Abstract
Fluorescent quantum dots (semiconductor nanocrystals) have the potential to revolutionize biological imaging, but their use has been limited by difficulties in obtaining quantum dots that are water soluble and biocompatible. The objectives of our research were to develop a methodology for encapsulation of cadnium-selenium (CdSe) quantum dots (QDs) in phospholipid nanoemulsion that mimics the natural lipoprotein core and to study their interactions with cultured non-small cell lung cancer cells (NSCLC). We found that CdSe QDs can be efficiently encapsulated in the phospholipid nanoemulsion. The QD nanoemulsion has a particle size ∼80 nm and appears physically stable. The QD nanoemulsion interacts well with cells. The intensity of cellular fluorescence imaging increases with the cell incubation time, indicating more QDs were taken up by the cells, respectively. Two types of fluorescence microscopies confirm that QDs are primarily localized in the cytoplasm but not in the nucleus of the cells.
Quantum dots (QDs) are fluorescent nanocrystals that are emerging as unique fluorescence probes in biomedicine (Dubertret et al. Citation2002; Gao et al. Citation2004; Dahan et al. Citation2003; Larson et al. Citation2003; Jaiswal et al. Citation2003; Klarreich Citation2001; Mitchell Citation2001). These nanocrystals can be excited by a single light source and function as broadly tunable fluorescence emitters. They also have exceptional photostability for continuous visualization. The emission spectra from these QDs are narrow and symmetrical. More importantly, the wavelength of the light from QDs is largely controlled by their size and material composition, and thus an entire family of distinct colors can be generated by the same material. These unique fluorescence properties of quantum dots provide distinct advantages over traditional fluorescent probes. However, most of the quantum dots, when manufactured, have organic ligand coating on their surface (Murray, Norris, and Bawendi Citation1993; Hines and Guyot-Sionnest Citation1996) and are extremely hydrophobic.
Because biological applications require water-soluble QDs, numerous methods have been applied to make QDs water soluble. A general approach is to exchange the organophilic surface species with more polar ones as monolayer or multilayer ligand shell(s) (Bruchez et al. Citation1998; Chan and Nie Citation1998). The approach, however, could significantly affect the optical properties of the QDs. Other methods utilized overcoating QDs to make them water soluble. They were overcoated with surfactants or polymers, making them water soluble and becoming good candidates for further bioconjugation (Gerion et al. Citation2002). QDs also were overcoated with adsorbed proteins through hydrophobic or ionic interactions, allowing the conjugation of specific biomolecules (Mattoussi et al. Citation2000). This method has been used for fluoroimmunoassays (Goldman et al. Citation2002).
A distinct different approach to make QDs water soluble is to use novel and biocompatible carrier systems. However, at present, there are only a very few studies for the development of novel carrier systems for QD applications. Dubertret et al. (Citation2002), encapsulated QDs in phospholipid block-copolymer micelles and demonstrated both in vitro and in vivo imaging capabilities. In our study, we have explored the feasibility of using phospholipid nanoemulsion to encapsulate QDs. The composition of the phospholipid nanoemulsion mimics the core structure of human lipoproteins that consists of a monolayer nanoemulsion core to encapsulate hydrophobic substances and possesses surface proteins to target cellular receptors (Sarkar et al. Citation2002). This type of phospholipid nanoemulsion has been studied in our laboratory for other non-QD applications and proven to be biocompatible and interactive with cellular systems (Shawer et al. Citation2002; Alanazi, Fu, and Lu Citation2004; Pan et al. Citation2003).
Our goal in this study was to encapsulate QDs in phospholipid nanoemulsion (similar to the structure of emulsion core of very low-density lipoprotein) and examine the physical stability, size distribution, and interactions with cancer cells. We found that cadmium selenium (CdSe) QDs can be efficiently encapsulated in the phospholipid nanoemulsion that serves as a biocompatible and water-soluble carrier to interact with cells. Because there is no surface modification on QDs, the CdSe QDs kept their original fluorescence properties. The QD-encapsulated phospholipid nanoemulsion is stable and interacts well with cultured cells to deliver the QDs inside the cells for fluorescence imaging. Therefore, this method could be a universal method to encapsulate hydrophobic QDs and allow further modifications on nanoemulsion structures for cellular targeting purpose (Sarkar et al. Citation2002).
EXPERIMENTS
CdSe QDs, purchased from Nanomaterials and Nanofabrication Laboratories (Fayetteville, AR, USA), were dissolved in toluene with a particle size ∼4.8 nm in diameter. The surfaces of these commercial CdSe QDs were protected with octadecylamine. It has an absorption peak at 604 nm and emission peak at 615 nm. Egg yolk phosphatidylcholine (99%), L-lysophosphatidylcholine (99%), triolein (99%), Dulbecco's Modified Eagle's Media (DMEM), and trypsin-EDTA were obtained from VWR Scientific Products (Bridgeport, NJ, USA). H1299 non-small cell lung cancer cells (NSCLC) were obtained from ATCC (Manassas, VA, USA). Analytical grade chloroform and other chemicals were purchased from Sigma-Aldrich. Particle size of the nanoemulsions was measured by NICOMP 380 submicron particle size analyzer (Santa Barbara, CA, USA). Olympus Fluoview confocal laser scanning microscope and Olympus (IX51) inverted microscope with U-LH100 HG mercury lamp were employed for fluorescence visualization of QDs in the cell culture experiments.
Phosphatidycholine (45.63 mg), L-lysophosphatidylcholine (4.6 mg), and triolein (154.5 μ L) were dissolved in 2.0 mL chloroform in a 25-mL round-bottom flask. CdSe QDs, 10 mg in 2.5 mL toluene, were added. The mixture was bubbled with N2 overnight until all organic solvents of toluene and chloroform were removed. The dark purple-colored remaining was added with 5 mL PBS and sonicated (with output setting at 200) at room temperature for 30 min (Branson Ultrasonics Corporation, Danbury, CT, USA). Sonication was conducted under nitrogen gas to reduce lipid oxidation during emulsification. The color of the mixture turned coffee-milky after sonication, indicating the formation of the nanoemulsion. The nanoemulsion was transferred into a sterile vial and kept in a refrigerator at 4°C. The concentration of QDs in this emulsion was ∼2 mg/mL.
Cells were seeded in 2-well Lab-Tek chambered slides (Nalge Nunc) and grown in DMEM supplemented with 10% fetal bovine serum in a humidified 5% CO2 incubator at 37°C (1.5 mL culture medium in each well). When the cells reached 70–80% confluence, 75 μ L of QD-encapsulated nanoemulsion was added to each chamber and incubated with the cells for 10 min, 30 min, 1 hr, 3 hr, and 4 hr in the CO2 incubator. The cells were then washed twice with phosphate-buffered saline (PBS) and fixed with 1 mL 2% formaldehyde in PBS for 5 min at room temperature. The fixed cells were washed 2 times with PBS. Some of the samples were further treated to stain the nuclei of cells using Dapi-blue (a traditional blue fluorescence probe for nuclei) with 1 mL PBS and 1 mu; L Dapi-blue added to the slide chamber and incubated for 10 min at room temperature. After cells were washed, 15 μ L mounting medium was dropped on the slides. The slides were covered by cover glass and kept overnight.
To ensure the proper QD concentration used in the cell uptake study, a preliminary experiment was performed in which different amounts of QDs emulsion (10 μ L, 25 μ L, 50 μ L, 75 μ L, and 100 μ L) were added to each chamber and the preparations were used to examine cellular distribution of QDs with the routine fluorescent microscope. The preparation with 75-μ L QDs nanoemulsion was found to be applicable because of the suitable brightness (clear visualization on the microscope). Higher amount of QD-encapsulated phospholipid nanoemulsion (100 μ L) in the culture medium resulted in some degree of cell death.
RESULTS AND DISCUSSION
The QD-encapsulated phospholipid nanoemulsion showed excellent homogenecity. shows both QDs solubilized in toluene (as originally purchased) (1a) and QD-encapsulated phospholipid nanoemulsion (1b). Similar to the original QDs in toluene with strong fluorescence emission, the QD-encapsulated phospholipid nanoemulsion emitted bright red fluorescence. was the fluorescent image of the QD-encapsulated phospholipid nanoemulsion taken by a routine epifluorescence microscope with the 40 × objective, with appropriate filter combination for fluorescence excitation while continuous-wave excitation was provided by a mercury lamp. The nanoemulsion sample showed even and bright fluorescence, indicating that CdSe QDs are well encapsulated and homogeneously distributed in the nanoemulsion. Microscopic comparison also was made between the QDs solubilized in toluene and in QD-encapsulated phospholipid nanoemulsion. Results indicated that QDs kept their original fluorescent brightness after encapsulation in nanoemulsion.
FIG. 1 (a) Commercial QDs with C18H37NH2 shell in toluene solution; (b) QD-encapsulated phospholipid nanoemulsion; (c) epifuorescence microscopic imaging of QD-encapsulated phospholipid nanoemulsion.
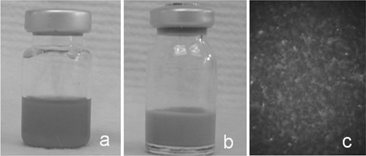
The mean particle size (measured as number weighted) was ∼80 nm (n = 5) (). The nanoemulsion appeared very stable. After the nanoemulsion was kept for 2 months in a refrigerator at 4°C, neither significant size change nor obvious precipitation was observed. The fluorescence intensity was not affected after the 2-month storage (data not shown).
FIG. 2 Size distribution of QD-encapsulated phospholipid nanoemulsion by photon correlation spectroscopy. The number weighted mean particle size was 80 nm.
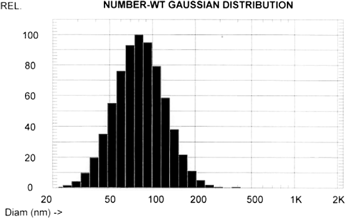
After the H1299 lung carcinoma cells were incubated with QD-encapsulated phospholipid nanoemulsion for 10 min, 30 min, 1 hr, 3 hr, and 4 hr, respectively, they were visualized by the epifluorescence microscope and by the confocal microscope. shows selected images from confocal microscopy. is the negative control containing no QD nanoemulsion and only showing a cellular autofluoresecence background. The autofluorescence, however, was photobleached immediately under the laser irradiation within 30 sec. On the other hand, the QDs were very photostable (). After autofluoresecence disappeared, QDs remained bright. After 10 min incubation of the cells with QD nanoemulsion, we found that QDs were located on cell periphery without significant distribution inside the cells (). However, the longer incubation times of 30 min, 1 hr, 3 hr, and 4 hr of the cells with QD nanoemulsion resulted in significant distribution of QDs inside the cells. and showed the cells that were incubated with QD nanoemulsion for 30 min and 3 hr, respectively. The intensity of fluorescence imaging increased with the incubation time, indicating more QDs were taken up by cells, respectively.
FIG. 3 Confocal microscopy of H1299 cells. (a) Negative control; (b) H1299 cells were incubated with QDs for 10 min; (c) Incubated for 30 min; (d) Incubated for 3 hr.
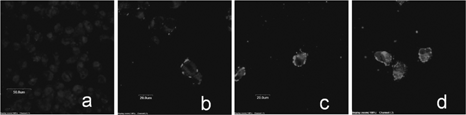
To further examine the location of QDs inside the cells, the confocal laser scanning microscope was adjusted to visualize each thin layer section () of the H1299 cells after the incubation with QD nanoemulsion for 4 hr. The scanning height between each layer was 1 μ m. The thickness for the fixed cells was ∼11 μ m. Therefore, totally 11 layers of images were recorded. , , , and are the scanning images of the 3rd, 5th, 9th, and 11th layers, respectively.
FIG. 4 Section imaging of H1299 lung cancer cells after the incubation with QD-encapsulated phospholipid nanoemulsion for 4 hr. The images were taken layer by layer using confocal laser scanning microscope, and the scanning height was 1 μ m. Totally 11 images were taken. (a), (b), (c), and (d) are the images of the selected, 3rd, 5th, 9th, and 11th layers, respectively.
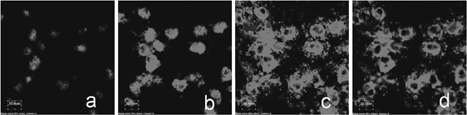
This series of scanning images shows a wide distribution of red fluorescence within the cells, except the nucleus section. The result indicated that the QDs were mainly localized in the cytoplasm in the cells. To further confirm the location of QDs within the H1299 cells, conventional fluorescence microscopy was employed to obtain the image after Dapi-blue staining of the nucleus. shows the three images taken by fluorescence microscopy. These images with different settings were taken separately for the same experimental area. First image was taken under bright field setting that showed the whole cells (). The second image was taken in the blue fluorescent setting in which the nuclei under Dapi-blue staining showed their sizes and shapes (). The third image was taken under red fluorescent emission in which the locations of QDs were seen (). The 3 images were merged into one picture that showed the nuclei and the QDs in the cells after the incubation with QD nanoemulsion (). demonstrated a consistent result with that from the confocal microscope imaging in . Both results indicated that the QDs were primarily localized in the cytoplasm in the H1299 lung carcinoma cells.
FIG. 5 The images were taken by routine Fluoview microscopy. (a) Bright field image for the cells; (b) The blue fluorescent image of nuclei stained by Dapi-blue dye; (c) red fluorescent image of QDs; (d) a photographically merged image that was composed from above three images.

Lipoproteins are natural protein-phospholipid complexes that have a hydrophobic emulsion core that can solubilize hydrophobic substances and carry them through blood circulation (Sarkar et al. Citation2002; Chung and Wasan Citation2004). As a new pharmaceutical bioimaging carrier, very low-density lipoprotein (VLDL) is particularly interesting because it contains a relatively small amount of proteins (∼5–10% protein) and a large amount of triglycerides (∼50–65% within the emulsion core) which can be used to sufficiently solubilize more hydrophobic substances. This type of phospholipid nanoemulsion mimicking the structure of VLDL was examined early in our laboratory as a carrier system for targeted delivery of a new hydrophobic compound to cancer cells (Derfus, Chan, and Bhatia Citation2004). The nanoemulsion demonstrated the sufficient capability to solubilize the hydrophobic compound. The structure of the phospholipid nanoemulsion was verified based on the molecular surface area and molecular volume for each component of the nanoemulsion in respect to the changes in particle size.
We demonstrated that similar to the natural lipoproteins phospholipid was predominately located at the surface and the hydrophobic compound, triolein, and cholesteryl oleate were mainly located in the core of the phospholipid nanoemulsion. Based on the earlier study, we believe that a similar structure exists for the QD-encapsulated phospholipid nanoemulsion. Its schematic representation is represented in . Because the particle size is ∼80 nm, we suggest that there are several CdSe nanocrystals (∼4.8 nm in diameter) located in each nanoemulsion core. The large proportion of triglycerides facilitates the incorporation of the CdSe nanocrystals.
FIG. 6 Schematic representation of QD-encapsulated phospholipid nanoemulsion containing fluorescent CdSe nanocrystals and triolein in the emulsion core.
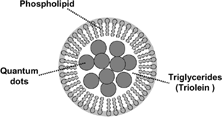
As seen from our cell culture study, the QD-encapsulated phospholipid nanoemulsion interacts well with the cells, and an increasing amount of QDs was taken up by the cells with the longer incubation time. Although the mechanism was not clearly demonstrated, similar phenomenon was observed in our early study of gene transfection using this type of nanoemulsion as a gene carrier (Alanazi et al. Citation2004). The study demonstrated a highly effective transfection capability in cell culture of rabies DNA vaccine carried by similar type of phospholipid nanoemulsion. It appears that the phospholipid nanoemulsion can interact with cells to deliver the contents. In another study (Pan et al. Citation2003), we demonstrated that a similar type of phospholipid nanoemulsion induced a low cytotoxicity and demonstrated the biocompatibility of the phospholipid nanoemulsion. In the experiment, the cell viability showed up with 75% using the phospholipid nanoemulsion compared to only 24% using Lipofectamine™ system, a commercial liposomal system. In the present study, the QD-encapsulated phospholipid nanoemulsion appeared compatible with the cultured cells, except when the concentration became high (100 μ L of QD-encapsulated phospholipid nanoemulsion in the slide chamber).
CONCLUSION
CdSe QDs nanocrystals can be encapsulated efficiently with the phospholipid nanoemulsion that mimics the natural lipoprotein core. Our approach introduces a new method to make QDs water soluble while maintaining the QDs' original fluorescence properties. QD-encapsulated phospholipid nanoemulsion has a size in ∼80 nm and appears physically very stable. The QD-encapsulated phospholipid nanoemulsion interacts with cells well to deliver the QDs into H1299 lung carcinoma cells. The intensity of cellular fluorescence imaging increased with the incubation time, indicating more QDs were taken up by the cells, respectively. Both fluorescence microscopies confirm that QDs were primarily localized in the cytoplasm but not in the nucleus of the cells.
This article was made possible by Temple University Research Bridge Grant to support Dr. Suwen Liu's postdoctoral fellowship. The authors also acknowledge Dr. Y. Wang at NNL for his QD samples and useful discussions.
Dr. Suwen Lu is currently working at the Connecticut Institute for Aging and Cancer, 75 Woodmont Rd., Milford, CT 06460.
REFERENCES
- Alanazi F., Fu Z. F., Lu D. R. Effective transfection of rabies DNA vaccine in cell culture using an artificial lipoprotein carrier system. Pharmaceut. Res. 2004; 21: 676–683, [CSA]
- Bruchez M., Moronne M., Gin P., Weiss S., Alivisatos A P. Semiconductor nanocrystals as fluorescent biological labs. Science 1998; 281: 2013–2016, [PUBMED], [INFOTRIEVE], [CROSSREF], [CSA]
- Chan W. C. W., Nie S. Quantum dot biocojugates for ultrosensitive nonisotopic detection. Science 1998; 281: 2016–2018, [PUBMED], [INFOTRIEVE], [CROSSREF], [CSA]
- Chung N. S., Wasan K. M. Potential role of the low-density lipoprotein receptor family as mediators of cellular drug uptake. Adv. Drug Del. Rev. 2004; 56: 1315–1334, [CROSSREF], [CSA]
- Dahan M., Levi S., Luccardini C., Rostaing P., Riveau B., Triller A. Diffusion dynamics of glycine receptors revealed by single-quantum dot tracking. Science 2003; 302: 442–445, [PUBMED], [INFOTRIEVE], [CROSSREF], [CSA]
- Derfus A. M., Chan W. C. W., Bhatia S. N. Nano Letters 2004; 4: 11–18, [CROSSREF], [CSA]
- Dubertret B., Skourides P., Norris D. J., Noireaux V., Brivanlou A. H., Libchaber A. In vivo imaging of quantum dots encapsulated in phospholipid micelles. Science 2002; 298: 1759–1762, [PUBMED], [INFOTRIEVE], [CROSSREF], [CSA]
- Gao X., Cui Y., Levenson R. M., Chung L. W., Nie S. In vivo cancer targeting and imaging with semiconductor quantum dots. Nat. Biotechnol. 2004; 22: 969–976, [PUBMED], [INFOTRIEVE], [CROSSREF], [CSA]
- Gerion D., Parak W. J., Williams S. C., Zanchet D., Micheel C. M., Alivisatos A. P. Sorting fluorescent nanocrystals with DNA. J. Am. Chemi Soc. 2002; 124: 7070–7074, [CROSSREF], [CSA]
- Goldman E. R., et al. Anal. Chem. 2002; 74: 841, [PUBMED], [INFOTRIEVE], [CROSSREF], [CSA]
- Hines M. A., Guyot-Sionnest P. J. Phy. Chem. 1996; 100: 468, [CROSSREF], [CSA]
- Jaiswal J. K., Mattoussi H., Mauro J. M., Simon S. M. Long-term multiple color imaging of live cells using quantum dot bioconjugates. Nat. Biotechnol. 2003; 21: 47–51, [PUBMED], [INFOTRIEVE], [CROSSREF], [CSA]
- Klarreich E. Biologists join the dots. Nature 2001; 413: 450–452, [PUBMED], [INFOTRIEVE], [CROSSREF], [CSA]
- Larson D. R., Zipfel W. R., Williams R. M., Clark S. W., Bruchez M. P., Wise F. W., Webb W. W. Water-soluble quantum dots for multiphoton fluorescence imaging in vivo. Science 2003; 300: 1434–1436, [PUBMED], [INFOTRIEVE], [CROSSREF], [CSA]
- Mattoussi H., et al. J. Am. Chem. Soc. 2000; 122: 12142–12150, [CROSSREF], [CSA]
- Mitchell P. Turning the spotlight on cellular imaging. Nat. Biotechnol. 2001; 19: 1013–1017, [PUBMED], [INFOTRIEVE], [CROSSREF], [CSA]
- Murray C. B., Norris D. J., Bawendi M. G. J. Am. Chem. Soc. 1993; 115: 8706, [CROSSREF], [CSA]
- Pan G., Shawer M., Øie S., Lu D. R. In vitro gene transfection to glioma cells using a novel and less cytotoxic artificial lipoprotein delivery system. Pharmaceut. Res. 2003; 20: 738–745, [CROSSREF], [CSA]
- Sarkar R., Halpern D. S., Jacobs S. K., Lu D. R. LDL-receptor mediated drug targeting to malignant tumors. Biomedical Aspects of Drug Targeting, V. R. Muzykantov, V. P. Torchilin. Kluwer Academic Publisher, Norwell, MA 2002 327–345
- Shawer M., Greenspan P., Øie S., Lu D. R. VLDL-resembling phospholipid-submicron emulsion for cholesterol-based drug targeting. J. Pharmaceut. Sci. 2002; 91(6)1405–1413, [CROSSREF], [CSA]