Abstract
The aim of our present study was to prepare and evaluate a carvone-based transdermal therapeutic system (TTS) of nicorandil to find its ability in providing the desired in vivo controlled release profile on dermal application to human volunteers. The effect of EVA 2825, and adhesive-coated EVA 2825, and adhesive-coated EVA 2825-rat skin composite on the in vitro permeation of nicorandil from a carvone-based HPMC gel drug reservoir was studied against a control (rat abdominal skin alone). The carvone-based drug reservoir system was sandwiched between adhesive-coated EVA 2825-release liner composite and a backing membrane. The resultant drug reservoir sandwich was heat-sealed to produce a circle-shaped TTS (20 cm2) that was subjected to in vivo evaluation on dermal application to human volunteers against oral administration of immediate-release tablets of nicorandil. The carvone-based TTS provided a steady-state plasma concentration of 20.5 ng/ml for ∼24 hr in human volunteers. We concluded that the carvone-based TTS of nicorandil provided the desired in vivo controlled-release profile of the drug for the predetermined period of time.
In spite of the excellent achievement of pharmaceutical scientists in transdermal drug delivery, only a few transdermal formulations are marketed for drugs such as scopolamine, nitroglycerin, nicotine, clonidine, fentanyl, estradiol, testosterone, lidocaine, and oxybutinin (Prausnitz, Mitragotri, and Langer Citation2004). This reflects the limitation to deliver sufficient quantities of therapeutic agents across the skin to maintain the desired plasma concentration. The stratum corneum is the barrier to transdermal permeation of drugs across the skin. Overcoming this barrier safely without a permanent damage is a fundamental problem that persists in transdermal delivery. Barrier properties of the stratum corneum may be manipulated by using several techniques. The most promising technique is the use of penetration enhancers that allow drug permeation through the skin at an appropriate rate for a suitable time.
Willams and Barry (Citation2004) have reviewed the use of penetration enhancers for use in transdermal drug delivery systems. The safety of chemical penetration enhancers is of primary consideration while selecting them for use in the development of a membrane-moderated transdermal therapeutic system (TTS). Terpenes are of low cutaneous irritancy, generally regarded as safe, provide excellent enhancement ability, and thus appear to be promising candidates for transdermal formulations (Williams and Barry Citation2004; Gao and Singh Citation1998). A variety of terpenes has been shown to increase the percutaneous absorption of both hydrophilic and lipophilic drugs (Zhao and Singh Citation1998; El-Kattan et al. Citation2001), and hence could be used as penetration enhancers for increasing the permeation of a lipophilic drug such as nicorandil from a TTS.
Nicorandil, a potassium channel activator characterized by its arterial vasodilator properties, is used in the treatment of angina pectoris (Frampton, Buckley, and Fitton Citation1992; Kerins Robertson, and Robertson Citation2001). It is subjected to hepatic first-pass metabolism following oral administration with systemic bioavailability of about 75% (Frydman Citation1992). Because of its short elimination half-life (1 hr), the drug has to be given frequently at 10 to 20 mg twice daily (Frampton et al. Citation1992). Thus, the conventional therapy may result in higher fluctuation in plasma concentration of the drug resulting in unwanted side effects. Thus, the development of a TTS for nicorandil that could provide the desired constant drug delivery for a predetermined period is beneficial for an effective and safe therapy of angina pectoris.
The various approaches for achieving transdermal drug delivery are reservoir-type membrane-moderated systems, adhesive diffusion-controlled systems, matrix diffusion-controlled systems, and microreservoir systems. Of these, the reservoir-type membrane-moderated TTS is considered advantageous in providing desired plasma concentration of the drug for the predetermined time with minimal fluctuations. Thus, the broad objective of the present study was to design a membrane-moderated TTS of nicorandil. In this context, Sato et al. (Citation1989, Citation1991) reported that nicorandil is a potential drug candidate for formulation as TTS after predicting its permeation across human skin based on its in vitro permeation through animal skin model. In addition to these preliminary reports, only one report existed in the literature on the development of a monolithic TTS for nicorandil (Tipre and Vavia Citation2002). Based on this information, studies were carried out with a broad objective of developing a membrane-moderated TTS using terpenes as penetration enhancers.
Earlier it was reported that hydroxypropyl methylcellulose (HPMC) gel drug reservoir prepared with 70%v/v ethanol-water solvent system containing 6%w/w of limonene was effective in promoting the in vitro transdermal delivery of nicorandil (Al-Saidan et al. Citation2004). Further studies were carried out to find the penetration enhancing effect of carvone and nerodilol on the in vitro permeation of nicorandil (Krishnaiah et al. Citation2004). The flux of nicorandil across rat abdominal skin was 365.6 ± 8.7 μg/ cm2 · h from the HPMC gel reservoir containing 12%w/w of carvone. This was 3.9 times the required flux for producing the desired plasma concentration for the predetermined period in humans. The required flux was calculated and shown as 94.7 μg/cm2 · h as per the procedure described earlier (Al-Saidan et al. Citation2004). In the light of these encouraging results, further studies were carried out to prepare and evaluate the carvone-based membrane-moderated TTS for its ability in providing the desired in vivo controlled release in humans.
In the present investigation, the in vitro permeation of nicorandil across rat abdominal skin, EVA 2825 membrane, (ethylene vinyl acetate copolymer with 28% vinyl acetate), adhesive-coated EVA 2825 membrane, and a composite of adhesive-coated EVA 2825 membrane and rat skin was studied from a carvone-based HPMC gel drug reservoir system to investigate their influence on the desired flux of nicorandil. Based on these results, a carvone-based membrane-moderated TTS of nicorandil were prepared. Further, the in vivo release of nicorandil on dermal application of carvone-based TTS was studied in human volunteers to find its ability in providing the desired plasma concentration of the drug for the predetermined time period.
MATERIALS AND METHODS
Nicorandil and carvone were obtained from M/s. Aarti Drugs Ltd., Mumbai, India, and M/s. Merck-Schuchardt, Germany, respectively. Ethylene vinyl acetate copolymer beads with 28% vinyl acetate (EVA 2825) were gift samples from M/s. National Organic Chemical Industries Limited (NOCIL), Mumbai, India. The water-based pressure-sensitive acrylic adhesive emulsion (Tackwhite A 4Med®) was a gift sample from M/s. Ichemco, Italy. Hydroxypropyl methylcellulose (HPMC) was a gift sample from M/s. Dr. Reddy's Laboratories Pvt. Ltd., Hyderabad, India, and was of USP/NF quality. Acetonitrile (HPLC grade) and water (HPLC), ethanol, potassium dihydrogen orthophosphate, ethyl acetate, sodium hydroxide, ammonia and glacial acetic acid (all AR grade) were obtained from M/s. Qualigens Fine Chemicals, Mumbai, India.
Preparation of Carvone-Based HMPC Gel Drug Reservoir
The HPMC was dispersed in 70%v/v ethanol-water to form a gel. Nicorandil (4%w/w) and carvone (12%w/w) were added to HMPC gel and mixed well for complete dissolution or dispersion. The drug reservoir systems, so prepared, were left overnight at room temperature (25°–28°C). The drug content of the reservoir system was determined by high performance liquid chromatography (HPLC) method.
HPLC Analysis of Nicorandil
The quantitative determination of nicorandil was performed by HPLC. A gradient HPLC (Shimadzu HPLC Class VP series) with two LC-10AT VP pumps, a variable wave length programmable UV/VIS Detector SPD-10A VP, a CTO-10AS VP column oven (Shimadzu), an SCL-10A VP system controller (Shimadzu), a disposable guard column LC-18 (Pelliguard™, LC-18, 2 cm, Supelco, Bellefonte, PA, USA) and a RP C-18 column (250 × 4.6 mm I.D., particle size 5 μm; YMC, Wilmington, NC, USA) were used.
The mobile phase consisted of acetonitrile and 0.02M phosphate buffer. The mobile phase components were filtered before use through 0.45-μm membrane filter and pumped in the ratio of 38:62 (acetonitrile:0.02M potassium dihydrogen orthophosphate) from the respective solvent reservoirs. The flow rate of the mobile phase was maintained at 0.8 ml/min, and the column temperature was maintained at 40°C. A series of drug solutions with varying concentration of nicorandil ranging from 0.2 to 20 μg/ml were prepared and injected into the HPLC column. The column pressure varied from 85 to 90 kgf/cm2. The eluent was detected by ultraviolet detector at 254 nm, and the data were acquired, stored, and analyzed with the software Class-VP series version 5.03 (Shimadzu). A good linear relationship was observed between the peak area of nicorandil and its concentration with a high correlation coefficient (r=0.9999). The method was found to be precise (intra- and interday variation was found to be less than 2.5%) and accurate (mean recovery 99.9%). The standard curve, constructed as described above, was used for estimating nicorandil in either permeate samples or carvone-based HPMC gel drug reservoir.
Quantitative Determination of Nicorandil
The content uniformity of nicorandil in carvone-based HPMC gel drug reservoir was estimated before preparing the TTS. First, 1g of the HPMC gel formulation was accurately weighed, placed in 100-ml volumetric flask containing 30 ml of mobile phase, stirred for 30 min, and made up to volume. The resultant mixture was filtered through a 0.45-μm membrane filter and injected into the HPLC system. The amount of nicorandil was estimated from the standard curve as described above.
Preparation of EVA 2825 Membranes
EVA 2825 copolymer membranes (vinyl acetate content 28%w/w) were prepared for their intended use as rate controlling membranes in the preparation of the proposed carvone-based membrane-moderated TTS of nicorandil. The membranes were prepared by solvent casting using a glass substrate technique. “Membrane-casting apparatus,” fabricated in our laboratory, was utilized for the preparation of the membranes. Briefly, the procedure involved pouring 4%w/v polymer (EVA) solution onto a glass frame (100 cm2) and allowing the solvent (chloroform) to evaporate slowly. The thickness of the resultant membrane was measured randomly at 10 points using a micrometer (M/s. Blue Steel Engineers Pvt. Ltd., Mumbai, India) to test thickness uniformity.
Application of Adhesive Coat
A pressure-sensitive adhesive emulsion, Tackwhite a 4Med®, was applied uniformly over the EVA 2825 membrane with the help of a glass rod and allowed to dry in the air at room temperature (25–28°C). The thickness of the EVA 2825 membranes and adhesive-coated EVA 2825 membrane was measured randomly at 10 points using a micrometer (M/s. Blue Steel Engineers).
Preparation of Rat Abdominal Skin
In the present study, rat abdominal skin was used as a skin model. The rats used were male albino rats (150–200 g) and obtained from M/s Ghosh Enterprises, Kolkota, India. They had a free access to food and water until used for the study. The care of the rats was in accordance with the institutional guidelines. The rats were euthanized using carbon dioxide asphyxiation before the experiments. The dorsal hair was removed with a clipper and full thickness skin was surgically removed from each rat. The rat abdominal skin was prepared by a heat separation technique (Zhao and Singh Citation1999). The entire abdominal skin was soaked in water at 60°C for 60 sec, followed by careful removal of the epidermis (abdominal skin). The rat abdominal skin, so prepared, was washed with water and examined for physical damage by using magnifying lens. The epidermis that was free from physical damage was used for in vitro permeation studies.
In Vitro Permeation Studies
Modified Keshary-Chien diffusion cells (Keshary and Chien Citation1984) were used in the in vitro permeation studies. The rat abdominal skin, EVA 2825 membrane, adhesive-coated EVA 2825 membrane or composite of adhesive-coated EVA 2825 membrane and rat abdominal skin was mounted between the two compartments of the vertical diffusion cells. The effective diffusion area was 6.6 cm2. The volume of receiver compartment was 35 ml. Then 2g of carvone-based drug reservoir containing 80 mg of nicorandil were added to the donor cell. The solvent system (70%v/v ethanol-water) was added to the receiver cell. The cells were placed on a magnetic stirrer with heater (M/s Remi Equipments, Mumbai, India) and temperature maintained at 37± 0.5°C. The contents in the receiver compartment were stirred with the help of a magnetic bar at 500 rpm. At predetermined times (1, 2, 4, 8, 12, 18, and 24 hr), permeate samples (0.5 ml) were withdrawn from the receiver compartment and replaced with an equivalent amount of drug-free solvent (70%v/v ethanol-water) to maintain a constant volume. The permeate samples were assayed for nicorandil by HPLC method.
In permeation studies with the adhesive-coated EVA 2825 membrane, the coated surface of the membrane was facing the receiver compartment. During the in vitro permeation studies with composite of adhesive-coated EVA 2825 membrane and rat skin composite, the coated surface of EVA 2825 membrane was stuck to the stratum corneum side of rat skin and mounted between two compartments of diffusion cell with uncoated surface of EVA 2825 membrane facing donor compartment.
Preparation of Carvone-Based TTS
The carvone-based membrane-moderated TTS of nicorandil was prepared by sandwiching HPMC gel drug reservoir between a drug-impermeable backing laminate (3M™ Scotchpak™ 9732, a polyester film laminate with ethylene vinyl acetate heat-sealable layer) and a rate-controlling EVA 2825 membrane coated with a water-based pressure-sensitive acrylic adhesive emulsion (Tackwhite A 4Med®). The drug reservoir system consisted of 4%w/w of nicorandil and a penetration enhancer (12%w/w of carvone) in 2%w/w of HPMC gel prepared with 70%v/v ethanol-water solvent system. The drug reservoir formulation was based on our earlier study (Krishnaiah et al. Citation2004). To ensure intimate contact of the TTS to the skin, Tackwhite A 4Med® adhesive emulsion was coated onto the rate-controlling EVA 2825 membrane and allowed to dry completely. A release liner (3M™ Scotchpak™ 1022, a polyester film coated with fluoropolymer) was pressed over the adhesive-coated surface of EVA 2825 membrane. Then 2g of carvone-based HPMC gel drug reservoir containing 80 mg of nicorandil (4%w/w) and 12%w/w of carvone were placed over the uncoated surface of adhesive-coated EVA 2825-release liner composite, which in turn was placed on a slightly grooved surface, and then covered with a backing laminate. The resultant sandwich was heat-sealed to form a circle-shaped TTS (20 cm2) with the drug reservoir in the center, and trimmed. Then, each TTS was kept in a sealed aluminum pouch to minimize the loss of solvent (ethanol).
In Vivo Evaluation in Human Volunteers
The study was conducted at M/s. Sipra Labs Pvt. Ltd., Hyderabad, India. The protocol of the study in human volunteers complied with the recommendation of the Declaration of Helsinki and was approved by the Institutional Ethics Committee. A total of 6 healthy male volunteers (55–60 kg, age 25–30 years) participated in the study, and all were nonsmokers and nonalcoholics. The biochemical examination of the volunteers revealed normal function of the kidney and liver. The nature and purpose of the study were fully explained to them. An informed written consent was obtained from every subject. The volunteers had a freedom to withdraw from the study at their discretion. None of the volunteers was on drug treatment 1 week prior to the participation of the study. The volunteers were divided into two groups (group I and group II), and a cross-over study was carried out. An immediate-release tablet dosage form containing 5 mg of nicorandil, chosen as a reference formulation, was administered to 3 volunteers (group I). The volunteers (group I) received the tablet formulation on empty stomachs with 240 ml of water. Group II (n=3) volunteers applied carvone-based TTS of nicorandil (20 cm2) to the anterior surface of their forearm near the elbow. Standard breakfast was served 2 hr after the study. After a washout period of 10 days, group I volunteers applied carvone-based TTS of nicorandil and group II received the reference formulation (immediate tablet dosage form). The volunteers had freedom to remove the TTS during the study (24 hr) at any sign of irritation at the application site. Blood samples were collected from the volunteer's cubical vein of the forearm via a hypodermic syringe (rinsed with dilute heparin solution) over a period of 24 hrs (0, 0.25, 0.5, 1, 1.5, 2, 4, 6, 8, 10, 12, 18, and 24 hr). The blood samples were mixed well, immediately centrifuged at 5,000 rpm, plasma separated, and stored at -40°C until analysis by HPLC.
HPLC Analysis
The quantitative determination of nicorandil in human plasma was performed by HPLC using the equipment described above. An aliquot (0.5 ml) of plasma sample was measured into a glass tube with a teflon-lined cap, followed by the addition of 0.2 ml of 0.1M sodium hydroxide and 2 ml of ethyl acetate. The mixture was vortexed for 5 min and centrifuged for 10 min at 3,000 rpm. The ethyl acetate extract (organic layer) was transferred to glass tube with a Teflon-lined cap. The aqueous portion was extracted again with another 2 ml of ethyl acetate and centrifuged. The second portion of the ethyl acetate extract was added to the first portion of ethyl acetate extract and evaporated to dryness under vacuum. The residue was reconstituted with 0.2 ml of mobile phase, and 20 μl of the resultant solution was injected into reverse phase C-18 column through which the mobile phase components (acetonitrile and 0.03M ammonium acetate buffer in the ratio of 26:74) were pumped at a flow rate of 0.8 ml/min. This yielded a column back pressure of 85–90 kgf/cm2. The eluents were monitored at 254 nm; the data acquired, stored, and analyzed with the software Class-VP series version 5.03 (Shimadzu).
The peak area of nicorandil was determined and then used to find the plasma concentration of nicorandil from the regression equation obtained after constructing the calibration curve. The calibration curve was obtained by spiking drug-free plasma with varying amount of nicorandil (5–200 ng/0.5 ml), treating the plasma samples as described above, and estimating the peak areas. A good linear relationship was observed between the plasma concentration of nicorandil and the peak area of nicorandil with a high correlation coefficient (r=0.9995) in the range of 10–200 ng/0.5 ml. However, the lower detection limit was found to be 5 ng/0.5 ml. The method was found to be precise (intra- and interday variation was less than 4%) and accurate (mean recovery 97.6%).
Data Analysis
The in vitro permeation parameters such as flux and permeability coefficient were calculated as described earlier (Al-Saidan et al. Citation2004). The plasma concentration of nicorandil at different time intervals was subjected to pharmacokinetic analysis to calculate various parameters such as maximum plasma concentration (Cmax), time to reach maximum concentration (Tmax), and area under the curve (AUC0-∝). The values of Cmax and Tmax were directly read from the arithmetic plot of time versus plasma concentration of nicorandil. The area under the curve of time versus plasma concentration of nicorandil (AUC0-∝) was calculated by using trapezoidal rule. Initially AUC0-t was calculated using trapezoidal rule and AUC0-∝ was obtained using the following equation:
where c is the plasma concentration of nicorandil at the last time-point t, and ke is the elimination rate constant. The elimination rate constant (ke) was calculated by subjecting the last three points of the semilogarithmic plot of time versus plasma concentration of nicorandil to regression analysis. The relative bioavailability of nicorandil from carvone-based TTS of nicorandil (dose 80 mg) when compared with reference formulation (dose 5 mg) was calculated by dividing its AUC0-∝ with that of immediate-release tablet dosage form (reference formulation) after applying dose correction.
Statistical Analysis
The statistical significance of the observed difference in the permeation of nicorandil across rat abdominal skin, EVA 2825 membrane, adhesive-coated EVA 2825 membrane, and adhesive-coated EVA 2825 membrane-rat skin composite was tested by analysis of variance (ANOVA) and Duncan's multiple range test with the help of Statistica™ computer program (Release 4.5, StatSoft Inc., 1993). The variance in the values of pharmacokinetic parameters was tested for equality of variance; on acceptance of the hypothesis, paired t-test was used to test the statistical significance of the observed difference in pharmacokinetic parameters of nicorandil after the application of carvone-based TTS and immediate-release tablet dosage form. Also, t-test with unequal variances was used to test the significance. In all the cases, a value of p<0.05 was considered statistically significant.
RESULTS AND DISCUSSION
To prepare a membrane-moderated TTS, the HMPC gel drug reservoir is sandwiched between a drug-impermeable backing laminate and a rate-controlling polymeric membrane. The rate-controlling membranes can be either a microporous or a nonporous polymer. Several polymers used as rate-controlling membranes are ethylene vinyl acetate copolymers, ethylene vinyl alcohol copolymers, and poly(2-hydroxyethylmethacrylate) polymers. Of these, ethylene vinyl acetate copolymer with 28% vinyl acetate content (EVA 2825) was chosen as the rate-controlling membrane because of its excellent film-forming properties, good water-vapor transmission, and appreciable tensile strength (Technical Brochure on EVA copolymers: nature and properties, supplied by M/s. National Organic Chemical Industries Limited, Mumbai, India). Also the selection of EVA 2825 as rate-controlling membrane was based on our earlier reports (Krishnaiah et al. Citation2002; Citation2003).
A pressure-sensitive adhesive coat on the rate-controlling membrane (EVA 2825) is necessary to provide intimate contact of the membrane-moderated TTS to the skin so that drug permeation takes place through skin from the drug reservoir system. Three types of pressure-sensitive adhesives are commonly used in the design of TTS: polyisobutylenes, polysiloxanes, and polyacrylic copolymers (Musolf Citation1987). The acrylic polymers gained much commercial acceptance. Hence, a commercially available water-based acrylic adhesive emulsion (Tackwhite A 4Med®) was chosen as a pressure-sensitive adhesive in the preparation of membrane-moderated TTS of nicorandil. The selection of Tackwhite A 4Med® pressure-sensitive adhesive also is based on our earlier report involving the study on three commercially available pressure-sensitive adhesives (Krishnaiah et al. Citation2002; Citation2003). But the coat of pressure-sensitive adhesive (Tackwhite A 4Med®) applied over the rate-controlling EVA 2825 membrane offers its own resistance to the permeation of nicorandil. Hence, the influence of the chosen rate-controlling EVA 2825 membrane and pressure-sensitive adhesive coat on the in vitro permeation of nicorandil from carvone-based HPMC gel drug reservoir system was studied before the preparation and evaluation of carvone-based TTS of nicorandil.
Preparation of EVA 2825 and Adhesive-Coated EVA 2825 Membranes
In addition to the physicochemical properties, the permeability characteristics of EVA 2825 membrane also depend on the method of preparation of the membranes. Thus, there are two widely used methods for preparing EVA copolymer membranes: hot-melt extrusion and solvent casting. In the present study, we planned to prepare EVA 2825 membranes by solvent casting technique wherein the solvent also affects the permeation characteristics of the membrane. Thus, an in vitro permeation study was carried out across the EVA 2825 membranes from carvone-based HPMC gel drug reservoir system. Such a study was carried out to find the efficacy of EVA 2825 membrane to act as rate controlling membrane.
In the present study, EVA 2825 copolymer membranes with 28% vinyl acetate content were prepared and evaluated for uniformity of thickness and permeability characteristics from carvone-based HPMC gel drug reservoir system. The thickness of the EVA 2825 membranes was 24.4± 0.7 μm. The low standard deviation (0.7 μm) around mean value of 24.4 μm indicates uniformity of thickness of the prepared EVA 2825 membrane. The water-based acrylic adhesive emulsion, Tackwhite A 4Med®, was applied with a glass rod and allowed to dry. On application of the adhesive coat, the thickness of the coated membrane was found to be 42.2± 0.9 μm. This shows that the thickness applied adhesive coat was 17.8 μm.
Influence on In Vitro Permeation of Nicorandil
The cumulative amount of nicorandil permeated from HPMC gel containing 4% of drug (nicorandil) and 12%w/w of carvone across EVA 2825 membrane (thickness = 24.4 ± 0.7 μm), adhesive-coated EVA 2825 membrane, and composite of adhesive-coated EVA 2825 membrane and rat skin is shown in . The amount of nicorandil permeated at the end of 24 hr (Q24) across EVA 2825 membrane from carvone-based drug reservoir was 5182.8± 199.1 μg/cm2. On application of the adhesive coat, it decreased to 4402.0± 84.7 μg/cm2 indicating that the pressure-sensitive adhesive coat offered its own resistance to the permeation of nicorandil. The value of Q24 further decreased to 3614.8± 135.6 μg/cm2 on application of the adhesive-coated EVA 2825 to rat abdominal skin also (composite of adhesive-coated EVA 2825 membrane and rat skin composite). This indicates that the rat skin also acted as barrier to the permeation of nicorandil.
FIG. 1 Mean (± S.D.) amount of nicorandil permeated from carvone-based HPMC gel drug reservoir across rat abdominal skin, EVA 2825 membrane, adhesive-coated EVA 2825, and adhesive-coated EVA 2825-rat skin composite (n=3).
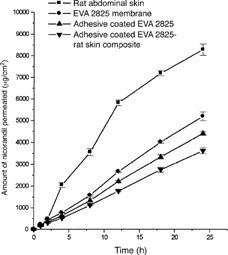
The flux of nicorandil with 12%w/w of carvone in the drug reservoir across the rat abdominal skin was 365.6± 8.7 μg/cm2·h wherein the flux obtained across the EVA 2825 membrane was 219.1± 6.2 μg/ cm2·h () indicating that the EVA 2825 membrane controlled the permeation of nicorandil. The permeability coefficient (kp) across EVA 2825 membrane also decreased significantly when compared with that obtained from rat abdominal skin. The results of the in vitro permeation study with EVA 2825 membrane indicated was acting as a rate-controlling membrane for the controlled delivery of nicorandil from HPMC drug reservoir system containing carvone as penetration enhancer.
TABLE 1 Mean (±S.D.) in vitro permeation parameters of nicorandil from carvone-based HPMC gel drug reservoir across rat abdominal skin, EVA 2825 membrane, adhesive-coated EVA 2825 membrane, and a composite of adhesive-coated EVA 2825 membrane and rat abdominal skin
The flux of nicorandil across EVA 2825 membrane further decreased to 185.3± 3.9 μg/cm2.h when an adhesive coat of (coat thickness 17.8 μ m) was applied over EVA 2825 membrane. When the adhesive-coated EVA 2825 membrane was stuck to the rat abdominal skin (composite of adhesive-coated EVA 2825 membrane and rat skin), the flux of nicorandil further decreased to 151.8 ± 5.6 μg/cm2·h indicating that rat abdominal skin further controlled the transdermal delivery of the drug. The permeability coefficient (kp) and Q24 of nicorandil across the composite of adhesive-coated EVA2825 membrane and rat skin also decreased significantly when compared with that obtained from adhesive-coated EVA 2825 membrane.
Thus, the results of the in vitro permeation studies from carvone-based HPMC gel drug reservoir across EVA 2825 membrane, adhesive-coated EVA 2825 membrane and composite of adhesive-coated EVA 2825 membrane and rat skin showed that EVA 2825 was effective as rate-controlling membrane. The results further indicated that the adhesive-coated EVA 2825 membrane and rat skin also were controlling the transdermal delivery of nicorandil. However, this flux of nicorandil across the composite of adhesive-coated EVA 2825 and rat skin was 1.6 times the required flux (94.7 μg/cm2·h). Still, the in vitro permeation data obtained using animal skin model (rat abdominal skin) could not be extrapolated to human skin to conclude usefulness of the proposed carvone-based membrane-moderated TTS of nicorandil. But the studies carried out so far provided enough evidence to study the in vivo evaluation of carvone-based TTS of nicorandil in human volunteers. The goal was to investigate its ability in providing the desired plasma concentration of the drug for the predetermined period of time.
Area of Carvone-Based TTS
The required flux of nicorandil (94.7 μg/cm2·h) across rat abdominal skin, for optimizing the formulation of TTS, was calculated for an exposed area of 6.6 cm2 (Al-Saidan et al. Citation2004). However, the permeability of rat skin was reported to be about 3 times more than that of human skin (Diez et al. Citation1991). Also, the oral bioavailability of nicorandil is only 75% (Frydman Citation1992). Hence, on application of the proposed TTS to human skin, the extent of nicorandil flux obtained across rat abdominal skin may not be achieved in humans with the exposed transdermal area of 6.6 cm2 used in the in vitro permeation studies. Also, the in vitro permeation studies through rat abdominal skin-EVA 2825 membrane composite showed only 1.6 times of the required flux, whereas the desired plasma concentration of the drug may not be obtained because of the possible resistance of the human stratum corneum. In view all these factors, the required TTS area was calculated, as detailed below.
The flux of nicorandil across the composite of adhesive-coated EVA 2825 membrane and rat skin from carvone-based HPMC gel drug reservoir (containing 12%w/w of carvone as penetration enhancer) was 151.8± 5.6 μg/cm2·h. Based on this flux, the area of TTS required for providing a steady-state concentration of 15 ng/ml for 24 hr was calculated from the daily transdermal dose of nicorandil, which in turn was calculated based on the bioavailability and daily oral dose of nicorandil in humans.
The total daily maximal dose of nicorandil is 20 mg, and considering the mean oral bioavailability as 75% (Frydman Citation1992), the calculated daily transdermal dose (bioavailability x daily oral dose of nicorandil) was 15 mg. The area of TTS required to maintain a steady-state concentration of 15 ng/ml for 24 hr from the membrane-moderated TTS in humans was calculated by using the following relationship (Diez et al. Citation1991) based on the observed mean flux (151.8 μg/cm2·h) across adhesive-coated EVA 2825 membrane/rat skin composite:
where t is the time period for which the steady state concentration is to be maintained (24 hr); J is the observed mean flux (151.8 μg/cm2.h) using rat abdominal skin as a skin model and 3 is the correction factor to be applied for predicting the permeability of the drug in humans based on the permeability flux obtained from rat abdominal skin (Diez et al. Citation1991).
Thus, the area of TTS required to provide a steady-state concentration of 15 ng/ml for 24 hr from a transdermal dose of 15 mg, based on the flux of nicorandil (151.8 μg/cm2 · h) obtained from rat abdominal skin, was 12.4 cm2. For the sake of convenience, we planned to prepare a TTS with an area of 20 cm2. The in vitro permeation studies have warranted the incorporation of 80 mg of nicorandil (4%w/w) in the HPMC gel drug reservoir to maintain a high thermodynamic activity in HPMC gel reservoir (Al-Saidan et al. Citation2004). Thus, a total of 80 mg of nicorandil was incorporated in the HPMC drug reservoir.
In Vivo Release of Nicorandil in Human Volunteers
The carvone-based TTS of nicorandil, before applying to volunteers, was intact without any leakage and contained 97.9± 3.8% of nicorandil indicating the content uniformity. The HPLC chromatograms with respect to the estimation of plasma concentration of nicorandil did not show any other interfering peaks indicating that other plasma components did not interfere with the HPLC estimation of nicorandil in human plasma. The mean plasma concentration of nicorandil at different time intervals following the dermal application of carvone-based TTS or oral administration of immediates release tablet dosage form shown in . On dermal application of TTS, the plasma concentration of nicorandil gradually increased and attained steady-state concentration with a lag period of 3.4 hr. The average steady-state plasma concentration of nicorandil obtained with TTS was 20.5 ng/ml, which was maintained at 24 hr also. The trend in the decline of plasma concentration indicates that the steady-state concentration of nicorandil would be maintained beyond 24 hr also with respect to the TTS. The occurrence of a higher steady-state concentration of nicorandil, well within the limits of reported levels (Frydman Citation1992), beyond 24 hr is most probably due to the incorporation of a higher amount of the drug incorporated in the drug reservoir (80 mg in place of the calculated transdermal dose of 15 mg; the in vitro permeation studies have warranted increasing the concentration of nicorandil in HPMC drug reservoir to provide a higher thermodynamic activity). However, the steady-state concentration of nicorandil, observed in the present study, was within the range of reported (Frydman Citation1992) steady-state concentration (15 to 30 ng/ml).
FIG. 2 Mean (± S.D.) plasma concentration of nicorandil following the oral administration of immediate release tablet (dose 5 mg) or application of carvone-based TTS (dose 80 mg) in human volunteers (n=6).
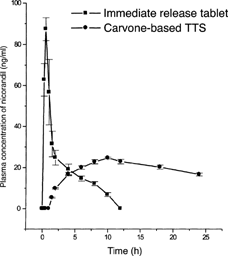
The pharmacokinetic parameters of nicorandil following oral administration of immediate-release tablet dosage form or application of carvone-based TTS are given in . The pharmacokinetic parameters of nicorandil after the application of TTS were significantly different from those obtained with immediate-release tablet dosage form. It took 9.8± 0.4 hr (Tmax) to reach maximum concentration of 24.6± 0.7 ng/ml (Cmax) from TTS. However, on oral administration of nicorandil immediate-release tablet, the Cmax (89.0± 5.3 ng/ml) of the drug reached within 0.6± 0.1 hr and declined rapidly.
TABLE 2 Mean (±S.D.) pharmacokinetic parameters of nicorandil following oral administration of immediate-release tablet (5 mg) or dermal application of a carvone-based TTS (dose 80 mg) in human volunteers (n=6)
The intersubject variation in plasma concentration of nicorandil after oral administration of immediate-release tablet was high (percent Coefficient of Variation (CV) 13.7–28.2). The low variation (percent CV 1.5–4.2) in peak plasma levels following dermal application of nicorandil TTS could be accounted for by uniformity in the transdermal permeation of the drug, which possibly is the same for all volunteers. The intersubject variation in plasma levels observed in the volunteers receiving immediate-release tablet could be due to the high variation in gastric emptying and gastro intestinal absorption etiology of individual volunteers (Hunt and Spurrell Citation1951; Hunt Citation1959).
The increase in the area under the curve (AUC0-∞) of nicorandil with the carvone-based TTS was significantly (P < 0.001) higher when compared with oral administration of immediate-release dosage form and indicated the improved bioavailability on transdermal delivery of the drug (). This was evident from the increase in the relative bioavailability of nicorandil from TTS when compared with oral immediate-release tablet dosage form. The TTS enhanced the bioavailability of nicorandil by about 0.25 times (mean relative bioavailability 24.7%) with reference to an immediate release tablet dosage form ().
Assuming the reported mean oral bioavailability of nicorandil as 75%, the TTS used in the present study provided 93.5% of bioavailability (Frydman Citation1992). This increased bioavailability might be due to the elimination of hepatic first-pass metabolism on transdermal delivery of nicorandil. Thus, the carvone-based membrane-moderated TTS, prepared in the present study, provided prolonged steady-state plasma concentration of nicorandil with minimal fluctuations and improved bioavailability.
The skin irritation and sensitization due to the application of TTS also were assessed, because severe skin irritation may affect the efficacy or safety of the TTS formulation. The components of TTS either alone or in conjunction with the drug substance may cause these reactions. Hence, the volunteers were asked to report any sign of skin irritation during the 24 hr of study. Also, the application sites of the volunteers were observed for signs of skin irritation/sensitization. There were no signs of skin irritation after the application of the TTS for 1 day. None of the volunteers reported any skin irritation indicating that the carvone-based TTS, developed in the present study, was well tolerated for 1 day. However, the possibility of skin irritancy on long-term usage of TTS needs to be studied. The TTS showed 90% adhesion as there was “no lifting” of it from the skin. Thus, the results of the in vivo evaluation of the carvone-based membrane-moderated TTS in human volunteers prompt further studies in patient volunteers suffering from angina pectoris in providing an effective and safe therapy.
CONCLUSIONS
The in vitro permeation studies from a carvone-based HPMC gel drug reservoir across EVA 2825, adhesive coated EVA 2835, and adhesive-coated EVA 2825-rat skin composite in comparison with control study (rat skin alone) showed that EVA 2825 was effective in acting as rate-controlling membrane for nicorandil. The in vivo evaluation in human volunteers showed that the carvone-based membrane-moderated TTS provided controlled release of nicorandil for ∼24 hr with improved bioavailability.
The authors acknowledge M/s. Nocil, Mumbai, India, M/s 3M drug delivery systems, USA, and M/s Ichemco, Italy, for the gift samples of EVA copolymers, release liner (3M™ Scotchpak™ 1022), backing membrane (3M™ Scotchpak™ 9732), and Tackwhite A 4Med®, respectively. The authors also acknowledge M/s Dr. Reddy's Laboratories Pvt. Ltd., Hyderabad, India, for the gift sample of HPMC.
REFERENCES
- Al-Saidan S. M., Krishnaiah Y. S. R., Chandrasekhar D. V., Lalla J. K., Rama B., Jayaram B., Bhaskar P. Formulation of an HPMC gel drug reservoir system with ethanol-water as a solvent system and limonene as a penetration enhancer for enhancing in vitro transdermal delivery of nicorandil. Skin Pharmacol. Physiol. 2004; 17: 310–320, [PUBMED], [INFOTRIEVE], [CSA]
- Diez I., Colom H., Moreno J., Obach R., Peraire C., Domenech J. A comparative in vitro study of transdermal absorption of a series of calcium channel antagonists. J. Pharm. Sci. 1991; 80: 931–934, [PUBMED], [INFOTRIEVE], [CSA]
- El-Kattan A. F., Asbill C. S., Kim N., Michniak B. B. The effects of terpene enhancers on the percutaneous permeation of drugs with different lipophilicities. Int. J. Pharm. 2001; 215: 229–240, [PUBMED], [INFOTRIEVE], [CROSSREF], [CSA]
- Frampton J., Buckley M. M., Fitton A. Nicorandil: a review of its pharmacology and therapeutic efficacy in angina pectoris. Drugs 1992; 44: 625–655, [PUBMED], [INFOTRIEVE], [CSA]
- Frydman A. Pharmacokinetic profile of nicorandil in humans: an overview. J. Cardiovasc. Pharmacol. 1992; 20(Suppl 3)S34–S44, [PUBMED], [INFOTRIEVE], [CSA]
- Gao S., Singh J. In vitro percutaneous absorption enhancement of lipophilic drug tamoxifen by terpenes. J. Control. Rel. 1998; 51: 193–199, [CROSSREF], [CSA]
- Hunt J. N. Gastric emptying and secretion in man. Physiol. Rev. 1959; 39: 491–533, [PUBMED], [INFOTRIEVE], [CSA]
- Hunt J. N., Spurrell W. R. The pattern of emptying of the human stomach. J. Physiol. 1951; 113: 157–168, [PUBMED], [INFOTRIEVE], [CSA]
- Kerins D. M., Robertson R. M., Robertson D. Drugs used for the treatment of myocardial ischemia. Goodman & Gilman's The Pharmacological Basis of Therapeutics, 10th ed., J. G. Hardman, L. E. Limbird. McGraw Hill Medical Publishing Division, New York 2001; 844
- Keshary P. R., Chien Y. W. Mechanism of transdermal controlled nitroglycerin administration. Part 2. Assessment of rate controlling steps. Drug Dev. Ind. Pharm. 1984; 10: 1663–1699, [CSA]
- Krishnaiah Y. S. R., Satyanarayana V., Bhaskar P. Influence of nerodilol on the bioavailability of nicardipine hydrochloride from membrane-moderated transdermal therapeutic systems in human volunteers. Int. J. Pharm. 2002; 247: 91–102, [PUBMED], [INFOTRIEVE], [CROSSREF], [CSA]
- Krishnaiah Y. S. R., Satyanarayana V., Bhaskar P. Influence of menthol and pressure sensitive adhesives on the in vivo performance of membrane-moderated transdermal therapeutic system of nicardipine hydrochloride in human volunteers. Eur. J. Pharm. Biopharm. 2003; 55: 329–337, [PUBMED], [INFOTRIEVE], [CROSSREF], [CSA]
- Krishnaiah Y. S. R., Al-Saidan S. M., Chandrasekhar D. V., Lalla J. K. Effect of nerodilol and carvone on the in vitro transdermal delivery of nicorandil. AAPSJ. 2004; 6(4), abstract W4263[CSA]
- Musolf M. C. Pressure-sensitive adhesives: science and engineering. Transdermal Controlled Systemic Medications, Y. W. Chien. Marcel Dekker, New York 1987; 93–112
- Prausnitz M. R., Mitragotri S., Langer R. Current status and future potential of transdermal drug delivery. Nat. Rev. Drug Discov 2004; 3: 115–124, [PUBMED], [INFOTRIEVE], [CROSSREF], [CSA]
- Sato K., Sugibayashi K., Morimoto Y., Omiya H., Enomoto N. Prediction of the in-vitro human skin permeability of nicorandil from animal data. J. Pharm. Pharmacol. 1989; 41: 379–383, [PUBMED], [INFOTRIEVE], [CSA]
- Sato K., Sugibayashi K., Morimoto Y. Species differences in percutaneous absorption of nicorandil. J. Pharm. Sci. 1991; 80: 104–107, [PUBMED], [INFOTRIEVE], [CSA]
- Tipre D. N., Vavia P. R. Formulation optimization and stability study of transdermal therapeutic system of nicorandil. Pharm. Dev. Technol. 2002; 7: 325–332, [PUBMED], [INFOTRIEVE], [CROSSREF], [CSA]
- Williams A. C., Barry B. W. Penetration enhancers. Adv. Drug Deliv. Rev. 2004; 56: 603–618, [PUBMED], [INFOTRIEVE], [CROSSREF], [CSA]
- Zhao K., Singh J. Mechanisms of percutaneous absorption of tamoxifen by terpenes: eugenol, D-nerodilol and menthone. J. Control. Rel. 1998; 55: 253–260, [CROSSREF], [CSA]
- Zhao K., Singh J. In vitro percutaneous absorption enhancement of propranolol hydrochloride through porcine epidermis by terpenes/ethanol. J. Control. Rel. 1999; 62: 359–366, [CROSSREF], [CSA]