Abstract
The stability of liposomal formulations is a key issue in drug delivery. Liposomes made of egg phosphatidylcholine (EPC), cholesterol (Chol), sphingomyelin (SM), and gangliosides (GM1 and GM type III) were incubated in different media to determine their stability. Mixtures containing GM1 or GM type III were found to be the most stable, and both showed similar stability trends in plasma at 37°C. EPC/Chol was the most susceptible to lysis in plasma. In acid media (pH 2), the highest stability corresponded to EPC/Chol, whereas in bile and pancreatin, liposomes with GM1 and GM type III were more stable than those containing SM. This study suggests that among the formulations used as oral drug carriers, those containing GM1 and GM type III have higher possibilities of surviving through the gastrointestinal tract.
Liposomes, one or more phospholipid bilayers enclosing an aqueous phase, were first proposed as carriers of biologically active-substances (Gregoriadis and Ryman Citation1971) and have been extensively studied as suitable vehicles for the delivery of drugs, proteins, peptides, and DNA (Allen Citation1997; Barrat Citation2000). Water–soluble drugs can be placed within the aqueous compartment and lipophylic or amphipathic compounds can be associated with the bilayer.
The stability of liposomal systems, both in vitro and in vivo, always has been a matter of interest, and therefore many particulates and liposomes of different compositions have been studied in our laboratory (Alonso-Romanowski et al. Citation2003; Fabani et al. Citation2002). The possible therapeutic benefits of liposomal-encapsulated drugs depend on their lifetime and distribution within the organism, which are factors related to their stability. After intravenous administration, liposomes are destabilized as a consequence of their interaction with circulating lipoproteins, and after subcutaneous or intraperitoneal administration, small liposomes and nanoparticles are taken up by regional lymph nodes (Moghimi et al. Citation1994; Maincent et al. Citation1992). Previous reports showed that small liposomes and those containing some negatively charged lipids, e.g., ganglioside GM1 or hydrogenated phosphatidylinositol, remain in the bloodstream longer than the other types (Woodle Citation1998). Moreover, small, rigid, hydrophilic vesicles that do not agglutinate proteins were found to have higher stability in plasma (Oku et al. Citation2000).
Oral delivery is the most easy and convenient route for drug administration. In the 1c decade, the interest in liposomes has increased enormously since they are considered suitable oral drug carriers. Therefore, it is important to determine the stability of these formulations in stomach acidic pH and gastrointestinal medium.
The environment of the gastrointestinal (GI) tract can disrupt a large percentage of these carriers. The concerted action of duodenal enzymes and bile salts destroy the lipid bilayer of most liposomes and consequently the transported drug, either encapsulated or bound at the membrane level, is released. There have been few reports on liposomes carrying antigens to enhance mucosal immune response, which were delivered to Peyer's patches without suffering degradation or release of entrapped substance during the passage through the GI tract (Aramaki et al. Citation1993).
Taking into account these considerations, it is important to elucidate the stability of liposomes in bile, pancreatin, and acidic solutions in relation to their application in oral delivery, e.g., oral vaccines.
The aim of this study was to assess liposome stability in adverse conditions to reveal the potential of the given formulations. Two different methods were applied: an enzymatic method that involved glucose-6-phosphate (G6P) release through glucose-6-phosphate-dehydrogenase (GPDH) activity, and a method based on the quantification of bovine serum albumin (BSA) entrapped in the vesicles. The selected formulations included egg lecithin (EPC), cholesterol (Chol), sphingomyelin (SM), and gangliosides (GM1 or GM type III), and were chosen due to their particular properties at pH 7.4 buffer and plasma.
Results indicate that formulations containing GM1 or GM type III may be suitable as oral delivery vehicles due to their stable behavior through the oral route.
MATERIALS AND METHODS
Materials
Phospholipids and sphingolipids were from Avanti Polar Lipids (Alabaster, AL, USA)—egg lecithin (EPC) and bovine brain sphingomyelin (SM). Gangliosides GM Type III, purified from bovine brain (20% N-acetylneuraminic acid), and GM1 (95%), BSA (minimum 96%-electrophoresis, A-8022); 4,4′-dicarboxy-2, 2′-biquinoline (Bicinchoninic acid: BCA); G6P and GPDH were from Sigma Co. (St. Louis, MO, USA). Chol was from Riedel de Häen (A. G, Germany). Sephadex G-50 and G-75 were from Pharmacia Fine Chemicals (Upsala, Sweden). Pancreatin USP (porcine pancreas) and bile (bacteriological grade) were from ICN, Biomedical (Aurora, OH, USA). Human saliva was obtained from healthy donors. All the other reagents were of analytical grade.
Methods
Liposome Preparation
Lipids were dissolved in chloroform or ethanol in a round bottom flask, dried in a rotary evaporator under reduced pressure at 37°C, and fluxed with N2 to form a film. The dry lipid film (40 μ mol total lipid concentration), which contained EPC/Chol (1:1, mol ratio), EPC/Chol/SM (1:1:1, mol ratio), EPC/Chol/SM/ GM type III (1:1:1:0.14, mol ratio), and EPC/Chol/SM/GM1 (1:1:1:0.14, mol ratio), was hydrated with 10 mM Tris-HCl buffer, pH 7.4, to a final 50 mM lipid concentration (Bangham, Standish, and Watkins Citation1965). The suspension buffer also contained 1M G6P for the enzymatic stability determination or 1 mg/ml BSA for the protein release assay.
The liposomal solution was kept for 1 hr at 37°C. Large unilamellar vesicles (LUV) of circa 0.2 μ m were obtained by passing the mixture, at least 15 times, through a Miniextruder (Avanti Polar Lipids) containing a 0.2-μ m pore polycarbonate membrane, with temperature control (50°C). Samples were loaded onto a Sephadex minicolumn (1 ml tuberculin syringe) and equilibrated with 10 mM Tris-HCl buffer, pH 7.4. Then, they were eluted at room temperature spinning at 2,000 g for 2 min to remove nonentrapped G6P (Sephadex-G50 column) or BSA (Sephadex-G75 column). Aliquots of 500 μ l liposomes were incubated in 1000 μ l 10 mM Tes buffer (pH 7.4), plasma, 10 mM citrate buffer (pH 2), 10% bovine bile, or 2.8% pancreatin solution in 10 mM Tris buffer (pH 7.4), with 154 mM NaCl. Incubations were carried out at room temperature or at 37°C.
Liposome Stability Determination
Enzymatic Method. In the glycolytic degradation pathway, glucose undergoes two enzymatic reactions: D-glucose → D-glucose-6-phosphate → D-glucono δ -lactone-6-phosphate + NADPH. The first reaction is catalyzed by hexokinase, and the second by GPDH. Glucose can be encapsulated and liposome stability, determined through glucose release, measuring NADPH enzymatic formation. We have chosen an alternative assay to encapsulate glucose-6-phosphate (G6P). In this case, hexokinase and ATP are not required. After encapsulation and separation of free G6P, 500 μ l liposomes were incubated in 1000 μl of the predetermined medium at the selected temperature. Released G6P was evaluated during 3 hr at 15-min intervals through GPDH enzyme kinetics, following the absorbance at λ = 340 nm in a Shimadzu UV 160-A UV-visible recording spectrophotometer (Japan) (Worthington Citation1988). The amount of G6P released at each time point was calculated from the slope of enzyme kinetics. Total sugar entrapped (100%) was determined after complete liposome lysis using 0.5% Triton X-100. Release percentage was calculated as follows:
where, At = absorbance after incubation at different time-points, A0 = absorbance at initial time, and A100 = absorbance after complete liposome lysis with 0.5% Triton X-100.
Protein Encapsulation Method. The other method used to evaluate liposome stability consisted in measuring the release of BSA encapsulated. After incubation in different media and/or temperatures, the concentration of the remaining BSA encapsulated was determined using the bicinchoninic acid method (BCA method) (Smith et al. Citation1985). Taking into account that BCA interacts with phospholipids, and particularly with unsaturated ones, samples were previously incubated with 0.2% Triton X-100 for 2 hr at room temperature (Ahl et al. Citation1990). Then, they were centrifuged at 14,000 g for 20 min and the protein assay was carried out using adequate aliquots of the supernatant. Leakage percentage was calculated on the basis of the ratio of BSA retained at different time points with respect to total BSA encapsulated and measured at the initial time, as follows:
where, At = absorbance corresponding to BSA after incubation at different time points and A0 = absorbance corresponding to BSA at the initial time. The determination was carried out for 3 hr with 30-min intervals.
This alternative method was performed because incubations at low pH or other extreme conditions may induce partial or total loss of enzyme activity, and GPDH-based enzymatic method described before may became unreliable.
RESULTS
Liposome Stability in Buffer and Plasma
Liposomes were prepared in a buffer containing G6P and stability was studied following the changes in absorbance observed in GPDH enzyme kinetics after incubation in buffer Tes, pH 7.4, or in plasma at 25°C and 37°C. Changes in enzyme kinetics were measured in the following formulations: EPC/Chol (1:1), EPC/Chol/SM (1:1:1), and EPC/Chol/SM/GM type III or GM1 (1:1:1:0,14), all expressed as mol ratio.
1 Stability of EPC/Chol (□), EPC/Chol/SM (○), EPC/Chol/SM/GM1 (♦), and EPC/Chol/SM/GM type III (dtrif;) liposomes in buffer Tes at 25°C (A) and 37°C (B) measured by the enzyme kinetics method. Each point represents mean values of experiments performed in triplicate. Percentage values varied less than ±5% between determinations.
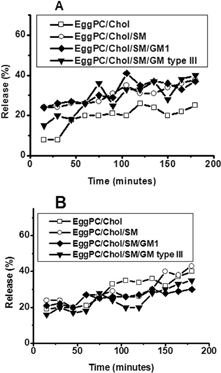
EPC/Chol showed the highest stability in buffer at 25°C, with leakage varying between 10–20% for 120 min and 20–25% between 120 and 180 min (). The other three formulations were less stable in these conditions, with leakage ranging between 25–35% for EPC/Chol/SM/GM1 and EPC/Chol/SM. On the other hand, the more stable formulations in buffer at 37°C were those containing GM1 or GM type III (). Both showed 15–30% leakage up to 3-hr incubation. EPC/Chol showed 20% leakage approximately for 90 min and increased to almost 50% at longer times.
2 Stability of EPC/Chol (□), EPC/Chol/SM (○), EPC/Chol/SM/GM1 (♦), and EPC/Chol/SM/GM type III (▾) liposomes in plasma at 25°C (A) and 37°C (B) measured by the enzyme kinetics method. Each point represents mean values of experiments performed in triplicate. Percentage values varied less than ±5% between determinations.

Liposome incubation in 100% plasma changed the stability pattern obtained. EPC/Chol was the formulation with the highest leakage, both at 25°C and 37°C (), and the level of G6P released was about 60% at both temperatures. The incorporation of SM diminished this percentage at lower times, but in ∼3-hr leakage was similar to the one observed in EPC/Chol alone. On the other hand, GM1 or GM type III added to EPC/Chol/SM reduced lysis to 10–30% at both temperatures assayed.
3 Stability of EPC/Chol (□), EPC/Chol/SM (○), EPC/Chol/SM/GM1 (♦), and EPC/Chol/SM/GM type III (▾) liposomes in acid media, pH: 2 at 37°C measured by BSA retained. Each point represents mean values of experiments performed in triplicate. Percentage values varied less than ±7% between determinations.

4 Stability of EPC/Chol (□), EPC/Chol/SM (○), EPC/Chol/SM/GM1 (♦), and EPC/Chol/SM/GM type III (▾) liposomes in bile (A) and pancreatin (B), incubated at 37°C, measured by the enzyme kinetics method. Each point represents mean values of experiments performed in triplicate. Percentage values varied less than ±5% between determinations.
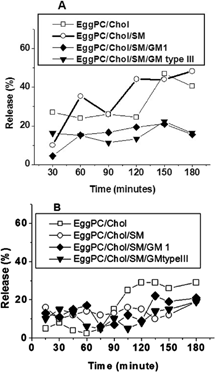
Liposome Stability at pH 2
G6PD is inactivated at pH 2. Therefore, it was crucial to assay stability in acidic conditions. The method selected consisted in the encapsulation of BSA, followed by the determination of BSA retained as a function of time. EPC/Chol incubation in an acidic buffer () showed 20% release within the first hour, and 30% on the following 2 hr. On the other hand, BSA release percentage in the formulation containing SM and gangliosides (GM 1 or GM type III) was 20% after 30 min, but it reached 60–65% after 3 hr.
Liposome Stability Altered by Bile or Pancreatin
Orally administered drugs can be altered by bile or pancreatin in the guts, so it is important to evaluate the effect of both substances on different liposomal formulations. shows liposome incubation in 10% bile solution containing 154 mM NaCl. Formulations made with GM1 and GM type III were the more stable (∼15% G6P release as a function of time). EPC/Chol exhibited 30% leakage for 120 min and 45% between 120 and 180 min. Values for EPC/Chol/SM were similar, whereas a higher leakage percentage was obtained for EPC/Chol at shorter times.
When liposomes were incubated in 2.8% pancreatin, EPC/ Chol showed the lowest leakage level (around 5%) for the first hour but after 90 min it exhibited the highest G6P release (30%) (). The other three formulations showed a similar behavior, with leakage levels between 5–15% for 120 min and an increase around 20% afterward.
These results indicate that the stability of liposomes containing GM1 and GM type III is relatively high in all media analyzed, except at pH 2. On the other hand, EPC/Chol showed the highest stability at pH 2, but the lowest stability in other conditions. As we mentioned, the results described refer to specific analyses of leakage percentages in different media. From a general point of view, however, none of the formulations follows a distinct pattern in acid pH, bile, or pancreatin. Nevertheless, it is possible EPC/Chol/SM samples are the less favorable for oral delivery, and that the other three formulations present similar stability behavior ().
DISCUSSION
The approach presented here was designed to compare experiments carried out at different conditions and with different methodologies, analyzing leakage percentages from a variety of lipid mixtures in the presence of undiluted human plasma or biological fluids like bile, pancreatin, and acid pH. This approach approximates in vivo conditions using these lipid systems as in vitro models to predict their stability as potential oral drug carriers.
5 Comparative stability of release percentage in different formulations. Column 1: EPC/Chol, column 2: EPC/Chol/SM, column 3: EPC/Chol/SM/GM1, and column 4: EPC/Chol/SM/ GM type III. Each column represents the percentage of drug released after 3 hr in acid pH (), bile (), and pancreatin (). Incubations were carried out at 37°C.

Liposome leakage is generally measured using a modification technique (Weinstein et al. 1977) in which a highly quenched fluorescent compound such as 6-carboxyfluorescein, fluorescein, or calcein is introduced within the liposome. Allen and Cleland (Citation1980) however, reported that when free calcein was incubated with plasma and the mixture was passed through a Sephadex G-50 column, a portion of the fluorescent compound was detected in the void volume, thus indicating that calcein binds to plasma proteins. This might complicate the interpretation of data obtained from in vivo or in vitro studies using any of these compounds as fluorescent markers.
In the enzymatic method already described, the enzyme itself could be used as a marker, measuring its activity to detect proteins inside and outside the liposomes. It is worth mentioning that the process of entrapment itself could have a deleterious effect on enzyme activity. However, this can be allowed for, if activity is measured before and after liposome solubilization. Another possibility is to entrap a substrate and then measure enzyme activity, which will be related to the substrate released from the liposomes.
The enzyme kinetics assay described has five main advantages:
G6PD kinetic curves are highly reproducible; a number of experiments carried out with different preparations of the same lipid composition yielded the same stability curves.
The probe can be easily inserted in the bilayer and the nonencapsulated by substance can be easily separated from free material.
It is a low-cost technique.
It is relatively insensitive to small variations in experimental conditions.
Results are easily calculated and analyzed.
The main disadvantage of the enzymatic determination is that it is not instantaneous and, therefore, it is not suitable in kinetic studies in which release is fairly rapid. Its most useful application has been the quantification of immune lysis of antigen-bearing liposomes in the presence of antibody and complement (Shichijo, Toffano, and Alving Citation1985).
Our results showed lower stability in plasma than in buffer in all the formulations assayed, since interaction with plasma proteins induced higher leakage than expected. In this regard, Allen and Cleland (Citation1980) demonstrated that the efflux of contents from small unilamellar vesicles of different compositions containing 175 mM calcein significantly increased in the presence of serum. Moreover, [14C] sucrose leakage in egg lecithin liposomes incubated at 37°C also was dramatically increased in the presence of serum. Likewise, they reported a 30-fold increment in calcein leakage from egg phosphatidylcholine liposomes incubated at 22°C in the presence of 50% and 70% serum. At the same experimental conditions, we observed an almost three times lower G6P leakage in EPC/Chol. This could be due to differences in the methodology and substrate used. Allen and Cleland (Citation1980) also reported that sucrose showed lower leakage than calcein. They argue that liposomes seem to have a finite leakage toward calcein, as compared with the less permeable sucrose molecule. According to Allen, this can be attributed to the nature of the calcein molecule, partially polar due to its charged carboxyl groups and partially nonpolar because of its aromatic rings.
On the basis of our results, we cannot say that G6P has a finite leakage, although it depends solely on the amount of G6P release. G6P is comparable to sucrose since it is a sugar, but it also has a negative charge (given by the phosphates) can be associated to calcein. It can be inferred, then, that this molecule has characteristics from both compounds.
Reports from Allen and Cleland (Citation1980) agreed with our results on higher 14C sucrose or calcein leakage levels observed in formulations incubated in buffer or serum at 37°C. This may be due to the higher release rate caused by the greater permeability observed in trapped compounds at temperatures close to transition values.
The incorporation of increasing molar ratios of cholesterol, gangliosides (either GM1 or GM type III), and sphingomyelin into liposomes reduced G6P release in samples incubated with buffer or serum. Gangliosides reduced the leakage of aqueous contents from liposomes, a phenomenon induced by plasma protein that has been correlated with a decrease in reticuloendothelial system uptake. It also has been reported that liposomes with the longest circulation half-life mimic the outer surface of red blood cell membranes. GM1 seems to have the unique ability of prolonging circulation half-life, partly due to its molecular conformation, the location of the negative charge regarding the phospholipid bilayer and carbohydrate backbone, and the packing characteristics of its phospholipid bilayer.
It also is worth mentioning the high stability observed in formulations containing sphingomyelin and gangliosides incubated in bile and pancreatin. In oral drug delivery it is important to guarantee the maintenance of the encapsulated material for longer periods of time. The worst barrier to overcome is stomach acid medium, in which these formulations showed higher leakage levels. Comparative methods used to measure G6P and BSA release percentage in EPC/Chol/SM/GM1 liposomes incubated in buffer Tes at 25°C showed similar trends, demonstrating that results were independent of the method used (data not shown). This discards the notion that the high lysis percentage observed at pH 2 depended on the methodology employed. In this regard, previous reports demonstrated that dioleoylphosphatidylethanolamine/dioleoylphosphatidylcholine/ bis-(6-hemisuccinyloxyhexyl) muconate and dioleoylphosphati- dylethanolamine/dioleoylphosphatidylcholine/bis-(6-hemisuccinyl-oxyhexyl) fumarate liposomes were stable at neutral pH, but leaky at acidic pH. Calcein release was pH-sensitive and time-dependent, since liposomes incubated at pH 4.4 and 5.8 had increased fluorescence intensity (20 to 60%) and reached a plateau within 40–50 min (Jin and Lee Citation1998). Using the same methodology, Han et al. (Citation1997) reported that liposomes composed of dipalmitoylphophatidylcholine and cholesterol showed higher leakage at pH 2 than in buffer at neutral pH (7.4). Finally, based on previous reports (Fabani et al. Citation2002) in which EPC/Chol/SM/GM1 was stable in saliva for at least 1 hr and on our own results, we believe that this formulation would be the most stable and therefore the most suitable for oral delivery.
An understanding of the main processes that affect liposome integrity in vivo is vital for the effective use of encapsulated drugs in the treatment of disease. The influence of liposome composition on their circulation lifetime is a priority for the biodistribution of liposomal content. It also is crucial to determine stability in different media and to have the optimal methodology according to the environment studied.
CONCLUSION
We described two methods to measure stability that can be used in the conditions in which this parameter is a key issue: stomach acid pH, synthetic neutral pH (buffer), and plasma neutral pH. In spite of using two different procedures, the data obtained are comparable and completely reliable. We believe that these observations make possible a number of liposome applications that have not been developed previously.
This work was supported by grants from the Ministerio de Salud de la Nación (Argentina), CONICET (Consejo Nacional de Investigaciones Científicas y Técnicas), and UNQ (Universidad Nacional de Quilmes). Dr. S. Alonso-Romanowski is a member of the Scientific Research Program of the CONICET.
REFERENCES
- Ahl P. L., Price R. P., Gaber B. P., Singh A.. 1990. Insertion of bacteriorhodopsin into polymerized diacetylenic phosphatidylcholine bilayers. Biochim. Biophys. Acta. 1028: 141–153. [PUBMED], [INFOTRIEVE]
- Allen T. M.. 1997. Liposomes–opportunities in drug-delivery. Drugs. 54: 8–14. [PUBMED], [INFOTRIEVE]
- Allen T. M., Cleland L. G.. 1980. Plasma-induced leakage of liposome contents. Biochim. Biophys Acta. 597: 418–426. [CROSSREF], [PUBMED], [INFOTRIEVE]
- Alonso-Romanowski S., Chiaramoni N. S., Lioy V. S., Gargini R. A., Viera L. I., Taira M. C.. 2003. Characterization of diacetylenic liposomes as carriers for oral vaccines. Chem. Phys. Lipids. 122: 191–203. [CROSSREF], [PUBMED], [INFOTRIEVE]
- Aramaki Y., Tomizawa H., Hara T., Yachi K., Kikuchi H., Tsuchiya S.. 1993. Stability of liposomes in vitro and their uptake by rat Peyer's patches following oral administration. Pharm Res.. 10: 1228–1231. [CROSSREF], [PUBMED], [INFOTRIEVE]
- Bangham A. D., Standish M. M., Watkins J. C.. 1965. Diffusion of univalent ions across lamellae of swollen phospholipids. J. Mol. Biol.. 13: 238–252. [PUBMED], [INFOTRIEVE]
- Barrat G. M.. 2000. Therapeutic applications of colloidal drug carriers. Physical structure to therapeutic applications. PSTT. 3(5)163–171
- Fabani M. M., Gargini R. A., Taira M. C., Iacono R., Alonso-Romanowski S.. 2002. Study of in vitro stability of liposomes and in vivo antibody response to antigen associated with liposomes after oral and subcutaneous immunization. J. Liposome Res.. 12(1,2)13–27. [CROSSREF], [PUBMED], [INFOTRIEVE]
- Gregoriadis G., Ryman B. E.. 1971. Liposomes as carriers of enzymes or drugs: A new approach to the treatment of storage diseases. Biochem. J.. 124(5)58P, [PUBMED], [INFOTRIEVE]
- Han M., Watarai S., Kobayashi K., Yasuda T.. 1997. Application of liposomes for development of oral vaccines: Study on In vitro stability of liposomes and antibody response to antigen associated with liposomes after oral immunization. J. Vet. Med. Sci.. 59(12)1109–1114. [CROSSREF], [PUBMED], [INFOTRIEVE]
- Jin J. Y., Lee Y. S.. 1998. New pH-sensitive liposomes using Bis-(6-hemisuccinyloxyhexyl) fumarate. Bull. Korean Chem. Soc.. 19(6)645–649
- Maincent P., Thouvenot P., Amicabile C., Hoffman M., Kreuter J., Couvreur P., Devissaguet J. P.. 1992. Lymphatic targeting of polymeric nanoparticles after intraperitoneal administration in rats. Pharm. Res.. 9: 1534–1539. [CROSSREF], [PUBMED], [INFOTRIEVE]
- Moghimi S. M., Hawley A. E., Christy N. M., Gray T., Illum L., Davis S. S.. 1994. Surface engineered nanospheres with enhanced drainage into lymphatics and uptake by macrophages of the regional lymph nodes. FEBS Lett.. 344(1): 5–30
- Oku N., Tokudome Y., Asai T., Tsukada H.. 2000. Evaluation of drug targeting strategies and liposomal trafficking. Curr. Pharm. Design.. 6: 1669–1691
- Shichijo S., Toffano G., Alving C. R.. 1985. Complement-dependant immune damage to liposomes containing Gangliosides. J. Immunol. Methods. 85(1)53–63. [CROSSREF], [PUBMED], [INFOTRIEVE]
- Smith P. K., Krohn R. I., Hermanson G. T., Mallia A. K., Gartner M. D., Provenzano F. H., Fujimoto E. K., Goeke N. M., Olson B. J., Klenck D. C.. 1985. Measurement of protein using bicinchoninic acid. Anal. Biochem.. 150: 76–85. [PUBMED], [INFOTRIEVE]
- Weinsten J. N., Yoshikami S., Henkart P., Blumenthal R., Hagins W. A.. 1977. Liposome-cell interaction: Transfer and intracellular release of a trapped fluorescent marker. Science. 195: 489–492
- Woodle M. C.. 1998. Controlling liposomes blood clearance by surface-grafted polymers. Adv. Drug Deliv. Res.. 32: 139–153. [CROSSREF]
- Worthington C. C., 1988; Worthington Manual. Enzymes and related biochemicals.. 159–161, Freehold, NJ, Worthington Biochemical Corporation