Abstract
Uptake of novel inherently fluorescent microspheres composed of a luminescent polyanhydride, poly[p-(carboxyethylformamido)- benzoic anhydride] (PCEFB), and poly(lactide-co-glycolide) (PLGA) (2:1, weight ratio) by the gastrointestinal tract was evaluated by fluorescent microscopy. Oral efficiency of the incorporated insulin also was determined by measuring reduction of plasma glucose levels after feeding diabetic rats with a single dose of the microspheres. We found that PCEFB/PLGA microspheres could adhere to the intestinal epithelium and traverse the absorptive cells. A large number of the spheres were observed in spleen, whereas few were detected in liver within the evaluated period of time. Apparent reduction of the plasma glucose levels was observed over a span of 6 h postfeeding. The unique properties of the delivery system such as biodegradability, bioadhesivity, and inherently luminescent characteristics render it an ideal “visible” tracer for monitoring oral fate of polymeric microspheres.
Oral absorption of polymeric nano- and microparticles by the gastrointestinal tract (GIT) has been extensively studied for the past decade. The factors affecting intestinal uptake of particles are now better known. Size, nature of the polymer, zeta potential, vehicle, and coating have been determined as critical factors (Ebel Citation1990; Jepson et al. Citation1993; Florence, Hillery, and Jani Citation1995; Thanos et al. Citation2000). Fluorometry was the most used tool to assess intestinal uptake, qualitatively (histology) as well as quantitatively due to its high sensitivity (Delie Citation1998). In general, fluorescent dyes were directly blended with the carriers to label the microspheres. However, the presence of fluorescent dyes may influence surface properties of the studied particles, therefore changing the intrinsic interaction of particles with tissues (Delie Citation1998). In addition, the dye entrapped in microspheres will leach into tissue and interfere with the detection of the studied samples (Thanos et al. Citation2000). Numerous biopolymers also can emit light, which may interfere with the detection of the particles in vivo. It is preferred to utilize biodegradable/biocompatible materials with intrinsic fluorescent properties to circumvent the above limitations.
In our previous works, we found a class of intrinsically fluorescent polyanhydrides, emission wavelength of which varies with the excitation wavelength, 520 nm with the excitation wavelength at 470 nm and 430 nm at 356 nm. No apparent fluorescence was observed for all the monomers and prepolymers (Jiang et al. Citation2001a–c). Such fluorescing properties of the polyanhydrides that the emission wavelength depends on the excitation wavelength may make it possible to prevent the detection of the polymers from the interference of in vivo fluorescent species in the background by excitation at two different wavelengths. Preliminary results of in vivo experiments suggested no apparent toxic reactions were taking place as the polymers were implanted subcutaneously into rat (Jiang et al. Citation2001b). Before using the fluorescent polyanhydride as in vivo visible tracer for monitoring oral fate of the microspheres, it is necessary to evaluate the bioadhesive property of poly(lactide-co-glycolide)/poly[p-(carboxyethylformamido)benzoic anhydride] (PLGA/PCEFB) microspheres by GIT by fluorescent microscopy. In addition, the oral bioavailability of microencapsulated insulin also was determined by measuring the plasma glucose levels of diabetic rats.
MATERIALS AND METHOD
Materials and Animals
D,L-PLGA 75:25 (Mw 12 000) were supplied by Birmingham Polymers, Inc. (Birmingham, AL, USA). PCEFB (Mw 9 200) was synthesized as the method previously described (Jiang et al. Citation2001c). Polyvinyl alcohol (PVA) of average Mw 31 000–50 000, 88% hydrolyzed, were obtained from Aldrich (Milwaukee, WI, USA). Bovine insulin (specific activity of 28.6 IU/mg) and bicinchoninic acid (BCA) were purchased from Sigma (St. Louis, MO, USA). Female SD rats (180–190 g) were used as animal test models. Diabetes was previously induced with a tail vein injection of 50 mg/kg of streptozotocin in pH 4.5, 0.1 M citrate buffer. An induction period of 3–5 days after strepotozotocin injection was necessary to appreciate the clinical onset of diabetes mellitus. When serum glucose levels reached a minimum of 35 mmol/l, the rats were considered diabetic.
Methods
Characterization
Microspheres were sized by laser diffractometry using a Malvern 2600D laser sizer. The freeze-dried microspheres were redispersed in double-distilled water and sonicated prior to analysis. Particle size was expressed as volume mean diameter in micrometers. Each sample was assayed in triplicate. The surface and internal morphology of different microspheres were analyzed by scanning electron microscopy (SEM, HITACA-520). Dried microspheres were mounted onto metal stubs using double-sided adhesive tape, vacuum-coated with a goldpalladium film, and directly analyzed under SEM. To characterize the internal morphology, the adhesive tape with stuck particles was first folded on itself and second roughly unfolded to fracture the microspheres.
Preparation of Microspheres
Insulin-loaded PCEFB/PLGA microspheres were prepared by a modified w/o/w emulsion solvent evaporation technique (Jiang and Zhu Citation2002). Briefly, 10 mg of insulin was dissolved in 1 ml of 100 mM Tris (pH 3.0), emulsified into 5 ml of polymer solution (30 mg/ml) in a mixture of methyl chloride and acetone with a mechanical stirrer to produce w/o emulsion, which was then poured into 20 ml of PVA aqueous solution (5%, w/v) under vigorous stirring. The w/o/w emulsion was subsequently stirred at room temperature for 30 min to allow solvent evaporation and microsphere formation. The microspheres were isolated by centrifugation, washed six times in double-distilled water, freeze-dried, and stored in refrigerator.
Determination of Insulin Entrapment in Microspheres
The amount of insulin in the microspheres was determined according to Hora et al. (Citation1990). About 20 mg of freeze-dried microspheres, accurately weighed, were dissolved in 5.0 ml of 0.1 M NaOH containing 5% (w/v) SDS, then neutralized by 1 N HCl solution. The sample was centrifuged and a (BCA) protein microassay was used to determine the insulin concentration in the supernatant. From this result, the percentage (w/w) of insulin entrapped per dry weight of microsphere was determined. Each sample was assayed in triplicate. The percentage of entrapment efficiency was expressed by relating the actual insulin entrapment to the theoretical insulin entrapment.
In Vitro Release Study
First, 20 mg of PCEFB/PLGA microspheres were placed in 2 ml of 0.1 M phosphate buffered saline (PBS), pH 7.4. The test was performed in a 37°C incubator-shaker at 50 rpm. Then at appropriate intervals, the samples were centrifuged at 4,000 g for 5 min, 1 ml was removed from the supernatant and replaced by fresh release medium. Insulin concentration was determined by BCA protein microassay.
Intraperitoneal Bioactivity of Insulin Formulations
Diabetic rats were fasted overnight, anesthetized by intraperitoneal injection with a mixture of ketamine HCl (87 mg/kg body wt) and xylazine (13 mg/kg body wt), weighted, and 0.2 ml blood samples were collected by tail vein bleed. Next, 15 mg of microspheres suspended in 1 ml of PBS was injected intraperitoneally. The rats were denied food, and blood samples were collected at 1.5 and 4 h postfeeding. The heparinized blood samples were centrifuged at 2000 g for 5 min and plasma was removed and saved for glucose monitoring.
Glucose Analysis
Plasma glucose levels were obtained by using the Trinder 100 glucose assay (Sigma) following the manufacturer's protocols.
Oral Bioactivity of Insulin Formulations
Microspheres in 2 ml of suspension buffer were fed to each anesthetized rat through a stainless steel orogastric tube. Repeat blood samples were taken at 1.5, 3, 4, and 6 h postfeeding. The blood samples were centrifuged and the plasma samples were collected for glucose analysis.
Histological Studies
Rats fasted overnight were fed a single dose of PCEFB/PLGA in 1 ml of saline and killed after 1.5, 4, 6, or 24 h. Segments of intestine, liver, spleen, and kidney were excised and embedded in OCT compound on dry ice. Sections of 6 μm thickness were obtained by using a cryostat (MicroM HM560) and were observed under fluorescent microscopy using a green filter for detection of the fluorescent microspheres (Olympus BX 50).
RESULTS AND DISCUSSION
Microspheres
Insulin-loaded PCEFB/PLGA (2:1, weight ratio) microspheres used for the in vivo experiments, had a mean particle size of 1.95 μm with an encapsulation efficiency of 48%. In general, the diameter of all the microspheres was less than 5 μm and ∼70% of the spheres are smaller than 2 μm. The microspheres were spherical and appeared to have a rough surface and rigid cross section and could be clearly visualized by fluorescent microscopy ().
1 Micrographs of insulin-loaded PCEFB/PLGA (2:1) microspheres by (a) fluorescent microscopy, (b) SEM representing surface morphology, and (c) SEM representing cross-section morphology.
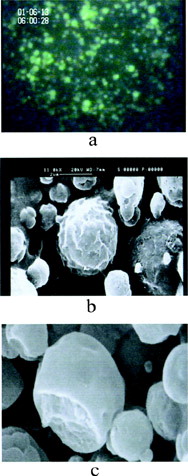
The in vitro release study demonstrated a burst effect with 28% of insulin released during the first 3 h followed by a 70% cumulative release by 4 days at exponentially decreasing release rates (). Only 10.4% of insulin was released from the microspheres within the first hour, which would be unavailable to intestinal uptake as it would be released in stomach and degraded rapidly. Such release characteristics are favorable for the microspheres to be used as an oral delivery system.
Histology
Mathiowitz et al. (Citation1997) reported that polymeric microspheres made of poly(fumaric-co-sebacic anhydride) (P(FA:SA)) displayed strong bioadhesion to intestinal epithelium. In vivo intestinal transmit studies indicated that P(FA:SA) 20:80 microspheres had a delayed intestinal transit when compared with particles made of alginate (Chickering and Mathiowitz Citation1995; Chickering et al. Citation1997). Moreover, large amounts of P(FA:SA) particles were seen in the intestinal absorptive cells, the Peyer's patches, and also in the spleen and liver tissues. In comparison, PLGA microspheres showed minimal uptake (Carino, Jacob, and Mathiowitz Citation2000). To evaluate the bioadhesive properties of the microspheres that were composed of polyanhydride (PCEFB) and PLGA, GIT uptake of PCEFB/PLGA microspheres was detected directly by fluorescent microscopy due to the inherently luminescent properties of PCEFB. It should be noted here that the fluorescent species were never observed in sections of tissue from untreated control animals under the evaluation conditions.
shows the cross-section of the rat intestine epithelium containing PCEFB/PLGA microspheres. As early as 1.5 h after feeding of a single dose, the microspheres were observed to traverse both the absorptive epithelium and the follicular epithelium. A large increase in uptake was found in absorptive cells and Peyer's patches after 6 h. Moreover, residence of the microspheres between epithelial cells was still observable 24 h postfeeding, indicating the strong bioadhesive properties of PCEFB/PLGA microspheres. Such results were similar to that reported by Carino et al. (Citation2000).
3 Micrographs of intestine samples (a) 1.5 h and (b) 6 h after feeding a single dose of PCEFB/PLGA microspheres.
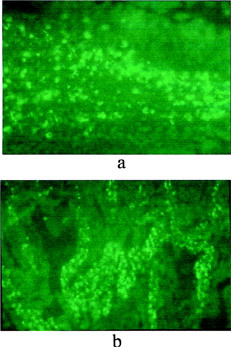
The presence of the microspheres in spleen and liver also was examined by fluorescent microscopy. In comparison with results reported by Mathiowitz et al. (Citation1997) that both spleen and liver tissue samples showed a large number of P(FA:SA) microspheres, PCEFB/PLGA microspheres were only found in spleen () and few microspheres resided in the liver samples during the evaluated period of time. There are numerous spheres observed in kidney at 6 h (). All the above results indicate that PCEFB/PLGA microspheres can be absorbed through the GIT tract and appeared in the blood.
Oral Efficiency of Insulin Formulations
The insulin formulation was found to be bioactive upon intraperitoneal injection, indicating that the microencapsulation process has little effect on the bioactivity of insulin. Oral efficiency of insulin formulation was evaluated by directly analyzing the plasma glucose level after feeding the rats with the microspheres containing 28 l U insulin. We observed that all four animals had a reduction of plasma glucose levels. Average glucose levels and derivation are represented in . It can be seen that as early as 1.5 h glucose levels have decreased to a rather low value, reach subcritical levels at 4 h, and then began to return to normal at about 6 h. Such results are similar to those reported by Mathiowitz et al. (Citation1997) who observed glucose reductions in normal rats fed the fumaric acid oligimer (FAO)/PLGA nanoparticles with Fe3O4 (Carino et al. Citation2000). They also found that the addition of Fe3O4 is necessary to reduce the plasma glucose to subcritical level. However, in our experiments it seemed that there was no need to add any additives into the microspheres to lower the plasma glucose to subcritical value.
6 Average plasma glucose levels in four rats fed 28 IU of insulin in PCEFB/PLGA (2:1) microspheres. The shadow refers to area under the curve for deviation from fasting plasma glucose levels (n = 6).
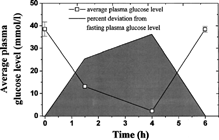
An approximate pharmacological availability can be calculated by the following equation:
[1]
where f is the pharmacological efficiency of the dose versus an i.p. dose, the AUCs0-6 are the areas under the reduction in serum glucose levels on a percent basis from 0 to 6 h, and the weight/dose are determined experimentally. The average deviations of plasma glucose levels measured in the four experimental rats are graphed versus time and the trapezoid rule is used to calculate the AUC0-6 (). Using that graph, the oral AUC is calculated to be 343.9% h. The AUCi.p was determined by the reduction of the plasma glucose levels after intraperitoneally administered insulin and was found to be 308.74% h at a dose of 4 IU in 180-g diabetic rats. Combining the two above results, the oral efficiency of insulin was calculated according to Eq. 1:
and was found to be 15.9%, which is comparable to the value of 11.4% reported by Carino et al. (Citation2000). These results further confirm that PCEFB/PLGA microspheres have strong bioadhesive properties and can traverse the absorptive cells.
CONCLUSION
The novel inherently fluorescent microspheres could be directly visualized in vivo by fluorescent microscopy without incorporating fluorescent dyes. Absorption of the microspheres by intestinal epithelium was observed, which could be attributed to the bioadhesive properties of PCEFB. Plasma glucose was reduced to subcritical levels within 4 h after feeding a single dose of insulin-loaded microspheres. Such unique properties of the microspheres as biodegradability, bioadhesion, and strong luminescent characteristics may render them ideal candidates as in vivo visible tracers for monitoring the oral absorption and transport process of bioadhesive microspheres.
This work is supported by the National Natural Science Foundation of China.
REFERENCES
- Carino G. P., Jacob J. S., Mathiowitz E.. 2000. Nanosphere based oral insulin delivery. J. Control. Rel.. 65: 261–269. [CSA]
- Chickering D. E., Mathiowitz E.. 1995. Bioadhesive microspheres: I. A novel electrobalance-based method to study adhesive interactions between individual microspheres and intestinal mucosa. J. Control. Rel.. 34: 251–261. [CROSSREF]
- Chickering D. E., Jacob J. S., Desai T. A., Harrison M., Harris W. P., Morrell C. N., Chaturvedi P., Mathiowitz E.. 1997. Bioadhesive microspheres. III. An in vivo transit and bioavailability study of drug-loaded alginate and poly(fumaric-co-sebacic anhydride) microspheres. J. Control. Rel.. 48: 35–48. [CROSSREF]
- Delie F.. 1998. Evaluation of nano- and microparticle uptake by the gastrointestinal tract. Adv. Drug Delivery Rev.. 34: 221–233. [CROSSREF], [CSA]
- Ebel J. P.. 1990. A method for quantifying particle absorption from the small intestinal of the rat. Pharm. Res.. 11: 205–214
- Florence A. T., Hillery A. M., Jani P. U.. 1995. Factors affecting the oral uptake and translocation of polystyrene nanoparticles: histological and analytical evidence. J. Drug Target. 3: 65–70. [PUBMED], [INFOTRIEVE], [CSA]
- Hora M. S., Rana R. S., Nunberg H., Tice T. R., Gilley R. M., Hudson M. E.. 1990. Release of human serum albumin from poly(lactide-co-glycolide) microspheres. Pharm. Res.. 7: 1190–1194. [PUBMED], [INFOTRIEVE], [CROSSREF]
- Jepson M. A., Simmons N. L., O'Hagan D. T., Hirsl, II, B.. 1993. Comparison of poly(DL-lactide-co-glycolide) and polystyrene microspheres to Peyer's patch M cells. J. Drug Target. 1: 245–249. [PUBMED], [INFOTRIEVE], [CSA]
- Jiang H. L., Zhu K. J., Dai L. J.. 2001a. New fluorescing polyanhydrides based on aliphatic and aromatic diacids. Macromol. Rapid Commun.. 22: 414–417. [CROSSREF]
- Jiang H. L., Tang G. P., Weng L. H., Zhu K. J.. 2001b. In vivo degradation and biocompatability of a new class of alternate poly(ester-anhydrides) based on aliphatic and aromatic diacids. J. Biomat. Sci. Polym Edt.. 12: 1281–1292
- Jiang H. L., Zhu K. J, Dai L. J.. 2001c. Synthesis and characterization of new biodegradable fluorescing poly(amide-anhydrides). Polym. Int.. 50: 722–727. [CROSSREF]
- Jiang H. L., Zhu K. J.. 2002. Bioadhesive fluorescent microspheres as visible carriers for local delivery of drugs. I. Preparation and characterization of insulin-loaded PCEFB/PLGA microspheres. J. Microencapsul.. 19: 451–461. [PUBMED], [INFOTRIEVE], [CROSSREF], [CSA]
- Mathiowitz E., Jacob J. S., Jong Y. S., Carino G. P., Chickering D., Charturved P., Santos C. A., Vijayaraghavan K., Montogomery S., Bassett M., Morrell C.. 1997. Biologically erodible microspheres as potential oral drug delivery systems. Nature. 386: 410–414. [PUBMED], [INFOTRIEVE], [CROSSREF]
- Thanos C. G., Yip K. P., Jacob Y. S., Mathiowitz E.. 2000. Effects of microsphere composition on uptake in the rabbit jejunum. Proc. Int. Symp. Control. Rel. Bioact. Mater.. 27: 780–781