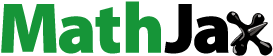
ABSTRACT
Ionic liquid 1,3-dimethylimidazolium dimethyl phosphate (DIDP) is used as a possible green inhibitor for the corrosion control of 6061 aluminium alloy in 0.25 mol/L HCl is described in the study. Study involved electrochemical methods carried out at various temperatures by changing the concentrations of DIDP. Kinetic and thermodynamic parameters were determined using the Arrhenius rate law and transition state equations, respectively. Physisorption of the inhibitor takes place and the adsorption follows Freundlich isotherm. Surface morphology was studied by scanning electron microscopy (SEM), atomic force microscopy (AFM), and energy-dispersive X-ray analysis (EDAX) techniques. Quantum chemical studies were done by the density functional theory (DFT). The maximum inhibition efficiency of DIDP on 6061 aluminium alloy was about 78% for the concentration of 1000 ppm at 303 K. The mechanistic aspects of DIDP adsorption onto the metal surface were supported by quantum chemical studies. HOMO and LUMO of the optimized structure and quantum chemical descriptors confirmed the adsorption of the inhibitor on the metal surface. Mulliken charge population was used to identify the DIDP molecule’s high electron density region, and Fukui indices confirmed the interaction between metal and inhibitor.
GRAPHICAL ABSTRACT
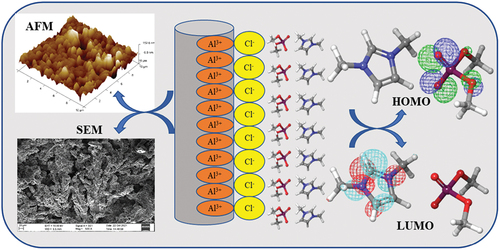
Introduction
Corrosion is an unintentional natural process having interaction between material and its environment, the interaction being either chemical or electrochemical reaction resulting in material loss and useful properties. Corrosion studies are important for various reasons such as economic, health hazards, material loss, loss of useful properties and failure of corroded parts in machine components and structures. The study of corrosion is equally important for the conservation of materials, particularly metals and also from aesthetic and academic points of view. Corrosion can lead to loss of products, plant shutdown, repair and replacement costs, loss of life, etc., A moderate estimate regarding the cost of corrosion is about 4% of a country’s GDP, and it could be more in the case of industrially advanced countries, matching almost with the amount spent on education. Corrosion at best can be combated but not prevented [Citation1,Citation2].
Aluminium alloys are commonly used in engineering structures and components that require lightweight and corrosion resistance. Aluminium has unique properties like low density, durability, high strength, good conductivity, corrosion resistivity, etc., making it a choice for many applications. In aerospace industry and transportation aluminium and its alloys are extensively used [Citation3]. Aluminium, on exposure to air, forms an oxidized layer on the metal surface which can control its further corrosion. Corrosion of aluminium and its alloys in many of the industrial environments is less compared to ferrous materials. Aluminium alloys exhibit good service life in air or in neutral aqueous chloride-free solutions at room temperature. However, it can corrode when exposed to an acid medium, or caustic environment. In industries, aluminium alloys undergo corrosion during the pickling or descaling process, and the thickness of the aluminium alloy will decrease. To prevent this, inhibitors are added to the corrosive medium. Nevertheless, aluminium alloys can offer a long service life by carefully choosing the proper alloy, good design, and use of corrosion inhibitors [Citation4,Citation5].
Corrosion inhibitors are either inorganic or organic heterocyclic compounds that decrease the corrosion rate without undergoing any chemical modification when added to a corrosive environment. The presence of heteroatom, unsaturation, low cost, non-toxic nature, readily available, miscible with the medium are the desirable features of a good inhibitor. The lone pair of electrons present in some of the hetero atoms are the reason for the inhibitor’s adsorption on the metal surface. Certain chemical inhibitors show excellent inhibition efficiency towards the corrosion of materials. A few examples of chemical inhibitors used in acid corrosion inhibition of aluminium are 6-diallylamino-1,3,5-triazine-2,4-dithiol monosodium (DAN) and 6-dibutylamino-1,3,5-triazine-2,4-dithiol monosodium (DBN) [Citation6], 2-bromo-2-nitro-1,3-propanediol (bronopol) [Citation7], and Sodium [(1,5-dimethyl-3-oxo-2-phenylpyrazol-4-yl)-methylamino] methanesulfonate (analgin) [Citation8]. Bronopol is a biocide, and analgin is a pain-killer and antipyretic.
However, some of the chemical inhibitors are toxic and costly. Some of the inhibitors may affect the health of humans and the environment. Corrosion inhibitors have always raised safety and environmental concerns in the industrial world because of the toxic effects. Eco-friendly and harmless green inhibitors are now widely recommended. Green inhibitors are non-toxic, biodegradable, do not contain heavy metals, and do not cause any harmful effects on the environment. Plant extracts, biopolymers, surfactants, and ionic liquids are some of the major green inhibitors reported in the literature.
Nowadays, ionic liquids are widely used. They contain an organic cation and an inorganic anion. Few ionic liquids have unique properties like biodegradability, non-inflammability, low toxicity, low volatility, and low vapour pressure, because of which it is considered a ‘green corrosion inhibitor’. There are protic and aprotic ionic liquids. Both protic and aprotic ionic liquids can be used for corrosion inhibition studies. Protic ionic liquids are more reactive towards metals than aprotic ionic liquids and hence suitable for corrosion inhibition studies [Citation9]. By proper selection of anion and cation, efficient tailor made ionic liquids can be designed as corrosion inhibitors [Citation10–12]. The present study uses ionic liquids as green corrosion inhibitors.
A few examples for ionic liquids which are successfully used as corrosion inhibitors for various metals are 1-allyl-3-ethylimidazolium bromide ([AEIM]Br), 1-allyl-3-butylimidazolium bromide ([ABIM]Br), 1-allyl-3-hexylimidazolium bromide ([AHIM]Br), and 1-allyl-3-octylimidazolium bromide ([AOIM]Br) are used for copper [Citation13], 1-ethyl-3- methylimidazolium bis(trifluoromethylsulfonyl)amide [EMIm] [NTf2],, 1-hexyl-3-methylimidazolium bis(trifluoromethylsulfonyl) amide [HMIm][NTf2], and 1-decyl-3-methylimidazolium bis(tri fluoromethylsulfonyl)amide [DMIm][NTf2] used for magnesium [Citation14], 1-butyl-3-methylimidazolium tetrafluoroborate [BMIM][BF4−] and 1-decyl-3-methylimidazolium tetrafluoroborate [DMIM][BF4−] used for zinc [Citation15]. Some of the ionic liquids used to control the corrosion of aluminium and its alloys are 1-octyl-3-methylimidazolium bromide ([OMIM]Br) and 1-allyl-3-octylimidazolium bromide ([AOIM]Br) [Citation16]. 1-butyl-3- methylimidazolium chlorides (BMIC), 1-hexyl-3-methylimidazolium chlorides (HMIC), and 1-octyl-3-methylimidazolium chlorides (OMIC) [Citation17], (poly(1-vinyl-3- dodecylimidazolium hexafluorophosphate) PImC12, poly(1-vinyl-3-octylimidazolium hexafluorophosphate) PImC8, and poly(1-vinyl-3-butylimidazolium hexafluorophosphate) PImC4 [Citation18]. tetradecylpyridinium bromide (TDPB) [Citation19], 1,3-bis(2-oxo-2-phenylethyl)-1H-imidazol-3-iumbromide (OPEIB) [Citation20]. From the above reports of ionic liquids used for aluminium and its alloys, it is evident that the inhibition efficiency is more in the imidazolium derivative than the pyridinium derivative, because of the presence of two nitrogen atoms. The nitrogen atoms are responsible for easy adsorption of inhibitors onto the metal surface. It is also observed that the increase in the length of the carbon side chain in the imidazolium derivative increases the inhibition efficiency. In the present work, only the methyl group is attached to both the nitrogen; still, the inhibitor showed very good inhibition efficiency.
In this paper, we present the results of ‘1,3-dimethylimidazolium dimethyl phosphate’ (DIDP) as an effective inhibitor. The corrosion inhibition study using DIDP has not been done so for aluminium alloy in 0.25 mol/L HCl. The molecular structure of DIDP is depicted in . The large number of heteroatoms like nitrogen, oxygen, and phosphorous makes 1,3-dimethylimidazolium dimethyl phosphate a good candidate as a corrosion inhibitor.
DFT studies help to identify the electron-rich and deficit site in DIDP. It also identifies which part of the molecule is adsorbed on the metal surface. Mulliken charge population helps to know the charge on each atom. Fukui indices analysis helps to identify the reactivity of atoms present in the molecule.
Materials and methods
Sample
The test coupon of 6061 aluminium alloy was cast with dental resin exposing a 1 cm2 surface area. The exposed surface area was polished with different grit emery papers and later disc polished with levigated Al2O3 suspension. shows the wt. (%) of the 6061 aluminium alloy.
Table 1. Composition of test specimen.
Medium
A stock solution of 1 mol/L HCl solution was prepared from procured HCl (Sigma-Aldrich, 35%) using double-distilled water. The stock solution was standardized using standard sodium carbonate solution with indicator methyl orange. 0.25 mol/L HCl was prepared as and when required from stock solution by diluting it with double distilled water.
Inhibitor
Commercially available (Sigma Aldrich make) DIDP was procured. Various known concentrations of DIDP in 0.25 mol/L HCl were prepared.
Electrochemical studies
The electrochemical workstation (CH600 D-series with beta software) was made use for conducting experiments of potentiodynamic polarization (PDP) and electrochemical impedance spectroscopy (EIS) studies. Pyrex glass beaker, along with three different electrodes such as platinum electrode (auxiliary electrode), saturated calomel (reference electrode), and the working electrode (6061 aluminium alloy), was used as an electrochemical cell.
The polished 6061 aluminium alloy was dipped in 0.25 mol/L HCl medium without and with the various DIDP concentrations at 303 K to 323 K temperature range. The electrochemical cell was placed in a thermostat set to a particular temperature of study. Without stirring, the measurements were carried out.
Before performing electrochemical measurements, open circuit potential (OCP) was noted after 1800 s.
PDP
To perform PDP measurements, 6061 aluminium alloy was polarised from OCP by applying − 250 mV cathodically to +250 mV anodically at a 1 mVs−1 scan rate. Corrosion current density and electrochemical parameters were obtained from the PDP plot. Corrosion current density was used to calculate corrosion rate by the Equationequation (1)(1)
(1)
K = proportionality constant which is equal to 0.00327 mm g μ A−1 cm−1 y−1, M (molar mass = 27), icorr = corrosion current density in μA cm−2, z (metal valence electrons = 3), and ρ (density of metal = 2.7 g cm−3)
EIS
At the OCP, an AC signal of amplitude 10 mV and 100 kHz to 0.01 Hz frequency range was applied. The resultant Nyquist plot were fit into the appropriate circuit using ZSimpwin software version 3.21. The double-layer capacitance (Cdl) and polarization resistance (RP) were determined.
EIS was conducted first followed by PDP without disturbing the experimental setup. For the addition of each inhibitor concentration at each temperature, both PDP and EIS techniques were done 3–4 times. The inhibition efficiency reported is averaged out separately for each technique.
Surface analysis
The surface morphology was examined by SEM (JEOL JSM-6380 L) at 500 X magnification. Three small coupons of 1 cm2 area each were polished using different grades of emery paper followed by a disc polisher. After polishing, the coupons were washed using distilled water and cleaned. The first coupon was used as such after polishing (blank). The second metal coupon was immersed in 0.25 mol/L HCl for 12 h. The third coupon was immersed in 0.25 mol/L HCl and 1000 ppm of DIDP inhibitor and kept for 12 h. All three coupons were used for SEM analysis. Elemental mapping was carried out by EDAX (Energy-dispersive X-ray) analysis at 200 µm magnification. The roughness in the surface was obtained by AFM (1B342 Innova model) technique by contact mode.
Computational studies
Schrodinger suite was used for theoretical calculations. The basis set for the analyses was (B3LYP) and 6-31 G. The highest occupied molecular orbital (EHOMO), lowest unoccupied molecular orbital (ELUMO), energy gap (ΔE), hardness (), softness (σ), electrophilicity (ω), nucleophilicity (ϵ), and so on was calculated by DFT technique. The electron density of different atoms of the DIDP molecule was known by the Mulliken charge population. Electron donating and accepting properties of DIDP molecule were evaluated by Fukui indices analysis.
Results and discussion
Potentiodynamic polarisation (PDP) technique
depicts the potentiodynamic polarization plot for 6061 aluminium alloy in 0.25 mol/L HCl for various DIDP concentrations at 313 K. At different temperatures, similar plots were obtained.
The anodic curve of the potentiodynamic polarisation plot depicts metal dissolution and cathodic curve hydrogen evolution in an acidic environment. As the anodic curve has an inflection point, corrosion current density was difficult to obtain. Hence, extending the linear part of the cathodic curve provided corrosion current density. The inhibition efficiency was derived using the corrosion current density from Equationequation (2)(2)
(2) .
icorr = corrosion current density (blank) and
icorr(inhi) = corrosion current density (inhibitor).
PDP data are given in . Tafel slopes reported in indicate no significant variation. This suggested that the added inhibitor did not affect the corrosion mechanism [Citation21,Citation22]. The inhibitor simply blocks the available sites thereby minimizing corrosion.
Table 2. PDP results of 6061 aluminium alloy corrosion in 0.25 mol/L HCl for different DIDP concentrations.
According to the PDP results, icorr and CR increased with increasing temperature. The corrosion rate decreased after the addition of DIDP, resulting in increased inhibition efficiency. The inhibition efficiency decreased as the temperature increased, implying that the DIDP molecule may have physically adhered to the metal’s surface.
According to the literature [Citation23,Citation24], when the change in corrosion potential (Ecorr) is greater than ±85 mV when inhibitor was added, then the inhibitor is considered anodic or cathodic. In the present study, the corrosion potential difference when the DIDP inhibitor was added was found to be below −85 mV, indicating that DIDP acted as a mixed inhibitor.
The maximum efficiency of DIDP is 78.38% for 1000 ppm of DIDP immersed in 0.25 mol/L HCl at 303 K.
Electrochemical impedance spectroscopic (EIS) method
Nyquist plot for 6061 aluminium alloy for various concentrations of DIDP in 0.25 mol/L HCl at 313 K is shown in .
The shape of the plot agrees completely with those previously reported for aluminium corrosion with HCl and other mediums [Citation25]. The Nyquist plot has two loops. The high-frequency capacitive loop is the alloy’s resistance towards corrosion and is also called charge transfer resistance. Initially, when no inhibitor was added, charge transfer resistance was low. That means corrosion takes place faster. After the addition of an inhibitor, resistance to charge transfer increases. An increase in the semicircle diameter indicates the same. Further, with a concentration increase in the inhibitor, resistance to the charge transfer increased. In other words, resistance to corrosion increases. Hence, there is an increase in the diameter of the Nyquist semicircle. A depressed Nyquist semicircle represents surface inhomogeneity [Citation26].
The inductive loop represents the adsorption and penetration of Cl− ions into the oxide film layer [Citation27,Citation28]. The aluminium oxide layer is regarded as a parallel circuit because of the conduction of oxide film and a capacitor due to its dielectric properties.
Polarization resistance increases when the concentration of the inhibitor increases. An increase in polarization resistance could be due to the inhibitor forming a protective film on the surface of alloy. An increase in double-layer thickness decreases the double-layer capacitance. % inhibition efficiency increases with an increase in the concentration of inhibitor [Citation29,Citation30]
ZSimpwin software was used for equivalent circuit fitment. It fits best into the five-element circuit. Of these, the resistances are solution resistance (RS), charge transfer resistance (Rct), and inductive resistance (RL). There is a constant phase element (Q) and an inductive element (L). shows the circuit fitment graph.
Charge transfer and inductive resistances were parallel. Therefore, polarization resistance was calculated by taking the sum of the reciprocal of RCT and RL, which is given in Equationequation (3)(3)
(3) .
Using Equationequation (4)(4)
(4) , Cdl was calculated.
Qdl denotes the CPE constant, ωmax denotes the frequency at which the imaginary impedance (Z//) is greatest, and n denotes the CPE exponent, which explains the electrode surface unevenness. When n = 1, CPE acts as an ideal capacitor.
The inhibition efficiency of the DIDP was calculated by the formula given in Equationequation (5)(5)
(5) [Citation31]:
Polarization resistance in the presence and absence of a DIDP is represented by RP (inh) and RP (blank). EIS results are given in for 6061 aluminium alloy.
Table 3. EIS results of 6061 aluminium alloy corrosion in 0.25 mol/L HCl containing different DIDP concentrations.
shows the Bode plot of 6061 aluminium alloy at various inhibitor concentrations.
The phase angle increases as the DIDP concentration increases. This represents the DIDP molecule that covers the 6061 aluminium alloy surface [Citation32]. The impedance modulus is greatest at 1000 ppm DIDP concentration, implying the maximum efficiency of DIDP at 1000 ppm [Citation33]. EIS studies show the highest efficiency of 72.46% for 1000 ppm of DIDP concentration in 0.25 mol/L HCl at 303 K.
Effect of temperature
Arrhenius rate law equation gives the activation energy from Equationequation (6)(6)
(6) .
The transition state equation entropy and enthalpy of activation from Equationequation (7)(7)
(7) .
CR = corrosion rate,
B = Arrhenius constant,
Ea = activation energy (obtained from the slope),
R = universal gas constant (8.314 JK−1 mol−1), and
T = temperature (K) [Citation34,Citation35].
N is Avogadro’s number (6.022×1023 mol−1), and
h is Planck’s constant (6.626×10−34 Js).
shows the lnCR vs. 1/T plot and lnCR/T vs. 1/T plot for 6061 aluminium alloy at varying DIDP concentrations in 0.25 mol/L HCl medium.
Figure 6. (a) Arrhenius plot and (b) Transition state plot of 6061 aluminum alloy corrosion in 0.25 mol/L HCl with different concentrations of DIDP.
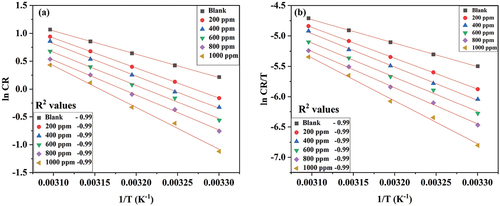
shows activation/kinetic parameters obtained from the Arrhenius rate law and transition state equations.
Table 4. Activation parameters.
With the increase in DIDP concentration, activation energy increased, resulting in decreased corrosion rate. This is due to the DIDP molecule, which is adsorbed, forming a barrier between the medium and metal. The enthalpy of activation (∆Ha) is similar to that of Ea, which explains the exothermic process of adsorption [Citation36]. The entropy of activation (∆Sa) has a negative value moving towards positive because of increased disorderliness while forming the activated complex and implies physical adsorption [Citation34].
Adsorption study
Adsorption isotherms like Temkin, Freundlich, Frumkin, and Langmuir have been tried to fit into the results obtained. None have fitted into the following adsorption isotherms except Freundlich. The best fitted is the one with an R-square value equal to unity [Citation37,Citation38]. Corrosion of 6061 aluminium alloy gave a linear plot in the presence of DIDP, which fits into the Freundlich adsorption isotherm and has a regression coefficient (R2) nearly equal to unity. The formula of Freundlich adsorption isotherm is in Equationequation (8)(8)
(8) .
K = adsorption/desorption constant [Citation39],
‘n’ = Freundlich constant, and
C = concentration of DIDP.
Different adsorption isotherm plots are shown in .
Figure 7. Plots for (a) Langmuir adsorption isotherm, (b) Frumkin adsorption isotherm, (c) Temkin adsorption isotherm, and (d) Freundlich adsorption isotherm.
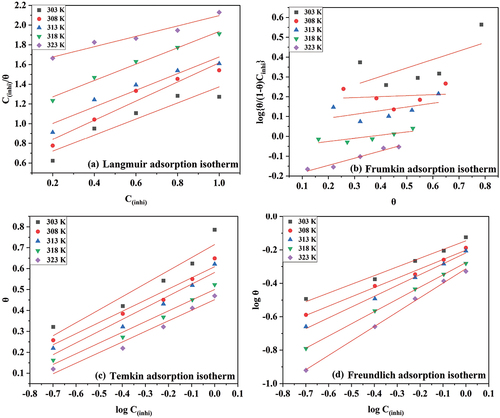
depicts a Freundlich adsorption isotherm plot. lists the parameters of the Freundlich adsorption isotherm.
Table 5. Freundlich adsorption isotherm parameters.
As the temperature increased, the value of K decreased, which implies physical adsorption. In , the slope value was below one, and the value of n was above 1. If the value of the slope is in the range of 0 and 1 and n in the range of 0 and 10, it favours the adsorption. The regression coefficient (R2) was nearly equal to one. As a result, the results best fit into the Freundlich adsorption isotherm.
The intercept of the Freundlich plot was used to calculate Kads. Standard free energy can be calculated by incorporating the value of K into Equationequation (9)(9)
(9) .
T = temperature in Kelvin,
R = universal gas constant [Citation40], and
55= molar concentration of water in the solution in mol dm−3.
Plotting the graph ΔG0ads Vs. Temperature, standard enthalpy of adsorption (ΔH0ads), and standard entropy of adsorption (ΔS0ads) can be determined. EquationEquation (10)(10)
(10) gives Gibb’s-Helmholtz equation.
depicts ΔG0ads Vs. T graph, for DIDP adsorbing on 6061 aluminium alloy in 0.25 mol/L HCl.
The thermodynamic parameters are given in .
Table 6. Thermodynamic parameters of 6061 aluminium alloy in 0.25 mol/L HCl on DIDP adsorption.
The standard free energy of adsorption (ΔG0ads) for DIDP adsorption onto the metal surface ranges from −8.81 to −9.25 kJ mol−1, referring to the physical adsorption of DIDP [Citation41]. For physical adsorption, the value of ΔG0ads must be below −20 kJ mol−1; chemisorption must be between −40 and −100 kJ mol−1 [Citation42]. The negative standard enthalpy of adsorption (△H0ads) refers to physical adsorption, whereas the negative standard entropy of adsorption (△S0ads) is due to a decrease in the system’s randomness [Citation43].
Mechanism of corrosion inhibition
Aluminium corrosion in the HCl medium is spontaneous. EquationEquations (11)(11)
(11) and (Equation12
(12)
(12) ) show anodic reactions.
Anodic reaction:
Aluminium loses its electrons during oxidation and converts to Al3+. From the HCl medium, chloride ions adhere to the surface of the aluminium to satisfy the positive charge on the alloy surface [Citation44]. The Al3+ attracts more chloride ions to form [Al(Cl)6]3-. At the cathode, hydrogen evolution occurs, as shown in the Equationequations (13)(13)
(13) and (Equation14
(14)
(14) ).
Cathodic reactions:
The H+ ions in the medium take up electrons released at the anode and liberate the H+ ions as hydrogen gas [Citation45]. EquationEquation (15)(15)
(15) depicts the adsorption of the positively charged organic part of the DIDP molecule forming complex on the surface of 6061 aluminium.
depicts the mechanism of DIDP molecule physical adsorption on 6061 aluminium surface.
Surface analysis
SEM analysis
shows SEM pictures of finely polished 6061 aluminium alloy and in 0.25 mol/L HCl in the presence and absence of DIDP for 12 h at normal room temperature.
Figure 10. SEM pictures of (a) Finely polished 6061 aluminum alloy, (b) 6061 aluminum alloy + 0.25 mol/L HCl (c) 6061 aluminum alloy + 0.25 mol/L HCl + 1000 ppm of DIDP.
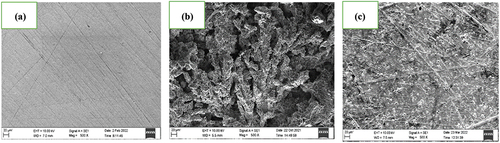
The metal surface looks smooth in freshly polished 6061 aluminium alloy. When the metal was immersed in 0.25 mol/L HCl, it appeared rough comparatively as more corrosion occurred [Citation46]. In the presence of a DIDP, the surface appeared smooth because the DIDP forms a protective layer and prevents corrosion.
EDX analysis
EDX data of 6061 aluminium alloy in 0.25 mol/L HCl in the presence and absence of DIDP is shown in .
Table 7. EDX data of 6061 aluminium alloy in 0.25 mol/L HCl.
The wt % composition of aluminium was more in the freshly polished 6061 aluminium alloy. When dipped in 0.25 mol/L hydrochloric acid, wt % aluminium composition decreased as corrosion occurred. Wt % composition of aluminium increased when DIDP is present, as DIDP formed a protective barrier and prevented aluminium from corroding. Chlorine present is because of the medium and metal interaction. Nitrogen is because of the interaction between metal and DIDP [Citation47,Citation48].
AFM studies
shows AFM images of finely polished 6061 aluminium alloy and when the alloy dipped in 0.25 mol/L HCl with and without DIDP.
Figure 11. AFM pictures of a) Freshly polished 6061 aluminum alloy, b) 6061 aluminum alloy + 0.25 mol/L HCl, c) 6061 aluminum alloy + 0.25 mol/L HCl + DIDP.
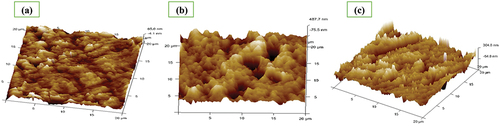
AFM pictures of 6061 aluminium alloy dipped in 0.25 mol/L HCl has comparatively more roughness than finely polished 6061 aluminium alloy and the alloy along with DIDP. The roughness values are given in .
Table 8. AFM data.
displays the values for average roughness (Ra), Root means square roughness (Rq), and maximum roughness (Rmax). As more corrosion occurs in 0.25 mol/L HCl medium, the roughness values for aluminium dipped in 0.25 mol/L HCl were higher. The roughness values for 6061 aluminium alloy immersed in 0.25 mol/L HCl medium and 1000 ppm of DIDP have decreased.
The mean height calculated over the measured length/area is the average roughness (Ra). The term (Ra) is commonly used to describe the roughness of machined surfaces. Roughness (Rq) is defined as the square root of the surface height distribution. Maximum peak-to-valley height (Rmax) describes the overall roughness of the surface.
This is because DIDP adsorbed on the alloy surface, by forming a barrier and stopping the attack from acid [Citation49,Citation50].
Computational studies
DFT technique
Quantum calculations were conducted using (B3LYP) and 6-31 G as the basis set. The structure of 1,3-dimethylimidazolium dimethyl phosphate (DIDP) is given in .
The aliphatic chain of the DIDP structure is planar. The presence of a high molecular weight anionic part of DIDP expands the size of the ionic liquid. The increased molecular size of the counter ion and the planarity property increases the DIDP’s surface coverage on the metal [Citation51]. The nitrogen in the heterocyclic ring structure enhanced the adsorption of DIDP on the metal surface. Therefore, it reduces the rate of corrosion [Citation52,Citation53]. The optimized structures of HOMO and LUMO are depicted in . The HOMO frontier molecular orbital helps in the donation of electrons towards the metal surface. The LUMO frontier molecular orbital helps in accepting the electrons from the metal surface [Citation54].
The electron distribution in HOMO and LUMO helps us identify the DIDP and metal interaction. The HOMO and LUMO structures refer to DIDP’s ability to donate and accept electrons [Citation4]. HOMO of DIDP is present in the counter ion, and LUMO is in the organic cationic part. Therefore, electron donation occurs through the anionic part and electron acceptance through the cationic part. DIDP’s adsorption occurs by sharing electrons from both anionic and cationic parts to the metal surface [Citation55]. shows the electronic parameters.
Table 9. Electronic parameters of DIDP molecule.
All units are in eV, except σ in eV−1 and dipole moment in Debye
The EHOMO and ELUMO values of the DIDP molecule are −6.950 eV and −6.324 eV. The energy gap (ΔE) predicts the reactivity of the DIDP and can be obtained by the formula given in Equationequation (16)(16)
(16) .
ELUMO is the lowest unoccupied molecular orbital, and EHOMO is the highest occupied molecular orbital. The energy gap of the DIDP molecule is 6.312 eV. Electronegativity (χ) refers to the ability to hold electrons and can be calculated using the Equationequation (17)(17)
(17)
DIDP has a χ value of 3.791 eV. Hardness () was calculated by the formula given in Equationequation (18)
(18)
(18)
I (Ionization potential) is negative of EHOMO value, and A (Electron affinity) is negative of ELUMO value. The hardness of DIDP was found to be 3.158 eV. Softness (σ) was calculated by taking the reciprocal of the hardness value and was found to be 0.316 eV−1. The electrophilicity index (ω) shows the DIDP’s ability to accept electrons. It also implies the DIDP molecule’s participation in a back donation. Electrophilicity was calculated by the formula given in Equationequation (19)(19)
(19) and was found to be 1.137 eV.
Where χ is the electronegativity and is the hardness value. The reciprocal of electrophilicity gives nucleophilicity (ϵ) which is 0.879 eV.
represents the fraction of electron transferred, calculated by the formula given in Equationequation (20)
(20)
(20) .
Where is the work function of aluminium which is 4.2 eV, χ represents the electronegativity of DIDP and
represents the hardness of DIDP. △N is a fraction of an electron transferred from an inhibitor molecule to a metal. Any value greater than zero indicates that electron transfer is taking place. Effective electron transfer means it easily forms a complex with the metal ion at the interface and forms a protective film. As per the reported literature [Citation56,Citation57], if the value of △N is more than zero and below 3.6 eV, then the electron-donating property is more. According to the present study, the value of △N is positive, which is 0.045 eV, indicating easy adsorption of DIDP on the metal surface and showing inhibition action. DIDP has a greater dipole moment of 13.127 Debye than a water molecule of 1.88 Debye. As a result, DIDP molecules easily displace water molecules, and the DIDP and aluminium alloy has a strong dipole-dipole interaction.
Mulliken charge density identifies the high electron density region of DIDP. The electron density was more around hetero atoms as hetero atoms containing lone pair of electrons, and the bond formed by heteroatoms will be strong [Citation28]. The accumulation of negative charges in oxygen and nitrogen is shown in . In the present study, the positively charged nitrogen adsorbs on the alloy surface and forms bond [Citation58,Citation59]. DIDP has more number of active sites. From the mechanism, it is confirmed that nitrogen with positive charge adsorbs on alloy surface. The total electron density value for organic cation part is more positive compared to inorganic anion part. Therefore, the positively charged organic cation will adhere on to the metal surface. In the overall charge on both the nitrogen is shown as negative probably due to resonance, although one of the nitrogen in organic cation part has positive charge.
Fukui indices analysis
shows the results of the Fukui indices analysis. Fukui indices analysis helps identify the reactivity of atoms present in the molecule. The results obtained values of DIDP are located in C1, C2, N3, C4, N5, H19, H22, and H23. This suggested that these atoms accept electrons readily and act as electrophiles [Citation60]. The
values of DIDP is located in P8, O9, O10, O11, and O12, indicating these atoms donate electrons and act as nucleophiles [Citation61,Citation62]. The atoms in the DIDP molecule contribute electrons to the metal and accept electrons from the metal [Citation57].
Table 10. Data of Fukui indices analysis.
The comparison table of previously studied ionic liquids and the present work are listed in .
Table 11. Comparison between the previous studies and the present study.
It is observed from the table that ionic liquid in the present study shows inhibition efficiency in the same order as those of reported molecules. The inhibition efficiency was observed to be 72.46% for 6061 aluminium alloy, which is considered an effective eco-friendly green inhibitor. The inhibition efficiency increases with the increase in the carbon side chains and the number of hetero atoms.
Conclusion
PDP study showed decreased corrosion current density in the presence of DIDP.
The percentage inhibition efficiency of DIDP decreased with an increase in temperature. The inhibition efficiency increased with the increase in the concentration of DIDP.
The maximum inhibition efficiency of DIDP on 6061 aluminium alloy was 78.38% for the concentration of 1000 ppm at 303 K.
The results of thermodynamic parameters were found to obey the Freundlich adsorption model and suggested physisorption.
SEM analysis showed a smooth surface after the addition of DIDP. EDAX analysis results also confirmed the interaction of DIDP on the 6061 aluminium alloy surface. AFM study showed a decrease in surface roughness after the addition of DIDP.
The computational study also helped to arrive at a suitable mechanism for adsorption of DIDP. DIDP acted as a potential green inhibitor in controlling the corrosion of 6061 aluminium alloy in 0.25 M HCl.
Acknowledgments
Mrs. Namitha Kedimar is grateful to MAHE Manipal for the Dr. TMA Pai scholarship. The authors are thankful to the Chemistry Department, MIT MAHE Manipal for the laboratory facilities. They also acknowledge Central Instrumentation Facilities, MIT MAHE Manipal.
Disclosure statement
No potential conflict of interest was reported by the authors.
References
- Guo H, Hua Y, Zhao L, et al. Structure investigations of rare-earth doped nano-particles – extracted from oxyfluoride glass ceramics by thermal induction and corrosion treatment. Developments in corrosion protection. InTech; 2014. doi: 10.5772/57213
- Martinez R, Olivares-Xomelt O, Likhanov N. Environmentally friendly corrosion inhibitors. developments in corrosion protection. InTech: 2014. doi: 10.5772/57252
- Abdelshafi NS, Sadik MA, Shoeib MA, et al. Corrosion inhibition of aluminum in 1 M HCl by novel pyrimidine derivatives, EFM measurements, DFT calculations and MD simulation. Arabian J Chem. 2022;15(1):103459–16. doi: 10.1016/j.arabjc.2021.103459
- John S, Joseph A. Quantum chemical and electrochemical studies on the corrosion inhibition of aluminium in 1 N HNO3 using 1,2,4-triazine. Mater Corros. 2013;64(7):625–632. doi: 10.1002/maco.201206782
- David Burleigh T. Corrosion of aluminum and its alloys. In: Handbook of Aluminum. CRC Press; 2003.
- Zhao Q, Tang T, Dang P, et al. The corrosion inhibition effect of triazinedithiol inhibitors for aluminum alloy in a 1 M HCl solution. Metals (Basel). 2017;7(2):44–55. doi: 10.3390/met7020044
- Bashir S, Thakur A, Lgaz H, et al. Corrosion inhibition efficiency of bronopol on aluminium in 0.5 M HCl solution: Insights from experimental and quantum chemical studies. Surf Interfaces. 2020;20:100542–100553. doi: 10.1016/j.surfin.2020.100542
- Bashir S, Lgaz H, Chung IM, et al. Effective green corrosion inhibition of aluminium using analgin in acidic medium: an experimental and theoretical study. Chem Eng Commun. 2021;208(8):1121–1130. doi: 10.1080/00986445.2020.1752680
- Dilasari B, Jung Y, Kwon K. Comparative study of corrosion behavior of metals in protic and aprotic ionic liquids. Electrochem Commun. 2016;73:20–23. doi: 10.1016/j.elecom.2016.10.009
- Nessim MI, Zaky MT, Deyab MA. Three new gemini ionic liquids: Synthesis, characterizations and anticorrosion applications. J Mol Liq. 2018;266:703–710. doi: 10.1016/j.molliq.2018.07.001
- Zunita M, Kevin YJ. Ionic liquids as corrosion inhibitor: From research and development to commercialization. Results Eng Elsevier B V. 2022;15:100562–100590. doi: 10.1016/j.rineng.2022.100562
- Zu P, Zhang Y, Li Y, et al. Structure design and performance investigation of 2-mercapto-5-methyl thiadiazole based ionic liquids as lubricants and corrosion inhibitors. Tribol Int. 2022;173:107682–107694. doi: 10.1016/j.triboint.2022.107682
- Qiang Y, Zhang S, Guo L, et al. Experimental and theoretical studies of four allyl imidazolium-based ionic liquids as green inhibitors for copper corrosion in sulfuric acid. Corros Sci. 2017;119:68–78. doi: 10.1016/j.corsci.2017.02.021
- Jiang Y, Liu Y, Gao S, et al. Experimental and theoretical studies on corrosion inhibition behavior of three imidazolium-based ionic liquids for magnesium alloys in sodium chloride solution. J Mol Liq. 2022;345:116998–117126. doi: 10.1016/j.molliq.2021.116998
- Manamela KM, Murulana LC, Kabanda MM, et al. Adsorptive and DFT studies of some imidazolium based ionic liquids as corrosion inhibitors for zinc in acidic medium. Int J Electrochem Sci. 2014;9(6):3029–3046. Internet. doi: 10.1016/S1452-3981(23)07989-0
- Zheng X, Zhang S, Li W, et al. Experimental and theoretical studies of two imidazolium-based ionic liquids as inhibitors for mild steel in sulfuric acid solution. Corros Sci. 2015;95:168–179. doi: 10.1016/j.corsci.2015.03.012
- Zhang QB, Hua YX. Corrosion inhibition of aluminum in hydrochloric acid solution by alkylimidazolium ionic liquids. Mater Chem Phys. 2010;119(1–2):57–64. doi: 10.1016/j.matchemphys.2009.07.035
- Arellanes-Lozada P, Olivares-Xometl O, Guzmán-Lucero D, et al. The inhibition of aluminum corrosion in sulfuric acid by poly(1-vinyl-3-alkyl-imidazolium hexafluorophosphate). Materials. 2014;7(8):5711–5734. doi: 10.3390/ma7085711
- Li X, Deng S, Fu H. Inhibition by tetradecylpyridinium bromide of the corrosion of aluminium in hydrochloric acid solution. Corros Sci. 2011;53(4):1529–1536. doi: 10.1016/j.corsci.2011.01.032
- Shetty SK, Shetty AN. Ionic liquid as an effective corrosion inhibitor on 6061 Al-15 Vol. Pct. SiC(p) composite in 0.1 M H2SO4 medium - an ecofriendly approach. Can Chem Trans. 2015;3:42–64.
- Charitha BP, Rao P. Electrochemical and adsorption studies for the corrosion control of 6061 Al alloy using eco-friendly inhibitor. Surf Engin Appl Electrochem. 2017;53(6):551–559. doi: 10.3103/S1068375517060035
- el Nagy HA, El Tamany EH, El Tamany EH, et al. Polymeric ionic liquids based on benzimidazole derivatives as corrosion inhibitors for X-65 carbon steel deterioration in acidic aqueous medium: Hydrogen evolution and adsorption studies. ACS Omega. 2020;5(47):30577–30586. doi: 10.1021/acsomega.0c04505
- Kumari PDR, Nayak J, Shetty AN. Corrosion inhibition of 6061 Al-15 vol. pct. SiC(p) Composite in sodium hydroxide solution by 4-amino-5-(4-nitrophenyl)-4H-1,2,4-triazole-3-thiol. Procedia Mater Sci. 2014;5:181–187. doi: 10.1016/j.mspro.2014.07.256
- Metikoš-Huković M, Babić R, Grubač Z. Corrosion protection of aluminium in acidic chloride solutions with nontoxic inhibitors. J Appl Electrochem. 1998;28(4):433–439. doi: 10.1023/A:1003200808093
- Deng S, Li X. Inhibition by Jasminum nudiflorum Lindl. leaves extract of the corrosion of aluminium in HCl solution. Corros Sci. 2012;64:253–262. doi: 10.1016/j.corsci.2012.07.017 Internet.
- Brett CMA. The application of electrochemical impedance techniques to aluminium corrosion in acidic chloride solution. J Appl Electrochem. 1990;20(6):1000–1003. doi: 10.1007/BF01019579
- Ennouri A, Lamiri A, Essahli M. Corrosion inhibition of aluminium in acidic media by different extracts of Trigonellafoenum-graecum L seeds. Portugaliae Electrochim Acta. 2017;35(5):279–295. doi: 10.4152/pea.201705279
- Kshama Shetty S, Nityananda Shetty A. Eco-friendly benzimidazolium based ionic liquid as a corrosion inhibitor for aluminum alloy composite in acidic media. J Mol Liq. 2017;225:426–438. doi: 10.1016/j.molliq.2016.11.037 Internet.
- Charitha BP, Rao P. Starch as an ecofriendly green inhibitor for corrosion control of 6061-Al alloy. J Mater Environ Sci. 2017;8:78–89.
- Fathima H, Pais M, Rao P. Anticorrosion performance of biopolymer pectin on 6061 aluminium alloy: Electrochemical, spectral and theoretical approach. J Mol Struct. 2021;1243:130775. doi: 10.1016/j.molstruc.2021.130775 Internet.
- Li E, Li Y, Liu S, et al. Choline amino acid ionic liquids as green corrosion inhibitors of mild steel in acidic medium. Colloids Surf A Physicochem Eng Asp. 2023;657:130541–130558. DOI:10.1016/j.colsurfa.2022.130541
- Preethi Kumari P, Shetty P, Rao SA. Electrochemical measurements for the corrosion inhibition of mild steel in 1 M hydrochloric acid by using an aromatic hydrazide derivative. Arabian J Chem. 2017;10(5):653–663. doi: 10.1016/j.arabjc.2014.09.005
- Arrousse N, Fernine Y, Al-Zaqri N, et al. Thiophene derivatives as corrosion inhibitors for 2024-T3 aluminum alloy in hydrochloric acid medium. RSC Adv. 2022;12(17):10321–10335. Internet. doi: 10.1039/D2RA00185C
- Elewady GY, El-Said IA, Fouda AS. Anion surfactants as corrosion inhibitors for aluminum dissolution in HCl solutions. Int J Electrochem Sci. 2008;3(2):177–190. doi: 10.1016/S1452-3981(23)15437-X
- Ashassi-Sorkhabi H, Ghasemi Z, Seifzadeh D. The inhibition effect of some amino acids towards the corrosion of aluminum in 1 M HCl + 1 M H 2 so 4 solution. Appl Surf Sci. 2005;249(1–4):408–418. doi: 10.1016/j.apsusc.2004.12.016
- Quraishi MA, Rafiquee MZA, Khan S, et al. Corrosion inhibition of aluminium in acid solutions by some imidazoline derivatives. J Appl Electrochem. 2007;37(10):1153–1162. doi: 10.1007/s10800-007-9379-0
- El-Hajjaji F, Ech-Chihbi E, Rezki N, et al. Electrochemical and theoretical insights on the adsorption and corrosion inhibition of novel pyridinium-derived ionic liquids for mild steel in 1 M HCl. J Mol Liq [Internet]. 2020;314:113737–113752. doi: 10.1016/j.molliq.2020.113737
- El Haleem SM A, El Wanees S A, El Aal EE A, et al. Factors affecting the corrosion behaviour of aluminium in acid solutions. I. nitrogen and/or sulphur-containing organic compounds as corrosion inhibitors for al in HCl solutions. Corros Sci [Internet]. 2013;68:1–13. doi: 10.1016/j.corsci.2012.03.021
- Ituen E, Akaranta O, James A. Evaluation of performance of corrosion inhibitors using adsorption isotherm models: An overview. Chem Sci Int J. 2017;18(1):1–34. doi: 10.9734/CSJI/2017/28976
- Sliem MH, Radwan AB, Mohamed FS, et al. An efficient green ionic liquid for the corrosion inhibition of reinforcement steel in neutral and alkaline highly saline simulated concrete pore solutions. Sci Rep. 2020;10(1):1–15. Internet. doi: 10.1038/s41598-020-71222-4
- Nesane T, Mnyakeni-Moleele SS, Murulana LC. Exploration of synthesized quaternary ammonium ionic liquids as unharmful anti-corrosives for aluminium utilizing hydrochloric acid medium. Heliyon. 2020;6(6):e04113–e04124. Internet. doi: 10.1016/j.heliyon.2020.e04113
- Yousefi A, Javadian S, Dalir N, et al. Imidazolium-based ionic liquids as modulators of corrosion inhibition of SDS on mild steel in hydrochloric acid solutions: Experimental and theoretical studies. RSC Adv. 2015;5(16):11697–11713. doi: 10.1039/C4RA10995C
- Ryl J, Wysocka J, Cieslik M, et al. Understanding the origin of high corrosion inhibition efficiency of bee products towards aluminium alloys in alkaline environments. Electrochim Acta [Internet]. 2019;304:263–274. doi: 10.1016/j.electacta.2019.03.012
- Chaubey N, Savita Singh VK, Singh VK, et al. Corrosion inhibition performance of different bark extracts on aluminium in alkaline solution. J Assoc Arab Univ Basic Appl Sci. 2016;22(1):38–44. Internet. doi: 10.1016/j.jaubas.2015.12.003
- Scendo M, Uznanska J. The effect of ionic liquids on the corrosion inhibition of copper in acidic chloride solutions. Int J Corros. 2011;2011:1–13. doi: 10.1155/2011/718626
- Li X, Deng S. Inhibition effect of Dendrocalamus brandisii leaves extract on aluminum in HCl, H 3PO 4 solutions. Corros Sci. 2012;65:299–308. doi: 10.1016/j.corsci.2012.08.033 Internet.
- Amin MA, Abd El-Rehim SS, El-Sherbini EEF, et al. The inhibition of low carbon steel corrosion in hydrochloric acid solutions by succinic acid. Part I. Weight loss, polarization, EIS, PZC, EDX and SEM studies. Electrochim Acta. 2007;52(11):3588–3600. doi: 10.1016/j.electacta.2006.10.019
- Bermúdez MD, Jiménez AE, Martínez-Nicolás G. Study of surface interactions of ionic liquids with aluminium alloys in corrosion and erosion–corrosion processes. Appl Surf Sci. 2007;253(17):7295–7302. doi: 10.1016/j.apsusc.2007.03.008
- Charitha BP, Rao P. Protection of 6061 Al-15%(v) SiC(P) composite from corrosion by a biopolymer and surface morphology studies. Prot Met Phys Chem Surf. 2016;52(4):704–713. doi: 10.1134/S2070205116040079
- Datta J, Samanta B, Jana A, et al. Role of Cl− and ions on the corrosion behavior of 20% SiCp reinforced 6061-Al metal matrix composite: A correlation between electrochemical studies and atomic force microscopy. Corros Sci. 2008;50(9):2658–2668. doi: 10.1016/j.corsci.2008.06.027
- Singh A, Ansari KR, Quraishi MA, et al. Corrosion inhibition and adsorption of imidazolium based ionic liquid over P110 steel surface in 15% HCl under static and dynamic conditions: Experimental, surface and theoretical analysis. J Mol Liq [Internet]. 2021;323:114608–114617. doi: 10.1016/j.molliq.2020.114608
- Abdelshafi NS, Sadik MA, Shoeib MA, et al. Corrosion inhibition of aluminum in 1 M HCl by novel pyrimidine derivatives, EFM measurements, DFT calculations and MD simulation. Arab J Chem Elsevier B V. 2022;15(1):103459–103485. doi: 10.1016/j.arabjc.2021.103459
- El-Rehim SS A, Hassan HH, Deyab MAM, et al. Experimental and theoretical investigations of adsorption andinhibitive properties of tween 80 on corrosion of aluminum alloy(a5754) in alkaline media. Z Phys Chem. 2016;230(1):67–78. doi: 10.1515/zpch-2015-0614
- Hajjaji F EL, Ech-Chihbi E, Salim R, et al. A detailed electronic-scale DFT modeling/MD simulation, electrochemical and surface morphological explorations of imidazolium-based ionic liquids as sustainable and non-toxic corrosion inhibitors for mild steel in 1 M HCl. Mater Sci Eng B Solid State Mater Adv Technol. 2023;289:116232–116248. doi: 10.1016/j.mseb.2022.116232
- Hsissou R, Benhiba F, Abbout S, et al. Trifunctional epoxy polymer as corrosion inhibition material for carbon steel in 1.0 M HCl: MD simulations, DFT and complexation computations. Inorg Chem Commun. 2020;115:107858–107869. doi: 10.1016/j.inoche.2020.107858
- Cao Z, Tang Y, Cang H, et al. Novel benzimidazole derivatives as corrosion inhibitors of mild steel in the acidic media. Part II: Theoretical studies. Corros Sci. 2014;83:292–298. doi: 10.1016/j.corsci.2014.02.025
- Singh A, Ansari KR, Banerjee P, et al. Corrosion inhibition behavior of piperidinium based ionic liquids on Q235 steel in hydrochloric acid solution: Experimental, density functional theory and molecular dynamics study. Colloids Surf A Physicochem Eng Asp. 2021;623:126708–126720. doi: 10.1016/j.colsurfa.2021.126708
- Lgaz H, Salghi R, Masroor S, et al. Assessing corrosion inhibition characteristics of hydrazone derivatives on mild steel in HCl: Insights from electronic-scale DFT and atomic-scale molecular dynamics. J Mol Liq [Internet]. 2020;308:112998–113011. doi: 10.1016/j.molliq.2020.112998
- Li X, Deng S, Xie X. Experimental and theoretical study on corrosion inhibition of oxime compounds for aluminium in HCl solution. Corros Sci. 2014;81:162–175. doi: 10.1016/j.corsci.2013.12.021. Internet.
- Hsissou R, Abbout S, Benhiba F, et al. Insight into the corrosion inhibition of novel macromolecular epoxy resin as highly efficient inhibitor for carbon steel in acidic mediums: Synthesis, characterization, electrochemical techniques, AFM/UV–visible and computational investigations. J Mol Liq. 2021;337:116492–116509. doi: 10.1016/j.molliq.2021.116492
- Mostafa MA, Ashmawy AM, Reheim MAMA, et al. Molecular structure aspects and molecular reactivity of some triazole derivatives for corrosion inhibition of aluminum in 1 M HCl solution. J Mol Struct. 2021;1236:130292–130310. doi: 10.1016/j.molstruc.2021.130292
- Guo L, Kaya S, Obot IB, et al. Toward understanding the anticorrosive mechanism of some thiourea derivatives for carbon steel corrosion: A combined DFT and molecular dynamics investigation. J Colloid Interface Sci. 2017;506:478–485. doi:10.1016/j.jcis.2017.07.082