Abstract
Lipospheres of carbamazepine were prepared by melt dispersion technique using Precifac® ATO 5 in the various drug-lipid ratios. The resulting free-flowing lipospheres were evaluated with respect to surface morphology, particle size distribution, encapsulation efficiency, and in vitro release behavior. The effect of druglipid ratio, the surfactant added, emulsion stabilizer, and stirring speed also were identified as the key variables affecting the formation of discrete spherical lipospheres and drug release rate. The preparation conditions were optimized by using 0.4% w/v span 20 (Hydrophilic-Lipophilic Balance, HLB=8.6) as a surfactant and 1% w/v gelatin solution as a stabilizer in presence of a high level of water.We found that the ratio of drug to lipid affects the size of the spheres. The incorporation efficiency was found to be high at all loadings. Increasing the lipid:drug ratio produced more spherical, smooth, and round lipospheres. All the prepared lipospheres exhibited slow release profiles dictating the Higuchi mode of release. We saw that the higher the sphere size and the ratio of Precifac®, the slower is in vitro drug release.
Carbamazepine (CZ) is one of the most important drugs for the therapy of psychomotor epilepsy, for to control of grand mal, and for the treatment of trigeminal neuralgia. It is now considered the primary drug for the treatment of partial and tonic-colonic seizers (Macdonald Citation1989; AHFS Drug Information Citation1990). The drug is characterized by a slow and irregular gastrointestinal absorption, due to its low water solubility (Lovrecich et al. Citation1994).
The biological half-life of CZ is in 20–40 hr during the initial period of treatment; however, after repetitive dosing it reaches only 8–12 hr (Bertilssonan and Tomson Citation1986). This lowering is attributed to the autoinduction of a microsomal enzyme system exhibited by CZ (Faigle and Feldman Citation1989). The normal frequency of dosing for conventional forms is 3–4 times daily (Porter Citation1989). This results in compliance problems and fluctuations in plasma concentrations that usually cause some intermittent side effects such as nausea, dizziness, and diplopia (Aldenkamp et al. Citation1987). The development of a controlled release form of CZ that can be administered once daily would eliminate these problems in addition to increasing the drug's potential for seizer control by permitting higher steady-state concentrations.
The encapsulation of drugs into inert matrices is the major approach used to extend drug release. Numerous carrier materials have been used in the development of extended-release systems. Among those materials, lipophilic polymers are the most popular.
Lipophilic materials have been successfully used for the preparation of extended-release tablets, granules, and microspheres (Naidoo Citation1989; Malamatoris et al. 1991; Akiyama et al. Citation1993; Miyagawa et al. Citation1996; Karasulu et al. Citation2003). The prepared formulation in most cases exhibited good and acceptable in vitro and in vivo properties. In addition lipophilic polymers are biocompatible, and biodegradable materials that neither adhere to the gastrointestinal tract nor accumulate in the gut (Gattefosse Corporation, Citation1989). The high partition coefficient exhibited by CZ suggests good percentage of drug entrapment into lipophilic microspheres.
The aim of the present study was to assess the feasibility of formulating CZ lipospheres and obtaining a suitable release pattern. We wanted to use a technique that was rapid and economical and did not use organic solvents. The effect of drug:lipid ratio, particle size of microspheres, and stirring speed were investigated.
MATERIALS AND METHODS
Carbamazepine was kindly supplied by Novartis Pharma (Cairo, Egypt). Precifac® ATO5 (cetyl palmitate) was received as a gift from Gateffosse, Saint Priest, Cedex, France. Span 20 and Tween 20 (Fluka, Buchs, Switzerland) and Poloxamer® (pluronic F-68) (BASF, Parsipanny, NJ, USA) were used as received. Gelatin (240 bloom) was kindly gifted from Arab Caps, Alexandria, Egypt. All other chemicals were commercially available products of analytical grades.
Selection of Optimum Surfactant
Preparation of CZ-loaded microspheres was based on a hot emulsion/congealing technique (Adeyeye and Price Citation1991; Hassan et al. Citation1994). As a preliminary study, different factors were examined to help choose suitable surfactant for proper microsphere production. A number of experiments were done using different concentrations (0.2–1.0% w/v) of Span 20, Tween 20, Pluronic F-68; also various concentrations of emulsion stabilizer, gelatin, were examined ranging from 0.5–2% w/v. The optimum surfactant is the one that produces discrete microspheres with very good flow properties, good recovery, and reasonable drug loading.
Preparation of Microspheres
The drug was dispersed in a suitable weight of the molten Precifac® ATO5 (PRF) to form 1:1; 1:2; 1:3; 1:4 drug to lipid ratio. The temperature of the formed suspension was maintained in the range 62–64°C (∼10°C above the melting point of PRF). An aqueous phase containing 1% w/v gelatin and 0.4% w/v Span 20, previously heated to 60°C, was gradually added to the melt with constant stirring at 400 rpm with a mechanical stirrer (Yellow Line OST Basic, Germany) to form o/w emulsion. The temperature was maintained at 70°C for 3 min, and then ice water was added until room temperature was reached. The resultant solid spherical particles were recovered by filtration and extensively rinsed with water to remove any undesirable residuals. They were allowed to dry at room temperature for 24 hr. Triplicate experiments were made for each ratio.
Percentage Yield of Microspheres
Yield of microsphere percent w/w was calculated according to the following formula:
Particle Size and Size Distribution of Microspheres
The obtained microspheres from each ratio were sieved with a combined sieving system. They were placed on a nest of 6 sieves with apertures between the 710–125 μm. The sieves (Retsch, Haan, Germany) were mechanically shaken for 20 min. The spheres retained on each sieve were lightly pressed using a spatula to separate further loosely aggregated particles. The weight of spheres on each sieve was measured and the size distribution was calculated.
Loading Efficiency
A total of 25-mg lipophilic microspheres in each drug-lipid ratio were finely powdered in a mortar and put in a 25-ml volumetric flask and the volume was adjusted with alcohol. The mixture was shaken for 30 min. at 100 cycles per min; the solutions were filtered through 0.45 um membrane filter and then samples were measured spectrophotometrically at λ = 285 nm against a blank prepared with microspheres containing no drug (Ultrospec Z100 pro spectrophotometer, England). Corresponding concentrations were calculated from a previously constructed standard curve that was linear up to 20 μg/ml. Finally, the loading efficiency of the microspheres was determined as the ratio of the actual amount to the theoretical amount of CZ in the microspheres.
Stirring Speed
According to Arshady (Citation1990), various manufacturing parameters (apparatus design, type of stirrer, stirring speed, and rate of cooling) affect the particle size. We have only investigated the effect of stirring speed on particle formation and particle size while keeping the other parameters constant. This was exemplified by preparing the batch 1:3 CZ-PRF using different stirring speeds from 400 to 800 rpm.
Microscopic Evaluation
An optical microscope (Olympus-Cover-018) with a camera attachment (Minolta) was used to observe the shape of the prepared microspheres for each drug: lipid ratio.
Thermal Analysis
A differential scanning calorimeter (DSC) (Du Pont Instruments, UK), was used to determine the crystalline state of the formulation components before and after formation of microspheres. Samples were scanned from room temperature to 225°C at a heating rate 5°C/min. The thermograms of the prepared microspheres were compared with thermograms of pure CZ, pure Precifac, and the physical mixture in amounts equivalent to the ratios present in the microspheres.
Drug Release Studies
The dissolution of CZ from each of the prevalent sphere-size from each of the drug:Precifac ratio prepared microspheres was monitored using USP 24 apparatus II (Erweka Apparatus, Germany). Amount of the spheres equivalent to 200 mg CZ was used. The dissolution media were 900 ml of simulated gastric fluid without enzymes (pH 1.2) for the first 3 hr and then the pH was adjusted to 7.4 for the next 9 excessive hr by the addition of a certain amount of trisodium phosphate. They were maintained at 37°C ± 0.5. Polysorbate 80 (0.2%, w/w) was added to the dissolution medium to improve the wetting of the microspheres. The stirring rate was 75 rpm ± 1. Then 5-ml aliquots were withdrawn at specific time intervals and the withdrawn samples were replaced with fresh dissolution fluid. The samples were then analyzed spectrophotometrically for CZ content. Each dissolution study was carried out in triplicate. The mean of three determinations was used to calculate the drug release from each of the formulations.
Kinetic Analysis of Release Profiles
To determine the release kinetic model and mechanisms, the dissolution data for microspheres that showed slow release were analyzed. Four kinetic models including the zero-order release equation [1], first-order equation [2], Higuchi equation [3] and Hixson-Crowell cube root model [4] were applied to process the in vitro data to find the equation with the best fit (James et al. Citation1997; Wu et al. Citation2002).
Where Q is the release percentage at time (t). k1, k2, k3, and k4 are the rate constant of zero-order, first-order, Higuchi, and Hixson-Crowell models, respectively.
RESULTS AND DISCUSSION
Formation of CZ Microspheres
The preparation method applied in this study was patented by Yamamoto and Baba (Citation1960). It involves cooling-induced solidification of the lipid phase of a two-phase system. Kowarski et al. (Citation1964) and later, Drapper and Becker (Citation1966) used this method for the preparation of sustained-release sulphonamide formulations. Modifications of the two phases have led to the production of microspheres of both water-soluble and insoluble drugs (Kawata et al. Citation1986; Das and Gupta Citation1988).
Giannola et al. Citation1994, Citation1995 reported that the addition of a surfactant reduces the tendency of agglomeration and formation of irregular matrices. In addition, it increases the wet ability of the fatty material. They tried several surfactants for the preparation of valproic acid–carnauba wax microspheres. Benita et al. (Citation1986) also tried several surfactans as polysorbate 40, macrogol ester, and Poloxamer. They found that only surfactant with high HLB can improve the quality of the prepared lipid microspheres.
An emulsion stabilizer (gelatin, methyl cellulose) usually is used to prevent phase separation by increasing emulsion viscosity and stabilizing the surfactant sheath around the internal phase.
We found from the preliminary experiments that the low surfactant concentration (0.2% w/v) failed to produce stable CZ dispersion. At least 0.4% w/v span 20 was needed to yield stable homogenous CZ dispersion in the melted fat that consequently produced desirable microsphere batches. No further improvement in the quality of the produced microspheres was achieved with the increase in concentration of surfactant up to 1% w/v. We also saw that changing the concentration of gelatin from 0.5–2% w/v provided insignificant changes in emulsion stability, drug loading, or particle size.
We also found that maximum ratio of drug-to-lipid material was approximately 1:1 higher drug loading led to the insufficient coating of drug particle by lipophilic material and formation of particles that aggregated during the cooling process. This in turn produced irregular, fluffy, and fragile particles that were deemed unsuitable for our purpose.
The amount of water used for the preparation of lipospheres also influenced the characteristics of the product. Large and aggregated irregular particles were obtained when level of water used was in the range 20–40 ml. Superior quality of lipospheres, i.e., smooth, round, and discrete in nature, was obtained when 80–100 ml water was used. These results are in agreement with the work of Gohel and Amin (Citation1997).
Based on the preliminary experiments, we decided to use 0.4% w/v span 20 (HLB = 8.6) as a surfactant and 1% w/v gelatin solution as stabilizer in presence of high level of water during the course of this work.
Lipospheres Morphology
Particle Size Distribution
shows the percentage distribution of each size range in each of the four prepared batches. It is clear that the ratio of drug-to-lipid affects the size of the produced spheres. Roughly, 60% of the spheres lie in the ranges of 630–800, 500–710, 315–630, and 250–500 for ratios 1:1, 1:2, 1:3, and 1:4, respectively.
FIG. 1 Particle size distribution of carbamazepine-loaded Precifac microspheres produced at 400 rpm.
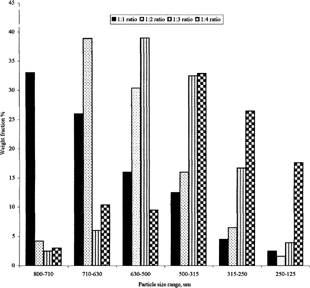
The extent of loading appears to influence the particles size distribution of microspheres. When the loading is high, the proportion of large particles formed also is high. This result was in agreement with the work done by Joseph et al. (Citation2002) who prepared piroxicam-loaded polycarbonate microspheres. Also, Kiliçarslan and Baykara (Citation2003) found that particle sizes of verapamil hydrochloride–Eudragit microspheres decreased with increasing polymer amounts and decreasing drug loading. On the other hand, Pongpaibul et al. (Citation1984) found that higher concentration of polymer produced a more viscous dispersion that formed larger droplets and consequently larger microspheres.
The incorporation efficiency of CZ was found to be good at all loadings. No marked variation in the drug amount to particle size ratio was observed. This indicates that the ratio between drug and fatty material remained practically constant regardless of the particle size distribution of the various microspheres in one batch. gives the average drug content and the incorporation efficiency at the 4 different loadings examined. The high entrapment efficiency of the drug is believed to be due to its poor aqueous solubility. No significant differences were observed in drug content with the use of the various surfactants in the preliminary study.
TABLE 1 Percentage yield values, incorporation efficiencies, and mean particle size of formulations
Stirring Speed
The effect of agitation speed on the mean size of the microspheres is exemplified by the CZ-PRF microspheres 1:3. We found that by increasing the stirring speed from 400 to 800 rpm, the average size of microspheres decreased gradually from 441 to 220 μm. The relationship between the mean size of the microspheres and the speed of agitation is not linear (). Kawashima et al. (Citation1981), using a modified aqueous dispersion method, observed a sharp decrease in average particle size for a certain speed range after which the size decreased only gradually and slowly. Babay et al. (Citation1988) also observed a similar trend when the stirring speed was increased in production of the empty Eudragit RL microspheres.
Microscopic Image
(, , , ) shows that at low drug:lipid ratio, the microspheres produced were irregular in shape. Increasing the lipid:drug ratio produced more spherical, smooth, and round microspheres.
Thermal Analysis
The thermal behavior of microparticles prepared by hot emulsion/congealing technique as well as the 1:2 CZ-to-lipid physical mixture in comparison with the thermograms of both pure CZ and PRF is illustrated in . The (DSC)-thermogram of the bulk PRF shows two adjacent endothermic peaks corresponding to melting at 48.4°C (ΔH = 137.2 J/g). The DSC trace of the pure CZ shows two endothermic peaks: one at 158.8°C, corresponding to transition from polymorph II to I and the second at 189.9°C, corresponding to melting of polymorph I. This attribute of polymorph II is in agreement with Rustichelli et al. (Citation2000) and Naima et al. (Citation2001).
FIG. 4 DSC thermograms of (a) pure CZ, (b) Pure PRF, (c) 1:2 CZ:PRF physical mixture, and (d) microsphere prepared from 1:2 CZ: PRF.
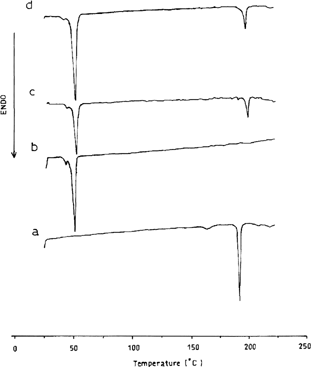
There is consensus in the literature that CZ crystallizes under three forms, at least: a trigonal (form II), a monoclinic (form III), and a high-temperature phase, previously named α by Lefebvre et al. (Citation1986) or phase I by Krahn and Mielck (Citation1989), whose structure has not yet been solved. However, this phase has been shown to crystallize in the triclinic system (Cèolin et al. 1996).
The thermogram of the physical mixture showed shifting of the two endothermic peaks of CZ to higher temperatures; in addition, the enthalpy of fusion decreased to 28.2 J/g. The thermogram of the prepared microspheres (trace d) showed disappearance of the CZ transition peak and tremendous reduction in CZ polymorph I enthalpy of fusion to 38.8 J/g. On the other hand, the enthalpy of fusion corresponding to PRF has been increased to 182.1 J/g. Both changes in the values of ΔH can be attributed to the partial solubility of CZ in the melted PRF during the preparation of the microspheres and the transition of the entire undissolved portion to polymorph I.
Release Characteristics
, , , represent the in vitro release profiles of the 4 batches. The dissolution test was conducted in simulated gastric fluid without enzymes (pH 1.2) for the first 3 hr to assess for stomach conditions. The mean gastric emptying time in average humans is 2 hr; however, lipids may delay the gastric emptying so we decided to extend the gastric release period to 3 hr. The dissolution profiles of each particle size range among each batch were monitored. One can notice that the CZ release from these lipospheres is independent of the pH of the medium.
FIG. 5 Release of carbamazepine from 1:1 carbamazepine-loaded microspheres of various sizes produced at 400 rpm.
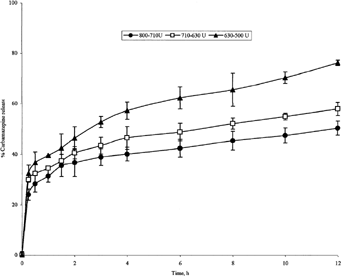
FIG. 6 Release of carbamazepine from 1:2 carbamazepine-loaded microspheres of various sizes produced at 400 rpm.
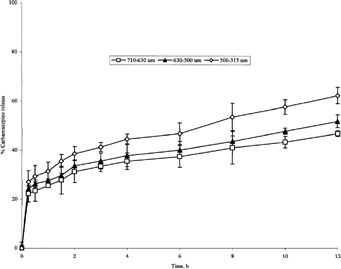
FIG. 7 Release of carbamazepine from 1:3 carbamazepine-loaded microspheres of various sizes produced at 400 rpm.
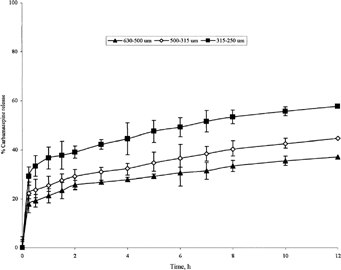
FIG. 8 Release of carbamazepine from 1:4 carbamazepine-loaded microspheres of various sizes produced at 400 rpm.
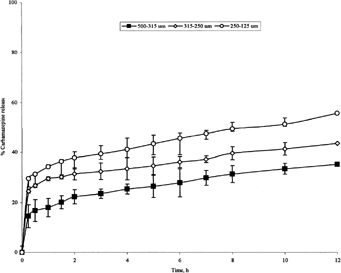
The dissolution of CZ from different particle size of different batches is shown in , , , . It is well known that sizes of lipospheres affect the release rates of the drug. As expected, smaller microspheres yielded more rapid CZ release for a given amount of drug than larger microspheres, because the combined surface area of the smaller microspheres is larger and the diffusional path is shorter (Wong et al. Citation1992).
Microspheres from 1:1 batch, in the size range of 710–800 μm, exhibited a slow release profile with only 50.4% of the drug content released after 12 hr front of 58% and 76.3% for 630–710 μm and 500–630 μm microsphere size ranges, respectively (). The same observation applies for the other 3 batches (, , ). This leads to a conclusion that the higher the sphere size, the slower is the in vitro drug release. This is in agreement with what Wong et al. (Citation1992) have reported.
Microspheres having size range 500–630 μm from batches I, II, and III exhibited different release profiles with cumulative percentages drug released after 12 hr equal to 76.3%, 51.8%, and 37.1%, respectively (, , ). In addition, lipospheres having size range 315–500 μm from batches II, III, and IV showed cumulative percentages drug released of 62.3%, 44.6%, and 35.2%, respectively (, , ). It is obvious that the ratio of the PRF in the prepared microspheres influences the rate of drug release. The higher is the ratio the slower is the drug release from the microspheres.
Kinetic Mechanism of CZ Release
After a comparative evaluation of r values (), one can see that all the formulae exhibited the highest correlation coefficient when the percentage released was plotted against the square root of time. These data dictate the Higuchi profile as the mode of drug release from fatty materials. These results are in agreement with what Sato et al. (Citation1997) and Miyagawa et at. (Citation1996) have concluded with other fatty materials.
TABLE 2 Values of correlation coefficients of different mathematical models for CZ
Other researchers (Schwartz et al. Citation1968a, Citation1968b) have reported that the kinetic data from some inert whole wax matrix tablets conformed with both first-order release and the Higuchi matrix model.
CONCLUSIONS
The results reported in this study show that reproducible extended-release CZ microspheres could be prepared using the congealable dispersion microencapsulation technique. CZ dispersed in the molten mass and congealed to form microspheres in which much of the drug does not recrystallize as the pure drug but forms a homogenous solid with the lipid material. The melt-dispersion method is simple, viable, and economical which does not imply the use of organic solvents. The method described achieves good incorporation efficiency and should be very useful for the development of extended-release microspheres of CZ and other drug with similar solubility and melting characteristics. Further studies will be done for in vivo performance of the selected promising formulation.
REFERENCES
- Adeyeye C., Price J. Development and evaluation of sustained-release ibuprofen-wax microspheres I. Effect of formulation variables on physical characteristics. Pharmaceut. Res. 1991; 8: 1377–1384, [CSA]
- AHFS Drug Information 90. G. K. McEvoy. American Society of Hospital Pharmacists, Bethesda, MD 1990; 1142–1145
- Akiyama Y., Yoshioka M., Horibe H., Hirai S., Kitamori N., Toguchi H. Novel oral controlled-release microspheres using polyglyceral esters of fatty acids. J. Control. Rel. 1993; 26: 1–10, [CROSSREF], [CSA]
- Aldenkamp A., Alpherts W., Moreland M., Ottevanger N., Van Parys J. Controlled release carbamazepine: cognitive side effects in patients with epilepsy. Epilepsia 1987; 28: 507–514, [PUBMED], [INFOTRIEVE], [CSA]
- Arshady R. Albumin microspheres and microcapsules: methodology of manufacturing techniques. J. Control. Rel 1990; 14: 111–131, [CROSSREF], [CSA]
- Babay D., Hoffman A., Benita S. Design and release kinetic pattern evaluation of indomethacin microspheres intended for oral administration. Biomaterials 1988; 9: 482–488, [PUBMED], [INFOTRIEVE], [CROSSREF], [CSA]
- Benita S., Zouai O., Benoit J. P. 5-Fluorouracil: carnauba wax microspheres for chemoembolization: an in vitro evaluation. J. Pharmaceut. Sci 1986; 75: 847–851, [CSA]
- Bertilsson L., Tomson T. Clinical pharmacokinetics and pharmacological effect of Carbamazepine and Carbamazepine-10, 11-epoxide: an update. Clin. Pharmacokin 1986; 11: 177–198, [CSA]
- Cèolin R., Toscani S., Gardette M.-F., Agafonov V., Dzyabchenko A., Bachet B. X-ray characterization of the triclinic polymorph of charpamazepine. J. Pharmaceut. Sci 1997; 86: 1062–1065, [CROSSREF], [CSA]
- Das S., Gupta B. Optimisation of controlled drug release through micropelletization. Drug Devel. Ind. Pharm 1988; 14: 1673–1697, [CSA]
- Draper E., Becker C. Some wax formulations of sulfaethylthiazidiazole produced by aqueous dispersion for prolonged release medication. J. Pharmceut. Sci. 1966; 55: 376–380, [CSA]
- Faigle J., Feldman K. Carbamazepine-biotransformation. Antiepileptic Drugs, 3rd ed. Raven Press, New York 1989; 491–504
- Gattefosse Corporation: Information Forum for Pharmaceutical Formulator: the right mix. 1989; 3: 1, [CSA]
- Giannola L., De Caro V., Di Stefano V. Comparative in vitro evaluation of cumulative release of the urinary antiseptic nalidixic acid, pipemidic acid, cioxacin and norfloxacin from white beeswax microspheres. Drug Devel. Ind. Pharm 1994; 20: 2285–2297, [CSA]
- Giannola L., De Caro V., Severino A. Carnauba wax microspheres loaded with valproic acid: preparation and evaluation of drug release. Drug Devel. Ind. Pharm 1995; 21: 1563–1572, [CSA]
- Gohel M., Amin A. Development and evaluation of lipospheres of diclophenac sodium. Ind. J. Pharmaceut. Sci. 1997; 59: 85–88, [CSA]
- Hassan E., Eshra A., Nada A. Formulation of prolonged release lipid micropellets by emulsion congealing. Optimization of ketoprofen entrapment and release. Int. J. Pharmaceut 1994; 121: 149–155, [CROSSREF], [CSA]
- James E., Singh G., Larry L., Vinod P. Method to compare dissolution profiles and a rationale for wide dissolution specification for metoprolol tartrate tablets. J. Pharmceut. Sci 1997; 86: 690–700, [CROSSREF], [CSA]
- Joseph N., Lakshmi S, Jayakrishnan A. A floating-type oral dosage form for piroxicam based on hollow polycarbonate microspheres: in vitro and in vivo evaluation in rabbits. J. Control. Rel 2002; 79: 71–79, [CROSSREF], [CSA]
- Karasulu E., Karasulu H., Ertan G., Kirilmaz L., Guneri T. Extended release lipophilicindomethacin microspheres: formulation factors and mathematical equations fitted drug release rates. Eur. J. Pharmaceut. Sci 2003; 19 99–104, [CROSSREF], [CSA]
- Kawashima Y., Ohno H., Takenaka H. Preparation of spherical matrixes of prolonged-release drugs from liquid suspension. J. Pharmaceut. Sci 1981; 70: 913–916, [CSA]
- Kawata M., Nakamura M., Goto S., Aoyama T. Preparation and dissolution pattern of Eudragit RS microcapsules containing ketoprofen. Chem. Pharmaceut. Bull 1986; 34 2618–2623, [CSA]
- Kiliçarslan M., Baykara T. The effect of the drug/polymer ratio on the properties of the verapamil HCL loaded microspheres. Inter. J. Pharmaceut 2003; 252 99–109, [CROSSREF], [CSA]
- Kowarski C., Volberger B., Versanno J., Kowarski A. A method of preparing sustained release sulfamethazine in small size batches. Am. J. Hosp. Pharm 1964; 21 409–410, [CSA]
- Krahn F. U., Mielck J. B. Effect of type and extent of crystalline order on chemical and physical stability of carbamazepine. Inter. J. Pharmaceut 1989; 53 25–34, [CROSSREF], [CSA]
- Lefebvre C., Guyot-Hermann A. M., Draguet-Brugmans M., Bouche R., Guyot J. C. Polymorphic transitions of carbamazepine during grinding and compression. Drug Devel. Ind. Pharm 1986; 12 1913–1927, [CSA]
- Lovrecich M., Orzincolo O., Rubersa F. Physicochemical aspects upon the dissolution behavior of different forms of Carbamazepine. Acta Technol. Legis. Med 1994; 5: 29–48, [CSA]
- Macdonald R. Ahtiepileptic Drugs, 3rd ed., R. H. Levy, R. H. Mattson, B. S. Meldrum, J. K. Penry. Press Raven, New York 1989; 447–455
- Malamatois S., Panagopoulou A., Hatzipantou P. Controlled release from glycerol palmito-stearate matrices prepared by dry heat granulation and compression at elevated temperature. Drug Devel. Ind. Pharm. 1991; 17: 1765–1777, [CSA]
- Miyagawa Y., Okabe T., Yamaguchi Y., Miyajimo M., Sato H., Sunada H. Controlled-release of diclofenac sodium from wax matrix granule. Inter. J. Pharmaceut 1996; 138: 215–224, [CROSSREF], [CSA]
- Naidoo N. Encapsulation and in-vitro release of indomethacin from semi-solid matrix capsules. Inter. J. Pharmaceut 1989; 55: 53–57, [CROSSREF], [CSA]
- Naima Z., Toscani S., Gines-Dorado J.-M., Chemtob C., Cèolin R., Duguè J. Interactions between carbamazepine and polyethylene glycol (PEG) 6000: characterizations of the physical solid dispersed and eutectic mixtures. Eur. J. Pharmaceut. Sci. 2001; 12: 395–404, [CROSSREF], [CSA]
- Pongpaibul Y., Price J., Whitworth C. Preparation and evaluation of controlled release indomethacin microspheres. Drug Devel. Ind. Pharm 1984; 10: 1597–1616, [CSA]
- Porter R. General principles: how to use antiepileptic drugs. Antiepileptic Drugs, 3rd ed. Raven Press, New York 1989; 117–131
- Rustichelli C., Gamberini G., Ferioli V., Gamberini M. C., Ficarra R., Tommasini S. Solid-state study of polymorphic drugs: carbamazepine. J. Pharmaceut. Biomed. Anal. 2000; 23: 41–54, [CROSSREF], [CSA]
- Sato H., Miyagawa Y., Okabe T., Miyajma M., Sunada H. Dissolution mechanism of diclofenac sodium from wax matrix granules. Inter. J. Pharmaceut. 1997; 86: 929–934, [CROSSREF], [CSA]
- Schwartz J., Simonelli A, Higuchi W. Drug release from wax matrices I: analysis of data with first-order kinetics and with the diffusion-controlled model. J. Pharmaceut. Sci. 1968a; 57: 274–277, [CSA]
- Schwartz J., Simonelli A., Higuchi W. Drug release from wax matrices II: application of a mixture theory to the sulfanilamide-wax system. J. Pharmaceut. Sci. 1968b; 57: 278–282, [CSA]
- Wong L., Gilligan C., Li Wan Po A. Preparation and characterization of sustained-release ibuprofen-cetostearyl alcohol spheres. Inter. J. Pharmaceut. 1992; 83: 95–114, [CROSSREF], [CSA]
- Wu P., Tsai M., Huang Y., Chang J., Tsai Y. In vitro and in vivo evaluation of potassium chloride sustained release formulation prepared with polyglycolyed glycerides matrices. Inter. J. Pharmaceut. 2002; 243: 119–124, [CROSSREF], [CSA]
- Yamamoto R., Baba M., Jap. Patent No. 1849, 1960