Abstract
Ketorolac tromethamine, a potent nonnarcotic analgesic agent and 800 times more potent than aspirin, is indicated for the short-term management of moderate to such severe painful states as post operative pain, acute musculoskeletal pain, and dental pain. It Given every 6 hr intramuscularly in patients for acute pain, to avoid frequent dosing and patient inconvenience Ketorolac from ethamine was found suitable for parenteral depot system by biodegradable microspheres for the present study. Ketorolac tromethamine-loaded microspheres were prepared by o/w emulsion solvent evaporation technique using different polymers viz. polycaprolactone, poly-dl-lactide (Resomer) and poly lactic acid (PLA). To tailor the release profile of drug for several days, blends of Resomer and PLA were prepared with polycaprolactone in different ratios. Higher encapsulation efficiency was obtained with microspheres made with pure Resomer. Surface topography was studied by scanning electron microscopy, which showed spherical shape of microspheres. Residual solvent analysis was carried out to determine the residual amount of dichloromethane in microspheres and the content was found within permissible limits. Differential scanning calorimetric studies also were carried out to study any drug polymer interactions. We concluded that with careful selection of different polymers and their combinations, we can tailor the release of ketorolac tromethamine for long periods.
Nowadays, much of the research is aimed at developing drug delivery systems with maximum therapeutic benefits for safe and effective management of diseases. The concepts are based on controlled drug delivery, biotechnology, and polymer sciences, which surpass all barriers of a disease. A probe into current drug delivery systems research suggests the development of drug delivery systems in future purport to maximize therapeutic performance, eliminate undesirable side effects of drugs, and increase patient compliance. With the development of various platform drug delivery technologies, a rebirthing of existing molecules has taken place with which their market values along with patent lives have increased. Worldwide development of these technologies and sale of drug delivery products are continuously increasing.
Despite the competition from alternative modalities, demand for controlled release (CR) parenteral drug delivery systems is rising 9.5% annually and expected to reach almost $20 billion in 2005. The field of CR parenteral drug delivery systems has grown exponentially in recent years, in parallel with the development of new protein therapeutics. Caused by the explosion of genomic information and the final mapping of the human genome, a large number of peptides and proteins may become candidates for therapeutic application. The understanding of protein functions in the etiopathology of currently incurable diseases could lead to new therapeutic approaches. Hence, the development of new injectable drug delivery systems, which protect proteins against denaturation in body fluids and allow sustained-release profiles are in great demand.
There also are important economic reasons to consider because development of an injectable CE formulation can extend the patent life of a drug. Since a number of recombinant proteins (biogenerics) fall into this category, parenteral delivery systems are an attractive proposition. Generally, parenteral depot systems could minimize side effects by achieving constant, “infusion-like” plasma level time profiles, especially important for proteins with narrow therapeutic indices. A dose reduction resulting from the avoidance of peaks and valleys, as well as the enhancement of patient compliance by reducing the frequency of application, are further potential benefits.
Due to poor absorption and low bioavailability of all noninjectable routes, the parenteral route becomes the most viable route for long-term delivery of drugs. Parenteral drug administration, especially intravenous infusion, leads to easy and complete absorption of drug in systemic circulation, eliciting a prompt drug response. But this requires direct medical supervision and hospitalization of the patient. Parenteral drug administration via the intramuscular route or the subcutaneous route has a fairly rapid onset of action followed by a rapid decline in the blood-drug level and leading to relatively short duration of therapeutic response. Thus, considering these problems associated with different modes of drug administration, injectable depot formulation seems to provide the solution. Parenteral depot formulations can overcome these problems by controlling the drug release over a predetermined time span usually in order of days to weeks to months (Okada et al. Citation1994, Citation1989). There are various means of formulating prolonged release preparations and the use of biodegradable microspheres is one of them (Cruaud, Benita, and Benoit Citation1999; Bozdag et al. Citation2001; Li et al. Citation2001; Thies and Bissery Citation1984).
Interest in biodegradable polymers developed for two reasons; one, surgical removal of drug depleted delivery system of nonbiodegradable polymers is difficult and nonremoval may pose toxicological problems; two, although diffusion controlled delivery systems are excellent means of achieving predetermined rates of drug delivery, they are limited by polymer permeability and characteristics of the drug. Various drugs have been encapsulated in these polymeric systems like tetanus toxoid (Jaganathan et al. Citation2005), human growth hormone (Jostel et al. Citation2005), naltrexone (Dean Citation2005), carbidopa/levodopa (Arica et al. Citation2005), cisplatin (Chandy et al. Citation2002), and calcitonin polypeptide (Prabhu, Sullivan, and Betager Citation2002) Various FDA-approved controlled-release parenteral formulations based on these biodegradable microspheres (Sinha and Trehan Citation2003) are available in the market: Lupron Depot® (leuprolide acetate), Nutropin Depot® (recombinant human growth hormone), Zoladex® (goserelin acetate), Decapeptyl® (triptorelin), Sandostatin LAR Depot® (octreotide acetate), Posilac® (recombinant bovine somatropin), buserelin (Suprecur® MP), minocycline hydrochloride (Arestin®), lanreotide (somatuline® LA), and risperidone (Risperidal® Consta ™).
MATERIALS AND METHODS
Biodegradable polymers—poly-dl-lactide (PDLLA, Resomer) and Poly lactic acid (PLA)—were procured from Boehringer Ingelheim (Germany); and Purac (Holland). Polycaprolactone (PCL) was obtained from Fluka, ketorolac tromethamine from Sun Pharma (Ahemdabad, India), methocel from Panacea Biotech (Lalru, India), and dichloromethane from Qualigens Fine Chemicals (India).
Preparation of Microspheres
Ketorolac tromethamine-loaded microspheres were prepared by o/w solvent evaporation technique (Sinha and Trehan Citation2005). Ketorolac tromethamine (25 mg) was dispersed in 10 ml of dichloromethane containing 500 mg of polymer. (In case of blends, the amount of 500 mg was divided according to ratios e.g., in case of 1:1, 250 mg of PLA/Resomer and 250 mg of PCL). This dispersion was then added to an acidic aqueous phase (50 ml) containing 0.5% of HPMC while stirring to form o/w emulsion. The stirring was continued until complete evaporation of the solvent was achieved. The resulting microspheres were filtered, washed with excess of water to remove any unentrapped drug, and finally dried.
Determination of Encapsulation Efficiency and Percentage Yield
Ketorolac tromethamine containing microspheres (10 mg) were mixed with 1 ml × dichloromethane, and then 10 ml of water was added into above solution for the extraction of ketorolac tromethamine. The solution was mixed by vortex mixer, clarified by standing for few minutes, and then upper layer (water) was analyzed for ketorolac tromethamine spectrophotometrically at 323 nm. The encapsulation efficiency and percent yield were calculated using the following formulae:
In Vitro Release Profile Studies
Microspheres (15 mg) were suspended in 1.5 ml of PBS (phosphate buffered saline; pH 7.4) in Eppendorf tubes and placed in an incubator-shaker (37°C) at 50 rpm. At predetermined time intervals, samples were centrifuged and supernatants were withdrawn and replaced with fresh PBS. The process was continued until the completion of the dissolution study. Concentration of ketorolac tromethamine in supernatants was analyzed spectrophotometrically at 323 nm.
Scanning Electron Microscopy
The surface topography of microspheres was investigated using scanning electron microscope (SEM) (JSM 6100 JEOL, Tokyo, Japan). Microspheres samples were mounted onto stubs using double-sided adhesive tape. The stubs were then vacuum coated with gold using fine coat ion sputter JFC 1100 (JEOL Japan). Then the microspheres were examined with SEM (JEOL JSM 6100).
Size Distribution Analysis
The mean diameter and particle size distribution were examined by laser diffractometry using Malvern Mastersizer 2000. For laser diffraction measurements, microspheres were suspended in distilled water, which was continuously homogenized at 12,000 rpm and sonicated for 90 sec prior to particle size determination.
Residual Solvent Analysis
The most popular and most appropriate specific residual solvent analysis is done by gas chromatography. To determine the amount of dichloromethane in microspheres, known weight of microspheres after dissolving in DMF (dimethylformamide) were directly taken in head space vial and injected into a gas chromatograph. The column used was DB-624, 30 m × 0.32 mm (fused silica, 1.8 μ film).
Differential Scanning Calorimetric Studies
A small amount of microsphere samples was placed in hermetically sealed aluminium pans and heated from 40°C to 300°C at a heat flow rate of 10°C/min under nitrogen spurge. The glass transition temperature (Tg°C) and melting point of samples were recorded as endotherms.
RESULTS AND DISCUSSION
Scanning electron micrographs of Resomer, Resomer: PCL, PLA, and PLA: PCL microspheres loaded with ketorolac tromethamine are shown in , , , , respectively. Microspheres of all the polymers were found to be spherical in nature. As evident from and , the microspheres prepared from the pure Resomer and Resomer:PCL were smooth, spherical, and porous. But there was much porosity in the Resomer: PCL microspheres as compared with pure Resomer microspheres. The surface topography of microspheres prepared from PLA and PLA:PCL is shown in and . The microspheres of PLA were smooth and spherical in nature with some very minute pores on their surface, whereas the microspheres prepared with blends of PCL and PLA in the ratio of 1:1 were spherical and highly porous in nature with big pores as clear from . This highly porous nature was attributed to a faster rate of salting out and precipitation of high molecular weight polymer during solvent evaporation compared with those of the lower molecular weight polymer (Elkheshen Citation1996; Wang et al. Citation1997).
FIG. 2 Scanning electron micrograph of ketorolac tromethamine-loaded PCL: Resomer (1:1) microspheres.
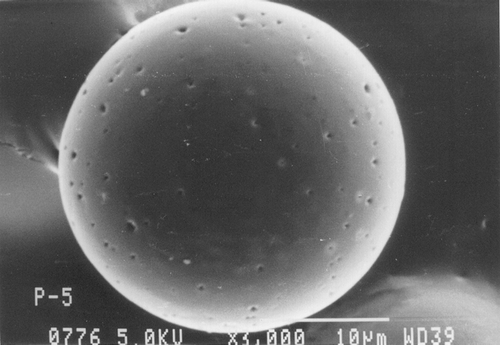
Percent Yield, EE, and Particle Size
The results of percent yield, percent encapsulation efficiency, and particle size analysis are listed in . Particle size distribution curves are shown in . Yield was reasonably good in all batches. Microencapsulation of highly water-soluble drugs like ketorolac tromethamine by o/w emulsion solvent evaporation technique results in quite low entrapment efficiency because of the solubility of drug in the outer phase. Thus, to increase encapsulation efficiency, pH of the external phase was adjusted toward the acidic side to minimize the solubility of the drug in external phase.
TABLE 1 Percentage yield, encapsulation efficiency EE and particle size data of PLA, Resomer and their blends with PCL
Higher encapsulation efficiency was obtained in the batch made with pure Resomer, which was 69% compared with its blends in which it varied from 50–55%. This significant difference in percent EE as compared with its blends might be attributed to the higher intrinsic viscosity of the pure polymer solution, which prevented the release or leakage of drug from the inner phase to outer phase and hence resulted in increased entrapment efficiency. With pure PLA also, higher encapsulation was obtained i.e., 61% as compared with its blends where it ranged from 53–56% due to the same reason as cited above. Higher encapsulation efficiency in pure resomer as compared with pure PLA might be attributed to higher intrinsic viscosity of the polymer. In general low encapsulation efficiency in batches could be attributed to high water solubility of ketorolac tromethamine, which partitions into the continuous aqueous phase during the manufacturing process (Selek et al. Citation2003).
Release Profiles
The release profile of different batches of microspheres is shown in (Resomer and its blends with PCL) and (PLA and its blends with PCL). In batches made with Resomer and its blends, burst effect ranged from 9–18%. About 50% of the drug was released in 2 days 1:3 (R4) (Resomer: PCL) in contrast to 5 days in 1:1(R2) (Resomer: PCL) and 30 days in 3:1(R3) (Resomer: PCL) microspheres. In pure Resomer (R1), only 37% of the drug was released in 60 days. Microspheres made with 1:3 (Resomer: PCL) released 77% of the drug in 60 days in contrast to 70% and 58% in 60 days in 1:1 (Resomer: PCL) and 3:1 (Resomer: PCL), respectively. Release profiles indicated lower burst effect of ∼6% in pure PLA (L1) as compared with ∼12% in case of 1:1 (L2) (PLA:PCL) and 1:3 (L4) (PLA:PCL) blends. About half of the drug i.e., 50% was released in 3 days in 1:3 (PLA: PCL) in contrast to 14 days in 1:1 (PLA: PCL), 30 days in 3:1 (L3) (PLA: PCL). In the of pure PLA, only 27% of the drug was released in 60 days. The results revealed that microspheres made with 1:3 (PLA: PCL) blend released 76% of the drug in 60 days as compared with 63% and 53% in 60 days in case of 1:1 (PLA:PCL), 3:1 (PLA:PCL) microspheres, respectively.
Two processes govern release of drug from biodegradable polymers: first, the release is diffusion controlled. Second and later on a major part of drug, which is bound to polymeric matrix, is released by matrix erosion mechanism (Selek et al. Citation2003). Comparatively higher release in Resomer batches as compared with PLA batches was due to higher molecular weight the latter, as higher molecular weight polymers degrade slowly. Release from pure polymers was slower than their corresponding blends due to semicrystalline nature of PCL. The result of which water can penetrate easily into the amorphous part of polymer and facilitate the release of drug by diffusion through the pores.
DSC Studies
Our DSC studies of drug, polymer, and microspheres attempted to define the physical state of drug in these carriers and the possibility of interaction between the drug and polymer within the network of polymer in microspheres. Results are illustrated in . DSC patterns of pure polymers—PLA, Resomer, and PCL—showed peaks at 180°C, 59°C, and 65.74°C, respectively. DSC pattern of Resomer microspheres showed one peak at 55°C whereas DSC thermograms of PLA microspheres revealed two peaks at 67°C and 177°C, respectively. For Resomer:PCL (1:1) microspheres, one peak at 65.71°C was observed. The endothermic peaks 60.26°C and 176.24°C were observed for PLA: PCL (1:1) microspheres. All peaks were observed near the melting point and glass transition temperature of polymer implying the absence of any drug-polymer interactions. The thermograms of microsphere batches did not show any endothermic peak of drug (161°C), indicating molecular dispersion of drug in microspheres.
FIG. 8 Differential scanning calorimetric thermograms of pure polymers and ketorolac tromethamine-loaded microspheres.
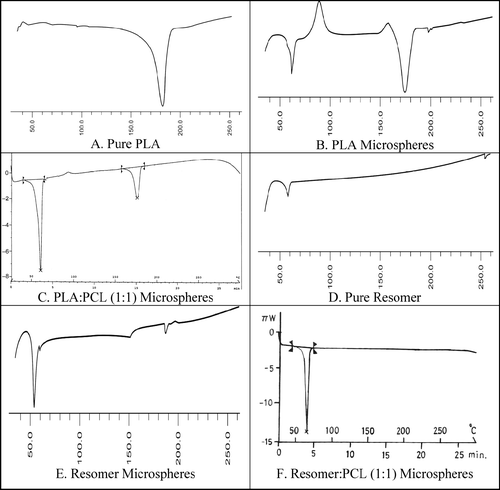
Residual content of dichloromethane was found to be 266 ppm, which is well below the pharmacopoeial limits of 600 ppm and indicates safe use of microspheres for administration ().
CONCLUSION
Studies revealed that with pure PLA and pure Resomer drug release was sustained for up to 60 days. When these polymers were blended with PCL in different ratios, drug release could be tailored from days up to months. We concluded that with careful selection of different polymers and their blending with PCL in appropriate ratios, release of ketorolac tromethamine can be tailored from several hours to several days. Ketorolac tromethamine is widely used to treat moderate to severe post-operative or postsurgery pains, acute musculoskeletal pain, chronic pain states, postpartum or labor pains, and posttraumatic pain, and treatment protocols that involve 2–3 injections per day. So, by following this approach of encapsulating it in biodegradable microspheres using different polymers, levels of ketorolac tromethamine can be maintained for long periods in the body, which can obviate painful injections given number of times per day.
We are most grateful to the University Grants Commission for providing financial assistance and to Boehringer Ingelheim for providing gift samples of Resomer.
REFERENCES
- Arica B., Kas H. S., Moghdam Akalan A., et al. Carbidopa/levodopa-loaded biodegradable microspheres: in vivo evaluation on experimental Parkinsonism in rats. J. Control. Rel. 2005; 102: 689–97
- Bozdag S., Calis S., Kas H. S., et al. In vitro evaluation and intra-articular administration of biodegradable microspheres containing naproxen sodium. J. Microencapsul. 2001; 18: 443–456
- Chandy T., Wilson R. F., Rao G. H., Das G. S. Changes in cisplatin delivery due to surface-coated poly (lactic acid)-poly (epsilon-caprolactone) microspheres. J. Biomater. Appl. 2002; 16: 275–291
- Cruaud O., Benita S., Benoit J. P. The characterization and release kinetics evaluation of beclofen microspheres designed for intrathecal injection. Int. J. Pharm. 1999; 177: 247–257
- Dean R. L. The preclinical development of Medisorb Naltrexone, a once a month long acting injection, for the treatment of alcohol dependence. Front Biosci. 2005; 10: 643–655
- Elkheshen S. Simplex lattice design for the optimization of the microencapsulation of a water soluble drug using poly(lactic acid) and poly(lactide-co-glycolide) copolymer. J. Microencapsul. 1996; 7: 447–462
- Jaganathan K. S., Rao Y. U., Singh P., et al. Development of a single dose tetanus toxoid formulation based on polymeric microspheres: a comparative study of poly (D, L-lactic-co-glycolic acid) versus chitosan microspheres. Int. J. Pharm. 2005; 294: 23–32
- Jostel A., Mukherjee A., Alenfall J., et al. A new sustained-release preparation of human growth hormone and its pharmacokinetic, pharmacodynamic and safety profile. Clin. Endocrinol. (Oxf) 2005; 62: 623–627
- Li F. Q., Hu J. H., Lu B., et al. Ciprofloxacin loaded bovine serum albumin microspheres: preparation and drug release in vitro. J. Microencapsul. 2001; 18: 825–829
- Okada H., Heya T., Igri Y., et al. One month release injectable microspheres of leuprolide acetate inhibit steroidogenesis and genital organ growth in rats. Int. J. Pharm. 1989; 54: 231–239
- Okada H., Doken Y., Ogawa Y., Toguchi H. Preparation of three-month depot injectable microspheres of leuprorelin acetate using biodegradable polymers. Pharm. Res. 1994; 11: 1143–1147
- Prabhu S., Sullivan J. L., Betageri G. V. Comparative assessment of in vitro release kinetics of calcitonin polypeptide from biodegradable microspheres. Drug Deliv. 2002; 9: 195–198
- Selek H., Sahin S., Ercan M. T., et al. Formulation and in vitro/in vivo evaluation of terbutaline sulphate incorporated in PLGA(25/75) and L-PLA microspheres. J. Microencapsul. 2003; 20: 261–271
- Sinha V. R., Trehan A. Biodegradable microspheres for protein delivery. J.Control. Rel. 2003; 90: 261–280
- Sinha V. R., Trehan A. Formulation, characterization and evaluation of ketorolac tromethamine loaded biodegradable microspheres. Drug Del. 2005; 12: 133–139
- Thies C., Bissery M. C. Biodegradable microspheres for parenteral administration. Biomed. Appl. Microencapsul, F. LiM. CRC Press, Boca Raton, FL 1984; 53–74
- Wang Y. M., Sato H., Horikoshi I. In vitro and in vivo evaluation of taxol release from poly (lactic-co-glycolic acid) microspheres containing isopropyl myristate and degradation of the microspheres. J. Control. Rel. 1997; 49: 157–166