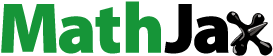
ABSTRACT
Ultra-fine Cu powders were synthesized using supercritical water as a reaction medium and formic acid as a reducing agent. The effects of reducing agent, reaction temperature, and complexing agent on the phase constituents, particle size distributions and morphology of the synthesized ultra-fine Cu powders were investigated. A complex mixture of Cu2O and Cu powders formed by pure supercritical water while high purity homogeneous ultra-fine Cu powders can be synthesized by supercritical water with formic acid as a reducing agent. Moreover, fine tuning of particle size and morphology can be realized by the addition of Ethylenediamine tetraacetic acid (EDTA). The modification mechanism of EDTA on the surfaces of ultra-fine Cu powders was investigated by X-ray photoelectron spectroscopy and Fourier transform infrared spectroscopy. EDTA adsorbed on the surfaces of nanoparticles by the dehydration reaction of carboxyl groups in the molecule of EDTA and hydroxyl groups on surfaces of copper nanoparticles (CuOH).
Introduction
Owing to their unique physical and chemical properties compared with those of bulk metals, ultra-fine/nano metal powders with particle sizes between 1 nm and 10 μm are widely used as raw material of additive manufacturing, powder metallurgy, printed electronics, chemical, biomedical, and electromagnetic shielding [Citation1–3]. Among them, ultra-fine/nano copper (Cu) powders draw great interest from both the domain of science and industrial fields owing to their novel electronic, optical, magnetic and catalytic, antimicrobial properties and low cost [Citation4–7]. Therefore, the synthesis of ultra-fine/nano Cu powders has become a key area of chemical engineering. To date, sorts of synthetic techniques including mechanical grinding, electrochemical, chemical vapour deposition, chemical reduction, thermal reduction and electrochemical deposition have been explored and developed for the synthesis of ultra-fine/nano Cu powders [Citation8–10]. However, some drawbacks of these approaches, such as, usage of relatively noxious chemicals and expensive precursors, a relatively longer period of reaction time, complex process, high energy consumption, production of chemical wastes, and low production rate limit the development of these methods. Thus, it is important to search for a relatively simple, inexpensive, and environmentally friendly method for synthesis of ultra-fine/nano Cu powders.
Supercritical water hydrothermal synthesis technique (Tc = 374.2°C, Pc = 22.1 MPa) recently proposed by Adschiri and Arai was identified as a simple and environmentally friendly method for materials synthesis [Citation11–13]. This new synthesis technology takes the advantage of the special properties of supercritical water including low viscosity, high diffusivity, negligible surface tension and has the advantages of low-cost, low energy consumption, high efficiency, simple operation and pollution free. The supercritical water can offer great synthetic flexibility due to its tunable physical and chemical properties with temperature and pressure. In addition, the utilisation of water as a solvent in this method is not only cost-effective but also highly eco-friendly and non-toxic. Currently, this technology has been widely used for the synthesis of metal oxide nanoparticles, such as CeO2 [Citation14], γ-Al2O3 [Citation15], TiO2 [Citation16], CuO [Citation17] and ZnO [Citation18]. However, research on the use of supercritical water for the synthesis of ultra-fine/nano metal powders remains limited. Recently, Chang et al. [Citation19]. reported the formation of silver (Ag) nanoparticles, nanowires, and nanobanner from Ag2O in supercritical water. Seong et al. successfully synthesised highly crystalline cobalt nanoparticles with low surface oxidation via supercritical hydrothermal process in the temperature range of 340 ~ 420°C [Citation20].
Therefore, this study aims to achieve the one-step synthesis of ultra-fine Cu powders by supercritical water hydrothermal synthesis technique and explore the preparation conditions (including reducing agent type, reaction temperature and complexing agent and so on) to realise size-controllable synthesis. Morphologies, sizes and phase constituents of the synthesised particles were analysed by scanning electron microscopy (SEM) and X-ray diffraction (XRD). Ethylenediamine tetraacetic acid (EDTA) was effective in suppressing the agglomeration and growth of the ultra-fine Cu powders in the supercritical hydrothermal synthesis process. The modification mechanism of EDTA on the surfaces of Cu powders was investigated by X-ray photoelectron spectroscopy and Fourier transform infrared spectroscopy.
Materials and methods
Materials
Copper sulphate precursor solution (Concentration of 0.1 M) was prepared by dissolving the analytical grade copper sulphate (CuSO4·5 H2O, purity >99.9 wt%) in deionized water (10 ml). Formic acid (approx. 99%) was adopted as the reducing agent. First, the copper sulphate solution and an appropriate amount of formic acid (Concentration of 1 M) were transferred into a batch type reactor (Inner volume about 10 ml) made from Hastelloy C alloy. Subsequently, various concentrations of Ethylenediamine tetraacetic acid disodium salt (EDTA, 0.05–1.00 g) was added to prevent the synthesized Cu particles agglomeration and control their growth. Then, the reactor was then sealed tightly and heated to 400 °C ~ 450°C for 5 min in an electric furnace. According to the total volume of loaded reactants at reaction temperature, the inner pressure reaction media was 25 MPa during residence time. After the reaction, the batch reactors were quickly cooled by quenching in cold water to terminate the reaction. Subsequently, the suspension was withdrawn from the reactor and centrifuged to separate the reaction products. Finally, the obtained powders were dispersed into methanol, freeze dried and stored in the glove box.
Characterization of ultra-fine/nano Cu powders
The size and morphology of the obtained metal powders, and their elemental makeup, were examined by SEM (Philips XL30, 20 kV) equipped with an energy-dispersive X-ray spectroscopy (EDS). The crystallographic phase of the obtained metal powders was evaluated by powder XRD (Rigaku Co., Japan) with Cu Kα (λ = 0.154 nm) over a range of 2θ angles from 20 to 100°. Infrared (FT-IR) spectra of organic functional groups on the surface of copper products were examined using a Fourier Transform Infrared Spectrometer (Vertex70) in the wave number range of 4000 ~ 400 cm-1. The elements on the surface of copper powders were analysed using x-ray photoelectron spectroscopy (XPS) (Thermo ESCALAB 250XI).
Results and discussion
Attempts at synthesis and the effect of reducing agent
shows the typical XRD patterns of obtained products using supercritical water with or without formic acid solutions at 25 MPa, and 400°C for 10 min. For the pure supercritical water without the formic acid, the XRD patterns exhibit Bragg reflections that correspond to the crystal structure of face centred cubic Cu and Cu2O respectively, indicating that a mixture of Cu and Cu2O was obtained. This indicates that the reducing power of the supercritical water at 400 °C/25 MPa was not enough to convert copper sulphate into zero valent Cu phase. In contrast, by the addition of a 0.1 mol/L formic acid aqueous solution, three peaks associated with highly crystalline single Cu phase at 2θ = 43.34°, 50.47°, 74.20° were identified. No peaks of Cu oxide or Copper hydroxide were detected, indicating the product with high purity.
Figure 1. XRD profiles of as-synthesized powders using supercritical water without or with formic acid solutions.
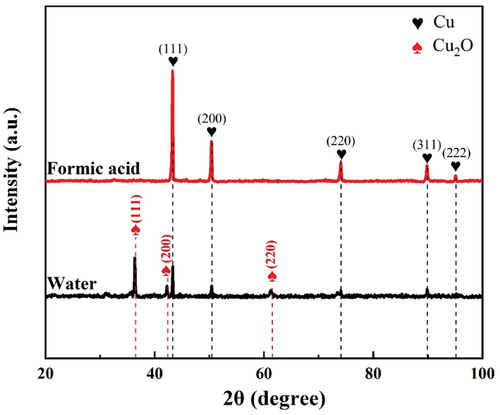
According to the facts, it can be said that a mixture of Cu and Cu2O was synthesised in pure supercritical water while pure metallic copper particles were synthesised in supercritical water with HCOOH as a reducing agent. This result indicates that introducing HCOOH results in a strong reducing atmosphere in supercritical water, which promotes the formation and precipitation of zero-valent Cu phase. The reducibility of HCOOH in supercritical conditions is attributed to the decomposition products. Yu and Savage [Citation21] have reported that the formic acid tend to decompose completely into H2 and CO2 in hydrothermal environments at reaction temperatures above 320°C. Moreover, under these reaction conditions, H2 is maximally soluble in supercritical water, thereby forming a strong homogeneous reducing atmosphere in the reactor, causing the reduction of Cu ions or Cu oxide into zero valent Cu phase.
SEM observations coupled with EDS analyses were performed to investigate the morphology, size distribution, and elemental composition of the synthesized complete metallic Cu particles. As shown in , Cu2O particles synthesised in pure supercritical water have square-like or truncated square-like shapes. Differently, shows that pure metallic copper particles synthesised in supercritical water with formic acid have spherical shape. In addition, reveals the aggregated and agglomerated copper powders with several micrometres in size. However, the figure clearly shows that the agglomeration consists of smaller nanoparticles, each approximately hundreds of nanometres in size. It is well know that the nanoparticles tend to aggregate and agglomerate due to their high surface energy. reveals the EDS results for the sample shown in . The dominant peak is copper, but a weaker oxygen peak is also visible. The presence of the oxygen peak suggests the possible existence of an oxide layer, which can form a few nanometres thick on the particle surface. This oxide layer is formed after exposure to air following the reaction, which is referred to as secondary oxidation.
Effect of complexing/dispersing agents
PVP-K30 and EDTA-2Na are commonly used as complexing/dispersing agents for stabilisation of the metal nanoparticles dispersions against the process of aggregation. We chose PVP-K30 and EDTA-2Na to study their effects on the size and morphology of the ultra-fine Cu powders synthesised in supercritical water with formic acid solutions. SEM images of the as-synthesised powders using supercritical water without dispersant and with the addition of different types of dispersants are shown in . The addition of dispersant has a very significant effect on the particle size and morphology of the copper powders. Without any dispersant, the copper powders tend to aggregate and agglomerate with each other with no obvious boundary, showing a ‘chunky’ feeling, as shown in . shows the SEM image of the as-synthesised powders after the addition of PVP-K30. Compared with the as-synthesised powders without the addition of dispersant, although the addition of PVP-K30 improved the dispersion of copper powders, the surface of the copper powders was still rough, and the boundary between the copper powders was still blurred, presenting the state of local stacking and agglomeration. shows the SEM image of the as-synthesised Cu powders after the addition of EDTA-2Na. Compared with the previous two cases, it is evident in that the surface of the product particles is smooth and flat, and there is a clear and sharp boundary between the copper powders. According to the above results, EDTA-2Na exhibits a superior stabilisation of the ultra-fine copper powders dispersions against the process of aggregation than the PVP-K30. Therefore, EDTA-2Na was selected as the complexing/dispersing agent for synthesis of ultra-fine Cu powders in supercritical with formic acid as a reducing agent.
Figure 3. SEM images of as-synthesized powders using supercritical water (a) without dispersant and with addition of different dispersants (b) 0.1g PVP-K30 (c) 0.1g EDTA-2Na.
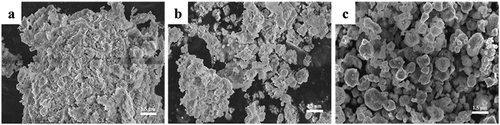
displays the SEM images of the products with varying EDTA concentrations. It can be seen clearly that the addition of EDTA significantly affects the size distribution and morphology of Cu powders. It is evident that the EDTA effectively prevents the agglomeration and growth of copper nanoparticles. Moreover, the uniformity of the ultra-fine/nano copper particles showed a significant improvement with the addition of EDTA. The mean particle size at molar ratios of EDTA to copper ion of 0.5:1, 1:1, 2:1, and 4:1 were 890.2305.5 nm, 793.9
189.6 nm, 437
130.2 nm, and 341
63.9 nm, respectively. With an increase in EDTA/copper ion molar ratio, the average particle size decreased and the uniformity of particle size increased. This result suggests that higher concentrations of EDTA result in higher degree of homogeneity and dispersity of the ultra/nano copper particles. This is mainly because increasing the level of EDTA can supply sufficient atoms to coordinate with Cu ions, forming strong chelating compounds during the supercritical hydrothermal synthesis process. This could have prevented early nucleation of copper nanoparticles. Based on the above results, metallic ultra-fine Cu powders with uniform particle size can be formed through a supercritical hydrothermal reduction process (400°C, 25 MPa and 10 min) using formic acid as the reducer and EDTA as a complexing agent.
The modification mechanism of EDTA on the surfaces of ultra-fine Cu powders
In order to study the modification mechanism of EDTA on the surfaces of ultra-fine Cu powders, Fourier Transform Infrared (FT-IR) analyses were conducted on the pure EDTA and copper products with a molar ratio of EDTA to Cu2+ of 4:1, as shown in . shows the FT-IR spectrum of pure EDTA, where -OH stretching causes the spectrum to absorb at 3700–3300 cm−1 and the peaks appearing at 3050–2950 cm−1 are due to -CH2- stretching vibrations, a strong absorption band at 1750–1350 cm−1 due to the stretching of the carboxyl group (−COOH), and the weak absorption spectra at wave numbers less than 1350 cm−1 (fingerprint region) are very complex and may be due to the stretching vibrations of C-N and C-OH stretching vibrations. shows the FTIR spectrum of the copper product at a molar ratio of EDTA to Cu2+ of 4:1. Compared with pure EDTA, the spectrum of this copper product shows a very similar absorption band structure, only with a slightly weaker absorption intensity, which suggests that there is indeed an attachment of EDTA on its surface. In addition, the weakening or even loss of some of the subtle structures in the fingerprint region can be seen, which may indicate that some of the EDTA has formed a complex with the Cu on the surface of the copper product, which is also the reason why EDTA can reduce the particle size of the copper product.
Figure 5. FT-IR spectra of pure EDTA and copper products with [EDTA]/[Cu2+]=4:1. (a) pure EDTA, (b) copper products with EDTA/Cu2+=4:1.
![Figure 5. FT-IR spectra of pure EDTA and copper products with [EDTA]/[Cu2+]=4:1. (a) pure EDTA, (b) copper products with EDTA/Cu2+=4:1.](/cms/asset/1195b498-6f19-46e5-8171-9f4f7f45d39d/ymte_a_2377478_f0005_oc.jpg)
XPS characterisation of copper particles was carried out to analyse the chemical bonding composition of the surface of the copper products. As shown in , peaks corresponding to C 1s, O 1s, Cu 2p3/2, and Cu 2p1/2 with peaks of 284.76 eV, 530.83 eV, 932.37 eV, and 951.96 eV, respectively, were detected in the XPS measurement spectra. The spectrum of C 1s is shown in , and three peaks with peaks of 284.80 eV, 285.82 eV and 288.48 eV were obtained after the operation of splitting and fitting the spectrum; the appearance of these peaks may be related to C-C, C-N and O-C=O in EDTA, respectively, proving that the surface of the Cu particles is covered by EDTA. The peak of O 1s was also split into two peaks, as shown in , with peaks of 530.72 eV (Cu-O) and 531.85 eV (O-C=O), where the presence of Cu-O also indicates its possible formation due to further complexation of EDTA with Cu atoms on the surface of the particles, suggesting that EDTA is attached to the surface of Cu particles in the form of copper complexes. shows the spectrum of Cu 2p showing the peaks of elemental copper (932.57 eV and 952.32 eV).
Effect of reaction temperature
Reaction temperature is one of the important factors affecting the particle size and morphology of copper nanoparticles. Combining the previous results, this experiment was carried out by changing the temperature only, under the conditions where other factors were optimal. The reaction temperatures were set at 420°C, 440°C and 460°C. The product particle sizes of the experimental groups with different reaction temperatures are shown in . As can be seen in , when the reaction temperature was 420°C, the particle size of the product was 382.2 ± 107.0 nm, which was coarser and the particle homogeneity was reduced as compared to that at a temperature of 400°C. Also multiple particles can be seen sticking to each other. At 440°C, the average particle size of the particles increased, as shown in . The particles in the local area are interconnected to form coarser particles, and there are also particles that have not fused and grown up for the time being, at this time, the particle size distribution of the particles varies greatly, and the uniformity is further reduced. When the temperature is increased to 460°C, the smaller particles continue to grow, the uniformity increases, and the overall particle size is even coarser, 496 ± 179.9 nm. This may be due to the fact that the entropy value in the system increases as the temperature rises, which exacerbates the collision of copper particles in the supercritical environment, and the particles collide and fuse with each other to form particles with a larger particle size. Therefore, in supercritical synthesis of copper powder, the particle size of copper particles grows with the increase of temperature.
Conclusions
Supercritical hydrothermal synthesis of ultra-fine copper powders were studied in this work. The effects of reducing agent, reaction temperature, and complexing agent on phase constituents, the particle size distributions and morphology of the synthesised particles were investigated. Supercritical water with formic acid as a reducing agent can synthesise pure ultra-fine copper powders, whereas pure supercritical water can only synthesise a mixture of Cu2O and Cu. The reaction power of formic acid was due to the H2 generated from the decomposition of formic acid and dissolution of free H2 as a reducing agent in the supercritical water. The addition of complexing agent EDTA is a key technique that can be used to effectively prevent agglomeration and growth of the ultra/nano copper particles in supercritical hydrothermal synthesis processes. The FT-IR and XPS results showed that EDTA adsorbed on the surfaces of ultra-fine copper powders by dehydration reaction of carboxyl group (COOH) in the molecule of EDTA and hydroxyl group on the copper surface (CuOH).
Credit authorship contribution statement
Xin He: Data curation, Writing-original draft. Shaoyu Li: Investigation, Formal analysis. Longfei Zeng: Conceptualisation, Methodology, Writing-review & editing, Formal analysis, Funding acquisition, Supervision. Pingan Jiang: Formal analysis, Investigation. Xuehui Zhang: Validation, Writing-review & editing.
Disclosure statement
No potential conflict of interest was reported by the author(s).
Data availability statement
The data that support the findings of this study are available upon reasonable request from the authors.
Additional information
Funding
References
- Reddy GB, Ramakrishna D, Madhusudhan A, et al. Catalytic reduction of p-nitrophenol and hexacyanoferrate (III) by borohydride using green synthesized gold nanoparticles. J Chin Chem Soc. 2015;62(5):420–7. doi: 10.1002/jccs.201400513
- Rao CNR, Cheetham AK. Science and technology of nanomaterials: current status and future prospects. J Mater Chem. 2001;11(12):2887–2894. doi: 10.1039/b105058n
- Lu X, Rycenga M, Skrabalak SE, et al. Chemical synthesis of novel plasmonic nanoparticles. Annu Rev Phys Chem. 2009;60:167–192. doi: 10.1146/annurev.physchem.040808.090434
- Cha SI, Mo CB, Kim KT, et al. Mechanism for controlling the shape of Cu nanocrystals prepared by the polyol process. J Mater Res. 2006;21(9):2371–2378. doi: 10.1557/jmr.2006.0285
- Sarkar A, Mukherjee T, Kapoor S. PVP-stabilized copper nanoparticles: a reusable catalyst for “click” reaction between terminal alkynes and azides in nonaqueous solvents. J Phys Chem C. 2008;112(9):3334–3340. doi: 10.1021/jp077603i
- Sun J, Jing Y, Jia Y, et al. Mechanism of preparing ultrafine copper powder by polyol process. Mater Lett. 2005;59(29–30):3933–3936. doi: 10.1016/j.matlet.2005.07.036
- Tanabe K. Optical radiation efficiencies of metal nanoparticles for optoelectronic applications. Mater Lett. 2007;61(23–24):4573–4575. doi: 10.1016/j.matlet.2007.02.053
- Park BK, Jeong S, Kim D, et al. Synthesis and size control of monodisperse copper nanoparticles by polyol method. J Colloid Interface Sci. 2007;311(2):417–424. doi: 10.1016/j.jcis.2007.03.039
- Hatakeyama Y, Morita T, Takahashi S, et al. Synthesis of gold nanoparticles in liquid polyethylene glycol by sputter deposition and temperature effects on their size and shape. J Phys Chem C. 2011;115(8):3279–3285. doi: 10.1021/jp110455k
- Kalyanaraman R, Yoo S, Krupashankara MS, et al. Synthesis and consolidation of iron nanopowders. Nanostruct Mater. 1998;10(8):1379–1392. doi: 10.1016/S0965-9773(99)00017-3
- Adschiri T, Kanazawa K, Arai K. Rapid and continuous hydrothermal synthesis of boehmite particles in subcritical and supercritical water. J Am Ceram Soc. 1992;75(9):2615–2618. doi: 10.1111/j.1151-2916.1992.tb05625.x
- Adschiri T, Kanazawa K, Arai K. Rapid and continuous hydrothermal crystallization of metal oxide particles in supercritical water. J Am Ceram Soc. 1992;75(4):1019–1022. doi: 10.1111/j.1151-2916.1992.tb04179.x
- Adschiri T, Arai K. Hydrothermal synthesis of metal oxide nanoparticles under supercritical conditions. Supercritical Fluid Technology in Materials Science and Engineering. 2002. p. 311–325.
- Middelkoop V, Tighe CJ, Kellici S, et al. Imaging the continuous hydrothermal flow synthesis of nanoparticulate CeO2 at different supercritical water temperatures using in situ angle-dispersive diffraction. J Supercrit Fluids. 2014;87:118–128. doi: 10.1016/j.supflu.2013.12.022
- Noguchi T, Matsui K, Islam NM, et al. Rapid synthesis of γ-Al2O3 nanoparticles in supercritical water by continuous hydrothermal flow reaction system. J Supercrit Fluids. 2008;46(2):129–136. doi: 10.1016/j.supflu.2008.04.011
- Mi JL, Johnsen S, Clausen C, et al. Highly controlled crystallite size and crystallinity of pure and iron-doped anatase-TiO2 nanocrystals by continuous flow supercritical synthesis. J Mater Res. 2013;28(3):333–339. doi: 10.1557/jmr.2012.234
- Sun P, Wang S, Zhang T, et al. Supercritical hydrothermal synthesis of submicrometer copper (ii) oxide: effect of reaction conditions. Ind & Eng Chem Res. 2017;56(21):6286–6294. doi: 10.1021/acs.iecr.7b00777
- Liu W, Wang S, Wang J, et al. Supercritical hydrothermal synthesis of nano-zinc oxide: process and mechanism. Ceram Int. 2022;48(16):22629–22646. doi: 10.1016/j.ceramint.2022.05.094
- Kim M, Son WS, Ahn KH, et al. Hydrothermal synthesis of metal nanoparticles using glycerol as a reducing agent. J Supercrit Fluids. 2014;90:53–59. doi: 10.1016/j.supflu.2014.02.022
- Seong G, Takami S, Arita T, et al. Supercritical hydrothermal synthesis of metallic cobalt nanoparticles and its thermodynamic analysis. J Supercrit Fluids. 2011;60:113–120. doi: 10.1016/j.supflu.2011.05.003
- Yu J, Savage PE. Decomposition of formic acid under hydrothermal conditions. Ind & Eng Chem Res. 1998;37(1):2–10. doi: 10.1021/ie970182e