ABSTRACT
β-BaTi2O5 (β-BT2) nanorods were obtained for the first time by a simple hydrothermal method from a dried gel precursor. The influence of NaOH solution concentration sfrom 0.1 M to 2 M on crystallisation and microstructure was discussed. β-BT2 nanorod with a single-crystal structure exhibited obvious piezoelectric response and piezocatalytic capability for rhodamine B. The degradation of RhB by β-BT2 nanorods under ultrasonic irradiation demonstrated that β-BT2 was a potential piezocatalyst. The proposed strategy can serve as a valuable reference for the synthesis of β-BT2 nanorods and their application in piezocatalysis.
Introduction
As a lead-free ferroelectric Ba-Ti-O based material, barium titanate (BaTiO3, BT) and its doped products or compounds with other substances are widely studied and applied in energy conversion devices [Citation1], energy storage devices [Citation2–4], catalysts [Citation5–8], memory storage devices [Citation9], and other devices due to its structural, electronic, and optical properties [Citation10], excellent piezoelectric and dielectric properties. As another member of the Ba-Ti-O based materials, barium dititanate(BaTi2O5, BT2) only has piezoelectricity along the b-axis, but a higher Curie temperature and a higher dielectric constant near the Curie temperature compared with BT. Akashi et al. reported for the first time in 2003 that BT2 single crystals prepared by floating-zone (FZ) melting method have obvious ferroelectric properties in the b-direction [Citation11]. The single-crystalline BT2 prepared by FZ melting showed a high Curie temperature (Tc, 430–475°C) and the maximum dielectric constant (ε, 20,500) along the b-axis (140 and 70 along the a- and c-axes, respectively), which is several times that of BT single crystal (~7600) [Citation12]. These features make BT2 an ideal filler for the preparation of high-performance piezoelectric composite devices requiring high dielectric constants or being able to work over a wide temperature range [Citation13]. In addition, the accessibility of one-dimensional morphology, nonlinear optical properties and other special properties of BT2 are also attracting the continuous attention of researchers over the past two decades.
To date, the preparation methods of BT2 primarily include the following. The containerless method is usually used to prepare large-size BT2 single-crystal glass [Citation14,Citation15], whereas the sol–gel [Citation16–18] and molten-salt [Citation19,Citation20] methods are used to obtain BT2 powder. BT2 films are used to further study and utilise their nonlinear optical, ferroelectric, or other special properties. Arc melting, laser ablation [Citation21–24], and laser chemical vapour deposition [Citation25–28] are also used to prepare BT2, but the corresponding equipment requirements and costs are daunting. Thus, a simple method to prepare ferroelectric BT2 nanocrystals should be developed urgently. However, few studies have reported on BT2 piezoelectric composite materials and devices. One of the important reasons is that they are thermodynamically unstable and exist only within a very narrow temperature range (1220–1230°C). They also easily decompose into other barium titanate materials [Citation29]. Meanwhile, the anisotropy of piezoelectric and the dielectric properties of BT2 crystals in different crystal planes limit their applications. For BT2 nanorods, the highest piezoelectricity is displayed along its radial direction, whereas very weak piezoelectricity is observed in other directions. Actually, two-dimensional (2D) piezoelectric and ferroelectric materials such as nanorods are very popular in piezocatalysis. Compared with 0D or 3D piezoelectric materials, the high aspect ratio of 2D nanorods enables its easy bending and deformation under mechanical vibration, resulting in a stronger piezoelectric potential for the degradation of organic pollutants or the decomposition of water. To our best knowledge, no study has reported on the application of piezoelectric BT2 nanorods in piezocatalysis.
In 2009, Yu et al. fabricated glass spheres of ferroelectric BT2 from melt through containerless processing. They observed that BT2 has three phase transitions (α, β, and γ) with a temperature rise, i.e. from glass to a metastable α phase at 699°C, then to a metastable β phase at 765°C, and finally to a stable monoclinic γ phase above 827°C. Yu et al. also analysed the α and β structures of metastable BT2 by combined X-ray, neutron diffraction analyses, and computer simulations. They found that the crystalline structure of metastable α and β BT2 were made of noncentrosymmetric TiO5 polyhedra, thereby providing higher potential to yield high dielectric constants than the structure of normal four- or six-coordinate Ti−O polyhedral [Citation14]. However, almost all BT2 single crystal, ceramic, or powder obtained through the above synthesis methods are monoclinic γ-phase structures. Stable single BT2 crystals or powders with α or β phase are rarely reported, and their ferroelectric or piezoelectric properties have not been confirmed.
Herein, β-BT2 nanorods were successfully obtained by a simple hydrothermal method from a dried gel. The influence of NaOH solution concentration from 0.1 M to 2 M on the crystallisation and microstructure of β-BT2 nanorods was discussed. The piezocatalytic performance of BT2 was discovered for the first time through Rhodamine B (RhB) degradation. The piezoelectric response of BT2 nanorods was also detected by piezoelectric force microscopy (PFM).
Experimental
Materials
Barium acetate (Ba(CH3COO)2) and sodium hydroxide (NaOH) were received from Macklin Biochemical Co., Ltd., Shanghai. Tetra-butyl titanate (Ti(OC4H9)4), glacial acetic acid (CH3COOH), and absolute alcohol (C2H5OH) were purchased from Sinopharm Chemical Reagent Co., Ltd., China. All chemicals were analytical grade and used as received without any further treatment.
Preparation of BT2 dried gel
The molar ratio of Ba:Ti was fixed to 1:2. In a typical procedure, 5.1082 g of Ba(CH3COO)2 was dissolved in 20 mL of 36 wt% CH3COOH solution to obtain solution A. Then, 13.6128 g of Ti(OC4H9)4 was dispersed in 20 mL of C2H5OH to obtain solution B. After adding solution B into solution A under vigorous stirring, BT2 gel was obtained after a while. Following ageing for 12 h at room temperature, the BT2 gel was dried in an oven at 80°C and ultimately the dried gel was obtained.
Synthesis of BT2 nanorods
BT2 dried gel (1 g) was dispersed in 50 mL of NaOH solution with setting molar concentration (0.1, 0.3, 0.5, 1, and 2 M) and stirred for 1 h. Then, the suspension was transferred into a Teflon lined autoclave (70 mL) and heated in the oven at 200°C for 24 h. The products were filtered and washed with deionised water and ethanol several times. Finally, the products were oven dried in air at 80°C overnight. The schematic of the synthesis is shown in .
Piezocatalytic test
To test the catalytic performance of β-BT2 nanorods, RhB was used as simulated pollutant, and an ultrasonic cleaner (JP-020, 40 kHz, 120 W, Skymen Cleaning Equipment Shenzhen Co., Ltd.) was used to provide mechanical vibration for the experimental system. β-BT2 nanorods (100 mg) were dispersed in 100 mL of RhB solution (10 mg•L–1) in a beaker. After magnetically stirring the mixed liquid for 30 min without light, the beaker was moved into the ultrasonic cleaner, and then 5 mL of turbid liquid was collected every 20 min and centrifuged (9000 rpm, 3 min) to obtain the supernatant to measure the UV–visible absorption of residual RhB. The degradation rate was calculated by converting absorbance into concentration through a dye standard curve to characterise the catalytic performance of β-BT2 nanorods in water.
Characterisation
The micromorphology and structure of samples were characterised by field-emission scanning electron microscopy (HITACHI S-4800) and high-resolution transmission electron microscopy (HRTEM; JEM-2100 F). The crystalline-phase structure was determined by X-ray powder diffraction (XRD; Bruker D8 Advance Diffractometer). PFM images were obtained using a Bruker Multimode 8 with a Pt-coated conductive tip. UV–vis absorption spectra were measured on a PE Lambda 950 spectrophotometer in diffuse reflectance mode from 200 nm to 800 nm.
Results and discussion
XRD and structural characterisation
shows the XRD patterns and SEM images of the products at 200°C for 24 h as a function of NaOH solution concentration from 0.1 M to 2.0 M. As shown in (a), no obvious diffraction peak was detected at 0.1 M NaOH concentration, indicating that the product may be an amorphous phase. When the NaOH concentration was greater than 0.3 M, all diffraction peaks corresponded with 162369 ICSD in the Inorganic Crystal Structure Database. It had different peak locations compared with the other standard cards, as shown in . Referring to the research of Jianding Yu et al. [Citation14], the product was β-BaTi2O5(β-BT2), different from γ-BaTi2O5(γ-BT2) synthesised by molten-salt method [Citation19,Citation20]. The intensity of the diffraction peaks gradually decreased with increased NaOH concentration. meanwhile, impurities (PDF#83-1876 BaTiO3) began to appear when the NaOH concentration exceeded1 M.
Figure 2. XRD patterns (a) and SEM images ((b) 0.1 M; (c) 0.3 M, (d) 0.5 M, (e) 1.0 M and (f) 2.0 M) of products in different NaOH concentrations.
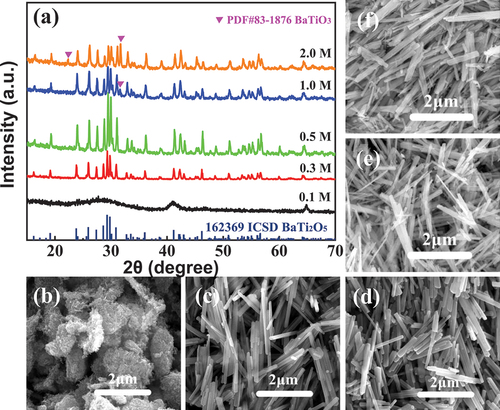
) shows the SEM image of products when the NaOH concentration was 0.1 M. The final product comprised irregular agglomerates with size above 1 μm. With increased concentration to 0.3 M, well-dispersed nanorods formed with a length of 2 μm and a diameter of 75 nm. The aspect ratio was close to 25, as shown in ). The aspect ratio of nanorods was slightly reduced when the NaOH concentration was as high as 0.5 M ()). When the NaOH concentration exceeded 1.0 M ()–(f)), the product morphologies became non-uniform and tended to form nanofiber bundles. Therefore, 0.3 M to 0.5 M was the optimum concentration range to obtain uniform and well-dispersed β-BT2 nanorods with high crystallinity.
The structure of β-BT2 nanorods obtained in 0.5 M NaOH solution was further investigated by HRTEM and the result was shown in . The low-magnification TEM images illustrated that the nanorod structures were similar to the SEM results ()), and the high-magnification TEM images showed that the nanorod was not a hollow or layered structure ()). The HRTEM image ()) showed clear lattice fringes in its edge region, indicating the high crystallinity of BT2. The measured spacing of the most obvious lattice fringe in the HRTEM image was 0.2668 nm and comprised the (013) plane, whose d-spacing was 0.2671 nm in 162,369 ICSD. The selected-area electron diffraction pattern ()) was a periodic arrangement of two-dimensional diffraction points, indicating that the tested sample was single crystal. When [−100] was set as the zone axis, the three diffraction spots closest to the transmission spot were calibrated to (013), (002), (011), respectively, belonging to Pnma(62). The calibration result of the pattern also well matched with 162369 ICSD. Ba, Ti, and O were uniformly distributed in each nanorod according to the EDX elemental-mapping images of the products with individual elements of Ba, Ti, and O, as shown in . Ti(OC4H9)4 is known to hydrolyse to Ti(OH)4 upon encountering water. However, two reaction processes occur in the CH3COOH (HAc) solution system, as shown in Eqs. (1) and (2):
Figure 4. (a)–(c) HRTEM images (inset is the corresponding FFT image), and (d)–(g) corresponding EDX elemental mappings of β-BT2 nanorods synthesised in 0.5 M NaOH solution.
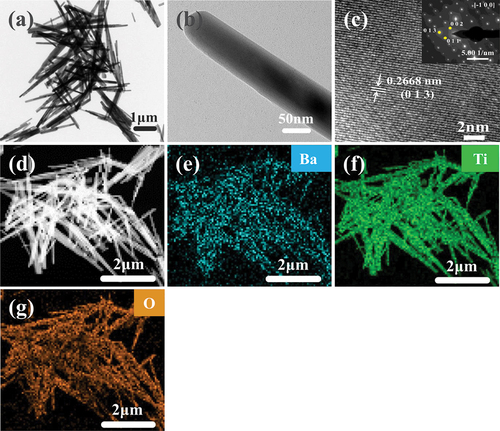
Ti(OR)4 + xCH3COOH → Ti(OR)4-x(CH3COO)x + xROH (1)
≡Ti-OR + H+ + HOH → ≡Ti-OH+R + HOH → ≡Ti-OH + ROH + H+ (2)
When the solution pH is less, Eq. (1) is the main effect, and the Ti(OR)4-x(CH3COO)x that is difficult to hydrolyse tends to form a stable Ti source solution [Citation30]. As the solvent evaporates or pH increases, the solution becomes a stable sol and then a gel.
When Ba2+ ions exist in the solution, Ba2+ does not participate in gelation and instead adsorbs onto the surface of Ti hydroxide. Thus, BaTi acetate gels can be considered as hydrated titanium acetate polymers with Ba2+ adsorbed onto the surface [Citation31]. We believe that BT2 gel was more conducive to obtaining stoichiometric products than the initial system of Ba2+ Ti4+ separation. The product was β-BT2 rather than γ-BT2, and BT impurities appeared instead of β-BT2, which changed into γ-BT2 in the product with increased NaOH concentration, which may be related to their reaction kinetics. We further speculate that in the hydrothermal system with dry gel as precursor, the order of conditions required to obtain the three substances was β-BT2 < BT < γ-BT2. As regards the specific influencing factors and the degree of influence, more research support is needed.
Piezocatalytic property
The piezocatalytic performance of β-BT2 nanorods obtained in 0.5 M NaOH solution was evaluated by RhB degradation in darkness with continuous ultrasonic irradiation only. RhB cannot be degraded by ultrasonic irradiation only in 120 min, as shown in ) by the black line. When the β-BT2 nanorods were added, the UV–vis absorption of the solution did not change significantly if it was stirred slowly only, as shown in ) by the pink line. Thus, β-BT2 did not significantly absorb RhB and did not degrade RhB obviously when the external stress was insufficient. However, the degradation rate of RhB was close to 36% in 120 min with ultrasonic working, as shown in ) by the cyan line. This finding indicated that β-BT2 was a new and effective piezoelectric catalyst for RhB degradation. The pseudo-first-order reaction rate constant (k1) of β-BT2 was 3.72 × 10–3·min–1, which was nearly 20 times that of pure RhB without β-BT2 (0.17 × 10–3·min–1) under ultrasonic irradiation, as shown in ). The corresponding UV–vis absorption spectra are shown in )–(e).
Figure 5. RhB degradation (a) and kinetic curves (b) of β-BT2 and RhB as a function of time under ultrasonic or stirring, and their time-dependent UV–vis absorption spectra of pure RhB (ultrasonic) (c), BT2+ RhB (stirring) (d), and BT2+ RhB (ultrasonic) by β-BT2 (e). Piezoelectric response amplitude curve of β-BT2 nanorods (f).
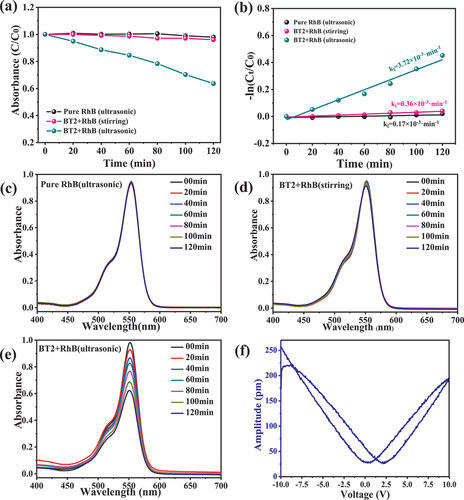
The local nanoscale piezoelectric response of β-BT nanorods was investigated by PFM, and results are shown in ). A typical ‘butterfly shape’ amplitude loop was observed with an applied voltage starting from −10 V to +10 V, suggesting different polarisation-switching behaviours and a constantly varied strain induced by the applied external electric field. PFM results proved that RhB degradation depended on the piezoelectric effect of β-BT2. The high aspect ratio of β-BT2 nanorods enabled its easy bending and deformation under ultrasonic vibration, and the piezoelectric potential induced by deformation shifted energetics. Consequently, the electron–hole pairs can separate and cross the piezoelectric/solution interface, thereby triggering redox reactions and then degrading organic pollutants.
Conclusions
β-BT2 nanorods were successfully synthesised by a simple hydrothermal method from dried gel. Well-dispersed β-BT2 nanorods with high crystallinity were obtained when the NaOH concentration, temperature, and reaction time of hydrothermal treatment were set to 0.3–0.5 M, 200°C, and 24 h, respectively. HRTEM images and the corresponding analysis showed that each nanorod was a β-BT2 single crystal. PFM results proved that the obtained nanorods showed piezoelectric response. The degradation of RhB by β-BT2 nanorods under ultrasonic irradiation demonstrated that β-BT2 was a potential piezocatalyst. The strategy utilised in this study can serve as a valuable reference for the synthesis of β-BT2 nanorods and their application in piezocatalysis.
Acknowledgments
This work is supported by the National Natural Science Foundation of China (NSFC No. U1904213), a Project Funded by the Priority Academic Program Development of Jiangsu Higher Education Institutions (PAPD), and State Key Laboratory of Mechanics and Control of Mechanical Structures (Nanjing University of Aeronautics and Astronautics) (MCMS-E-0521G02).
Disclosure statement
The authors declare that they have no known competing financial interests or personal relationships that could have appeared to influence the work reported in this paper.
Additional information
Funding
References
- Wang Z, Cheng J, Xie Y, et al. Lead-Free piezoelectric composite based on a metamaterial for electromechanical energy conversion. Adv Mater Technol. 2022;2200650:1–7.
- Jain A, Wang YG, Shi LN. Recent developments in BaTiO3 based lead-free materials for energy storage applications. J Alloys Compd. 2022;928:167066.
- Yan G, Xu L, Fang B, et al. Achieving high pulse charge–discharge energy storage properties and temperature stability of (Ba0.98-xLi0.02Lax)(Mg0.04Ti0.96)O3 lead-free ceramics via bandgap and defect engineering. Chem Eng J. 2022;450:137814.
- Nie X, Zhu K, Sun Q, et al. Enhanced discharged energy density of nanocomposites with dopamine@BaTiO3 whiskers, mater. Technol. 2020;35:515–521.
- Li H, Song Y, Zhang J, et al. Turbulence enhanced ferroelectric-nanocrystal-based photocatalysis in urchin-like TiO2/BaTiO3 microspheres for hydrogen evolution. Nanoscale Adv. 2021;3:5618–5625.
- Guo SL, Lai SN, Wu JM. Strain-Induced ferroelectric heterostructure catalysts of hydrogen production through piezophototronic and piezoelectrocatalytic system. ACS Nano. 2021;15:16106–16117.
- Wang P, Li X, Fan S, et al. Impact of oxygen vacancy occupancy on piezo-catalytic activity of BaTiO3 nanobelt. Appl Catal B Environ. 2020;279:119340.
- Singh S, Kumar A, Pandey SK, et al. Synthesis of Bi4Ti3O12-BaTiO3 nanocomposite, manifesting high dielectric and unique magnetic nature applicable in heterogeneous photocatalytic activity for degradation of rhodamine B dye, Mater. Technol. 2021;36:476–491.
- Liu W, Jin Y, Zhao Y, et al. The effect of acceptor dopant on the memory effect of BaTiO3 ceramics. J Alloys Compd. 2022;928:167182.
- Tihtih M, Ibrahim JEFM, Basyooni MA, et al. Structural, optical, and electronic properties of barium titanate: experiment characterisation and first-principles study, Mater. Technol. 2022;00:1–11.
- Akashi T, Iwata H, Goto T. Preparation of BaTi2O5 single crystal by a floating zone method, mater. Trans. 2003;44:802–804.
- Akashi T, Iwata H, Goto T. Dielectric property of single crystalline BaTi2O5 prepared by a floating zone method, mater. Trans. 2005;44:1644–1646.
- Liu X, Shang Y, Liu J, et al. 3D printing-enabled in-situ orientation of BaTi2O5 nanorods in β-PVDF for high-efficiency piezoelectric energy harvesters. ACS Appl Mater Interfaces. 2022;14:13361–13368.
- Yu J, Kohara S, Itoh K, et al. Comprehensive structural study of glassy and metastable crystalline BaTi2O5. Chem Mater. 2009;21:259–263.
- Ge X, Hu Q, Lu W, et al. Polymorphic transition and nucleation pathway of barium dititanate (BaTi2O5) during crystallization from undercooled liquid, Sci. Rep. 2019;9:1–17.
- Xu Y, Huang G, Long H. Sol-gel synthesis of BaTi2O5. Mater Lett. 2003;57:3570–3573.
- Gong Y, Liu C, Guo D, et al. Effect of Zr content on microstructure of BaTi2O5 powders prepared by sol-gel method, Mater. Lett. 2012;73:72–74.
- Liu W, Tsukada S, Akishige Y. Dielectric properties of spark plasma sintered BaTi2O5 ceramics prepared through sol-gel process. Funct Mater Lett. 2014;7:5–8.
- Wang X, Leng K, Pei Z, et al. Microstructural characterization and dielectric properties of single crystalline BaTi2O5 nanowires prepared by molten-salt method, SN Appl. Sci. 2019;1:1–10.
- Zou W, Zhou G, He W, et al. Process, fundamental and application of one-step molten-salt synthezed BaTi2O5 nanorods. J Alloys Compd. 2020;826:154064.
- Wang C, Tu R, Goto T. Effect of oxygen partial pressure on structure and dielectric property of BaTi2O5 films prepared by laser ablation, Mater. Trans. 2007;48:625–628.
- Bin Wang C, Tu R, Goto T. Epitaxial growth of B-Axis oriented BaTi2O5 films by laser ablation, Key Eng. Mater. 2007;352:311–314.
- Wang C, Tu R, Goto T. Ferroelectric BaTi2O5 thin film prepared by laser ablation. J. Vac. Sci. Technol. A Vacuum, Surfaces, Film. 2007;25:304–307.
- Bin Wang C, Tu R, Goto T. Structure and electric property of BaTi2O5 film by laser ablation, Key Eng. Mater. 2007;350:103–106.
- Guo D, Ito A, Tu R, et al. Growth of b-axis-oriented BaTi2O5 nanopillars by laser chemical vapor deposition. Key Eng Mater. 2012;508:185–188.
- Ito A, Guo D, Tu R, et al. Preparation of (0 2 0)-oriented BaTi2O5 thick films and their dielectric responses. J Eur Ceram Soc. 2012;32:2459–2467.
- Guo D, Ito A, Tu R, et al. High-speed epitaxial growth of BaTi2O5 thick films and their in-plane orientations, Appl. Surf Sci. 2012;259:178–185.
- Guo D, Ju Y, Ito A, et al. Dielectric properties of BaTi2O5 thick films prepared on Pt-coated MgO(110) single-crystal substrate by laser chemical vapor deposition. Ceram Int. 2016;42:11464–11467.
- Zhu N, West AR. Formation and stability of ferroelectric BaTi2O5. J Am Ceram Soc. 2010;93:295–300.
- Xie D, Pan W. Study on BaBi4Ti4O15 nanoscaled powders prepared by sol-gel method, Mater. Lett. 2003;57:2970–2974.
- Hennings D, Rosenstein G, Schreinemacher H. Hydrothermal preparation of barium titanate from barium-titanium acetate gel precursors. J Eur Ceram Soc. 1991;8:107–115.