pubmed/24784336
- DeMatteo, R., Keith, M. M., Brophy, J. T., Wordsworth, A., Watterson, A. E., Beck, M., Ford, A. R., Gilbertson, M., Pharityal, J., Rootham, M., & Scott, D. N. (2012). Chemical exposures of women workers in the plastics industry with particular reference to breast cancer and reproductive hazards. New Solutions: A Journal of Environmental and Occupational Health Policy: NS, 22(4), 427–448. https://doi.org/10.2190/NS.22.4.d
- Diamanti-Kandarakis, E., Bourguignon, J.-P., Giudice, L. C., Hauser, R., Prins, G. S., Soto, A. M., Zoeller, R. T., & Gore, A. C. (2009). Endocrine-disrupting chemicals: An endocrine society scientific statement. Endocrine Reviews, 30(4), 293–342. https://doi.org/10.1210/er.2009-0002
- Eisele, L., Prinz, R., Klein-Hitpass, L., Nückel, H., Lowinski, K., Thomale, J., Moeller, L. C., Dührsen, U., & Dürig, J. (2009). Combined PER2 and CRY1 expression predicts outcome in chronic lymphocytic leukemia. European Journal of Haematology, 83(4), 320–327. https://doi.org/10.1111/j.1600-0609.2009.01287.x
- Elert, E. (2013). Living with leukaemia. Nature, 498(7455), S2–S3. https://doi.org/10.1038/498S2a
- Fader, K. A., Nault, R., Doskey, C. M., Fling, R. R., & Zacharewski, T. R. (2019). 2,3,7,8-Tetrachlorodibenzo-p-dioxin abolishes circadian regulation of hepatic metabolic activity in mice. Scientific Reports, 9(1), 6514. https://doi.org/10.1038/s41598-019-42760-3
- Fellmann, C., Gowen, B. G., Lin, P.-C., Doudna, J. A., & Corn, J. E. (2017). Cornerstones of CRISPR-Cas in drug discovery and therapy. Nature Reviews. Drug Discovery, 16(2), 89–100. https://doi.org/10.1038/nrd.2016.238
- Filippini, T., Hatch, E. E., Rothman, K. J., Heck, J. E., Park, A. S., Crippa, A., Orsini, N., & Vinceti, M. (2019). Association between outdoor air pollution and childhood leukemia: A systematic review and dose-response meta-analysis. Environmental Health Perspectives, 127(4), 46002. https://doi.org/10.1289/EHP4381
- Fonken, L. K., & Nelson, R. J. (2014). The effects of light at night on circadian clocks and metabolism. Endocrine Reviews, 35(4), 648–670. https://doi.org/10.1210/er.2013-1051
- Gachon, F., Nagoshi, E., Brown, S., Ripperger, J., & Schibler, U. (2004). The mammalian circadian timing system: From gene expression to physiology. Chromosoma, 113(3), 103–112. https://doi.org/10.1007/s00412-004-0296-2
- Garrett, R. W., & Gasiewicz, T. A. (2006). The aryl hydrocarbon receptor agonist 2,3,7,8-tetrachlorodibenzo-p-dioxin alters the circadian rhythms, quiescence, and expression of clock genes in murine hematopoietic stem and progenitor cells. Molecular Pharmacology, 69(6), 2076–2083. https://doi.org/10.1124/mol.105.021006
- Gasiewicz, T. A., Singh, K. P., & Casado, F. L. (2010). The aryl hydrocarbon receptor has an important role in the regulation of hematopoiesis: Implications for benzene-induced hematopoietic toxicity. Chemico-Biological Interactions, 184(1–2), 246–251. https://doi.org/10.1016/j.cbi.2009.10.019
- Geary, N. (2013). Understanding synergy. American Journal of Physiology. Endocrinology and Metabolism, 304(3), E237–E253. https://doi.org/10.1152/ajpendo.00308.2012
- Gebel, S., Gerstmayer, B., Kuhl, P., Borlak, J., Meurrens, K., & Müller, T. (2006). The kinetics of transcriptomic changes induced by cigarette smoke in rat lungs reveals a specific program of defense, inflammation, and circadian clock gene expression. Toxicological Sciences: An Official Journal of the Society of Toxicology, 93(2), 422–431. https://doi.org/10.1093/toxsci/kfl071
- Gery, S., Gombart, A. F., Yi, W. S., Koeffler, C., Hofmann, W.-K., & Koeffler, H. P. (2005). Transcription profiling of C/EBP targets identifies Per2 as a gene implicated in myeloid leukemia. Blood, 106(8), 2827–2836. https://doi.org/10.1182/blood-2005-01-0358
- Hanoun, M., Eisele, L., Suzuki, M., Greally, J. M., Hüttmann, A., Aydin, S., Scholtysik, R., Klein-Hitpass, L., Dührsen, U., & Dürig, J. (2012). Epigenetic silencing of the circadian clock gene CRY1 is associated with an indolent clinical course in chronic lymphocytic leukemia. PLoS One, 7(3), e34347. https://doi.org/10.1371/journal.pone.0034347
- Hansen, K. E. A., Johanson, S. M., Steppeler, C., Sødring, M., Østby, G. C., Berntsen, H. F., Zimmer, K. E., Aleksandersen, M., Paulsen, J. E., & Ropstad, E. (2019). A mixture of Persistent Organic Pollutants (POPs) and Azoxymethane (AOM) show potential synergistic effects on intestinal tumorigenesis in the A/J Min/+ mouse model. Chemosphere, 214, 534–542. https://doi.org/10.1016/j.chemosphere.2018.09.126
- Haus, E., Lakatua, D. J., Swoyer, J., & Sackett-Lundeen, L. (1983). Chronobiology in hematology and immunology. The American Journal of Anatomy, 168(4), 467–517. https://doi.org/10.1002/aja.1001680406
- Hernández, A., & Menéndez, P. (2016). Linking pesticide exposure with pediatric leukemia: Potential underlying mechanisms. International Journal of Molecular Sciences, 17(4), 461. https://doi.org/10.3390/ijms17040461
- Hirayama, J., Sahar, S., Grimaldi, B., Tamaru, T., Takamatsu, K., Nakahata, Y., & Sassone-Corsi, P. (2007). CLOCK-mediated acetylation of BMAL1 controls circadian function. Nature, 450(7172), 1086–1090. https://doi.org/10.1038/nature06394
- Huang, A., Garraway, L. A., Ashworth, A., & Weber, B. (2020). Synthetic lethality as an engine for cancer drug target discovery. Nature Reviews. Drug Discovery, 19(1), 23–38. https://doi.org/10.1038/s41573-019-0046-z
- Hwang, J., Sundar, I. K., Yao, H., Sellix, M. T., & Rahman, I. (2014). Circadian clock function is disrupted by environmental tobacco/cigarette smoke, leading to lung inflammation and injury via a SIRT1-BMAL1 pathway. FASEB Journal: Official Publication of the Federation of American Societies for Experimental Biology, 28(1), 176–194. https://doi.org/10.1096/fj.13-232629
- IARC. (2004). Tobacco smoke and involuntary smoking. In IARC monographs on the evaluation of carcinogenic risks to humans (Vol. 83). World Health Organization.
- Jatkowska, N., Kudłak, B., Lewandowska, P., Liu, W., Williams, M. J., & Schiöth, H. B. (2021). Identification of synergistic and antagonistic actions of environmental pollutants: Bisphenols A, S and F in the presence of DEP, DBP, BADGE and BADGE·2HCl in three component mixtures. The Science of the Total Environment, 767, 144286. https://doi.org/10.1016/j.scitotenv.2020.144286
- Katada, S., & Sassone-Corsi, P. (2010). The histone methyltransferase MLL1 permits the oscillation of circadian gene expression. Nature Structural & Molecular Biology, 17(12), 1414–1421. https://doi.org/10.1038/nsmb.1961
- Koual, M., Tomkiewicz, C., Cano-Sancho, G., Antignac, J.-P., Bats, A.-S., & Coumoul, X. (2020). Environmental chemicals, breast cancer progression and drug resistance. Environmental Health, 19(1), 117. https://doi.org/10.1186/s12940-020-00670-2
- Kudłak, B., Wieczerzak, M., & Namieśnik, J. (2019). Bisphenols (A, S, and F) affect the basic hormonal activity determined for pharmaceuticals - Study of Saccharomyces cerevisiae. Environmental Pollution (Barking, Essex: 1987), 246, 914–920. https://doi.org/10.1016/j.envpol.2018.12.052
- Laermans, J., & Depoortere, I. (2016). Chronobesity: Role of the circadian system in the obesity epidemic. Obesity Reviews: An Official Journal of the International Association for the Study of Obesity, 17(2), 108–125. https://doi.org/10.1111/obr.12351
- Lagunas-Rangel, F. A., & Bermúdez-Cruz, R. M. (2020). Natural compounds that target DNA repair pathways and their therapeutic potential to counteract cancer cells. Frontiers in Oncology, 10(November), 598174. https://doi.org/10.3389/fonc.2020.598174
- Lagunas-Rangel, F. A., & Chávez-Valencia, V. (2017). FLT3–ITD and its current role in acute myeloid leukaemia. Medical Oncology, 34(6), 114. https://doi.org/10.1007/s12032-017-0970-x
- Lagunas-Rangel, F. A., Chávez-Valencia, V., Gómez-Guijosa, M. Á., & Cortes-Penagos, C. (2017). Acute myeloid leukemia-genetic alterations and their clinical prognosis. International Journal of Hematology-Oncology and Stem Cell Research, 11(4), 328–339. Retrieved from http://www.ncbi.nlm.nih.gov/pubmed/29340131
- Lee, D.-H., Lind, P. M., Jacobs, D. R., Salihovic, S., van Bavel, B., & Lind, L. (2011). Polychlorinated biphenyls and organochlorine pesticides in plasma predict development of Type 2 diabetes in the elderly. Diabetes Care, 34(8), 1778–1784. https://doi.org/10.2337/dc10-2116
- Lindsey, S., & Papoutsakis, E. T. (2012). The evolving role of the Aryl Hydrocarbon Receptor (AHR) in the normophysiology of hematopoiesis. Stem Cell Reviews and Reports, 8(4), 1223–1235. https://doi.org/10.1007/s12015-012-9384-5
- Lisheng, W., Chutse, W., Xuetao, P., Li, X., Jurong, C., Huihua, X., Ke, Y., Aiping, T., & Shuquan, J. (1996). Dibutyl phthalate purged autologous bone marrow transplant in the treatment of leukemia. Leukemia Research, 20(4), 343–348. https://doi.org/10.1016/0145-2126(95)00143-3
- Liu, W., Cao, H., Liao, S., Kudłak, B., Williams, M. J., & Schiöth, H. B. (2021). Dibutyl phthalate disrupts conserved circadian rhythm in Drosophila and human cells. The Science of the Total Environment, 783, 147038. https://doi.org/10.1016/j.scitotenv.2021.147038
- Loganathan, N., Salehi, A., Chalmers, J. A., & Belsham, D. D. (2019). Bisphenol A alters Bmal1, Per2, and Rev-Erba mRNA and requires Bmal1 to increase neuropeptide Y expression in hypothalamic neurons. Endocrinology, 160(1), 181–192. https://doi.org/10.1210/en.2018-00881
- Lu, G., Qin, D., Wang, Y., Liu, J., & Chen, W. (2018). Single and combined effects of selected haloacetonitriles in a human-derived hepatoma line. Ecotoxicology and Environmental Safety, 163(July), 417–426. https://doi.org/10.1016/j.ecoenv.2018.07.104
- Lupu, D., Andersson, P., Bornehag, C. G., Demeneix, B., Fritsche, E., Gennings, C., … Rüegg, J. (2020). The endpoints project: Novel testing strategies for endocrine disruptors linked to developmental neurotoxicity. International Journal of Molecular Sciences, 21(11), 3978. https://doi.org/10.3390/ijms21113978
- Manz, P., Cadeddu, R.-P., Wilk, M., Fritz, B., Haas, R., & Wenzel, F. (2014). Impact of Di(2-ethylhexyl)phthalate on migration rate of human promyelocytic leukemia cells (HL-60). Clinical Hemorheology and Microcirculation, 58(1), 241–246. https://doi.org/10.3233/CH-141903
- Masri, S., & Sassone-Corsi, P. (2018). The emerging link between cancer, metabolism, and circadian rhythms. Nature Medicine, 24(12), 1795–1803. https://doi.org/10.1038/s41591-018-0271-8
- Mauderly, J. L., & Samet, J. M. (2009). Is there evidence for synergy among air pollutants in causing health effects? Environmental Health Perspectives, 117(1), 1–6. https://doi.org/10.1289/ehp.11654
- Méndez-Ferrer, S., Lucas, D., Battista, M., & Frenette, P. S. (2008). Haematopoietic stem cell release is regulated by circadian oscillations. Nature, 452(7186), 442–447. https://doi.org/10.1038/nature06685
- Ndikung, J., Storm, D., Violet, N., Kramer, A., Schönfelder, G., Ertych, N., & Oelgeschläger, M. (2020). Restoring circadian synchrony in vitro facilitates physiological responses to environmental chemicals. Environment International, 134, 105265. https://doi.org/10.1016/j.envint.2019.105265
- Ngamwong, Y., Tangamornsuksan, W., Lohitnavy, O., Chaiyakunapruk, N., Scholfield, C. N., Reisfeld, B., & Lohitnavy, M. (2015). Additive synergism between asbestos and smoking in lung cancer risk: A systematic review and meta-analysis. PLOS One, 10(8), e0135798. https://doi.org/10.1371/journal.pone.0135798
- Niu, J., Straubinger, R. M., & Mager, D. E. (2019). Pharmacodynamic drug-drug interactions. Clinical Pharmacology and Therapeutics, 105(6), 1395–1406. https://doi.org/10.1002/cpt.1434
- Oh, G., Koncevičius, K., Ebrahimi, S., Carlucci, M., Groot, D. E., Nair, A., Zhang, A., Kriščiūnas, A., Oh, E. S., Labrie, V., Wong, A. H. C., Gordevičius, J., Jia, P., Susic, M., & Petronis, A. (2019). Circadian oscillations of cytosine modification in humans contribute to epigenetic variability, aging, and complex disease. Genome Biology, 20(1), 2. https://doi.org/10.1186/s13059-018-1608-9
- Oliver, M., Kudłak, B., Wieczerzak, M., Reis, S., Lima, S. A. C., Segundo, M. A., & Miró, M. (2020). Ecotoxicological equilibria of triclosan in Microtox, XenoScreen YES/YAS, Caco2, HEPG2 and liposomal systems are affected by the occurrence of other pharmaceutical and personal care emerging contaminants. The Science of the Total Environment, 719, 137358. https://doi.org/10.1016/j.scitotenv.2020.137358
- Paatela, E., Munson, D., & Kikyo, N. (2019). Circadian regulation in tissue regeneration. International Journal of Molecular Sciences, 20(9), 2263. https://doi.org/10.3390/ijms20092263
- Park, H.-J., Oh, J. H., Yoon, S., & Rana, S. V. S. (2008). Time dependent gene expression changes in the liver of mice treated with benzene. Biomarker Insights, 3(3), BMI.S590. https://doi.org/10.4137/bmi.s590
- Prokkola, J. M., & Nikinmaa, M. (2018). Circadian rhythms and environmental disturbances – underexplored interactions. Journal of Experimental Biology, 221(16), 1–9. https://doi.org/10.1242/jeb.179267
- Puram, R. V., Kowalczyk, M. S., de Boer, C. G., Schneider, R. K., Miller, P. G., McConkey, M., Tothova, Z., Tejero, H., Heckl, D., Järås, M., Chen, M. C., Li, H., Tamayo, A., Cowley, G. S., Rozenblatt-Rosen, O., Al-Shahrour, F., Regev, A., & Ebert, B. L. (2016). Core circadian clock genes regulate leukemia stem cells in AML. Cell, 165(2), 303–316. https://doi.org/10.1016/j.cell.2016.03.015
- Rahman, S., Al-Hallaj, A.-S., Nedhi, A., Gmati, G., Ahmed, K., Jama, H. A., Trivilegio, T., Mashour, A., Askar, A. A., & Boudjelal, M. (2017). Differential expression of circadian genes in leukemia and a possible role for Sirt1 in restoring the circadian clock in chronic myeloid leukemia. Journal of Circadian Rhythms, 15(1), 3–13. https://doi.org/10.5334/jcr.147
- Rana, S., Munawar, M., Shahid, A., Malik, M., Ullah, H., Fatima, W., Mohsin, S., & Mahmood, S. (2014). Deregulated expression of circadian clock and clock-controlled cell cycle genes in chronic lymphocytic leukemia. Molecular Biology Reports, 41(1), 95–103. https://doi.org/10.1007/s11033-013-2841-7
- Rivard, G. E., Infante-Rivard, C., Dresse, M.-F., Leclerc, J.-M., & Champagne, J. (1993). Circadian time-dependent response of childhood lymphoblastic leukemia to chemotherapy: A long-term follow-up study of survival. Chronobiology International, 10(3), 201–204. https://doi.org/10.3109/07420529309073888
- Rogers, V. E., Zhu, S., Ancoli-Israel, S., & Hinds, P. S. (2014). Impairment in circadian activity rhythms occurs during dexamethasone therapy in children with leukemia. Pediatric Blood & Cancer, 61(11), 1986–1991. https://doi.org/10.1002/pbc.25147
- Sancar, A., & Van Gelder, R. N. (2021). Clocks, cancer, and chronochemotherapy. Science, 371(6524), eabb0738. https://doi.org/10.1126/science.abb0738
- Scharf, P., Broering, M. F., Oliveira da Rocha, G. H., & Farsky, S. H. P. (2020). Cellular and molecular mechanisms of environmental pollutants on hematopoiesis. International Journal of Molecular Sciences, 21(19), 6996. https://doi.org/10.3390/ijms21196996
- Shafi, A. A., & Knudsen, K. E. (2019). Cancer and the circadian clock. Cancer Research, 79(15), 3806–3814. https://doi.org/10.1158/0008-5472.CAN-19-0566
- Shostak, A. (2017). Circadian clock, cell division, and cancer: From molecules to organism. International Journal of Molecular Sciences, 18(4), 873. https://doi.org/10.3390/ijms18040873
- Siggins, R., Hossain, F., Rehman, T., Melvan, J., Zhang, P., & Welsh, D. (2014). Cigarette smoke alters the hematopoietic stem cell niche. Medical Sciences (Basel, Switzerland), 2(1), 37–50. https://doi.org/10.3390/medsci2010037
- Singh, K. P., Wyman, A., Casado, F. L., Garrett, R. W., & Gasiewicz, T. A. (2009). Treatment of mice with the Ah receptor agonist and human carcinogen dioxin results in altered numbers and function of hematopoietic stem cells. Carcinogenesis, 30(1), 11–19. https://doi.org/10.1093/carcin/bgn224
- Smaaland, R., Sothern, R. B., Laerum, O. D., & Abrahamsen, J. F. (2002). Rhythms in human bone marrow and blood cells. Chronobiology International, 19(1), 101–127. https://doi.org/10.1081/CBI-120002594
- Snyder, R., & Hedli, C. C. (1996). An overview of benzene metabolism. Environmental Health Perspectives, 104(Suppl 6), 1165–1171. https://doi.org/10.1289/ehp.96104s61165
- Steur, L. M. H., Kaspers, G. J. L., Van Someren, E. J. W., Van Eijkelenburg, N. K. A., Van der Sluis, I. M., Dors, N., Van den Bos, C., Tissing, W. J. E., Grootenhuis, M. A., & Van Litsenburg, R. R. L. (2020). Sleep–wake rhythm disruption is associated with cancer-related fatigue in pediatric acute lymphoblastic leukemia. Sleep, 43(6), 1–9. https://doi.org/10.1093/sleep/zsz320
- Sulli, G., Lam, M. T. Y., & Panda, S. (2019). Interplay between circadian clock and cancer: New frontiers for cancer treatment. Trends in Cancer, 5(8), 475–494. https://doi.org/10.1016/j.trecan.2019.07.002
- Sun, C.-m., Huang, S.-f., Zeng, J.-m., Liu, D.-b., Xiao, Q., Tian, W.-j., Zhu, X.-d., Huang, Z.-g., & Feng, W.-l. (2010). Per2 inhibits K562 leukemia cell growth in vitro and in vivo through cell cycle arrest and apoptosis induction. Pathology Oncology Research: POR, 16(3), 403–411. https://doi.org/10.1007/s12253-009-9227-0
- Sung, H., Ferlay, J., Siegel, R. L., Laversanne, M., Soerjomataram, I., Jemal, A., & Bray, F. (2021). Global cancer statistics 2020: GLOBOCAN estimates of incidence and mortality worldwide for 36 cancers in 185 countries. CA: A cancer journal for clinicians, 71(3), 209–249. https://doi.org/10.3322/caac.21660
- Swerdlow, S. H., Campo, E., Pileri, S. A., Harris, N. L., Stein, H., Siebert, R., Advani, R., Ghielmini, M., Salles, G. A., Zelenetz, A. D., & Jaffe, E. S. (2016). The 2016 revision of the World Health Organization classification of lymphoid neoplasms. Blood, 127(20), 2375–2390. https://doi.org/10.1182/blood-2016-01-643569
- Tang, J., Wennerberg, K., & Aittokallio, T. (2015). What is synergy? The Saariselkä agreement revisited. Frontiers in Pharmacology, 6, 181. https://doi.org/10.3389/fphar.2015.00181
- Taniguchi, H., Fernández, A. F., Setién, F., Ropero, S., Ballestar, E., Villanueva, A., Yamamoto, H., Imai, K., Shinomura, Y., & Esteller, M. (2009). Epigenetic inactivation of the circadian clock gene BMAL1 in hematologic malignancies. Cancer Research, 69(21), 8447–8454. https://doi.org/10.1158/0008-5472.CAN-09-0551
- Terasaka, H., Kadoma, Y., Sakagami, H., & Fujisawa, S. (2005). Cytotoxicity and apoptosis-inducing activity of bisphenol A and hydroquinone in HL-60 cells. Anticancer Research, 25(3B), 2241–2247. Retrieved from http://www.ncbi.nlm.nih.gov/pubmed/16158970
- Tischkau, S. A. (2011). AHR and the circadian clock. In The AH receptor in biology and toxicology (pp. 511–522). John Wiley & Sons, Inc. https://doi.org/10.1002/9781118140574.ch35
- Tomita, T., Kurita, R., & Onishi, Y. (2017). Epigenetic regulation of the circadian clock: Role of 5-aza-2′-deoxycytidine. Bioscience Reports, 37(3), 1–10. https://doi.org/10.1042/BSR20170053
- Tsutsumi, A., Ozaki, M., Chubachi, S., Irie, H., Sato, M., Kameyama, N., Sasaki, M., Ishii, M., Hegab, A. E., Betsuyaku, T., & Fukunaga, K. (2020). Exposure to cigarette smoke enhances the stemness of alveolar type 2 Cells. American Journal of Respiratory Cell and Molecular Biology, 63(3), 293–305. https://doi.org/10.1165/rcmb.2019-0188OC
- Vasu, V. T., Cross, C. E., & Gohil, K. (2009). Nr1d1, an important circadian pathway regulatory gene, is suppressed by cigarette smoke in murine lungs. Integrative Cancer Therapies, 8(4), 321–328. https://doi.org/10.1177/1534735409352027
- Verlande, A., & Masri, S. (2019). Circadian clocks and cancer: Timekeeping governs cellular metabolism. Trends in Endocrinology and Metabolism: TEM, 30(7), 445–458. https://doi.org/10.1016/j.tem.2019.05.001
- Vinceti, M., Rothman, K. J., Crespi, C. M., Sterni, A., Cherubini, A., Guerra, L., Maffeis, G., Ferretti, E., Fabbi, S., Teggi, S., Consonni, D., De Girolamo, G., Meggiato, A., Palazzi, G., Paolucci, P., & Malagoli, C. (2012). Leukemia risk in children exposed to benzene and PM10 from vehicular traffic: a case-control study in an Italian population. European Journal of Epidemiology, 27(10), 781–790. https://doi.org/10.1007/s10654-012-9727-1
- Wang, Q., Bai, J., Ning, B., Fan, L., Sun, T., Fang, Y., Wu, J., Li, S., Duan, C., Zhang, Y., Liang, J., & Gao, Z. (2020). Effects of bisphenol A and nanoscale and microscale polystyrene plastic exposure on particle uptake and toxicity in human Caco-2 cells. Chemosphere, 254, 126788. https://doi.org/10.1016/j.chemosphere.2020.126788
- Wang, L.-S., Liu, H.-J., Zhang, J.-H., & Wu, C.-T. (2002). Purging effect of dibutyl phthalate on leukemia cells involves FAS independent activation of caspase-3/CPP32 protease. Cancer Letters, 186(2), 177–182. https://doi.org/10.1016/S0304-3835(02)00324-5
- Wang, N., Mi, M., Wei, X., & Sun, C. (2020). Circadian clock gene Period2 suppresses human chronic myeloid leukemia cell proliferation. Experimental and Therapeutic Medicine, 20(6), 147. https://doi.org/10.3892/etm.2020.9276
- Wang, Y., Zhou, J., Zhou, S., Fang, G., Li, Y., Qiu, L., … Liu, X. (2011). Promoter methylation status of hPer3 gene in AML patients and the in vitro effect of decitabine on the status. Zhonghua Xue Ye Xue Za Zhi = Zhonghua Xueyexue Zazhi, 32(5), 317–321. Retrieved from http://www.ncbi.nlm.nih.gov/pubmed/21729600
- Ward, M. H., Colt, J. S., Metayer, C., Gunier, R. B., Lubin, J., Crouse, V., Nishioka, M. G., Reynolds, P., & Buffler, P. A. (2009). Residential exposure to polychlorinated biphenyls and organochlorine pesticides and risk of childhood leukemia. Environmental Health Perspectives, 117(6), 1007–1013. https://doi.org/10.1289/ehp.0900583
- Whitehead, T. P., Metayer, C., Wiemels, J. L., Singer, A. W., & Miller, M. D. (2016). Childhood leukemia and primary prevention. Current Problems in Pediatric and Adolescent Health Care, 46(10), 317–352. https://doi.org/10.1016/j.cppeds.2016.08.004
- Wieczerzak, M., Namieśnik, J., & Kudłak, B. (2021). Genotoxicity of selected pharmaceuticals, their binary mixtures, and varying environmental conditions - study with human adenocarcinoma cancer HT29 cell line. Drug and Chemical Toxicology, 44(2), 113–123. https://doi.org/10.1080/01480545.2018.1529783
- Winters, A. C., & Bernt, K. M. (2017). MLL-Rearranged Leukemias-An update on science and clinical approaches. Frontiers in Pediatrics, 5(February), 4–13. https://doi.org/10.3389/fped.2017.00004
- Wu, C., Yang, K., Pei, X., Tang, A., Wang, F., & Wang, L. (1995). Bone marrow purging with dibutyl phthalate—Experimental basis and a preliminary clinical application. Leukemia Research, 19(8), 557–560. https://doi.org/10.1016/0145-2126(95)00038-P
- Xu, C.-X., Krager, S. L., Liao, D.-F., & Tischkau, S. A. (2010). Disruption of CLOCK-BMAL1 transcriptional activity is responsible for aryl hydrocarbon receptor-mediated regulation of Period1 gene. Toxicological Sciences: An Official Journal of the Society of Toxicology, 115(1), 98–108. https://doi.org/10.1093/toxsci/kfq022
- Yang, Y., Dong, F., Liu, X., Xu, J., Wu, X., & Zheng, Y. (2019a). Dysregulation of circadian rhythm in zebrafish (Danio rerio) by thifluzamide: Involvement of positive and negative regulators. Chemosphere, 235, 280–287. https://doi.org/10.1016/j.chemosphere.2019.06.153
- Yang, Y., Dong, F., Liu, X., Xu, J., Wu, X., & Zheng, Y. (2019b). Flutolanil affects circadian rhythm in zebrafish (Danio rerio) by disrupting the positive regulators. Chemosphere, 228, 649–655. https://doi.org/10.1016/j.chemosphere.2019.04.207
- Yang, M.-Y., Lin, P.-M., Hsiao, H.-H., Hsu, J.-F., Lin, H. Y.-H., Hsu, C.-M., Chen, I.-Y., Su, S.-W., Liu, Y.-C., & Lin, S.-F. (2015). Up-regulation of PER3 expression is correlated with better clinical outcome in acute leukemia. Anticancer Research, 35(12), 6615–6622. Retrieved from http://www.ncbi.nlm.nih.gov/pubmed/26637877
- Zarus, G. M., Muianga, C., Hunter, C. M., & Pappas, R. S. (2021). A review of data for quantifying human exposures to micro and nanoplastics and potential health risks. The Science of the Total Environment, 756, 144010. https://doi.org/10.1016/j.scitotenv.2020.144010
- Zhang, S., Li, J., Fan, J., & Wu, X. (2020). Bisphenol A triggers the malignancy of acute myeloid leukemia cells via regulation of IL-4 and IL-6. Journal of Biochemical and Molecular Toxicology, 34(1), e22412. https://doi.org/10.1002/jbt.22412