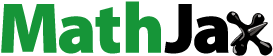
Abstract
The relationship between radical-scavenging rate constants (k) in an aprotic medium and thermodynamic parameters calculated by density functional theory (DFT) was investigated for 7 flavonols, which are myricetin (Myr), quercetin (Que), morin (Mor), kaempferol (Kae), 2′-methylquercetin (2′-MeQue), 5′-methylquercetin (5′-MeQue), and 2′,5′-dimethylquercetin (Me2Que). The k values were determined for the reaction between the flavonols and galvinoxyl radical used as a reactivity model of reactive oxygen species in deaerated acetonitrile at 298 K. The energy difference values (DHT, HT: hydrogen transfer) between the flavonols and the corresponding radicals, which equal to the relative O–H bond dissociation energies of the OH groups in the flavonols and ionisation potentials (IP) were calculated by DFT at the B3LYP/6-31++G(d) level with C-PCM solvation model parameterised for acetonitrile. Among the 7 flavonols used in this study, calculated IP values of 4 flavonols exhibited a linear correlation with log k, suggesting that the radical-scavenging reaction of these flavonols may proceed via an electron transfer as the rate determining step.
Introduction
Flavonols, such as quercetin (Que), myricetin (Myr), morin (Mor), and kaempferol (Kae), are known to show significant multiple beneficial effects on human health, such as antioxidative, antiestrogenic, anticancer, anti-inflammatory, cardioprotective effects, and so on [Citation1,Citation2]. Thus, flavonols are expected to be used as cosmetics, pharmaceuticals, and food supplements [Citation2]. It is also known that flavonols have one or more hydroxy (OH) groups in the molecule and show an efficient scavenging activity against reactive oxygen species (ROS) and related radicals. However, the detailed radical-scavenging mechanism of flavonols, for example, which OH group is responsible for the radical-scavenging activity, has yet to be fully clarified. In alcohols or alcoholic aqueous solutions, the scavenging reactions of 2,2-diphenyl-1-picrylhydrazyl radical (DPPH•) by Que were reported to proceed via sequential proton-loss electron transfer (SPLET) mechanism, in which a deprotonation of the phenolic OH group in Que is followed by a fast electron transfer from the corresponding phenolate anion of Que to DPPH• [Citation3,Citation4]. It is also reported that the disproportionation of the phenoxyl radical generated from Que in the reaction with radicals plays an important role to the activity of Que [Citation5]. On the other hand, it is known that there are two possible mechanisms for the initial step of radical-scavenging reactions of phenolic antioxidants [Citation6–13]. The first one is a one-step hydrogen-atom transfer from the phenolic OH group to radicals. The second mechanism is an electron transfer from phenolic antioxidants to radicals followed by proton transfer. It is of considerable importance to clarify the structure–activity and structure–mechanism relationships to develop novel synthetic antioxidants with stronger activity than naturally occurring ones. Recently, we have reported the synthesis and radical-scavenging activity of methylated quercetin derivatives [Citation14]. The methyl groups on the C-2′ and/or C-5′ positions in the B ring () significantly enhanced the radical-scavenging activity of Que. However, little is known about the radical-scavenging mechanism of these methylated quercetin derivatives. Density functional theory (DFT) calculations are powerful tool to estimate the radical-scavenging mechanisms of phenolic antioxidants. In fact, a linear correlation was observed between the calculated O–H bond dissociation energies for artepillin C and its derivatives by DFT and the logarithms of their experimental radical-scavenging rate constants (k) (log k) [Citation8,Citation9]. We report herein the radical-scavenging rate constants (k) for seven flavonols, which are Myr, Que, Mor, Kae, and three methylated quercetin analogs (2′-MeQue, 5′-MeQue, and Me2Que) () in an aprotic medium. The mechanisms of the radical-scavenging reaction are discussed based on the relationships between the k values and thermodynamic parameters calculated by DFT, providing an excellent opportunity to understand the structure–activity and structure–mechanism relationships affected by the substituents in the B ring of flavonols.
Materials and methods
Materials
Commercially available reagents, such as galvinoxyl radical (GO•), Myr, Que, Mor, and Kae, were the best available purity and used without further purification unless otherwise noted. Acetonitrile (MeCN) used as a solvent was purchased from Nacalai Tesque, Inc. Japan, and used as received. Methylated quercetin derivatives were synthesised according to literature procedures [Citation14].
Spectral and kinetic measurements
To avoid the effect of molecular oxygen (O2), the reactions were carried out under strictly deaerated conditions. A continuous flow of argon (Ar) gas was bubbled through an MeCN solution (3.0 mL) containing GO• or an antioxidant in a quartz cuvette or a reservoir of the spectrophotometer for 7 min. UV-vis spectra were recorded on an Agilent 8453 photodiode array spectrophotometer. The rates of the scavenging reaction of GO• in deaerated MeCN by flavonols were determined by monitoring the absorbance at 428 nm due to GO• (ε = 1.4 × 105 M−1 cm−1) using a stopped-flow technique on a UNISOKU RSP-1000-02NM spectrophotometer. The pseudo-first-order rate constants (kobs) were determined by a least-squares curve fit using an Apple MacBook Pro personal computer. The first-order plots of ln(A – A∞) vs. time (A and A∞ are denoted as the absorbance at the reaction time and the final absorbance, respectively) were linear until three or more half-lives with the correlation coefficient r > 0.999.
Theoretical calculations
Density functional theory (DFT) calculations were performed on a 16-processor high performance computer (ForScientist XD1, HPC System Inc., Japan). The geometry optimisations were performed using the B3LYP/6-31++G(d) basis set with C-PCM solavation model parameterised for acetonitrile as implemented in the Gaussian 09 (Revision A.02, Gaussian, Inc.) [Citation15]. The calculated DHT (HT: hydrogen transfer) values are defined as the energy-difference values of heat of formations of phenoxyl radicals (ΔH(phenoxyl radical)) and phenols (ΔH(phenol)) (EquationEquation (1)(1)
(1) ). The bond dissociation energy (BDE) includes the heat of formation of hydrogen atom (ΔH(H•)) (EquationEquation (2)
(2)
(2) ). The ionisation potential (IP) values were calculated from the energy difference between the flavonol and the radical cation.
(1)
(1)
(2)
(2)
Results and discussion
The radical-scavenging rate constants (k) were determined using GO• as a reactivity model of ROS by a stopped-flow technique in deaerated MeCN.[Citation16] Upon mixing of GO• with Myr, the absorption band at 428 nm due to GO• decreased immediately as shown in . The decay of the absorbance at 428 nm monitored by a stopped-flow technique obeyed pseudo-first-order kinetics, when the concentration of Myr ([Myr]) was maintained at more than a 10-fold excess of GO• concentration. The pseudo-first-order rate constants (kobs) linearly increased with increasing [Myr] (). From the slope of the linear plot, the second-order-rate constant (k) for the GO•-scavenging by Myr was determined to be 3.0 × 104 M−1 s−1. The k values for other flavonols were also determined in a similar manner and the results are listed in .
Figure 2. Spectral change (interval: 30 ms) during the reaction of Myr (2.4 × 10−4 M) with GO• (6.1 × 10−6 M) in MeCN at 298 K; Inset: the first-order plot of the absorbance at 428 nm.
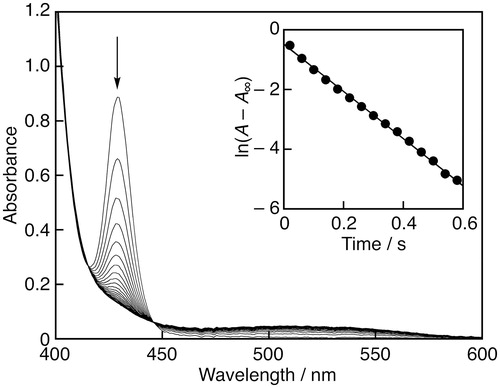
Table 1. Second-order rate constants (k) for GO•-scavenging reaction of the flavonols in MeCN at 298 K, the lowest energy difference values (DHT), and ionisation potentials (IP).
If the radical-scavenging reaction proceeds via the one-step hydrogen-atom transfer as the rate-determining step, the O–H bond dissociation energy of the OH group is an important parameter to control the reactivity of the radical-scavenging reaction. Thus, the O–H bond dissociation energies of all the OH groups in the flavonols, which equal the energy difference values (DHT) between the parent flavonols and the corresponding radicals, were determined by DFT calculation at the B3LYP/6-31++G(d) level with C-PCM = acetonitrile. The results are shown in and the smallest DHT values for each flavonol are listed in . On the other hand, the reactivity of the electron-transfer reaction of antioxidants in aprotic solvents, such as MeCN, is related to their ionisation potentials (IP), while in protic solvents, such as alcohols, the deprotonation energies for the phenolic OH groups as well as the IP values for the corresponding phenolate anions should be taken into account. Thus, the IP values for the flavonols were also calculated by DFT and the results are shown in .
Figure 4. DHT values (in kcal mol−1) for hydroxy groups in the flavonols calculated by DFT (B3LYP/6-31++G(d) level with C-PCM solvation model parameterised for acetonitrile).
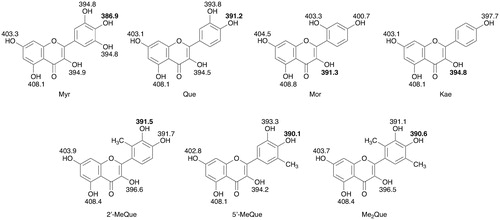
The DHT values for the OH groups at the C-4′ position are the smallest for Myr, Que, 5′-MeQue, and Me2Que, while the smallest DHT value in 2′-MeQue is for the OH groups at C-3′. On the other hand, the DHT values for the OH group at the C-3 position in the C ring are the smallest for Mor and Kae. The hydrogen-donor ability increases in the order Kae < 2′-MeQue ≈ Mor ≈ Que < Me2Que < 5′-MeQue < Myr. On the other hand, the electron-donor ability increases in the order Mor < 2′-MeQue < Kae ≈ Me2Que < Myr ≈ Que < 5′-MeQue. shows plots of log k values vs. DHT or IP values. Among the seven flavonols, the log k values of four flavonols, which are 5′-MeQue, Myr, Me2Que, and 2′-MeQue, revealed the linear correlation with the IP values as shown in . Thus, the GO•-scavenging reaction of these four flavonols may proceeds via an electron transfer followed by proton transfer. No linear correlation was observed in the plot of log k vs. DHT as shown in . Further experiments including effects of redox-inactive metal ions, which are known to accelerate the electron-transfer reaction [Citation10–12,Citation17–20], on the radical-scavenging rates are underway to elucidate the detailed radical-scavenging mechanism of flavonols.
Disclosure statement
The authors report no conflict of interest.
Additional information
Funding
References
- Quideau S, Deffieux D, Douat-Casassus C, et al. Plant polyphenols: chemical properties, biological activities, and synthesis. Angew Chem Int Ed Engl. 2011;50(3):586–621.
- Sharma A, Kashyap D, Sak K, et al. Therapeutic charm of quercetin and its derivatives: a review of research and patents. Pharm Pat Anal. 2018;7(1):15–32.
- Musialik M, Kuzmicz R, Pawłowski TS, et al. Acidity of hydroxyl groups: an overlooked influence on antiradical properties of flavonoids. J Org Chem. 2009;74(7):2699–2709.
- Foti MC, Daquino C, DiLabio GA, et al. Kinetics of the oxidation of quercetin by 2,2-diphenyl-1-picrylhydrazyl (dpph•). Org Lett. 2011;13(18):4826–4829.
- Stepanić V, Matić S, Amić A, et al. Effects of conjugation metabolism on radical scavenging and transport properties of quercetin – In silico study. J Mol Graph Model. 2019; 86:278–285.
- Wright JS, Johnson ER, DiLabio GA. Predicting the activity of phenolic antioxidants: theoretical method, analysis of substituent effects, and application to major families of antioxidants. J Am Chem Soc. 2001;123(6):1173–1183.
- Leopoldini M, Marino T, Russo N, et al. Antioxidant properties of phenolic compounds: H-atom versus electron transfer mechanism. J Phys Chem A. 2004;108(22):4916–4922.
- Kawashima T, Manda S, Uto Y, et al. Kinetics and mechanism for the scavenging reaction of the 2,2-diphenyl-1-picrylhydrazyl radical by synthetic artepillin C analogues. BCSJ. 2012;85(8):877–883.
- Manda S, Nakanishi I, Ohkubo K, et al. Enhanced radical-scavenging activity of naturally-oriented artepillin C derivatives. Chem Commun. 2008;(5):626–628.
- Nakanishi I, Shimada T, Ohkubo K, et al. Involvement of electron transfer in the radical-scavenging reaction of resveratrol. Chem Lett. 2007;36(10):1276–1277.
- Nakanishi I, Kawashima T, Ohkubo K, et al. Electron-transfer mechanism in radical-scavenging reactions by a vitamin E model in a protic medium. Org Biomol Chem. 2005;3(4):626–629.
- Nakanishi I, Miyazaki K, Shimada T, et al. Effects of metal ions distinguishing between one-step hydrogen- and electron-transfer mechanisms for the radical-scavenging reaction of (+)-catechin. J Phys Chem A. 2002;106(46):11123–11126.
- Nakanishi I, Fukuhara K, Shimada T, et al. Effects of magnesium ion on kinetic stability and spin distribution of phenoxyl radical derived from a vitamin E analogue: mechanistic insight into antioxidative hydrogen transfer reaction of vitamin E. J Chem Soc, Perkin Trans 2. 2002;(9):1520–1524.
- Imai K, Nakanishi I, Ohkubo K, et al. Synthesis of methylated quercetin analogues for enhancement of radical-scavenging activity. RSC Adv. 2017;7(29):17968–17979.
- Frisch J, Trucks GW, Schlegel HB, et al. Gaussian 09, Revision A.02. Wallingford, CT: Gaussian, Inc.; 2009.
- Shi H, Noguchi N, Niki E. Galvinoxyl method for standardizing electron and proton donation activity. Methods Enzymol. 2001;335:157–166.
- Fukuzumi S, Ohkubo K. Metal ion-coupled and decoupled electron transfer. Coord. Chem. Rev. 2010;254(3-4):372–385.
- Fukuzumi S, Ohkubo K, Morimoto Y. Mechanisms of metal ion-coupled electron transfer. Phys Chem Chem Phys. 2012;14(24):8472.
- Nakanishi I, Ohkubo K, Miyazaki K, et al. A Planar Catechin Analogue Having a More Negative Oxidation Potential than (+)-Catechin as an Electron Transfer Antioxidant against a Peroxyl Radical. Chem Res Toxicol. 2004;17(1):26–31.
- Nakanishi I, Kawaguchi K, Ohkubo K, et al. Scandium Ion-accelerated Scavenging Reaction of Cumylperoxyl Radical by a Cyclic Nitroxyl Radical via Electron Transfer. Chem Lett. 2007;36(3):378–379.