ABSTRACT
This study was conducted with the aim of synthesising nanoemulsion of apricot kernel oil (AKO-NE) and evaluating its cytotoxic, pro-apoptotic, antioxidant and anti-angiogenic effects. The AKO-NE was synthesised by ultrasound (150 watt for 2 min) using a combination of Tween 20 and 80 as surfactant and ethylene glycol as co surfactant. Antioxidant capacity (ABTS, DPPH scavenging free radicals) and toxicity of AKO-NE (MTT assay) and its effects on the process of apoptosis (Flow cytometry and qPCR for BCL-2, Caspase 3, 7, 9) were evaluated by specialised methods. Then, the effect of AKO-NE on angiogenesis was measured by CAM and qPCR (VEGF and VEGFR) methods. The AKO-NE showed acceptable antioxidant power and selective toxicity effects against lung cancer cells (162.7 µl/mL). The pro-apoptotic effects of AKO-NE were confirmed by increasing the number of SubG1 phase cells in flow cytometry and increasing the expression of Cas-3, 7 and 9 genes and decreasing the expression of BCL-2 gene in qPCR method. Decreased vascular and foetal growth factors in the CAM model as well as decreased expression of VEGF and VEGF-R genes in AKO-NE-treated cells confirmed the inhibitory effects of AKO-NE on angiogenesis. Inhibitory effects of AKO-NE on the growth and proliferation of A549 cancer cells and induction of apoptosis, as well as its effects on reducing angiogenesis, can suggest this nanoemulsion as a useful combination in cancer therapy.
Introduction
Mutations in DNA normally occur in all cells, but many DNA mutations are repaired in normal cells. In some cases, cells are unable to repair the DNA mutation, and this mutation can activate some genes and convert proto-oncogenes to oncogenes, which in turn increases the ability of the cell to proliferate and escape cell death. As a result, the cell achieves high bioavailability and unlimited proliferation that is known as a malignant cell [Citation1]. At first, the cancer cells use the diffusion method to meet their needs, but when they reach a certain size of growth, due to hypoxia, they begin to secrete pro-angiogenesis factors, and thus the angiogenesis of the tumour tissue begins [Citation2]. Angiogenesis in tumour tissue, in addition to the transfer of nutrients and oxygen to the tumour, can cause the transfer of tumour cells to surrounding tissues and metastasise or spread the tumour [Citation3]. One of the features of tumour vessels that paves the way for the treatment of cancer is the difference in the structure of these vessels with normal vessels [Citation4]. In other words, the vessels-forming cells of the tumour are located at long distances from each other, and on the other hand, lymphatic drainage at the tumour site is insufficient, and these reasons provide the possibility of drug delivery to the tumour tissue [Citation5,Citation6]. Such features have led to the replacement of new methods and therapeutic agents for the effective treatment of cancer [Citation5,Citation6]. Replacement of chemical and synthetic compounds with natural therapeutic agents to reduce side effects and transfer these agents to the target site using nanocarriers is a turning point in the treatment of many diseases, including cancer [Citation7].
Natural compounds due to a wide range of active ingredients such as flavonoids, terpenoids, alkaloids, and so on, have anti-tumour, antioxidant, anti-inflammatory, antibacterial, and so on, properties. Some of these compounds are able to inhibit the proliferation and growth of cancer cells by preventing cell proliferation and inducing apoptosis [Citation8].
Apricot (Prunus armeniaca L) belonging to the Rosacea family, is one of the oldest oilseed products that plays an important role in human health. Its kernel oil contains various active ingredients including lipids, polyphenols (phenolic acids, flavonoids and anthocyanins), amygdalin, carotenoids, organic acids and minerals [Citation9] with a wide range of therapeutic effects such as antioxidants [Citation10], anti-tumour [Citation11], antimicrobial [Citation12], anti-inflammatory and liver and heart protective [Citation13,Citation14] activities.
Despite the superiority of bioactive compounds over chemical compounds, due to the reduction of side effects, some limitations such as instability and low solubility in water have prevented the clinical application of these compounds in medicine and treatment.
In order to eliminate the low solubility and efficient delivery of therapeutic agents, the use of nanocarriers with various structures including nanoemulsions, Nanospheres, Nanosuspensions, Nanoshells, Nanocapsules, nanotubes, dendrimers and lipid nanoparticles has been considered by researchers [Citation9]. Nanoemulsions are the most common carriers for transporting molecules with low solubility in water [Citation15]. These colloidal dispersions are prepared from harmless components including oil, water and surfactant and increase the stability and solubility of active compounds [Citation16]. Nanoemulsions have a large surface area, long circulating half-life, surface charge and many other unique properties that make them a good candidate for therapeutic applications. Their nanometre dimensions allow them to pass through the space of the cells that make up the tumour blood vessels, and thus, by being present in the tumour microenvironment, they can exert more therapeutic effects. In addition, nanoemulsions can be used to entrap distinct types of drugs and are designed for specific purposes [Citation17].
In this study, in order to increase the bioavailability and therapeutic effects, apricot kernel oil was encapsulated in nanodroplet form (AKO-NE), and its anti-cancer effects were investigated by focusing on the antioxidant, cytotoxic, pro-apoptosis and angiogenesis effects.
Materials and methods
Material
The requirements of the emulsification including apricot kernel oil, Tween 20 and 80 (T-20 and T-80), ethylene glycol, ABTS, DPPH, potassium per sulphate and ethanol were purchased from Merck Co. The materials needed for cell culture including culture medium (RPMI and DMEM), trypsin enzyme and antibiotics were prepared from Invitrogen. Propidium iodide (PI) and MTT dye, Triton X100 along with DMSO were purchased from Sigma Aldrich. The A459 and HFF cell lines were obtained from Cell Bank of Ferdowsi University of Mashhad, Iran.
Preparation of nanoformulation from apricot kernel oil
To prepare a nanoemulsion of apricot kernel oil, ultrasonic waves with a power of 150 watts (hielscher-UP400-24 kHz, Germany) were used for 2 minutes. First, 1% of the oil was added to 200 mL of the surfactants’ mixture (Tween 20 and 80). Next, 1% of ethylene glycol was added to the mixture and stirred vigorously. Finally, deionised water (300 mL) was added to the oily phase consisting of apricot oil, and surfactant to be sonicated by an ultrasound machine.
Physicochemical properties of AKO-NE
The size of nanoparticles varies depending on the method and compounds used, and this factor affects the biological properties and therapeutic efficacy of the resulting compound [Citation18]. DLS provides comprehensive information on the size and dispersion index of nanoparticles. In order to investigate the nanoparticles using the DLS method, first the nanoemulsion was dispersed at a ratio of 1:100 in deionised distilled water and then analysed at 25°C using particle size analyser (Cordouan – Vasco3, France). TEM images were used to evaluate the morphology and confirm the results of the DLS method. For this purpose, diluted nanoemulsion was sprayed on a special grade and after drying, it was coated with uranium salt and examined under a TEM microscope. The stability of nanoparticles was measured by evaluating the surface charge of the nanoparticles using Zeta seizer systems (CAD – Zeta Compact, France).
Inhibitory power of free radicals by AKO-NE
The antioxidant capacity of nanoemulsion was evaluated using its inhibitory power on ABTS and DPPH free radicals.
Scavenging of ABTS free radicals
First, ABTS solution was prepared and then oxidised using potassium sulphate to produce ABTS free radicals. After 16 hours of incubation at room temperature, the solution containing free radicals to reach absorption of 0.7 at a wavelength of 734 nm was diluted with distilled water. Then, different concentrations of nanoemulsion (500 µL/mL) were prepared and combined with an equal volume of free radicals. After incubation at room temperature for 1 h, the absorbance of the samples was recorded at a wavelength of 734 nm and the amount of inhibition was calculated by the following formula [Citation19]: % ABTS scavenging Power = OD control – sample adsorption/control adsorption × 100
Scavenging of DPPH free radicals
DPPH free radicals were prepared by dissolving 1 mg of DPPH powder in 16.9 mL of 96% ethanol. Then, different dilutions of nanoemulsion (500 µL/mL) were prepared and mixed with an equal volume of DPPH free radicals. After 30 minutes of incubation at 37°C, the adsorption of the samples was measured at 517 nm [Citation20].
% DPPH Scavenging Power = OD control – sample adsorption/control adsorption × 100
MTT assay
Cell viability was assessed by MTT method based on the reduction of tetrazolium bromide salt by the enzyme succinate dehydrogenase of living cells and formation of insoluble formazan crystals. For this purpose, the cells were transferred to the 96-well culture plate and after 24 hours, they were exposed to different concentrations of AKO-NE. Forty-eight hours after treatment, the treatment medium was replaced with 20 μL of MTT solution and transferred to an incubator for reaction. After 4 hours, the MTT medium was drained and 100 μL of DMSO was added to dissolve the formazan crystals, and the adsorption of the samples at 570 nm was evaluated [Citation20,Citation21].
Flow cytometry analysis
In order to examine the cells by flow cytometry assay, first 5 × 105 cells were transferred to 6-well culture plate, after ensuring the connection of cells to the plate floor (24 later), cells with different concentrations of AKO-NE were treated and after 48 hours, they were separated from the bottom of the plate using trypsin/EDTA and centrifuged. The supernatant was drained and the cells were washed with PBS and centrifuged again. Then, 300 μL of PI (0.5 mg/mL PI and 0.2% Triton X100) dye was added to the cells and after 10 minutes of incubation they were analysed by FACScan laser flow cytometer (FACSCalibur, Becton Dickinson, USA) [Citation22,Citation23].
Gene expression assay
In order to perform molecular analysis and evaluate the expression of genes in treated samples compared to untreated cells, the A549 cells were first cultured and treated with different concentrations of AKO-NE. After 48 hours, RNAs of cells were extracted and examined for quantitative evaluations with a nanodrop device and then used as a template for CDNA synthesis. Real-time PCR reaction solution with a volume of 20 μL including cyber Green, CDNA, specific primer () and water was prepared and then analysed using CFX-96 Bio-Rad [Citation24].
Table 1. Sequence of primers.
CAM assay
First, 40 Ross fertilised eggs were purchased, and after disinfection, they were placed in an incubator for 48 hours to form an air chamber. On the second day, a window was made in the eggshell, and after closing it, the eggs were transferred to the incubator for another 6 days. On the eighth day, a gelatin sponge was placed on the chorioallantoic membrane and 10 μL of different treatments were loaded on the sponge and after closing the window, the samples were transferred to the incubator. On the 12th day, the windows in the shell were opened, and then the treatment area was imaged with the stereomicroscope and these images were examined with ImageJ software. Foetal height and weight were also measured with digital callipers and scales [Citation25].
Statistical analysis
SPSS software version 20 was used to analyse the data. For this purpose, the one-way ANOVA and LSD test were used. Data were presented as ±SD.
Results
AKO-NE characterisation
The size and PDI of AKO-NE were evaluated by using DLS technique and these results confirmed the efficiency of the formulation used in the presence of particles with an actual size of 39.16 nm, hydrodynamic diameter of 124.5 nm and dispersion index of 0.25 (). The formed particles are considered as mono-dispersed particles according to the acceptable range for particles with uniform dispersion (0–7) [Citation26]. The TEM results showed the spherical morphology of the particles in addition to confirming the particle size obtained by the DLS method (). The stable nature of nanoemulsions is described in terms of their surface potential, and particles with a surface potential between −30 and −40 mV are in a stable state. Also, some studies have shown that particles with higher zeta potential −25 mV have the necessary energy to repel and prevent agglomeration [Citation27], so AKO-NE with a surface charge of −29.44 mV can be described as stable particles ().
Scavenging activity of AKO-NE for ABTS and DPPH free radicals
The inhibitory effect of AKO-NE on ABTS and DPPH free radicals is shown in . Comparing the inhibition rate of free radicals by AKO-NE shows a higher inhibitory effect of AKO-NE on DPPH free radicals. The median concentration of AKO-NE in DPPH free radical scavenging is about 500 μL/mL, while at this concentration, only about 29.49% of ABTS free radicals are inhibited. In addition, both diagrams show the concentration-dependent inhibitory effect of AKO-NE on free radicals.
AKO-NE Cytotoxicity
Comparing the toxicity of AKO-NE against normal (HFF) and cancer (A549) cells shows that AKO-NE has a greater inhibitory effect on cancer cells. As shown in , at concentrations above 100 μL/mL, cancer cells are highly inhibited, while at concentrations of 200 μL/mL, no inhibitory effect is applied to normal cells. These results show the selective toxicity effect of AKO-NE on cancer cells and its non-toxicity at similar concentrations on normal cells.
Cell cycle analysis by flow cytometery
As the results of flow cytometry show, the rate of apoptotic cells in the control sample is about 7.8% (SubG1 phase). In nanoparticle-treated samples, the amount of SubG1, which indicates apoptotic cells, increased to 37.2%, 59.1% and 71.8% at concentrations of 140, 160 and 180 μL/mL, respectively, indicating the pro-apoptotic effect of the AKO-NE (). Examination of the cell cycle () in treated cells in comparison with control shows a decrease in the percentage of cells present in different phases of the cell cycle (G1, S, G2-M). As can be seen, cell arrest is observed only in the SubG1 phase, indicating the occurrence of apoptosis in cells treated with AKO-NE.
Figure 4. Flow cytometric diagram to investigate the occurrence of apoptosis in AKO-NE-treated cells. In section (a) only the cells in the SubG1 phase are shown. Section b shows the different phases (SubG1, G1, S and G2-M) of the cell cycle (b). An increase in the percentage of SubG1 phase cells is shown in both graphs.
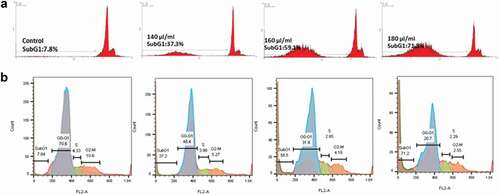
Altered expression of genes involved in apoptosis
Expression analysis of genes involved in apoptosis showed that in cells treated with AKO-NE, the expression of BCL2 gene in both concentrations was significantly reduced, while Cas-9 as a gene involved in the internal pathway of apoptosis and Cas-3 and 7 as genes involved in the common pathway of apoptosis showed a significant increase. The results of this study show the pro-apoptotic effects of AKO-NE by activating genes involved in the internal apoptosis pathway and inhibiting the Bcl-2 gene as an apoptosis inhibitory gene.
Effect of AKO-NE on angiogenesis
CAM assay
Angiogenesis alterations in the CAM model were evaluated qualitatively and quantitatively. The results of stereomicroscope images showed that the rate of blood vessels decreases dramatically with increasing AKO-NE concentration (). In evaluating the number and length of blood vessels, no significant difference in the mean of the indices was observed between the control and laboratory control groups, while a significant (**P < 0.01, ***P < 0.001) decrease was reported in all three concentrations treated with AKO-NE ( and ). Examination of embryonic growth factors showed no significant difference in both indicators (embryo height and weight) between control, laboratory control and treated samples with concentrations of 125 and 250 μL/mL of AKO-NE, and only in concentration of 500 μL/mL, foetal height and weight showed a significant decrease ( and ).
VEGF and VEGF-R genes analysis
The VEGF gene and its receptor (VEGFR) are the most important genes involved in angiogenesis. As shown in , the AKO-NE significantly reduced VEFR expression at both concentrations, while inhibiting VEGF expression at only 160 μL/mL. In general, the anti-angiogenic effects of AKO-NE can be confirmed by the results of CAM test and its effect on the expression of angiogenic genes.
Discussion
Nanoemulsions are colloidal dispersions that have nanometre dimensions and are formed by mixing two immiscible liquids such as water and oil. Surfactants, as active surface molecules, have a hydrophilic and lipophilic component (amphiphilic property) and therefore allow the mixing of immiscible two-phase and reduce interfacial stress [Citation28]. Co-surfactants also act as an adjunct to surfactants and facilitate the preparation of nanoparticles. Co-surfactants are actually active amphiphilic molecules, but due to the small size of their polar heads, they are not able to stabilise an emulsion alone [Citation29]. In addition to the compounds used in the synthesis of nanoparticles, synthesis methods can also affect the stability and other properties of the formulations [Citation30]. In general, the synthesis of nanoemulsions is based on two different methods, including low-energy and high-energy emulsification [Citation31]. In low-energy methods, stable thermodynamic nanoparticles are formed without external energy input [Citation31], while in high-energy methods, the required energy is supplied by high-energy mechanical devices, such as high-pressure homogenisers, microfilzers and sonicates [Citation29].
To the best of our knowledge, this is the first study encapsulating apricot kernel oil by utilising a hybrid composition of tween-80/tween-20 surfactants and ethylene glycol as a co-surfactant, which was studied to investigate the anticancer potential of apricot kernel oil on A546 cancer cell line. The ultrasonic method and the combination of two non-ionic surfactants (T-20 and T-80) with ethylene glycol as a co-surfactant were used for synthesis of AKO-NE (Spherical particles with Ps: 39.44 nm, PDI: 0.25 and ζP: −29.44). In previous studies, various methods have been used to synthesise nanoemulsions. For example, we can refer to the synthesis of peppermint oil by high-pressure homogenisation method [Citation32], fish oil by microfluidisation method [Citation33], ibuprofen nanoemulsion by spontaneous synthesis [Citation34] method and synthesis of nanoemulsions of cherry seed oil [Citation35], cinnamon [Citation36], eucalyptus [Citation37] and Cuminum cyminum essential oil [Citation38] by ultrasonic method which is comparable to the present study.
In high-energy methods, in addition to the synthesis method, the amount of energy used also affects the properties of nanoparticles. The particle size analysis of nanoemulsion of cherry kernel oil (SCPO-NE) after 14 minutes of ultrasound with 18 watts of power reported the formation of nanoparticles with a size of 36.5 nm and PDI of about 0.4 [Citation35], which has a similar size and higher PDI compared to AKO-NE that was sonicated at 150 watts for 2 minutes. In another study conducted in 2019, nanoemulsion of dill essential oil in nanometre dimensions was synthesised using waves with a power of 200 watts for 30 minutes [Citation39], and compared to the present study, waves with higher power and more time have been used.
In a study conducted by Kentish et al., ultrasonic method and non-ionic surfactant (Tween 40) were used to synthesise a nanoemulsion of flaxseed oil with a size of 70 nm [Citation40]. Due to the smaller size of AKO-NE, the efficiency of the formulation containing the combined surfactants of T-20 and 80 against T-40 can be confirmed. The use of T-20 and T-80 similar to the present study has been reported in the nanoformulation of cherry kernel and clove sprout oils, while previous studies have usually used only one surfactant such as T-20 and T-80 for encapsulating basil and black seed oil, respectively [Citation41].
Nanoemulsions due to some properties such as large surface area, surface load, long half-life in blood circulation, and so on, have a wide range of therapeutic uses. Some of the advantages of nanoemulsions include increasing drug absorption due to increasing the level and improving the bioavailability of the drug [Citation42], protecting the drug against oxidative damage [Citation43], adjusting the droplet size of nanoemulsions to increase their penetration into various tissues [Citation44]. The effect of EPR caused by irregular and heterogeneous characteristics of tumour vessels and instability of lymphatic vessels and insufficient discharge of substances from the tumour growth medium increases the penetration of nanocarriers and their presence time to the microenvironment of tumour and improves their effectiveness [Citation16]. Hydrophobic drugs can exert their therapeutic effect on tumour tissue by using the benefits of the EPR effect by passive targeting [Citation45]. In this study, apricot kernel oil (as a hydrophobic compound) was used due to its therapeutic properties for the synthesis of nanoemulsions and its toxicity, antioxidant, pro-apoptotic and anti-angiogenic effects were investigated. Evaluation of the inhibition capacity of ABTS (above 1000 µl/mL) and DPPH (500 µl/mL) free radicals by AKO-NE confirmed the medium antioxidant power of AKO-NE (). A review of studies conducted in 2018 and 2019 regarding the antioxidant effect of nanoemulsions synthesised from natural compounds and vegetable oils shows acceptable antioxidant effects in relation to various formulations. For example, propolis nanoemulsion showed its inhibitory effect on DPPH and ABTS free radicals with IC50 of about 0.023 and 0.004 μg/mL [Citation46]. In another study, the median concentrations of cumin essential oil nanoemulsions on DPPH and ABTS free radicals were reported to be 125 and 31.25 μg/mL, respectively, which in comparison with AKO-NE shows higher inhibitory effects [Citation47]. The inhibitory effect of flaxseed oil nanoemulsion on ABTS and DPPH free radicals with moderate concentrations of 350 and 235 μg/mL, respectively, [Citation48] was confirmed in a study in 2020, which is comparable to the present study. In other studies, the inhibitory effect of nanoemulsions prepared from tomato extract [Citation49], red raspberry seed oil [Citation50], cumin [Citation51] and neem oil [Citation52] was evaluated against free radicals ABTS and DPPH, which is comparable to the results of the present study.
The toxicity of AKO-NE was confirmed 48 hours after treatment with a median concentration of 162.7 μL/mL against A549 cancer cells, while at the same concentration, no toxicity effect was reported against HFF cells (). The pro-apoptotic effects of AKO-NE were confirmed by increasing the number of SubG1 phase cells in flow cytometry, which shows apoptotic cells (). Examination of the genes involved in this process showed that the AKO-NE exerts its effect on inhibiting the Bcl-2 gene and regulating the expression of cas9, 3 and 7 pro-apoptotic genes, and these results indicate the activation of the intrinsic apoptotic pathway ().
In a 2020 study, the toxicity of naringenin nanoemulsion (NAR-NE) on a549 cells was evaluated and 19.28 μg/mL was reported as the median concentration. The results of this study showed cell cycle arrest in G2/M and SubG1 phases [Citation53], while the pro-apoptotic effects of AKO-NE were confirmed only by cell cycle arrest in subg1 phase. In addition, similar to the present study, it was shown that NAR-NE is able to show its inductive effect on apoptosis by increasing the expression of caspase-3 gene and inhibitory effects on bcl-2 gene [Citation53]. In another study in 2021, the effect of chlorophyll NE on the expression of genes involved in apoptosis in melanoma A375 cells was investigated and the results, showed an increase in the expression of caspase 3, 8 and 9 genes and a decrease in BCL-2 expression [Citation54]. Similarly, in the present study, increased expression of caspase genes and decreased bcl-2 were also confirmed.
Nanoemulsion technology is currently used for targeted delivery of angiogenesis inhibitors to reduce associated toxicity and increase therapeutic efficacy. In this study, the anti-angiogenic effects of AKO-NE were evaluated by CAM and QPCR methods. Reduction of mean length and number of blood vessel branches, reduction of foetal growth factors in CAM model as well as decreased expression of VEGF and VEGFR genes in AKO-NE-treated cells showed inhibitory effects of AKO-NE on angiogenesis (). Similar findings were observed by others [Citation55]. In a study conducted in 2019, the effects of Carvacrol Nanoemulsion (C-NE) on angiogenesis were investigated, and the results showed that C-NE is able to inhibit angiogenesis in in-vitro conditions by reducing the expression of COX-2, VEGF and CD31 genes [Citation56]. Evaluation of anti-angiogenic effects of betulin nanoemulsion (BetNE) interfered by exhibiting a decreased expression of VEGF gene in treated chicken chorioallantoic membrane samples [Citation57]. In another study, the cam method was used to evaluate the angiogenic effect of nanoemulsion of lemon essential oil, which showed a reduced number and length of blood vessels [Citation58].
Conclusion
In this study, spherical nanoparticles with a diameter of less than 50 nm were formed with great stability and uniform distribution. AKO-NE with moderate antioxidant power had selective toxicity effects on cancer cells. On the other hand, the induced effects of AKO-NE on the apoptosis process were confirmed by decreasing the expression of Bcl-2 gene, increasing the expression of caspase genes and increasing the number of SubG1 phase cells. The anti-angiogenic effects of this compound were also confirmed by CAM and qPCR methods. In general, the results show that the synthesised nanoparticles are able to inhibit lung cancer cells with different strategies, so this nanoemulsion can be used as an effective compound in lung cancer chemotherapy.
Disclosure statement
No potential conflict of interest was reported by the author(s).
References
- Balabhaskar R, Kumar A R, S S, et al. Potential natural products with anticancer properties and their applications. Asian J Pharm Clin Res. 2019:27–33. doi:10.22159/ajpcr.2019.v12i5.32817
- Teleanu RI, Chircov C, Grumezescu AM, et al. Tumor angiogenesis and anti-angiogenic strategies for cancer treatment. J Clin Med. 2020;9(1):84.
- Lugano R, Ramachandran M, Dimberg A. Tumor angiogenesis: causes, consequences, challenges and opportunities. Cell Mol Life Sci. 2020;77(9):1745–1770.
- Baban DF, Seymour LW. Control of tumour vascular permeability. Adv Drug Deliv Rev. 1998;34(1):109–119.
- Hobbs SK, Monsky WL, Yuan F, et al. Regulation of transport pathways in tumor vessels: role of tumor type and microenvironment. Proc Nat Acad Sci. 1998;95(8):4607–4612.
- Maeda H. The enhanced permeability and retention (EPR) effect in tumor vasculature: the key role of tumor-selective macromolecular drug targeting. Adv Enzyme Regul. 2001;41(1):189–207.
- Seca AM, Pinto DC. Plant secondary metabolites as anticancer agents: successes in clinical trials and therapeutic application. Int J Mol Sci. 2018;19(1):263.
- Seelinger M, Popescu R, Giessrigl B, et al. Methanol extract of the ethnopharmaceutical remedy Smilax spinosa exhibits anti-neoplastic activity. Int J Oncol. 2012;41(3):1164–1172.
- Hrichi S, Rigano F, Chaabane-Banaoues R, et al. Identification of fatty acid, lipid and polyphenol compounds from Prunus armeniaca L. Kernel Extracts. Foods. 2020;9(7): 896.
- Gomaa EZ. In vitro antioxidant, antimicrobial, and antitumor activities of bitter almond and sweet apricot (Prunus armeniaca L.) kernels. Food Sci Biotechnol. 2013;22(2):455–463.
- Cassiem W, de Kock M. The anti-proliferative effect of apricot and peach kernel extracts on human colon cancer cells in vitro. BMC Complement Altern Med. 2019;19(1):1–12.
- Yiğit D, Yiğit N, Mavi A. Antioxidant and antimicrobial activities of bitter and sweet apricot (Prunus armeniaca L.) kernels. Braz J Med Biol Res. 2009;42(4):346–352.
- Yurt B, Celik I. Hepatoprotective effect and antioxidant role of sun, sulphited-dried apricot (Prunus armeniaca L.) and its kernel against ethanol-induced oxidative stress in rats. Food Chem Toxicol. 2011;49(2):508–513.
- Erdogan-Orhan I, Kartal M. Insights into research on phytochemistry and biological activities of Prunus armeniaca L. (apricot). Food Res Int. 2011;44(5):1238–1243.
- Ganta S, Talekar M, Singh A, et al. Nanoemulsions in translational research—opportunities and challenges in targeted cancer therapy. Aaps Pharmscitech. 2014;15(3):694–708.
- Sánchez-López E, Guerra M, Dias-Ferreira J, et al. Current applications of nanoemulsions in cancer therapeutics. Nanomaterials. 2019;9(6):821.
- Tiwari S, Tan Y-M, Amiji M. Preparation and in vitro characterization of multifunctional nanoemulsions for simultaneous MR imaging and targeted drug delivery. J Biomed Nanotechnol. 2006;2(3–4):217–224.
- Qian C, McClements DJ. Formation of nanoemulsions stabilized by model food-grade emulsifiers using high-pressure homogenization: factors affecting particle size. Food Hydrocoll. 2011;25(5):1000–1008.
- Fazelifar P, Tabrizi MH, Rafiee A. The Arachis hypogaea essential oil nanoemulsion as an efficient safe apoptosis inducer in human lung cancer cells (A549). Nutr Cancer. 2021;73(6):1059–1067.
- Khatamian N, Soltani M, Shadan B, et al. Pinus morrisonicola needles essential oil nanoemulsions as a novel strong antioxidant and anticancer agent. United Kingdom: Inorganic and Nano-Metal Chemistry; 2021. p. 1–9.
- Soltani M, Parivar K, Baharara J, et al. Putative mechanism for apoptosis-inducing properties of crude saponin isolated from sea cucumber (Holothuria leucospilota) as an antioxidant compound. Iran J Basic Med Sci. 2015;18(2):180.
- Alhajamee M, Marai K, Al Abbas S.M.N, et al. Co-encapsulation of curcumin and tamoxifen in lipid-chitosan hybrid nanoparticles for cancer therapy. United Kingdom: Materials Technology; 2021. p. 1–12.
- Tavakkol Afshari HS, Homayouni Tabrizi M, Ardalan T, et al. Anethum Graveolens Essential Oil Nanoemulsions (AGEO-NE) as an exclusive apoptotic inducer in human lung adenocarcinoma (A549) Cells. Nutr Cancer. 2022; 74(4):1411–1419.
- Javanshir A, et al. The antioxidant and anticancer potential of Ricinus communis L. essential oil nanoemulsions. J Food Meas Charact. 2020;14:1356–1365.
- Keykhasalar R, et al. The apoptotic, cytotoxic, and antiangiogenic impact of Linum usitatissimum seed essential oil nanoemulsions on the human ovarian cancer cell line A2780. Nutr Cancer. 2021;73:2388–2396.
- Baboota S, Shakeel F, Ahuja A, et al. Design, development and evaluation of novel nanoemulsion formulations for transdermal potential of celecoxib. Acta Pharm. 2007;57(3):315–332.
- Che Marzuki NH, Wahab RA, Abdul Hamid M. An overview of nanoemulsion: concepts of development and cosmeceutical applications. Biotechnol Biotechnol Equip. 2019;33(1):779–797.
- Kralova I, Sjöblom J. Surfactants used in food industry: a review. J Dispers Sci Technol. 2009;30(9):1363–1383.
- Pavoni L, Perinelli DR, Bonacucina G, et al. An overview of micro-and nanoemulsions as vehicles for essential oils: formulation, preparation and stability. Nanomaterials. 2020;10(1):135.
- Choudhury H, et al. Nanoemulsions as effective carriers for the treatment of lung cancer, in Nanotechnology-Based Targeted Drug Delivery Systems for Lung Cancer. Elsevier. 2019;217–247.
- Choudhury H, Gorain B, Chatterjee B, et al. Pharmacokinetic and pharmacodynamic features of nanoemulsion following oral, intravenous, topical and nasal route. Curr Pharm Des. 2017;23(17):2504–2531.
- Liang R, Xu S, Shoemaker CF, et al. Physical and antimicrobial properties of peppermint oil nanoemulsions. J Agric Food Chem. 2012;60(30):7548–7555.
- García-Márquez E, Higuera-Ciapara I, Espinosa-Andrews H. Design of fish oil-in-water nanoemulsion by microfluidization. Vol. 40. Innovative Food Science & Emerging Technologies, Netherlands; 2017. p. 87–91.
- Zhao T, Maniglio D, Chen J, et al. Design and optimization of self-nanoemulsifying formulations for lipophilic drugs. Nanotechnology. 2015;26(12):125102.
- Maragheh AD, Tabrizi MH, Karimi E, et al. Producing the sour cherry pit oil nanoemulsion and evaluation of its anti-cancer effects on both breast cancer murine model and MCF-7 cell line. J Microencapsul. 2019;36(4):399–409.
- Sugumar S, Ghosh V, Nirmala MJ, et al. Ultrasonic emulsification of eucalyptus oil nanoemulsion: antibacterial activity against Staphylococcus aureus and wound healing activity in Wistar rats. Ultrason Sonochem. 2014;21(3):1044–1049.
- Ghosh V, Saranya S, Mukherjee A, et al. Cinnamon oil nanoemulsion formulation by ultrasonic emulsification: investigation of its bactericidal activity. J Nanosci Nanotechnol. 2013;13(1):114–122.
- Nirmala MJ, Durai L, Rao KA, et al. Ultrasonic nanoemulsification of Cuminum cyminum essential oil and its applications in medicine. Int J Nanomedicine. 2020;15:795.
- Tavakkol Afshari HS, Homayouni Tabrizi M, Ardalan T. Evaluation of antioxidant and anticancer effects of nanoemulsions prepared using dill essential oil. J Arak Uni Med Sci. 2019;22(4):40–51.
- Kentish S, Wooster TJ, Ashokkumar M, et al. The use of ultrasonics for nanoemulsion preparation. Innovative Food Sci Emerg Technol. 2008;9(2):170–175.
- Periasamy VS, Athinarayanan J, Alshatwi AA. Anticancer activity of an ultrasonic nanoemulsion formulation of Nigella sativa L. essential oil on human breast cancer cells. Ultrason Sonochem. 2016;31:449–455.
- Khan I, de Sousa JR, Araujo MTF, et al. In vitro and in vivo antitumor potential of carvacrol nanoemulsion against human lung adenocarcinoma A549 cells via mitochondrial mediated apoptosis. Sci Rep. 2018;8(1):1–15.
- Singh Y, Meher JG, Raval K, et al. Nanoemulsion: concepts, development and applications in drug delivery. J Control Release. 2017;252:28–49.
- Hwang YY, Ramalingam K, Bienek DR, et al. Antimicrobial activity of nanoemulsion in combination with cetylpyridinium chloride in multidrug-resistant Acinetobacter baumannii. Antimicrob Agents Chemother. 2013;57(8):3568–3575.
- Maeda H, Wu J, Sawa T, et al. Tumor vascular permeability and the EPR effect in macromolecular therapeutics: a review. J Control Release. 2000;65(1–2):271–284.
- Seibert JB, et al. Development of propolis nanoemulsion with antioxidant and antimicrobial activity for use as a potential natural preservative. Vol. 287. United Kingdom: Food chemistry; 2019. p. 61–67.
- Khatamian N, Ardalan P, Homayouni Tabrizi M. Effect of Carum carvi essential oil nanoemulsion on tubo cancer cells and L929 normal cells and evaluation of antioxidant activity. J Urmia Univ Med Sci. 2019;30(4):315–321.
- Keykhasalar R, Homayouni Tabrizi M, Ardalan P. Antioxidant property and bactericidal activity of Linum usitatissimum seed essential oil nanoemulsion (LSEO-NE) on Staphylococcus aureus. Int J Infect. 2020;7(2). DOI: 10.5812/iji.101639
- Ha TVA, et al. Antioxidant activity and bioaccessibility of size-different nanoemulsions for lycopene-enriched tomato extract. Vol. 178. United Kingdom: Food chemistry; 2015. p. 115–121.
- Gledovic A, et al. Low-energy nanoemulsions as carriers for red raspberry seed oil: formulation approach based on Raman spectroscopy and textural analysis, physicochemical properties, stability and in vitro antioxidant/biological activity. PLoS One. 2020;15(4):e0230993.
- Allahghadri T, Rasooli I, Owlia P, et al. Antimicrobial property, antioxidant capacity, and cytotoxicity of essential oil from cumin produced in Iran. J Food Sci. 2010;75(2):H54–H61.
- Rinaldi F, Hanieh PN, Longhi C, et al. Neem oil nanoemulsions: characterisation and antioxidant activity. J Enzyme Inhib Med Chem. 2017;32(1):1265–1273.
- Md S, Alhakamy NA, Aldawsari HM, et al. Formulation design, statistical optimization, and in vitro evaluation of a naringenin nanoemulsion to enhance apoptotic activity in A549 lung cancer cells. Pharmaceuticals. 2020;13(7):152.
- Liu M-H, Li Y-F, Chen B-H. Preparation of chlorophyll nanoemulsion from pomelo leaves and its inhibition effect on melanoma cells A375. Plants. 2021;10(8):1664.
- Rekha N, Nagesha DK, Rajasree PH, et al. Formulation, characterization and evaluation of anti-inflammatory and anti-angiogenic activities of memecylaene nanoemulsion. J Drug Deliv Therap. 2018;8(5–s):126–131.
- Khan I, Bhardwaj M, Shukla S, et al. Carvacrol encapsulated nanocarrier/nanoemulsion abrogates angiogenesis by downregulating COX-2, VEGF and CD31 in vitro and in vivo in a lung adenocarcinoma model. Colloids Surf B Biointerfaces. 2019;181:612–622.
- Dehelean CA, Feflea S, Gheorgheosu D, et al. Anti-angiogenic and anti-cancer evaluation of betulin nanoemulsion in chicken chorioallantoic membrane and skin carcinoma in Balb/c mice. J Biomed Nanotechnol. 2013;9(4):577–589.
- Yousefian Rad E, Homayouni Tabrizi M, Ardalan P, et al. Citrus Lemon essential oil nanoemulsion (CLEO-NE), a safe cell-depended apoptosis inducer in human A549 lung cancer cells with anti-angiogenic activity. J Microencapsul. 2020;375:1–24.