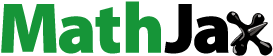
ABSTRACT
Grain boundary (GB) segregation was experimentally studied in a bulk high entropy Cantor alloy, which indicated for the first time that Cr strongly segregates to the GBs along with the weak segregation of Mn, implying co-segregation of Cr and Mn to the GBs. The strong segregation of Cr and weak segregation of Mn are explained in terms of the driving forces for GB segregation, where alloy interaction is favourable and stronger in the case of Cr, while elastic strain energy governs Mn segregation.
KEYWORDS:
Introduction
High entropy alloys (HEA) and their coatings are of significant interest because of their unique mechanical and high temperature properties [Citation1]. They consist of five or more elements with identical atomic percentages [Citation1–6]. The high entropy term [Citation4,Citation6] comes from their configurational entropy, which is maximum when all elements have identical atomic percentage. In these multi-component HEA systems, grain boundary (GB) segregation can occur, which is important for high-temperature applications. Depletion of elements from bulk can lead to nucleation of stable phases [Citation4]. Multicomponent systems are used for structural components, for instance, in aircraft engines and powerplant turbines. Previous studies on ternary and quaternary systems, CrMoV-, NiCrMoV-power plants steels, conducted by Misra’s group, indicated GB segregation of alloying and trace elements involving co-operative and site-competitive interaction processes with impact on mechanical properties [Citation7–15]. The degree of GB segregation and the nature of GB interaction processes were governed by applied stress and rate of cooling after heat treatment.
Based on the above discussion, the objective of the study is to explore GB segregation phenomena in Cantor alloy, Co20Ni20Cr20Fe20Mn20 because it is extensively studied [Citation4,Citation16–21]. To the best of our understanding, we report ‘first experimental study’ demonstrating GB segregation in bulk HEA. The study will lead to ab-initio design of complex multicomponent systems for high-temperature applications. Furthermore, segregation or depletion of elements at the grain boundary has an important effect in determining if GBs are in equilibrium with minimum GB energy.
Experimental
Cantor alloy was melted using high purity elements in a vacuum induction melting (VIM) furnace and cast in a preheated mould. Prior to segregation experiments, small samples cut from the ingot were homogenized at 1273K for 24 h. An electron backscatter diffraction (EBSD) image of homogenized alloy showing large grain size is presented in . Subsequently, they were heated at three different temperatures of 773K, 823K, 873K for 6 h and rapidly cooled to room temperature. We heat treated them for several hours to enhance GB segregation for easy elemental mapping by energy dispersive x-ray spectroscopy (EDS) in scanning electron microscopy (SEM). These temperatures were selected because at low temperatures the face centred cubic (FCC) phase in the Cantor alloy may be unstable [Citation4,Citation22,Citation23]. The polished samples were examined using SEM equipped with EDS. Elemental maps with GB point analysis were obtained to study GB segregation.
Results and discussion
It is pertinent to mention that after homogenization at 1000K for 24 h the grain size was large approaching 1 mm and no segregation of any element was observed. Thus, the data presented below is a true representative of GB phenomena. summarize the results of three experimental temperatures. Cr segregated strongly to the GBs, while segregation of Mn was weak, and nicely decorated along the GBs with a necklace-type appearance (). In , an illustration of point analysis at GB is presented. At the GB regions there was a clear depletion of Co, Fe and Ni. To delineate the change in Cr and Mn concentration as a function of temperature, we have plotted Cr/Mn ratio. Cr/Mn ratio at 773K and 823K was similar, but significantly greater at 873K because of greater diffusion at 873K. The data in indicate that significant degree of segregation (several monolayers) occurred at the GBs.
Figure 2. An example of EDS elemental mapping showing stronger GB segregation of Cr, weaker segregation of Mn and depletion of Fe, Co and Ni at the GBs.
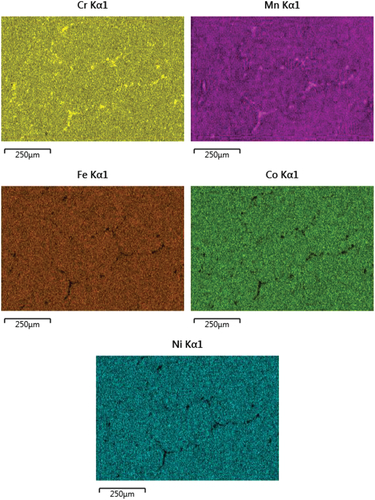
Figure 3. An example of EDS point analysis at the GB showing stronger segregation of Cr and weaker segregation of Mn at the GBs and depletion of Fe, Co and Ni at the GBs in the alloy.
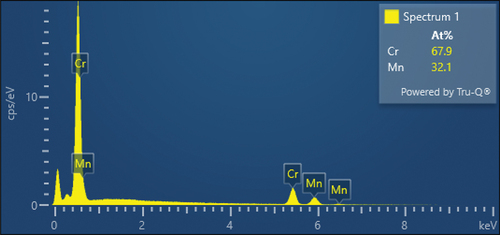
Figure 4. Cr/Mn atomic% ratio as a function of temperature demonstrating stronger GB segregation of Cr and weaker GB segregation of Mn. Bars indicate the range over which the values were observed.
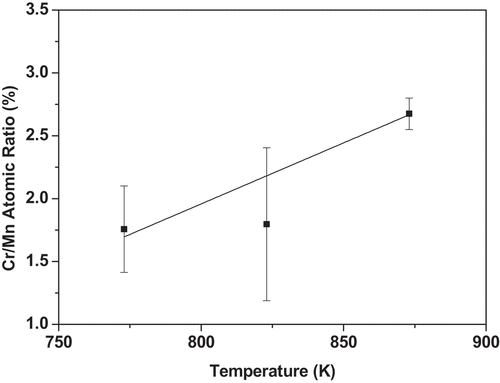
The driving force for GB or interface segregation consists of three aspects [Citation4,Citation24]: (a) interfacial energy term, (b) alloy interaction term [Citation4,Citation25] and (c) elastic solute strain energy McLean [Citation4,Citation26]. In a two-component system, the relationship between the atomic fraction of segregant at the GB, xgb, and its bulk atom fraction, xb, is given by [Citation4,Citation24,Citation27]:
where is enthalpy of segregation and is driving force for segregation to the GB, R is gas constant and T is absolute temperature. EquationEquation 1
(1)
(1) is a relationship between GB segregation and bulk composition and does not take into consideration the mass balance constraint [Citation4,Citation24,Citation27]. Based on three terms of driving force for GB segregation,
, segregation can be expressed by [Citation4,Citation24,Citation27]:
In Equationequation 2(2)
(2) , GB or interfacial energy is the first term, where
are the interfacial energies of ith component and
is molar area. The alloy interaction term is the second term consisting of regular solution constant wAB and is related to the enthalpy of mixing of a binary alloy, zl and zv are the number of bonds of an atom to neighbours that are present either within the same atom plane or in adjacent planes, respectively. The elastic strain energy is the third term comprising elastic moduli function, F(M) and ∆r is the difference in atomic radii between the GB segregating element and bulk component [Citation4,Citation24,Citation27].
Our previous studies focused on multicomponent systems consisting of three or more components and indicated that co-operative (co-segregation) and site-competitive processes occur at the GBs [Citation7–15]. Here, site-competitive process occurs between two elements when both have affinity towards the GB but have a mutually repulsive interaction. Under such circumstances, the element with stronger affinity to the GB substitutes or replaces the other. Cooperative process occurs when both the segregating species have different degrees of attraction to the GB such that both the elements are attracted. Thus, in our case, it can be implied that Cr has stronger interaction with the GB, while Mn is weakly attracted to the GB. Considering that Cr was strongly segregated to the GB in comparison to Mn (), the addition of three terms in Equationequation 2(2)
(2) (
) should be more negative for Cr in comparison to Mn, favouring stronger GB segregation of Cr and weaker segregation of Mn.
However, the values reported in the literature for surface energy (Cr = 2006 mJ/m2, Mn = 1298 mJ/m2) [Citation4,Citation28] suggest that Mn has lower surface energy. Thus, elastic strain energy in Equationequation 2(2)
(2) depends on the square of the difference in atomic radii between the segregating element and the bulk alloy (rCr-rCantor = 0.0019 nm; rMn-rCantor = 0.0032 nm, when rCr = 0.1290 nm; rMn = 0.1303 nm; rCantor = 0.1271 nm) [Citation4]. A higher value of rMn-rCantor suggests that elastic strain energy favours GB segregation of Mn, followed by Cr [Citation4]. Thus, the two driving forces in Equationequation 2
(2)
(2) are expected to favour segregation of Mn rather than Cr. This is contrary to the experimental observations. We therefore conclude that greater driving force for GB segregation of Cr must come from the alloy interaction term in Equationequation 2
(2)
(2) . This is difficult to evaluate because it is governed by the regular solution constant (and bond energy) in Equationequation 2
(2)
(2) , which is a cumulative contribution of five different elements in the Cantor alloy. Recently, the affinity between Cr-Cr and Mn-Mn atoms was studied in terms of Cr-Cr and Mn-Mn bonds and it was concluded that Cr-atoms are more likely to exhibit GB segregation compared to Mn-atoms, implying that Cr-atoms are expected to segregate where Cr-atoms previously segregated [Citation4]. Based on the above discussion, we conclude that the segregation of Mn is favoured by the GB energy (term 1 in Equationequation 1
(1)
(1) ) and the elastic strain energy (term 3) terms of driving force for segregation, but the observation of stronger GB segregation of Cr is related to greater and dominant interaction energy term 2 in Equationequation 2
(2)
(2) of the driving force for segregation. Our observations of segregation of Cr and Mn agree with similar observation on thin film of Cantor alloy deposited on Si tips, followed by the study with atom probe tomography [Citation16].
It is important to mention the consequence of GB segregation on the stability of high HEA and nucleation of phase at the GB. GB segregation of Cr and consequent depletion of Cr in the bulk alloy are envisaged to render the FCC alloy stable and the Cr-rich GB region may act as a potential site for nucleation of Cr-containing phase on heat treatment for extended period [Citation4,Citation22,Citation23].
Conclusions
Stronger GB segregation of Cr and weak segregation of Mn were experimentally observed in the bulk Cantor alloy, i.e. co-operative segregation of Cr and Mn. The differences in the behaviour of Cr and Mn segregation were explained in terms of three constituents of driving force for GB, where strong alloy interaction dominated and favoured GB segregation of Cr, while Mn segregation was favoured by GB energy and elastic strain energy terms of driving force that were less dominant. GB segregation is envisaged to positively impact the stability of the FCC alloy of Cantor alloy, and the GB Cr-rich region may act as a nucleation site for precipitation of Cr-containing phase.
Acknowledgments
The authors thank Dr. C. Bradley and Veronica Contreras for their help with sample preparation and Charles Meyer for microscopy. The authors were encouraged by Drs. Paul Wynblatt, Dominique Chatain study on modeling of surface segregation in HEA.
Disclosure statement
No potential conflict of interest was reported by the authors.
References
- Gong N, Meng TL, Cao J, et al. Laser-cladding of high entropy alloy coatings: an overview. Mater Technol. 2022;38(1):2151696. DOI:10.1080/10667857.2022.2151696
- Tsai SP, Tsai Y-T, Chen Y-W, et al. High-entropy CoCrFeMnNi alloy subjected to high-strain-rate compressive deformation. Mater Charact. 2019;147:193. doi:10.1016/j.matchar.2018.10.028
- Zhang M, George EP, Gibeling JC, et al. Tensile creep properties of a CrMnFeCoNi high-entropy alloy. Scripta Mater. 2021;194:11363. doi:10.1016/j.scriptamat.2020.113633
- Wynblatt P, Chatain D. Modeling grain boundary and surface segregation in multicomponent high-entropy alloys. Phys Rev Mater. 2019;3(5):05004. doi:10.1103/PhysRevMaterials.3.054004.
- Cantor B, Chang ITH, Knight P, et al. Microstructural development in equiatomic multicomponent alloys. Mater Sci Eng A. 2004;375:213. doi:10.1016/j.msea.2003.10.257
- Ranganathan S. Alloyed pleasures: multimetallic cocktails. Curr Sci. 2003;85:1404.
- Misra RDK, Balasubramanian TV, Rao PR, et al. On interactions amongst trace and alloying elements at the grain boundaries of a low alloy steel. Acta Metall. 1987;35(12):2995. DOI:10.1016/0001-6160(87)90298-7
- Misra RDK, Balasubramanian TV. Co-operative and site-competitive interaction processes at the grain boundaries of a Ni-Cr-Mo-V steel. Acta Metall. 1989;37(5):1475. doi:10.1016/0001-6160(89)90179-X.
- Misra RDK, Balasubramanian TV. Stress enhanced grain boundary segregation of impurity elements in a low alloy steel. Acta Metall. 1990;38(7):1263. doi:10.1016/0956-7151(90)90197-O.
- Misra RDK, Balasubramanian TV. Effects of microstructure on grain boundary segregation processes in low alloy steels. Acta Metall. 1990;38(11):2357. doi:10.1016/0956-7151(90)90103-N.
- Misra RDK, Rama Rao P. On the grain boundary chemistry of chromium containing martensitic steels. Acta Metall. 1991;39(9):2183. doi:10.1016/0956-7151(91)90188-7.
- Misra RDK, Rama Rao P. Grain boundary segregation in a low alloy steel under tensile loading conditions. Acta Metall. 1992;40(6):1223. doi:10.1016/0956-7151(92)90420-J.
- Misra RDK, Rama Rao P. Influence of tensile stress on behaviour of grain boundary segregants and related interactions between trace and solute elements in 2· 6Ni–Cr–Mo–V low alloy steel. Mater Sci Technol. 1993;9:497.
- Misra RDK. An observation concerning the contribution of temperature on the behaviour of grain boundary segregants for varied microstructures in a NiCrMoV steel. Scripta Mater. 1991;25(5):1109. DOI:10.1016/0956-716X(91)90510-8
- Misra RDK, Rao PR. Grain boundary segregation isotherms. Mater Sci Technol. 1997;13(4):277. doi:10.1179/mst.1997.13.4.277.
- Li YJ, Savan A, Kostka A, et al. Accelerated atomic-scale exploration of phase evolution in compositionally complex materials. Mater Horiz. 2018;5(1):86. DOI:10.1039/C7MH00486A
- Haase C, Tang F, Wilms MB, et al. Combining thermodynamic modeling and 3D printing of elemental powder blends for high-throughput investigation of high-entropy alloys – Towards rapid alloy screening and design. Mater Sci Eng A. 2017;688:180. doi:10.1016/j.msea.2017.01.099
- Li Z, Tasan CC, Pradeep KG, et al. A TRIP-assisted dual-phase high-entropy alloy: grain size and phase fraction effects on deformation behavior. Acta Mater. 2017;131:323. doi:10.1016/j.actamat.2017.03.069
- Thurston K, Gludovatz B, Hohenwarter A, et al. Effect of temperature on the fatigue-crack growth behavior of the high-entropy alloy CrMnFeCoNi. Intermetallics. 2017;88:65. doi:10.1016/j.intermet.2017.05.009
- Koch CC. Nanocrystalline high-entropy alloys. J Mater Res. 2017;32(18):3435. doi:10.1557/jmr.2017.341.
- Bracq G, Laurent-Brocq M, Perrière L, et al. The FCC solid solution stability in the Co-Cr-Fe-Mn-Ni multi-component system. Acta Mater. 2017;128:327. doi:10.1016/j.actamat.2017.02.017
- Pickering EJ, Muñoz-Moreno R, Stone HJ, et al. Precipitation in the equiatomic high-entropy alloy CrMnFeCoNi. Scripta Mater. 2016;113:106. doi:10.1016/j.scriptamat.2015.10.025
- Oto F, Iadicola MA, Gnäupel-Herold T, et al. Multiaxial constitutive behavior of an interstitial-free steel: measurements through X-ray and digital image correlation. Acta Mater. 2016;112:84–5. doi:10.1016/j.actamat.2016.04.013
- Wynblatt P, Ku RC. Surface energy and solute strain energy effects in surface segregation. Surf Sci. 1977;65(2):511. doi:10.1016/0039-6028(77)90462-9.
- Defay R, Ilya P. Surface tension and adsorption. New York: Wiley; 1966. p. 158.
- Mclean D. Grain boundaries in metals. London: Oxford University Press; 1957.
- Wynblatt P, Chatain D. Anisotropy of segregation at grain boundaries and surfaces. Metall Mater Trans A. 2006;37(9):2595. doi:10.1007/BF02586096.
- Tyson W, Wynblatt P. Observations of a two-dimensional compositional phase transition at the surface of a polycrystalline Pb?Bi?Ni alloy. Surf Sci. 1994;302(1–2):179. doi:10.1016/0039-6028(94)91107-X.