ABSTRACT
Clinical treatment of hepatocellular carcinoma (HCC) is notoriously tricky because radiofrequency ablation (RFA) might encourage metastasis of remaining HCC. . The therapeutic efficacy of arsenic (A) and promising anticancer drug gemcitabine (G)-loaded zeolitic imidazolate framework-8 nanocomposites (AG@ZIF-8 NCs) on tumour remnants wasinvestigated. Results demonstrated that RFA significantly increased proliferation, induced metastasis, and triggered angiogenesis in recurrent tumours. We show that significant angiogenesis following RFA can increase the enhanced permeability and retention (EPR) effects and emphasize ZIF-8 carriers in recurrent tumours. e effectively fabricated biocompatible AG@ZIF-8 NCs. And they were superior to free gemcitabine in inhibiting cell proliferations, inducing apoptosis, blocking cell invasion and migration, and reversing in vitro EMT following sublethal heat treatment. In addition, AG@ZIF-8 NCs showed significantly improved therapeutic efficiency by reducing residual tumour development and in vivo metastasis compared to free gemcitabine. This study establishes a new standard for managing HCC that persists after RFA.
Introduction
Liver cancer that begins in the liver is an essential issue for the public. The third most prevalent cause of mortality from cancer, hepatocellular carcinoma (HCC), is in the top five most common neoplasms worldwide[. Curative and palliative treatments are the two main categories typically used to describe HCC care. Although surgical excision is often successful, its efficacy drops dramatically when dealing with many tumours or metastases [Citation1]. Developing more effective chemotherapeutic drugs is paramount to increasing the survival rate of patients with advanced or recurrent HCC following surgical therapy. Liver transplantation, surgical resection, and radiofrequency ablation (RFA) are all effective first-line therapy for patients with early-stage HCC. Although surgical resection and liver transplantation are considered the gold standard in treatment, they are not always an option due to donor shortages, compromised liver function, or inability to fulfil severe (Milan) selection criteria [Citation2]. Repetitive foam ablation (RFA) is a minimally invasive and repetitive therapy with low procedure-related morbidity, improving cost-effectiveness and quality of life [Citation3–5]. Those waiting for a liver transplant or not candidates for surgical resection may also be candidates for RFA. Numerous studies have shown that for a single small HCC of ≤3 Cm, the survival results with RFA and surgical excision are comparable [Citation6]. Local control and disease-free survival appear to be reduced by RFA compared to surgical resection. Some clinical centres have also shown that HCC exhibits more aggressive biological behaviour following RFA, with the neoplastic development and metastasis of the primary tumour occurring more quickly inside the liver [Citation7]. Although our understanding of the processes driving HCC advancement following RFA remains limited, developing tailored treatment with the potential to enhance patient outcomes is urgently needed [Citation8].
The clinical use of chemotherapeutic drugs is widespread [Citation9]. Acute promyelocytic leukaemia has been treated with gemcitabine alone and with other drugs. As2O3 therapy has also made steady progress in treating solid tumours like HCC. Cancer cells that have survived treatment may benefit from being exposed to As2O3. This property of As2O3 allows it to improve therapy with minimal ratios of recurrence and metastasis than conventional antitumor drugs by decreasing the malignancy behaviour and metastatic consequence of extant tumour cells in chemotherapy [Citation10]. However, the drug’s quick clearance in blood circulation makes it challenging to produce efficient As2O3 build-up inside a solid tumour [Citation11]. The therapeutic activity can be maintained with a high dosage, but this comes with the risk of systemic side responses. The primary goal of most investigations has been to figure out how to keep a therapeutic concentration of As2O3 within target solid tumour tissue for a long time with few systemic adverse responses. One potential therapeutic option is localized chemotherapy [Citation12]. Direct intratumoral injection, one kind of localized chemotherapy, has garnered much interest. The retention period of chemotherapeutic drugs at the dosage site can be increased via local drug delivery systems, leading to greater continuous effectiveness and fewer adverse responses in healthy organs and tissues [Citation13–15].
The clinical benchmark for treating PDAC is the chemotherapeutic agent gemcitabine (GEM), a derivative of the nucleoside deoxycytidine. GEM is absorbed into replicating DNA (and RNA) and stops chain elongation, leading to cell death in rapidly reproducing cancer cells [Citation16–18]. Even though this drug is widely used to treat PDAC patients, the therapeutic effects are mainly disappointing, with only a 6.7-month increase in median survival for PDAC patients. GEM’s therapeutic efficacy is severely hindered by its inherent limitations, such as its short half-life in circulation (8–17 minutes), fast deactivation and excretion, the emergence of drug resistance, and the risk of severe adverse effects [Citation19]. Therefore, there is a significant incentive to create straightforward yet effective methods of fully harnessing GEM, as doing so has the potential to increase this therapeutic class’s applicability in treating life-threatening pancreatic cancer [Citation20–22].
An example of a metal-organic framework (MOF) is the zeolite imidazole framework (ZIF). Zinc ion and 2-methylimidazole form ZIF-8 were investigated because of their high thermal stability and specific surface region. ZIF-8 crystals are a cage-type of frameworks, taking the shape of rhombic dodecahedrons [Citation23]. Sodalite cages have an aperture of 3.4 nm and a pore diameter of 11. 6 nm. Some examples of synthesis techniques are the simple reaction technique, the solvothermal technique, the electrodeposition-solvent synthesis technique, the microfluidic synthesis technique, etc [Citation24–26]. In situ, encapsulation of drugs bigger than the pore diameter of ZIF-8 May be employed to increase drug loading capacity, and the drugs-coloaded ZIF-8 is primarily generated using the single-pot approach [Citation27]. Cancer treatment modalities such as immunotherapy, photoacoustic imaging (PAI), computed tomography (CT), starvation therapy (ST), magnetic resonance imaging (MRI), gene therapy (GT), chemotherapy and phototherapy have all been identified as potential applications for ZIF-8-based nanoplatforms. When applied to tumour treatment, it has many beneficial characteristics: (1) a highly porous and a large-targeted surface region; (2) good toxic profile; (3) decomposition in acidic environments; (4) production of ROS; (5) the enzymatic cleavage reaction of nucleases catalysed by Zn2+ as a cofactor; (6) orientation of autophagy. ZIF-8 can be utilized as a self-assembly framework to prevent drug leakage from a nanoreactor. Unfortunately, ZIF-8 tends to polymerize and has difficulty dispersing in water [Citation28]. Therefore, hyaluronic acid (HA) or polyethylene glycol (PEG) is commonly used to modify the surface of ZIF-8. Ligand adjustment on the surface of ZIF-8 is required to enable active transport to tumour cells. Common ligands include Arg-Gly-Asp (RGD) peptide, folic acid (FA), lactobionic acid (LA), and heparan sulphate (HA). Erythrocyte membrane or cancer cells coating the ZIF-8 allows for prolonged blood circulation and immunological evasion via biomimetics on the drug’s surface [Citation29]. However, the features of ZIF-8, such as the primary methods of its impacts on in vivo long-term toxicity and tumour growth, have not received enough attention in the available research. More in vitro and in vivo study of ZIF-8 is required before its clinical translation as a drug delivery system [Citation30].
We hypothesized that significant post-RFA angiogenesis could increase the EPR effect and NCs accumulation in remaining tumours, leading us to construct ZIF-8-based NCs to administer gemcitabine. Finally, we used a sublethal heat model to test the effectiveness of these NCs in vitro against surviving tumour cells, assessing their capacity to limit cell growth, induce cell death, reduce invasion and migration, and suppress epithelial – mesenchymal transition (EMT). The effectiveness on tumour development and in vivo metastasis was further evaluated using tumour xenografts models. When applied to the treatment of HCC that has not been completely eradicated, this innovative approach shows great promise.
Experimental section
Reagents and methods
All initial reagents were used without further purification. Deionized (DI) water with a resistivity of 18.2 MΩ·cm was utilized throughout all the solution preparations. Roswell Park Memorial Institute (RPMI-1640), penicillin/streptomycin, foetal bovine serum (FBS) and other reagents related to cell culture were supplied by Gibco BRL (Grand Island, U.S.A.). NaAsO2 and Accutase® solution were obtained from Sinopharm Chemical Reagent Co., Ltd. (Shanghai, China). Endothelial cell medium (ECM) and Anti-E-cadherin and Anti-vimentin) were purchased from Macklin (Shanghai, China). Anti-N-cadherin and anti-CD34 were purchased from Thermo Fisher Scientific. A Cell Counting Kit-8 (CCK-8) was purchased from MP (Santa Ana, CA, U.S.A.). Polyethylene glycol (2000), 2-methylimidazole, and Zn(NO3)2·6 H2O were all purchased from Merck (Kenilworth, NJ, U.S.A.). Indocyanine green (ICG) and fluorescein isothiocyanate (FITC) was purchased from Sangon Biotech (Shanghai, China). Calcein AM, DAPI and Annexin V-FITC/PI staining kit were obtained from the Beyotime Institute of Biotechnology (Haimen, China). Hepatocellular SMMC7721, Hep3B cells and HCC-LM3) were purchased from Nanjing KeyGEN Biotech Co., Ltd. (Nanjing, China). SMMC7721, Hep3B and HCC-LM3 cells were cultured in a Roswell Park Memorial Institute (RPMI-1640) supplemented with 10% FBS and 1% penicillin/streptomycin at 37°C, 5% CO2 and 100% humidity.
Preparation of AG@PEG/ZIF-8
A methanol solution (7 mL) containing zinc nitrate hexahydrate (200 mg). After blending the solution for 15 minutes, dimethyl imidazole methanol solution (75 mg/mL in 7 mL) was incorporated drop by drop. Solution B was obtained by centrifuging a white product and rinsing twice with methanol before being diluted to 2 mL with ultrapure water. After combining solutions A and B for 15 minutes, we added NaAsO2 solution (60 mg/mL in 1 mL), 1 mL of gemcitabine (30 mg/mL) and 30 µL of COOH-PEG-COOH (40 mg/mL) to generate AG@PEG/ZIF-8. By omitting the NaAsO2 step, ZIF-8 NCs were successfully synthesized [Citation31].
Characterization
Transmission electron microscopy (TEM) experiments were performed on a JEM 2100Plus microscope (JEOL, Japan). Ultraviolet – visible (UV – vis) absorption and photoluminescence spectra were recorded with a Shimadzu UV-3600 spectrometer and Shimadzu RF-5301PC spectrofluorophotometer (RF-5301PC, Shimadzu, Japan), respectively. Hydrodynamic sizes and zeta potentials were measured on a Zetasizer instrument (Nano ZS, Malvern). Fourier transform infrared (FTIR) spectra were collected using a Shimadzu IR Prestige-21 FTIR spectrometer. A BRUKER D8-discover XRD system characterised powder X-ray diffraction (XRD). SPECSLab and Kratos SUPRA recorded X-ray photoelectron spectra (XPS).
In vitro drug release
At room temperature, 20 mg of AG@PEG/ZIF-8 was dissolved in phosphate-buffered saline (10 mL) (pH = 5.5 and 7.5) to investigate the nanomedicine’s release profile in vitro. Liquid (1 mL) was placed into the centrifugal tube after being agitated for a set period, and the supernatant was collected after 15 minutes of centrifugation. The pH of the buffer solution was adjusted by adding 1 mL of freshly prepared PBS at the appropriate level. ICP-OES confirmed the quantity of arsenic that was emitted [Citation32]. Data represented as mean ± SD of three independent experiments (n = 3).
Sub lethally heated cell model
A cell model with temperatures just below lethal was developed based on published research. In this case, HCC cells were plated. After 24 h, the medium in the cultures was swapped for new, warmed media. The dishes were instantly wrapped in parafilm and placed in the water bath at 37°C, 42°C, 44°C, 46°C, and 48°C. The plates were heated for 15 minutes and then placed in an incubator at 37°C with 5% CO2 for 24 h [Citation33]. Data represented as mean ± SD of three independent experiments (n = 3).
Colony forming test
Hep3B cells grown in the 6-well plates needed to be cultured for 24 h and then incubated with fresh medium containing gemcitabine, ZIF-8 NCs and AG@ZIF-8 NCs for 48 h. After seven days, cultured cells were restored in cold methanol and dyed with crystal violet. Six parallel wells were tested for each sample to give a mean value and standard deviation [Citation34]. Data represented as mean ± SD of three independent experiments (n = 3).
Cell viability assay and apoptosis investigations
Cells grown in the 96-well plates needed to be cultured for 24 h and then incubated with fresh medium containing gemcitabine, ZIF-8 NCs and AG@ZIF-8 NCs for 48 h. CCK-8 determined the cell viability after four h incubation. At last, the absorbance of each well at 450 nm was measured by a Thermo Scientific Multiskan MK3 ELISA reader (Waltham, MA). Six parallel wells were tested for each sample to give a mean value and standard deviation [Citation35–39]. Hep3B cells were seeded in the 35 mm glass culture dish and incubated for 24 h. Then the medium was replaced with 2 mL of fresh medium containing gemcitabine, ZIF-8 NCs and AG@ZIF-8 NCs (10 μg/mL) for 12 h. Then, cells were washed with PBS and stained by Calcein-AM and PI for 30 min. The fluorescent microscope was employed to image the stained cells. Cells (untreated cells as Control, were seeded in 6-well plates (2 × 105 cells per well) and incubated in 2 ml of RPMI medium containing gemcitabine, ZIF-8 NCs and AG@ZIF-8 NCs for 24 h. Subsequently, the medium was replaced with fresh RPMI medium containing supplements. After co-incubation for 24 h, the cells were digested by trypsin and collected [Citation40–44]. Data represented as mean ± SD of three independent experiments (n = 3). Cell survival was assessed by CCK-8 assay after treatment with a similar process of apoptosis analysis.
Cellular uptake experiment
The cellular uptake of NCs by Hep3B cells was next checked to prove their targeting ability. Hep3B cells were seeded in a 12-well plate at a density of 1 × 105 cells per well with 1 mL of RPMI. After overnight incubation, the medium was replaced with 1 mL of fresh RPMI containing FITC@ZIF-8 NCs for 45 min. After 4 h and 8 h incubation, the cells were washed with PBS three times, trypsinized, counted, and digested by aqua regia (nitric acid/hydrochloric acid, v/v = 1: 3) for 4 h. Data represented as mean ± SD of three independent experiments (n = 3).
Migration and invasion assays
The trans-well polycarbonate filter inserts with a diameter of 6 mm and a pore size of 8 µm (Corning) for 24-well plates were used for the invasive assay [Citation45,Citation46]. The upper chambers of trans-well inserts were pre-coated with 100 μl of Matrigel (1:8 dilution by serum-free RPMI) for 4 h at 37°C and then placed into a 24-well plate. Cells were suspended in 200 μl of serum-free RPMI and seeded into upper chambers at a density of 2 × 105 cells per well. 600 µl of RPMI (10% FBS) was added to the lower chambers to form a chemoattractant environment. After incubation at 37°C for 24 h, the non-invasive cells remaining in the upper chambers were removed by cotton swabs. The cells that invaded the lower chambers were rinsed with PBS twice, fixed with 4% paraformaldehyde for 20 min, and stained with 0.1% crystal violet for 10 min. Finally, the invading cells were visualized by an inverted microscope (Olympus CKX53). Data represented as mean ± SD of three independent experiments (n = 3).
Wound healing assay
Cells were seeded in 6-well plates (2 × 105 cells per well). After growing to 90% confluence, the cell layers were scratched with a sterile 200 µl micropipette tip and were washed twice with PBS to remove the debris and cultured in fresh RPMI medium (1% FBS) containing samples [Citation47]. Healing of cellular scratches within 24 h was monitored by a live cell imaging system (Olympus CKX53) under a magnification of 100. Data represented as mean ± SD of three independent experiments (n = 3).
Effect of antitumor growth and antitumor metastasis in vivo
The well-grown cells were digested, collected, and prepared into a cell suspension with PBS. BALB/c mice with good growth conditions at 6–8 weeks were inoculated subcutaneously (s.c.) with 106 cells in the abdomen. When the tumour grew to about 80 mm3, the mice were randomized (n = 5) and treated with gemcitabine, AG@ZIF-8 NCs and ZIF-8 NCs. An i.v administered the treatment, injection every four days for five doses. The tumour volume and mice’s body weight were recorded every other day. After 21 days, all mice were sacrificed according to animal ethics, and tumours were dissected and weighed. The equation estimated the tumour volume: V = (the shortest diameter)2 × (the longest diameter) × 0.5. The tumour inhibition rate (IR) was calculated as a formula estimated by the equation: IR (%) = (1 - tumour weight in the experimental group (g)/tumour weight in the control group (g)) × 100%.
The 21 days after RFA, the lungs, liver, kidney, spleen, heart, and tumour were removed for histological investigations. Organs and tissues in each group were fixed using a 4% paraformaldehyde buffer, embedded in paraffin, and cut into 5 μm slices. These sections were stained with haematoxylin and eosin (H&E) and examined under a light microscope (CKX53, Olympus, Tokyo, Japan).
Immunohistochemistry staining
Tumour sections were removed and fixed in 4% paraformaldehyde at RT for 30 min, rinsed thrice with PBS, embedded in paraffin, and then cut to a thickness of 5 μm. The sections were incubated with 1% bull serum albumin (BSA) in phosphate-buffered saline tween-20 (PBST) for 30 min to block unspecific binding of antibodies and incubated with primary antibody (anti-N-cadherin and anti-E-cadherin) for 2 h at 37°C in a humidified chamber, followed by incubation with the corresponding secondary antibody for 1 h at 37°C in the dark. Finally, the samples were analysed using a microscopy system. Data represented as mean ± SD of three independent experiments (n = 3).
Statistical analysis
Data are presented as mean ± standard deviation (SD). The statistical significance between groups was assessed using one-way ANOVA (Bonferroni’s posthoc model) with GraphPad Prism 9.0 software. Statistical significance was established at P < 0.05, where * P < 0.05; ** P < 0.01; *** P < 0.001, and **** P < 0.0001.
Results
Fabrication and characterization of the nanocomposites
depicts the AG@PEG/ZIF-8 synthesis process. 2-methylimidazole and Zn(NO3)2·6 H2O were combined in methanol to create the ZIF-8 nanocarrier. AG@PEG/ZIF-8 was then successfully synthesized by loading NaAsO2, GEM, and COOH-PEG-COOH incorporated into ZIF-8 in the aqueous medium. ZIF-8 NCs with a mean size of 74.13 ± 1.35 nm were successfully fabricated (), as shown by transmission electron microscopy (TEM) analysis (). Additionally, the negatively charged surface of NaAsO2 and -COOH-PEG-COOH caused the zeta potential ZIF-8 to shift from 31.58 ± 2.35 mV to −21.54 ± 2.57 mV (). According to the results, NaAsO2 in AG@ZIF-8 NCs may be loaded by electrostatic adsorptions. Also, O-As, C-H, and -OH-As vibrations in the PEG were ascribed to four additional peaks in the FTIR spectral analysis of AG@PEG/ZIF-8 () at 713 cm−1, 641 cm−1, 540 cm−1, and 2900 cm−1. Furthermore, the PXRD findings confirmed that the distinctive bands of the synthesized nanocomposites (AG@PEG/ZIF-8, AG@ZIF-8, and ZIF-8) coincided strongly with the ZIF-8 simulation, demonstrating that the clear morphology of ZIF-8 was not significantly changed after alteration ().
Figure 2. Fabrication and characterization of ZIF-8, AG@ZIF-8 and AG@PEG/ZIF-8 nanocomposites. a and b) TEM images of ZIF-8 and AG@PEG/ZIF-8. Scale bar= 100 nm. c) Zeta potential of ZIF-8, AG@ZIF-8 and AG@PEG/ZIF-8 NCs. d) FTIR spectra of ZIF-8, AG@ZIF-8 and AG@PEG/ZIF-8 NCs (The line dots represent the important peaks). e) PXRD spectra of ZIF-8, AG@ZIF-8, AG@PEG/ZIF-8 NCs and simulated ZIF-8 (The line dots represent the important peaks).
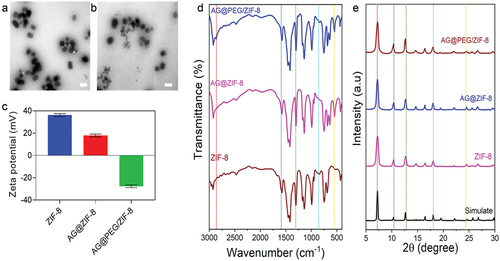
RFA encouraged the growth, metastasis, and angiogenesis
We subjected Hep3B and SMMC7721 cells to RFA-like conditions in vitro by heating them to 42°C, 44°C, 46°C, and 48°C for 15 minutes. Within 24 h of exposure to 46 and 48°C, there was a dramatic drop in cell viability (). Spindle forms were observed in cells heated to 44°C 3 days later, while heat treatment at 46°C and 48°C generated vacuolar alterations (). Cell proliferation was shown to be increased at 44°C compared to 42°C in colony formation experiments while raising the temperature to 46°C and 48°C had an inhibition (). At 44°C heat treatment raised the cell migration ratio of SMCC7721 and Hep3B cells by 69.25 ± 9.47% and 36.87 ± 6.48%, respectively (), and the cell invasion ratio of SMCC7721 and Hep3B cells by 132.47 ± 10.25% and 248.21 ± 13.59%, as measured by transwell assays (). However, cell migration and invasion were suppressed after cells were exposed to 46 and 48°C (). Considering these findings, future research utilized a sublethal heating temperature of 44°C while working with Hep3B and SMMC7721 cells.
Figure 3. RFA induced the growth, metastasis, and EMT of HCC. a) Colony formation of SMMC7721 and Hep3B cells after heating at various temperatures for 15 min. b) Respective cell numbers of the colony. b) Migration of SMMC7721 and Hep3B cells after heating at different temperatures for 15 min. c) Respective ratio of migration of SMMC7721 and Hep3B cells. e) Invasion of SMMC7721 and Hep3B cells after heating at different temperatures for 15 min. c) Respective ratio of invasion of SMMC7721 and Hep3B cells. Scale bar= 100 µm. Data represented as mean ± SD of three independent experiments (n = 3). The significance levels were set at probabilities of **p < 0.01.
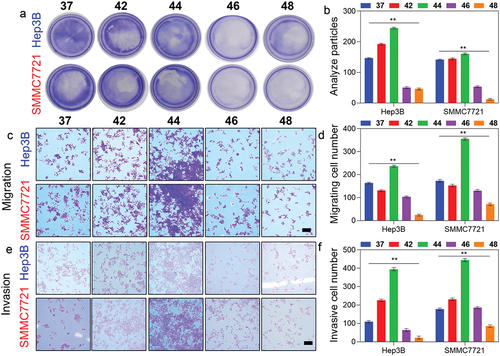
NCs reduced the proliferation and induced the apoptosis
Cellular absorption by HCC cells was tracked using FITC-labelled ZIF-8 NCs. After around 40 min of incubation, Hep3B cells internalized some FITC@ZIF-8 NCs, and these NCs were dispersed throughout the cells by 8 h (). In addition, three different hepatocellular cells and L02 hepatocytes showed low toxicity levels when exposed to ZIF-8 nanocarrier doses up to 150 µM (). Besides, HCC cells and hepatocytes were treated with gemcitabine or AG@ZIF-8 NCs at a range of AG concentrations for 48 h, and IC50 was determined. As shown in , the results demonstrated that AG@ZIF-8 NCs were more efficient than gemcitabine in inhibiting the viability of HCC cells. Compared to gemcitabine, the IC50 values for AG@ZIF-8 NCs in SMCC7721 and Hep3B cells were 7.98 ± 0.29 µM, and 5.01 ± 0.97 µM, respectively (). shows that the IC50 for AG@ZIF-8 NCs in L02 was 57.48 ± 2.14 µM. The significantly reduced cytotoxicity that their much-decreased IC50 values demonstrated AG@ZIF-8 NCs generated in normal L02. In a consistent colony formation experiment, AG@ZIF-8 NCs were shown to elicit a more pronounced reduction of colony formation in sublethally heated SMMC7721 and Hep3B cells than that achieved with gemcitabine And as shown by double labelling of live and dead cells in , AG@ZIF-8 NCs were more efficient than gemcitabine in killing HCC cells. The ZIF-8 nanocarrier had a minimal effect in any of the studies. Our data revealed that AG@ZIF-8 NCs had good therapeutic effectiveness against sublethal heating of in vitro HCC cells.
Figure 4. Cellular uptake of FITC@ZIF-8 NCs by Hep3B cells at 40 min, 4 h and 8 h. Scale bar= 100 µm.
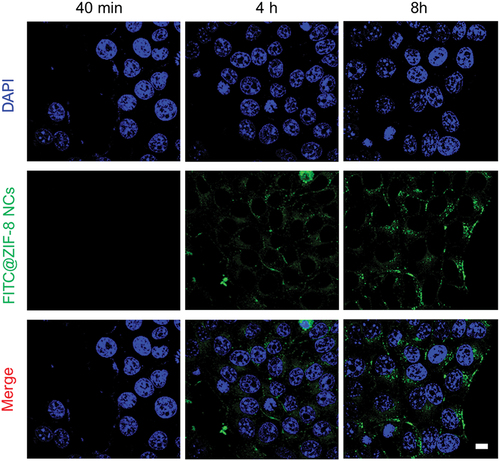
Figure 5. Ag@ZIF-8 NCs reduced the proliferation and stimulated the apoptosis of sublethally heated cells in vitro. a-c) Cell viabilities of L02, SMMC7721 and Hep3B after incubation with 48 h treatment with GEM and AG@ZIF-8 NCs. d-g) L02, MHCC97H, SMMC7721, and Hep3B cells after incubation for 48 h. Data represented as mean ± SD of three independent experiments (n = 3). The significance levels were set at probabilities of *p < 0.05, **p < 0.01, and ***p < 0.001.
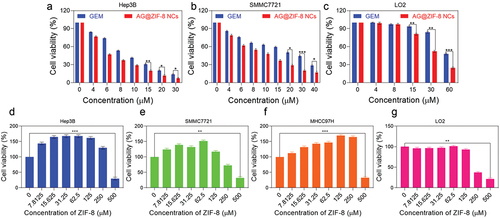
Ag@ZIF-8 NCs reduced the migration, invasion
We determined the AG@ZIF-8 NCs affected the migration and invasion of HCC cells subjected to sublethal heating, as these cells are characterized by increased metastatic capacity. AG@ZIF-8 NCs showed more efficiency in inhibiting the migration of sublethal-heated HCC cells than gemcitabine (), as measured by the wound healing ratio of the monolayer at the respective periods. Additionally, the transwell migration results showed that AG@ZIF-8 NCs inhibited the cell migration of sublethally heated SMMC7721 and Hep3B cells by 56.87 ± 2.10% and 80.24 ± 2.21%, respectively, while gemcitabine reduced their migration percentage by 31.25 ± 7.48% and 58.47 ± 2.19% (). Transwell invasion assays displayed that AG@ZIF-8 NCs significantly inhibited the invasiveness of sublethally heated SMMC7721 and Hep3B cells (91.24 ± 0.29% and 95.47 ± 1.12%, respectively, compared to gemcitabine relative inhibitory rates of 65.13 ± 2.97% and 70.14 ± 2.58%; ). SMMC7721 and Hep3B cells treated with ZIF-8 NCs did not undergo noticeable alterations after exposure to sublethal heat. In conclusion, these results showed that AG@ZIF-8 NCs were superior to other nanocomposites in inhibiting tumour cell migration, invasion, and EMT following sublethal heating.
Figure 7. Ag@ZIF-8 NCs reduced the migration of sublethally heated Hep3B and SMMC7721 cells. Wound healing assay of sublethally heated cells after incubation with free GEM, ZIF-8 NCs, and AG@ZIF-8 NCs for 24 h. Scale bar=100 µm. Data represented as mean ± SD of three independent experiments (n = 3). The significance levels were set at probabilities of **p < 0.01.
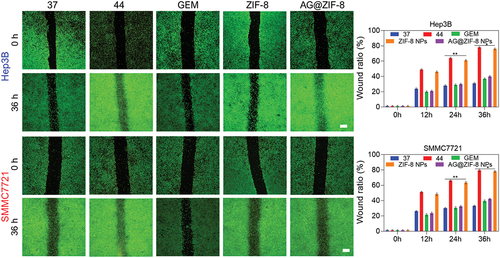
Figure 8. Ag@ZIF-8 NCs reduced the migration of sublethally heated Hep3B and SMMC7721 cells. a and c) Typical images of the migration and invasion of sublethally heated SMMC7721 and Hep3B cells after the treatment. Scale bar=100 µm. b and d) Respective quantitative evaluation. Data represented as mean ± SD of three independent experiments (n = 3). The significance levels were set at probabilities of **p < 0.01..
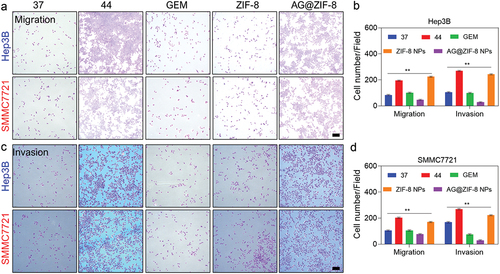
NCs reduced residual tumour growth and metastasis
Nude mice with HCC tumours were used to test whether the encouraging in vitro effectiveness of AG@ZIF-8 NCs and the higher enhancement in residual tumours after RFA translate into therapeutic benefits in vivo. After tumours reached 200–400 mm3, we randomly divided 20 mice (5 per group) into 4 groups and treated them to RFA to determine the results. From day 5 to day 21 post-RFA, the mice received intravenous injections of free gemcitabine, AG@ZIF-8 NCs, or ZIF-8 NCs (). The tumour volumes were measured throughout time, and the subsequent growth curvature is shown in . The AG@ZIF-8 NCs group had the most striking suppression of residual tumour development, in contrast to the ZIF-8 nanocarrier group. Notably, gemcitabine partly slowed tumour development, although its anticancer impact was less potent than the AG@ZIF-8 NCs. Dissected tumour tissue pictures taken on day 21 showed that the tumour size in the AG@ZIF-8 NCs group was much smaller than in the other groups. In contrast, the tumour size in the free gemcitabine group was smaller than in the control group but substantially higher than in the AG@ZIF-8 NCs group (). The AG@ZIF-8 NCs group also had a greater antitumor rate than the free gemcitabine group (61.25 ± 3.15%. AG@ZIF-8 NCs also appeared to have little adverse effects, with no significant changes in body weight among treated mice (). No major organs showed apparent histological alterations upon haematoxylin and eosin (HE) staining ().
Figure 9. Ag@ZIF-8 NCs reduced the in vivo metastasis tumour growth. a) Pictures of tumours removed 21 days after various treatments were administered. b) Tumor growth curve. c) Body weight. d) Tumor weight. e) Immunohistochemical staining of E-cadherin and N-cadherin in tumour tissues 21 days. Scale bar=100 µm. F) Quantification of the H-score. Data represented as mean ± SD of three independent experiments (n = 5). The significance levels were set at probabilities of *p < 0.05, **p < 0.01, and ***p < 0.001.
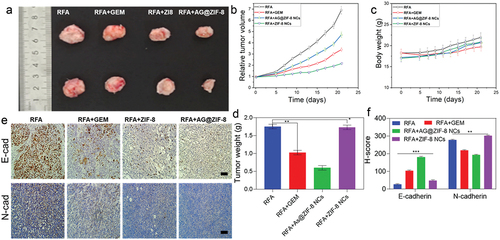
Figure 10. After treatment with different formulations, HE stains images of tissue in the mice’s organs (heart, liver, spleen, lung, and kidney). Scale bar=100 µm.
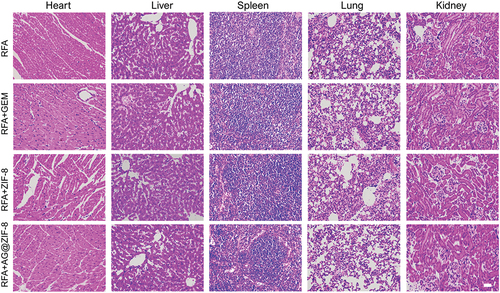
We established a liver metastasis model in mice by injecting cells that had been sublethally heated to examine the drug’s effect of AG@ZIF-8 NCs on metastatic suppressions of SMMC7721 and Hep3B cells in vivo. This study demonstrated that AG@ZIF-8 NCs were superior to gemcitabine in inhibiting the spread of tumours in these animals (). Subcutaneous tumours were analysed immunohistochemically across all groups, and the AG@ZIF-8 NCs consistently produced the most striking reduction of N-cadherin expression and the highest elevation of E-cadherin expression (). Based on these findings, using AG@ZIF-8 NCs to treat tumour remnants offers promise as a viable method with high biocompatibility.
Discussion
The fast growth of any remaining tumour following RFA is still a major clinical obstacle. Several variables increase the likelihood of RFA, including the closeness of vital organs or tissues to the tumour, its irregular form or volume, and the heat sink impact of primary capillaries in its immediate vicinity [Citation48]. To be technically adequate, the ablation zone must cover the tumour and a buffer zone of at least 5 mm of healthy tissue on all sides. However, current clinical imaging tools do not always make it possible to see the precise ablation boundary [Citation49]. Because of this ambiguity, the rate at which tumours persist following RFA is higher than it should be. Even at temperatures below those required to kill, RFA can confer a more aggressive phenotype on tumours that survive [Citation50].
The invasion and metastasis of SMMC7721 and Hep3B cells that have not been eradicated by chemotherapy rely on EMT, which RFA triggers. EMT-inducing transcription factors (EMT-TFs), including twist1, slug and snail, have been shown in many studies to be upregulated in RFA-treated cancer survivors. Matrix metalloproteinase expression is induced, lateral cell-cell junction genes are repressed, apical-basal polarity genes are suppressed, and the epithelial-mesenchymal transition transcription factors (EMT-TFs) orchestrate the process. Importantly, cells have front-rear polarity, and remodelling occurs in cell contacts with other extracellular matrices and cells; this may prompt the remaining hepatocellular cells to become mobile and spread. Our findings suggest that the sublethally heated cells underwent remodelling, contributing to their enhanced ability to migrate and invade. Difficult therapeutic obstacles include the significant metastatic potential and chemo-resistance these malignant translations eventually give to remnant HCC cells.
Increased angiogenesis is one of the most important contributors to the malignant development of residual HCC following RFA. In previous research, RFA has been found to cause hypoxia in the tumour’s transition zone. After RFA, proangiogenic factors, including hypoxia-inducible factor-1 and vascular endothelial growth factor (VEGF), induce neovascularization surrounding the necrotic lesion [Citation51]. Consistent with the literature, we found RFA-induced tubule formation in HUVECs in vitro. In addition, we found that RFA in vivo increases the fraction of blood vessels in cancer that has not been wholly eradicated. Because of this angiogenesis, the remaining tumours benefit from increased nutrition and oxygen, which leads to fast development. The negative consequences of EMT are compounded by the fact that newly created tumour arteries have undeveloped morphological characteristics and jumbled vascular systems, which may aid in the intravasation of remaining tumour cells and their subsequent colonization of distant sites [Citation52]. These variables significantly limit treatment efficacy by promoting the growth of any remaining tumours.
The aftereffects of RFA remain a problem, even though significant efforts have been undertaken to eradicate any remaining tumours after RFA. Considering this, we investigate that focusing on the natural features of tumour remnants may provide a more successful strategy. The EPR effect describes the selective permeability of solid tumours and the retaining of lipid particles and macromolecular compounds. Several pathophysiological and anatomical features of solid tumours lead to the development of EPR [Citation53].
These include the high concentrations of vascular mediators’ immature, impaired lymphatic function, and abnormal vascular structure, such as interleukins, proinflammatory cytokines, prostaglandins nitric oxide, and bradykinin. Numerous ways have been used to increase the EPR effects, most of which use angiogenic drugs to boost angiogenesis [Citation53]. Vascular promotion strategy-inspired substantial angiogenesis induction following RFA appears to be an appropriate technique for enhancing the nanodrug enrichment and EPR effect. We built a model of unilateral tumour growth using RFA and found that the ICG@ZIF-8 NCs significantly improved in tumours that persisted after treatment. Increased angiogenesis is just one of the numerous subtle alterations in the tumour microenvironment that RFA may produce and contribute to the concentration of nanocarriers in tumours. First, RFA has been demonstrated to enhance vascular permeability because it increases the leukocytes, increasing NO production. Second, the mechanism of RFA promoting vascular permeability activates proinflammatory cytokines (such as interleukins and tumour necrosis factors). Third, the hyperthermia generated by RFA may lose the lymphatic capillaries in the tumour, causing the NCs to remain at the tumour sites longer than they otherwise would [Citation54–56]. Based on these data, we hypothesize that there may be a pathophysiological and natural anatomical for amplifying the EPR effect in persistent malignancy following RFA [Citation56].
We synthesized gemcitabine-loaded ZIF-8 NCs and investigated their therapeutic and biosafety results after seeing an increased EPR effect in tumours that remained after RFA. The AG@ZIF-8 NCs demonstrated excellent biocompatibility (), mainly caused by their good stability in a neutral milieu and biodegradability in the acidic milieu. Then with traditional chemotherapy, the adverse effects in vivo are reduced to the drug’s good stability in the blood’s neutral environment. AG@ZIF-8 NCs may provide less possible safety issues and a higher probability of clinical transformation due to their improved biodegradability and harmless breakdown; AG@ZIF-8 NCs showed significantly improved therapeutic effectiveness in suppressing residual tumour development, inhibiting metastasis, and reversing EMT. Our analysis and findings suggest that these results can be explained as follows. Sublethal heat causes an increase in drug accumulation at locations of persistent tumour growth by both self-augmented EPR and excessive angiogenesis [Citation57]. The microenvironments of solid tumours tend to be acidic. AG@ZIF-8 NCs’ bioavailability and therapeutic efficacy may be improved by their nanocomposite’s diameter and PEG alteration, which may increase their time in circulation. ZIF-8’s ability to modify the drug delivery channel in cells has been shown to have a beneficial influence on chemotherapeutic drugs’ anticancer effect. More specifically, ZIF-8 can speed up the distribution of drugs to the complete cell, as opposed to just the nucleus, and disrupt mitochondrial activity, leading to faster cell death than is shown with free pharmaceuticals. Zinc deficiency has been linked to the development and spread of HCC, and previous research has demonstrated that hepatoma cells have a much lower zinc content than normal liver cells [Citation58]. Degradation of ZIF-8 releases zinc, which may increase cancer cell susceptibility to chemotherapeutic treatments and suppress HCC growth. The AG@ZIF-8 NCs had reduced IC50 rates and reduced migration and invasion of HCC cells compared to the free groups, which may be partly due to these factors.
Several issues were raised by this research that need to be investigated further. The increased EPR effect following RFA is a dynamic pathophysiological process. It is possible that the EPR impact was not immediately apparent in the early post-RFA period when blood flow and vascular density in the remaining malignancy were reduced. When a hypoxic microenvironment develops in the remaining tumour, it promotes angiogenesis uniformly across the tumour, steadily increasing the EPR effect. However, specific blood arteries in tumours rupture due to the rapid expansion of remaining tumours, resulting in an uneven concentration of perfusing blood and the potential for inhomogeneous enrichment of nanodrugs [Citation59]. For this reason, pinpointing the opportune moment to combine nanodrugs with the therapy of residual HCC following RFA is crucial [Citation53]. Second, numerous pharmacological techniques, such as increasing vascular permeability with inflammatory cytokines, normalizing vessels with anti-VEGF(R) drugs, and boosting angiogenesis with angiogenic drugs, can improve the EPR impact. Therefore, combining gemcitabine and vascular mediators in nanocomposites may further enhance the EPR effect. Third, because RFA causes the release of cellular components and cell necrosis, an inflammatory reaction may ensue, and numerous natural adaptive immune cells and immune cells may infiltrate locations with remaining malignancy [Citation60]. AG@ZIF-8 biomimetic NCs may be more successful at suppressing tumour development in its residual stages if coated with cell membranes and directed at the immune microenvironment. Additionally, it is feasible to lessen the number of drugs entering the bloodstream. Finally, drug leakage is a potential issue in the electrostatic adsorption-based synthesis of AG@PEG/ZIF-8; consequently, in situ, the arsenic loading into the porous of the nanoframeworks during the crystal formation might aid in suppressing AG release under neutral circumstances [Citation61].
Conclusion
In conclusion, RFA strongly promotes malignant transformation in lingering HCC tumours. We loaded ZIF-8 NCs with arsenic to stop the spread of the remaining tumours. Our study is the first to show that significant angiogenesis following RFA boosts the EPR effect and increases ZIF-8 NCs concentration in tumour remnants. As a result of these specific accumulations, the AG@ZIF-8 NCs show markedly improved therapeutic effectiveness over gemcitabine concerning suppressing residual tumour development, metastasis, and EMT. Therefore, this study’s results demonstrate evidence of an idea for managing hepatocellular that persists following radiofrequency ablation.
Author contribution statement
Junmei Jia, and Haile Qiu were involved in conceptualization, methodology, investigation and writing the original draft.; Yanyan Liu, Chenan Liu, Xiaoyuan Wei took part in conceptualization, methodology, investigation, and writing-reviewing editing; Dongwen Rong participated in resources and writing-reviewing and editing.
Ethical approval
All the in vivo experiments were implemented according to the criteria of the National Regulation of China for the Care and Use of Laboratory Animals. The Ethical Committee for Research Involving Animal of the First Hospital of Shanxi Medical University, Taiyuan 030001, China, permitted all animal experiments in this investigation.
Disclosure statement
No potential conflict of interest was reported by the author(s).
Data availability statement
Data will be made available on request.
References
- Bosch FX, Ribes J, Díaz M, et al. Primary liver cancer: worldwide incidence and trends. Gastroenterology. 2004;127(5):S5–14. doi: 10.1053/j.gastro.2004.09.011
- Anwanwan D, Singh SK, Singh S, et al. Challenges in liver cancer and possible treatment approaches. Biochimica Et Biophysica Acta (BBA) - Rev can. 2020;1873(1):188314. doi: 10.1016/j.bbcan.2019.188314
- Yamashita T, Wang XW. Cancer stem cells in the development of liver cancer. J Clin Investig. 2013;123(5):1911–1918. doi: 10.1172/JCI66024
- Dhar D, Baglieri J, Kisseleva T, et al. Mechanisms of liver fibrosis and its role in liver cancer. Exp Biol Med. 2020;245(2):96–108. doi: 10.1177/1535370219898141
- Bruix J, Han K-H, Gores G, et al. Liver cancer: approaching a personalized care. J Hepatol. 2015;62(1):S144–S156. doi: 10.1016/j.jhep.2015.02.007
- Kumar CS, Raja MD, Sundar DS, et al. Hyaluronic acid co-functionalized gold nanoparticle complex for the targeted delivery of metformin in the treatment of liver cancer (HepG2 cells). Carbohydr Polym. 2015;128:63–74. doi: 10.1016/j.carbpol.2015.04.010
- Xia Q, Li L, Zhao L. Silica nanoparticle‑based dual‑responsive nanoprodrug system for liver cancer therapy. Exp Ther Med. 2017;14(3):2071–2077. doi: 10.3892/etm.2017.4768
- Ashokan AP, Paulpandi M, Dinesh D, et al. Toxicity on dengue mosquito vectors through myristica fragrans-synthesized zinc oxide nanorods, and their cytotoxic effects on liver cancer cells (HepG2). J Clust Sci. 2017;28(1):205–226. doi: 10.1007/s10876-016-1075-y
- Lee MH, Kim E-J, Lee H, et al. Liposomal texaphyrin theranostics for metastatic liver cancer. J Am Chem Soc. 2016;138:16380–16387. doi: 10.1021/jacs.6b09713
- Liu P, Xu S, Zhang M, et al. Anticancer activity in human multiple myeloma U266 cells: synergy between cryptotanshinone and arsenic trioxide. Metallomics. 2013;5(7):871–878. doi: 10.1039/C3MT20272K
- Wang G, Zhang T, Sun W, et al. Arsenic sulfide induces apoptosis and autophagy through the activation of ROS/JNK and suppression of Akt/mTOR signaling pathways in osteosarcoma. Free Radic Biol Med. 2017;106:24–37. doi: 10.1016/j.freeradbiomed.2017.02.015
- Xin J, Zhang K, Huang J, et al. Facile synthesis of aquo-cisplatin arsenite multidrug nanocomposites for overcoming drug resistance and efficient combination therapy. Biomater Sci. 2019;7(1):262–271. doi: 10.1039/C8BM01039K
- Yuan T, Zhang H, Chen B, et al. Association between lung cancer risk and inorganic arsenic concentration in drinking water: a dose–response meta-analysis. Toxicol Res. 2018;7(6):1257–1266. doi: 10.1039/C8TX00177D
- Adil M, Kandhare AD, Ghosh P, et al. Sodium arsenite-induced myocardial bruise in rats: Ameliorative effect of naringin via TGF-β/Smad and Nrf/HO pathways. Chem Biol Interact. 2016;253:66–77. doi: 10.1016/j.cbi.2016.05.015
- Adil M, Kandhare AD, Visnagri A, et al. Naringin ameliorates sodium arsenite-induced renal and hepatic toxicity in rats: decisive role of KIM-1, Caspase-3, TGF-β, and TNF-α. Ren Fail. 2015;37(8):1396–1407. doi: 10.3109/0886022X.2015.1074462
- Konstantinopoulos PA, Cheng S-C, Wahner Hendrickson AE, et al. Berzosertib plus gemcitabine versus gemcitabine alone in platinum-resistant high-grade serous ovarian cancer: a multicentre, open-label, randomised, phase 2 trial. Lancet Oncol. 2020;21(7):957–968. doi: 10.1016/S1470-2045(20)30180-7
- Awasthi N, Zhang C, Ruan W, et al. BMS-754807, a small-molecule inhibitor of insulin-like growth factor-1 receptor/insulin receptor, enhances gemcitabine response in pancreatic cancer. Mol Cancer Ther. 2012;11(12):2644–2653. doi: 10.1158/1535-7163.MCT-12-0447
- Soni N, Soni N, Pandey H, et al. Augmented delivery of gemcitabine in lung cancer cells exploring mannose anchored solid lipid nanoparticles. J Colloid Interface Sci. 2016;481:107–116. doi: 10.1016/j.jcis.2016.07.020
- Habib S, Singh M. Recent advances in lipid-based nanosystems for gemcitabine and gemcitabine–combination therapy. Nanomaterials. 2021;11(3):597. doi: 10.3390/nano11030597
- Zhang Y, Kim WY, Huang L. Systemic delivery of gemcitabine triphosphate via LCP nanoparticles for NSCLC and pancreatic cancer therapy. Biomaterials. 2013;34(13):3447–3458. doi: 10.1016/j.biomaterials.2013.01.063
- Thompson BR, Shi J, Zhu H-J, et al. Pharmacokinetics of gemcitabine and its amino acid ester prodrug following intravenous and oral administrations in mice. Biochem Pharmacol. 2020;180:114127. doi: 10.1016/j.bcp.2020.114127
- Heinemann V. Gemcitabine: progress in the treatment of pancreatic cancer. Oncology. 2001;60(1):8–18. doi: 10.1159/000055290
- Xie H, Liu X, Huang Z, et al. Nanoscale zeolitic imidazolate framework (ZIF)–8 in cancer theranostics: current challenges and prospects. Current Challen Prosp Can. 2022;14(16):3935. doi: 10.3390/cancers14163935
- Abdelhamid HN. Zeolitic imidazolate frameworks (ZIF-8) for biomedical applications: a review. Curr Med Chem. 2021;28(34):7023–7075. doi: 10.2174/0929867328666210608143703
- Wang Q, Sun Y, Li S, et al. Synthesis and modification of ZIF-8 and its application in drug delivery and tumor therapy. RSC Adv. 2020;10(62):37600–37620. doi: 10.1039/D0RA07950B
- Vasconcelos IB, da Silva TG, Militão GCG, et al. Cytotoxicity and slow release of the anti-cancer drug doxorubicin from ZIF-8. RSC Adv. 2012;2(25):9437–9442. doi: 10.1039/c2ra21087h
- Bi J, Lu Y, Dong Y, et al. Synthesis of folic acid-modified DOX@ ZIF-8 nanoparticles for targeted therapy of liver cancer. J Nanomater. 2018. 1357812. doi: 10.1155/2018/1357812
- Sandomierski M, Jakubowski M, Ratajczak M, et al. Zeolitic Imidazolate Framework‑8 (ZIF-8) modified titanium alloy for controlled release of drugs for osteoporosis. Sci Rep. 2022;12:9103. doi: 10.1038/s41598-022-13187-0
- Zhang H, Li Q, Liu R, et al. A versatile prodrug strategy to in situ encapsulate drugs in MOF nanocarriers: a case of cytarabine-IR820 prodrug encapsulated ZIF-8 toward chemo-photothermal therapy. Adv Funct Mater. 2018;28(35):1802830. doi: 10.1002/adfm.201802830
- Qin Y-T, Peng H, He X-W, et al. Ph-responsive polymer-stabilized ZIF-8 nanocomposites for fluorescence and magnetic resonance dual-modal imaging-guided chemo-/photodynamic combinational cancer therapy. ACS Appl Mater Inter. 2019;11(37):34268–34281. doi: 10.1021/acsami.9b12641
- Prabhu R, Anjali R, Archunan G, et al. Ecofriendly one pot fabrication of methyl gallate@ ZIF-L nanoscale hybrid as pH responsive drug delivery system for lung cancer therapy. Process Biochem. 2019;84:39–52. doi: 10.1016/j.procbio.2019.06.015
- Fu X, Zhang G, Zhang Y, et al. Co-delivery of anticancer drugs and cell penetrating peptides for improved cancer therapy. Chin Chem Lett. 2021;32(4):1559–1562. doi: 10.1016/j.cclet.2020.10.011
- Deng Q, Chen S, Fu C, et al. Long noncoding RNA expression profiles in sub-lethal heat-treated hepatoma carcinoma cells. World J Surg Oncol. 2017;15(1):1–14. doi: 10.1186/s12957-017-1194-4
- Zhang T, Wei Q, Zhou H, et al. Sustainable release of vancomycin from micro-arc oxidised 3D-printed porous Ti6Al4V for treating methicillin-resistant Staphylococcus aureus bone infection and enhancing osteogenesis in a rabbit tibia osteomyelitis model. Biomater Sci. 2020;8(11):3106–3115. doi: 10.1039/C9BM01968E
- Mohamed Kasim MS, Sundar S, Rengan R. Synthesis and structure of new binuclear ruthenium(II) arene benzil bis(benzoylhydrazone) complexes: investigation on antiproliferative activity and apoptosis induction. Inorg Chem Front. 2018;5(3):585–596. doi: 10.1039/c7qi00761b
- Mohamed Subarkhan MK, Ramesh R, Liu Y. Synthesis and molecular structure of arene ruthenium(II) benzhydrazone complexes: Impact of substitution at the chelating ligand and arene moiety on antiproliferative activity. New J Chem. 2016;40(11):9813–9823. doi: 10.1039/c6nj01936f
- Mohan N, Mohamed Subarkhan MK, Ramesh R. Synthesis, antiproliferative activity and apoptosis-promoting effects of arene ruthenium(II) complexes with N, O chelating ligands. J Organomet Chem. 2018;859:124–131. doi: 10.1016/j.jorganchem.2018.01.022
- Swaminathan S, Haribabu J, Mohamed Subarkhan MK, et al. Coordination behavior of acylthiourea ligands in their Ru(II)–benzene complexes─Structures and anticancer activity. Organometallics. 2022;41(13):1621–1630. doi: 10.1021/acs.organomet.2c00127
- Balaji S, Mohamed Subarkhan MK, Ramesh R, et al. Synthesis and structure of arene Ru(II) N∧O-chelating complexes: in Vitro cytotoxicity and cancer cell death mechanism. Organometallics. 2020;39(8):1366–1375. doi: 10.1021/acs.organomet.0c00092
- Swaminathan S, Haribabu J, Mohamed Subarkhan MK, et al. Impact of aliphatic acyl and aromatic thioamide substituents on the anticancer activity of Ru(ii)-p-cymene complexes with acylthiourea ligands—in vitro and in vivo studies. Dalton Trans. 2021;50(44):16311–16325. doi: 10.1039/D1DT02611A
- Wang Y, Jin J, Shu L, et al. New organometallic ruthenium(ii) compounds synergistically show cytotoxic, antimetastatic and antiangiogenic activities for the treatment of metastatic cancer. Chem Eur J. 2020;26(66):15170–15182. doi: 10.1002/chem.202002970
- Pilliadugula R, Haribabu J, Mohamed Subarkhan MK, et al. Effect of morphology and (Sn, Cr) doping on in vitro antiproliferation properties of hydrothermally synthesized 1D GaOOH nanostructures. J Sci. 2021;6(3):351–363. doi: 10.1016/j.jsamd.2021.03.003
- Giriraj K, Mohamed Kasim MS, Balasubramaniam K, et al. Various coordination modes of new coumarin Schiff bases toward Cobalt (III) ion: Synthesis, spectral characterization, in vitro cytotoxic activity, and investigation of apoptosis. Appl Organomet Chem. 2022;36(3):e6536. doi: 10.1002/aoc.6536
- Sathiya Kamatchi T, Mohamed Subarkhan MK, Ramesh R, et al. Investigation into antiproliferative activity and apoptosis mechanism of new arene Ru(ii) carbazole-based hydrazone complexes. Dalton Trans. 2020;49(32):11385–11395. doi: 10.1039/D0DT01476A
- Mohamed Subarkhan MK, Ren L, Xie B, et al. Novel tetranuclear ruthenium(II) arene complexes showing potent cytotoxic and antimetastatic activity as well as low toxicity in vivo. Eur J Med Chem. 2019;179:246–256. doi: 10.1016/j.ejmech.2019.06.061
- Subarkhan MKM, Ramesh R. Ruthenium(ii) arene complexes containing benzhydrazone ligands: synthesis, structure and antiproliferative activity. Inorg Chem Front. 2016;3(10):1245–1255. doi: 10.1039/C6QI00197A
- Zhong Z, Huang Y, Hu Q, et al. Elucidation of molecular pathways responsible for the accelerated wound healing induced by a novel fibrous chitin dressing. Biomater Sci. 2019;7(12):5247–5257. doi: 10.1039/C9BM00404A
- Rangamuwa K, Leong T, Weeden C, et al. Thermal ablation in non-small cell lung cancer: a review of treatment modalities and the evidence for combination with immune checkpoint inhibitors. Transl Lung Cancer Res. 2021;10(6):2842–2857. doi: 10.21037/tlcr-20-1075
- Palussière J, Chomy F, Savina M, et al. Radiofrequency ablation of stage IA non–small cell lung cancer in patients ineligible for surgery: results of a prospective multicenter phase II trial. J Cardiothorac Surg. 2018 91;13(1). doi: 10.1186/s13019-018-0773-y
- Löffler MW, Nussbaum B, Jäger G, et al. A non-interventional clinical trial assessing immune responses after radiofrequency ablation of liver metastases from colorectal cancer. Front Immunol. 2019;10:2526. https://www.frontiersin.org/article/10.3389/fimmu.2019.02526
- Xu M, Hu Y, Ding W, et al. Rationally designed rapamycin-encapsulated ZIF-8 nanosystem for overcoming chemotherapy resistance. Biomaterials. 2020;258:120308. doi: 10.1016/j.biomaterials.2020.120308
- Ojha T, Pathak V, Shi Y, et al. Pharmacological and physical vessel modulation strategies to improve EPR-mediated drug targeting to tumors. Adv Drug Delivery Rev. 2017;119:44–60. doi: 10.1016/j.addr.2017.07.007
- Fang J, Islam W, Maeda H. Exploiting the dynamics of the EPR effect and strategies to improve the therapeutic effects of nanomedicines by using EPR effect enhancers. Adv Drug Delivery Rev. 2020;157:142–160. doi: 10.1016/j.addr.2020.06.005
- Nijkamp MW, Van Der Bilt JDW, De Bruijn MT, et al. Accelerated perinecrotic outgrowth of colorectal liver metastases following radiofrequency ablation is a hypoxia-driven phenomenon. Ann Surg. 2009;249(5):814–823. doi: 10.1097/SLA.0b013e3181a38ef5
- Dongre A, Weinberg RA. New insights into the mechanisms of epithelial–mesenchymal transition and implications for cancer. Nat Rev Mol Cell Biol. 2019;20(2):69–84. doi: 10.1038/s41580-018-0080-4
- Hosui A, Kimura E, Abe S, et al. Long-term zinc supplementation improves liver function and decreases the risk of developing hepatocellular carcinoma. Nutrients. 2018;10(12):1955. doi: 10.3390/nu10121955
- Xue Y, Yu B, Liu Y, et al. Zinc promotes prostate cancer cell chemosensitivity to paclitaxel by inhibiting epithelial‐mesenchymal transition and inducing apoptosis. Prostate. 2019;79(6):647–656. doi: 10.1002/pros.23772
- Zheng H, Zhang Y, Liu L, et al. One-pot synthesis of metal–organic frameworks with encapsulated target molecules and their applications for controlled drug delivery. J Am Chem Soc. 2016;138(3):962–968. doi: 10.1021/jacs.5b11720
- Dumolard L, Ghelfi J, Roth G, et al. Percutaneous ablation-induced immunomodulation in hepatocellular carcinoma. Int J Mol Sci. 2020;21(12):4398. doi: 10.3390/ijms21124398
- Franklin RB, Levy BA, Zou J, et al. ZIP14 zinc transporter downregulation and zinc depletion in the development and progression of hepatocellular cancer. J Gastrointest Cancer. 2012;43(2):249–257. doi: 10.1007/s12029-011-9269-x
- Chu KF, Dupuy DE. Thermal ablation of tumours: biological mechanisms and advances in therapy. Nat Rev Cancer. 2014;19914(3):199–208. doi: 10.1038/nrc3672