Abstract
Nanomaterials (NMs) possess many unique properties that are increasingly used in environmental applications. Twenty-six articles in Critical Reviews in Environmental Science and Technology (CREST) from 2018 to 2021were identified that enhance our understanding and provide insight about future research directions with NMs in the environment. The first section focuses on environmental applications of NMs, including sensors to detect contaminants and environmental conditions, novel membrane materials to treat water and wastewater, and nano-enabled remediation of contaminants by adsorption, photocatalytic degradation, and/or disinfection. The second section reports on risks and the fate of NMs in the environment, including mechanisms and models of environmental transport, the role of nanoscale heterogeneities on particle attachment, and contaminant associations and ecotoxicity. The final section discusses research pertaining to emerging applications and ecotoxicity associated with nanosulfur and nanoplastics. This virtual article collection demonstrates that recent nanotechnology advances show great promise for addressing many critical challenges in environmental science and technology. However, many of these studies have been conducted under highly idealized laboratory conditions and still need to be upscaled. Caution is warranted and new approaches are still needed to detect and control the mobility of NMs, and to quantify potential impacts on ecosystems under realistic field conditions.
Graphical abstract
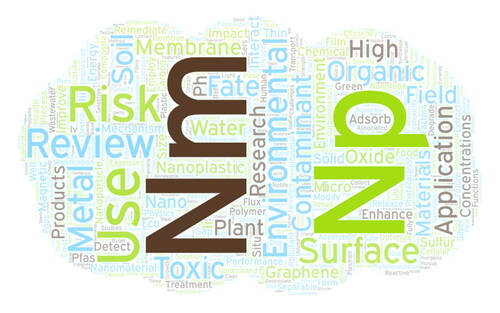
1. Introduction
Nanoparticles (NPs) are particles that are < 100 nm in size in all dimensions and nanomaterials (NMs) are < 100 nm in at least one dimension. Synthesis and naturally occurring NPs are the two main sources through which NMs are being produced. NMs are typically grouped into: (i) metal-based or inorganic NMs, (ii) carbonaceous NMs, (iii) polymer-based NMs, and (iv) composite NMs supported on inert materials (e.g., activated carbon, biochar, clay minerals, biodegradable polymers, and zeolites). NMs possess various physical, chemical, electrical, and/or optical properties that can be exploited in numerous industrial applications, including agriculture, automotive, cosmetics, construction, electronics, environment, food, home appliances, medicine, petroleum, printing, renewable energies, sports and fitness, and textile. Thousands of tons of NMs are currently being produced and utilized each year. For example, the global production of engineered NMs was estimated to be 2.68-3.18 million metric tons in 2010, and this number has increased by 25% annually since then (Younis et al., Citation2018). The global nanotechnology market is likely to exceed 125 billion US$ by 2024 (HTF Market Report, Citation2018).
Most synthesized NMs will eventually be released into the environment with wastewater effluent, sewage sludge, solid wastes, and atmospheric emissions. Furthermore, soils and sediments, water resources, and plants can be directly exposed to NMs during agricultural and environmental applications. For example, NMs are employed as nano-fertilizers, nano-pesticides, and nano-sensors to increase crop yield, quality, and resilience. There is growing interest to employ NMs in environmental remediation due to their large specific surface area, high chemical reactivity, and chemical selectivity. These NMs can be classified based on their functions, as adsorbents, catalysts, and membrane materials.
Exposure to NMs in the environment poses potential health risks to ecosystems and humans that is presently not fully understood or quantified. The risk of NMs depends on their environmental concentrations and associated contaminants, transformation, consumption/uptake, bioaccumulation, and ecotoxicity. A thorough understanding and ability to quantify these factors are therefore needed. For example, transport and fate processes determine the spatial and temporal evolution of concentrations of NMs and associated contaminants in environmental compartments.
Considerable research has been directed to examine applications and risks of NMs in the environment. A special collect of review manuscripts from Critical Reviews in Environmental Science and Technology (CREST) published between 2018 and 2021 was therefore assembled to provide readers with an up-to-date state of the knowledge on this topic, to identify research gaps, and to outline future research directions. This introduction to the special collection is divided into three broad sections: (1) Environmental applications of NMs; (2) Environmental exposure risks and fate of NMs; and (3) Nanosulfur and nanoplastics as emerging NMs.
1.1. Environmental applications of nanomaterials
The rapid development of nanotechnology has led to significant advances in design and synthesis of novel NMs for application and assistance in solving environmental issues. This collection of CREST review papers presents applications of NMs for treatment of contaminants in the environment through adsorption and remediation, photocatalyst, disinfection, and membrane filtration, and detection of contaminants as sensors.
1.1.1. Nano-enabled remediation
Depending on structure and physico-chemical properties, environmental contaminants can adsorb onto surfaces as a result of intermolecular forces such as van der Waals forces, electrostatic attraction, and chemisorption (covalent bonding). NMs have high specific areas that can be functionalized to strongly adsorb contaminants in aqueous or gaseous phases with greater efficiency and rapidity than conventional adsorbents (Adeleye et al., Citation2016).
Per-and poly-fluoroalkyl substances (PFAS) are a group of chemicals that have been used in many commercial and industrial products. PFAS include the sub-group of perfluoroalkyl acids (PFAAs) such as the highly bioaccumulative perfluorooctane sulfonate (PFOS) and perfluorooctane carboxylic acids (PFOA), and otherwise among the PFAAs categorized as the "forever chemicals" due to their high environmental and chemical stability. Widespread release of PFAS in the environment is of great concern because of their persistence, mobility, toxicity, and bioaccumulation. Liu et al. (Citation2020) evaluated literature on the adsorption of PFOS and PFOA on carbonaceous NMs under various solution chemistry conditions. These PFAAs were readily adsorbed to carbonaceous NMs via hydrophobic and electrostatic interactions, ligand exchange and hydrogen bonding. However, there are potential ecological risks if carbonaceous NMs are released into the environment. The development and application of new carbonaceous NMs such as carbon nanotube sponges and electrodes could mitigate these dangers.
In addition to adsorption, NMs can remove or immobilize contaminations through photodegradation, catalytic degradation, microorganisms (nano-bioremediation), and/or the deployment of electrical fields (electro-nanoremediation). Mukhopadhyay et al. (Citation2021) analyzed publications on nanoremediation approaches to clean up contaminated soil and water via the aforementioned mechanisms in a rapid, safe, and cost-effective manner. Nanoremediation technologies can occur in situ and if properly designed and optimized can eliminate the need for costly excavation and transportation of contaminated soils. In addition, NMs can be regenerated and reused for treatment using separation approaches such as magnetic separation of iron NPs and recovery of metals from spent nanosorbents. Potential drawbacks for field-scale nanomaterial application includes problems with in-situ delivery to the source zone and the stability there, as well as, toxicity, and costs.
NMs have also been combined with other techniques to facilitate remediation. Song et al. (Citation2019) scrutinized articles on the use of NMs for phytoremediation. Phytoremediation employs plants to remediate contaminated soils in a simple, low-cost, and widely adaptable way that is accepted by the public due to its esthetic appearance. NMs can improve phytoremediation by directly removing pollutants, promoting plant growth, and increasing pollutant phytoavailability. However, the use of NMs during phytoremediation is still in the exploratory phase and more research is required to optimize performance and assess potential risks.
Photoreactions can be enhanced in the presence of photocatalysts that generate electron-hole pairs and superoxide radicals to degrade organic contaminants. Photocatalytic reactions are successful approaches to rapidly remove organic contaminants from the environment due to its low cost and environmental compatibility. Heterogeneous photocatalytic nanocomposites are promising NMs for renewable energy generation and degradation of environmental pollutants. Shekofteh-Gohari et al. (Citation2018) critically discussed the design and application of magnetically-separable photocatalyst nanocomposites based on ZnO. Incorporation of photocatalysts in magnetic materials can facilitate recycling using an external magnetic field. Strategies are discussed to modify ZnO with narrow band gap semiconductors, noble metals, organic dyes, and elemental doping to overcome existing challenges from a wide band gap, poor solar-light utilization, rapid recombination of the photoinduced charge carriers, and photocorrosion.
Photocatalytic degradation of toxic organic compounds such as dyes under UV-visible light irradiation by metal and metal oxide NPs has recently attracted considerable attention. Nagajyothi et al. (Citation2020) provided an overview about the use of microbes, plants, and plant extract for green-synthesized metal and metal oxide NPs in photocatalytic degradation. Green synthesis approaches are eco-friendly and cost-effective because they do not require high temperatures, pressures, energy, and toxic chemicals. Industrial application of green synthesized photocatalyst NPs is presently inhibited by low yields, up-scaling challenges, and poor recycling. New insight is provided on the coupling of plasmonic metals on green-synthesized photocatalysts and the localized surface plasmon resonance mechanism for noble metals on metal oxide photocatalysts.
Nano-silver exhibits excellent antimicrobial properties that have been employed in many industrial and commercial applications. Biophysiochemical properties are found to influence the use of nano-silver based materials to replace or enhance disinfection methods for point of use water treatment (Bahcelioglu et al., Citation2021). Nano-silver based materials can achieve high removal efficiencies with less disinfection-by-products, energy requirements, and clogging than conventional approaches. However, more research is needed to better understand factors or combinations that influence their antimicrobial performance.
1.1.2. Membranes
Obaid et al. (Citation2020) synthesized literature on the fabrication and modification of electrospun nanofibers that are employed in thin film composite membranes for forward osmosis applications, such as desalination, wastewater treatment, and food processing. Electrospinning is a cheap, scalable, and simple technique, which produces electrospun mats with low structural parameters and allows for direct paths for salt and water diffusion that greatly enhances membrane performance. NPs and NMs (e.g., multi-walled carbon nanotubes, porous zeolite NPs, titanium dioxide NPs, and graphene oxide) are frequently employed in the fabrication process of thin film composite membranes to create morphologies (nanofibers, nanobelts, nanorods, and nanotubes) with high aspect areas, high surface areas, high mechanical robustness, and uniform pore sizes. More research is required to enhance the mechanical properties, optimize the pore size, and overcome delamination problems with electrospun nanofiber substrates.
Bodzek et al. (Citation2020) dedicated his review to the use of nanotechnology in next generation membranes for desalination and wastewater treatment. A special focus was on the use of nanoporous graphene, graphene oxide, and polymeric/ceramic membranes modified with the use of graphene-based NMs. This was because of their excellent mechanical properties, single-atom layer structure, large specific surface area and wide modification possibilities. Membranes based on NMs achieved higher water/permeate fluxes and revealed unique antifouling and antibacterial properties in comparison to currently used thin film composite membranes of polyamide, which can therefore be used for a wider range of applications. Future research should focus on the improvement of their separation features, determining interaction mechanisms, and overcoming upscaling challenges as well as potential disadvantages from mechanical instability, nanosheets formation, non-uniform distribution of layers and surface damage.
Castro-Muñoz et al. (Citation2021) evaluated the synthesis and properties of graphene materials (such as graphene oxide, reduced graphene oxide, functionalized reduced graphene oxide, and graphene quantum dots) for selective membrane separations. Incorporation of graphene materials into polymer membranes improved their separation performance by enhancing antifouling properties, water flux, thermal features, altering hydrophilicity/hydrophobicity, and creating a higher porosity and mechanical strength. Future advances can be achieved by functionalizing graphene surfaces and/or creating composite materials, although long-term studies are needed to better quantify their performance.
Gontarek-Castro et al. (Citation2021) assessed the state of knowledge on the use of superhydrophobic membranes in membrane distillation applications. Superhydrophobic membranes generally improve membrane stability, enhanced vapor flux, and permeate quality. NPs are used on the surface and in fillings (including graphene and carbon nanotubes) of these membranes to tailor their properties to improve performance. Cheaper filling materials are needed to upscale from the laboratory to commercial applications.
1.1.3. Detection
Persistent organic pollutants (POPs) are a very broad class of substances that are of great concern in the environment because of their presence in global environmental compartments, and bioaccumulation in biota, exposure effects, and toxicity. Wu et al. (2021) critically examined the use of magnetic NPs in conjunction with magnetic solid-phase extraction to determine trace concentrations of POPs (e.g., organochlorine pesticides, polychlorinated biphenyls, pentachlorobenzene, pentachlorophenol, polybrominated diphenyl ethers, perfluorinated compounds, brominated flame retardants, and polycyclic aromatic hydrocarbons) in environmental samples. Advantages of this approach include increased extraction efficiency and reductions in time, reagents, and labor. However, properties of environmental samples impact recovery efficiencies of POPs. Improved performance has been achieved by surface modification of magnetic NPs (with inorganic materials, carbonaceous materials, organic compounds, metal–organic frameworks, biosorbents, or their hybrid forms) to increase the dispersibility, stability, reusability, selectivity, and adsorption capacity. Additional progress can be achieved by novel functionalization of magnetic NPs, optimization and automation of joint extraction and detection techniques.
Surface Enhanced Raman Spectroscopy (SERS) is an emerging analytical tool for detecting environmental pollutants. Sarfo et al. (Citation2019) studied fabrication methods and conductive solid materials that are used to create nanostructures on SERS substrates, including the use of various NPs and NMs to improve analytical performance. Limited field applications of SERS to detect environmental toxicants have been shown, but further investigation is needed to optimize their performance.
Solution pH plays a critical role in many chemical, biological, and biogeochemical processes in the environment. Avolio et al. (Citation2020) evaluated recent developments on solid-state pH sensors. Nano-engineered pH sensing devices have emerged as promising approaches because of their improved sensitivity, response time, flexibility, and ease of fabrication. The greatest success has been achieved using NMs based on metal oxides or polymer composites, whereas use of carbon nanoparticles has been less fruitful.
In addition to sensing pollutants and environmental factors using NMs, it is also important to develop techniques to detect toxic NMs for evaluation of their environmental risks. Majumdar and Keller (Citation2021) rigorously examined the use of omics (e.g., genomics, transcriptomics, proteomics, and metabolomic) and bioinformatic tools to better assess the potential risks of NMs to plants. Application of omic approaches for NMs in agriculture are still in the early stages of development but are expected to identify biomarkers of nanotoxicity under different growth stages and environmental conditions. This information should facilitate the development and design of environmentally-safe NMs for agricultural applications.
1.2. Environmental fate and risks of nanomaterials
Knowledge of the (long-range) transport and fate of NPs in the environment is needed to assess potential risks or to optimize beneficial applications. Many processes and factors that influence the ecotoxicity and migration of NPs are incompletely quantified. The fate and transport of micropollutants are also changed once they are associated with NPs.
1.2.1. Transport
Engineered NPs show potential to enhance the in situ remediation of contaminants in soil and groundwater. However, widespread application of NPs in remediation has been hampered by challenges in accurately delivering them to contaminated sites due to their rapid aggregation and/or retention. An understanding of processes that influence the environmental transport and fate of nanoparticles is critical for optimizing environmental applications and assessing risks. Liu et al. (Citation2020) discussed processes and models influencing and simulating the transport and fate of engineered NPs in porous media. A special focus was on various factors to increase NP mobility in the field by modifying their surface properties using surfactants and/or polymers. The authors note that additional research and new models are needed to determine NP fate under realistic field conditions.
Shen et al. (Citation2020) revealed the critical role of nanoscale roughness and charge heterogeneity on the attachment and detachment of colloids and NPs on surfaces. Nanoscale heterogeneities are ubiquitous on natural surfaces and have a large impact on interaction energies between natural surfaces and NPs, which determine the strength of the adhesive force. Additional research is needed to better characterize and more realistically represent the influence of nanoscale heterogeneities on nanoparticle interactions at different spatial scales.
1.2.2. Contaminant interactions
Micropollutants (such as POPs and additional pesticides, pharmaceuticals, personal care products, antibiotics, hormones, flame retardants, and microplastics) are found at low concentrations in effluent from wastewater treatment plants, surface water bodies, and soils. Besha et al. (Citation2020) uncovered the sorption behavior of micropollutants on high surface area NPs under various solution chemistry conditions (e.g., pH, ionic strength, and natural organic matter). They pointed out that most studies have only considered equilibrium or kinetic sorption of micropollutants by NPs under highly idealized conditions (e.g., high concentrations of individual compounds). However, additional research is needed is assess the full complexity and risks of micropollutant-NP associations in natural systems, including their co-transport, competitive sorption, toxicity, and transformations.
1.2.3. Toxicity
NPs may cause toxicity to microbes and multi-cellular organisms from oxidative stress due to the production of reactive oxygen species during direct contact or dissolution of toxic ions (Abbas et al., Citation2020). Although NPs can have stimulatory effects on plants at low concentrations, they have inhibitory impacts at high concentrations. Phytotoxicity occurs when NPs modify biochemical pathways or up and down-regulate genes involved in cellular functions. The authors propose to use various approaches to test, handle, and treat wastes to minimize negative effects of NPs.
Sun et al. (Citation2021) evaluated the impact of metal NPs on soil health, including soil physical properties, the availability of plant nutrients and heavy metals, and soil biological community. They mentioned that soil biological parameters are more susceptible to metal NPs than soil physical or chemical properties. In particular, they pointed out that additional research is needed to address the impacts of metal NPs and their transformation products on plant nutrient availability, environmental pollutant fate, soil-borne diseases, and antibiotic resistance genes at environmentally-relevant concentrations.
Zhao et al. (Citation2021) summarized and discussed the environmental concentrations and ecotoxicity of four representative NMs (i.e., TiO2 for high production and environmental concentrations, Ag for high production and toxicity, CuO for high toxicity, and graphene for rapidly increasing production). Environmental concentrations for these four NMs are very low in surface water and soil, but higher for sediments, wastewater effluents, and sewage sludges. The ecotoxicity of these NMs in surface water and soil are of low concern, whereas they show higher toxicity in wastewater and sludge. However, laboratory values of ecotoxicity may be reduced under field conditions because of the dependence on other environmental factors.
1.3. Nanosulfur and nanoplastics
Two emerging NMs (nanosulfur and nanoplastics) are demonstrated in this CREST collection. The antimicrobial properties of nanosulfur, environmental applications of the sulfur-based NMs, the toxicity of the nanoplastics, and their interaction with organic contaminants, natural organic matter (NOM), and biomolecules are presented.
1.3.1. Nanosulfur
Nanosulfur has been used as an antimicrobial, adsorbent, catalyst, and fertilizer. Shankar et al. (Citation2021) inspected antimicrobial applications of sulfur NPs. The mechanisms contributing to antimicrobial properties of sulfur NPs are still not yet firmly established. Future research is needed for large-scale production of cheap and abundant sulfur NPs with high stability and functionalities.
Teng et al. (Citation2019) reviewed environmental applications and challenges to use different sizes and forms of sulfur. Electric and magnetic responses of plasmonic sulfur composite NMs can potentially be engineered for applications in mesoscopic physics, nanoscale devices, energy, and environmental remediation and monitoring. Additional research is needed to better understand the mode of action, ecological risks, and environmental fate of nanosulfur and sulfur composite NMs.
1.3.2. Nanoplastics
Extensive use of plastic materials has resulted in the widespread dissemination of microplastics in the environment. Degradation or fragmentation of microplastics can produce higher surface area NPs that exhibit different environmental fate and risks, which are much less well understood or characterized. Besseling et al. (Citation2019) provided a preliminary risk assessment for NPs based upon available data for microplastics. This analysis suggests that NPs could pose a risk to sensitive aquatic organisms in near-shore surface waters. Improved detection approaches are needed to better determine the fate and risks of NPs in the environment.
The impact of micro- and nanoplastics on human health is still not fully resolved. Shi et al. (Citation2021) provided an overview of recent in vitro studies related to their impacts on human health. Micro- and nanoplastics exhibited adverse effects on various cell types through cellular uptake and toxicity of adhering and leaching contaminants. However, the cytotoxicity of micro- and nanoplastics varied with cell type. Toxicity studies are warranted for more environmental relevant concentrations and conditions, and to assess risks at the individual level.
Micro- and nanoplastics have a high affinity for organic contaminants. Zhang and Xu (Citation2020) assessed the sorption/desorption behavior of micro- and nanoplastics for organic contaminants. Interactions of organic contaminants with micro- and nanoplastics change their environmental fate, uptake, bioaccumulation, and risks. Most previous research has been limited to well defined laboratory systems and more long-term research is needed under realistic field conditions.
Micro- and nanoplastics interact with NOM and biomolecules in the environment to form an eco-corona or biofilm layer. Junaid and Wang (Citation2021) scrutinized their interactions with NOM and biomolecules in freshwater environments. These interactions are controlled by surface properties and concentrations of plastics and organics, and the solution chemistry. Eco-corona and biofilm layers on plastics alter their environmental fate, bioreactivity, and toxicity. Field investigations are needed to better quantify the ecological and human implications of organic coatings on plastics.
2. Concluding thoughts
This special CREST collection of review papers illustrates many promising opportunities for the use of NMs in contaminant remediation, membrane materials for water and wastewater treatment, as sensors to detect pollutants and environmental conditions, and to improve plant growth (e.g., nanofertilizers or nanopesticides). In general, these nano-enabled technologies are still in the preliminary stages of development and application. Additional research is needed to extend and optimize laboratory bench-top results to real-world scenarios. For example, controlled delivery of NMs to contaminated source zones in the subsurface is needed to cost-effectively remediate pollutants, but suspensions of NMs frequently exhibit limited mobility because of rapid aggregation or retention. It is possible that natural or engineered surface coatings on NMs could enhance their mobility in the environment, but this may also widely disseminate NMs and associated contaminants. Research is therefore warranted to control NM stability and mobility. Furthermore, the reactivity and selectivity of NMs can be diminished under real environmental conditions by aggregation, retention, chemical reactions, and adsorption of solutes and organics. This implies that the desired performance and risks of NMs will change with aging, but these effects still have not been fully quantified.
The potential impacts of NMs on ecosystem and human health is still not fully known, and this hampers wide-spread environmental applications and public acceptance. It is critical to determine NM concentrations in the environment for risk assessment. Improved analytical techniques are needed to isolate and determine environmentally relevant concentrations of NMs. Mathematical models can also be calibrated and used to simulate the fate of NMs in the environment, but our knowledge of controlling factors, processes, and our ability to characterize surface heterogeneity, aging, and model parameters are still incomplete, especially under field conditions. Standardized approaches to rapidly and economically determine risks of NMs to ecosystems and humans are still needed. It is possible that omics (e.g., genomics, transcriptomics, proteomics, and metabolomic) and bioinformatic tools could be utilized for this purpose, but more research is needed on the individual and population levels.
Disclosure statement
No potential conflict of interest was reported by the authors.
Additional information
Funding
References
- Abbas, Q., Yousaf, B., Ullah, H., Ali, M. U., Ok, Y. S., & Rinklebe, J. (2020). Environmental transformation and nano-toxicity of engineered nano-particles (ENPs) in aquatic and terrestrial organisms. Critical Reviews in Environmental Science and Technology, 50(23), 2523–2559. https://doi.org/10.1080/10643389.2019.1705721
- Adeleye, A. S., Conway, J. R., Garner, K., Huang, Y., Su, Y., & Keller, A. A. (2016). Engineered nanomaterials for water treatment and remediation: Costs, benefits, and applicability. Chemical Engineering Journal and the Biochemical Engineering Journal, 286, 640–662. https://doi.org/10.1016/j.cej.2015.10.105
- Avolio, R., Grozdanov, A., Avella, M., Barton, J., Cocca, M., De Falco, F., Dimitrov, A. T., Errico, M. E., Fanjul-Bolado, P., Gentile, G., Paunovic, P., Ribotti, A., & Magni, P. (2020). Review of pH sensing materials from macro- to nano-scale: Recent developments and examples of seawater applications. Critical Reviews in Environmental Science and Technology, 2020, 1–43. https://doi.org/10.1080/10643389.2020.1843312
- Bahcelioglu, E., Unalan, H. E., & Erguder, T. H. (2021). Silver-based nanomaterials: A critical review on factors affecting water disinfection performance and silver release. Critical Reviews in Environmental Science and Technology, 51(20), 2389–2432. https://doi.org/10.1080/10643389.2020.1784666
- Besha, A. T., Liu, Y., Fang, C., Bekele, D. N., & Naidu, R. (2020). Assessing the interactions between micropollutants and nanoparticles in engineered and natural aquatic environments. Critical Reviews in Environmental Science and Technology, 50(2), 135–215. https://doi.org/10.1080/10643389.2019.1629799
- Besseling, E., Redondo-Hasselerharm, P., Foekema, E. M., & Koelmans, A. A. (2019). Quantifying ecological risks of aquatic micro- and nanoplastic. Critical Reviews in Environmental Science and Technology, 49(1), 32–80. https://doi.org/10.1080/10643389.2018.1531688
- Bodzek, M., Konieczny, K., & Kwiecińska-Mydlak, A. (2020). Nanotechnology in water and wastewater treatment. Graphene – the nanomaterial for next generation of semipermeable membranes. Critical Reviews in Environmental Science and Technology, 50(15), 1515–1579. https://doi.org/10.1080/10643389.2019.1664258
- Castro-Muñoz, R., Cruz-Cruz, A., Alfaro-Sommers, Y., Aranda-Jarillo, L. X., & Gontarek-Castro, E. (2021). Reviewing the recent developments of using graphene-based nanosized materials in membrane separations. Critical Reviews in Environmental Science and Technology, 1–38. https://doi.org/10.1080/10643389.2021.1918509
- Gontarek-Castro, E., Castro-Muñoz, R., & Lieder, M. (2021). New insights of nanomaterials usage toward superhydrophobic membranes for water desalination via membrane distillation: A review. Critical Reviews in Environmental Science and Technology, 1–46. https://doi.org/10.1080/10643389.2021.1877032
- HTF Market Report. (2018). Global nanotechnology market (by component and applications), funding & investment, patent analysis and 27 companies profile & recent developments – Forecast to 2024. Edison.
- Junaid, M., & Wang, J. (2021). Interaction of micro(nano)plastics with extracellular and intracellular biomolecules in the freshwater environment. Critical Reviews in Environmental Science and Technology, 1–25. https://doi.org/10.1080/10643389.2021.2002078
- Liu, G., Zhong, H., Ahmad, Z., Yang, X., & Huo, L. (2020). Transport of engineered nanoparticles in porous media and its enhancement for remediation of contaminated groundwater. Critical Reviews in Environmental Science and Technology, 50(22), 2301–2378. https://doi.org/10.1080/10643389.2019.1694823
- Liu, L., Liu, Y., Gao, B., Ji, R., Li, C., & Wang, S. (2020). Removal of perfluorooctanoic acid (PFOA) and perfluorooctane sulfonate (PFOS) from water by carbonaceous nanomaterials: A review. Critical Reviews in Environmental Science and Technology, 50(22), 2379. https://doi.org/10.1080/10643389.2019.1700751
- Majumdar, S., & Keller, A. A. (2021). Omics to address the opportunities and challenges of nanotechnology in agriculture. Critical Reviews in Environmental Science and Technology, 51(22), 2595–2636. https://doi.org/10.1080/10643389.2020.1785264
- Mukhopadhyay, R., Sarkar, B., Khan, E., Alessi, D. S., Biswas, J. K., Manjaiah, K. M., Eguchi, M., Wu, K. C. W., Yamauchi, Y., & Ok, Y. S. (2021). Nanomaterials for sustainable remediation of chemical contaminants in water and soil. Critical Reviews in Environmental Science and Technology, 1–50. https://doi.org/10.1080/10643389.2021.1886891
- Nagajyothi, P. C., Prabhakar Vattikuti, S. V., Devarayapalli, K. C., Yoo, K., Shim, J., & Sreekanth, T. V. M. (2020). Green synthesis: Photocatalytic degradation of textile dyes using metal and metal oxide nanoparticles-latest trends and advancements. Critical Reviews in Environmental Science and Technology, 50(24), 2617–2723. https://doi.org/10.1080/10643389.2019.1705103
- Obaid, M., Abdelkareem, M. A., Kook, S., Kim, H. Y., Hilal, N., Ghaffour, N., & Kim, I. S. (2020). Breakthroughs in the fabrication of electrospun-nanofiber-supported thin film composite/nanocomposite membranes for the forward osmosis process: a review. Critical Reviews in Environmental Science and Technology, 50(17), 1727–1769. https://doi.org/10.1080/10643389.2019.1672510
- Sarfo, D. K., Izake, E. L., O’Mullane, A. P., & Ayoko, G. A. (2019). Fabrication of nanostructured SERS substrates on conductive solid platforms for environmental application. Critical Reviews in Environmental Science and Technology, 49(14), 1294–1329. https://doi.org/10.1080/10643389.2019.1576468
- Shankar, S., Jaiswal, L., & Rhim, J.-W. (2021). New insight into sulfur nanoparticles: Synthesis and applications. Critical Reviews in Environmental Science and Technology, 51(20), 2329–2356. https://doi.org/10.1080/10643389.2020.1780880
- Shekofteh-Gohari, M., Habibi-Yangjeh, A., Abitorabi, M., & Rouhi, A. (2018). Magnetically separable nanocomposites based on ZnO and their applications in photocatalytic processes: A review. Critical Reviews in Environmental Science and Technology, 48(10-12), 806–857. https://doi.org/10.1080/10643389.2018.1487227
- Shen, C., Jin, Y., Zhuang, J., Li, T., & Xing, B. (2020). Role and importance of surface heterogeneities in transport of particles in saturated porous media. Critical Reviews in Environmental Science and Technology, 50(3), 244–329. https://doi.org/10.1080/10643389.2019.1629800
- Shi, Q., Tang, J., Liu, R., & Wang, L. (2021). Toxicity in vitro reveals potential impacts of microplastics and nanoplastics on human health: A review. Critical Reviews in Environmental Science and Technology, 1–33. https://doi.org/10.1080/10643389.2021.1951528
- Song, B., Xu, P., Chen, M., Tang, W., Zeng, G., Gong, J., Zhang, P., & Ye, S. (2019). Using nanomaterials to facilitate the phytoremediation of contaminated soil. Critical Reviews in Environmental Science and Technology, 49(9), 791–824. https://doi.org/10.1080/10643389.2018.1558891
- Sun, W., Dou, F., Li, C., Ma, X., & Ma, L. Q. (2021). Impacts of metallic nanoparticles and transformed products on soil health. Critical Reviews in Environmental Science and Technology, 51(10), 973–1002. https://doi.org/10.1080/10643389.2020.1740546
- Teng, Y., Zhou, Q., & Gao, P. (2019). Applications and challenges of elemental sulfur, nanosulfur, polymeric sulfur, sulfur composites, and plasmonic nanostructures. Critical Reviews in Environmental Science and Technology, 49(24), 2314–2358. https://doi.org/10.1080/10643389.2019.1609856
- Wu, A., Zhao, X., Wang, J., Tang, Z., Zhao, T., Niu, L., Yu, W., Yang, C., Fang, M., Lv, H., Liu, S., & Wu, F. (2021). Application of solid-phase extraction based on magnetic nanoparticle adsorbents for the analysis of selected persistent organic pollutants in environmental water: a review of recent advances. Critical Reviews in Environmental Science and Technology, 51(1), 44. https://doi.org/10.1080/10643389.2020.1720493
- Younis, S. A., El-Fawal, E. M., & Serp, P. (2018). Nano-wastes and the environment: Potential challenges and opportunities of nano-waste management paradigm for greener nanotechnologies. In C. M. Hussain (Ed.), Handbook of environmental materials management (pp. 1–72). Springer. https://doi.org/10.1007/978-3-319-58538-3_53-1
- Zhang, M., & Xu, L. (2020). Transport of micro- and nanoplastics in the environment: Trojan-Horse effect for organic contaminants. Critical Reviews in Environmental Science and Technology, 1–37. https://doi.org/10.1080/10643389.2020.1845531
- Zhao, J., Lin, M., Wang, Z., Cao, X., & Xing, B. (2021). Engineered nanomaterials in the environment: Are they safe? Critical Reviews in Environmental Science and Technology, 51(14), 1443–1478. https://doi.org/10.1080/10643389.2020.1764279