Abstract
A new vegetable oil based oil-in-water microemulsion is developed and characterized as a prospective delivery system for in vivo application A particular weight percent composition 5/30/65 (clove oil/Tween-20/water) was selected (V1) from the clear oil-in-water zone of the pseudoternary phase diagram comprising clove oil, polyoxyethylene sorbitan monolaurate (Tween-20), and water. Two modifications of V1, (V2 and V3) were prepared by addition of dipalmitoyl phosphatidyl choline (DPPC), and a mixture of DPPC and cholesterol, respectively. A model drug diospyrin (a plantderived quinonoid compound) was encapsulated in the dispersed clove oil droplets of the three systems and designated as DV1, DV2, and DV3, respectively. The size of the dispersed clove oil droplets ranged between 9–20 nm as determined by dynamic light scattering. The stability of the vehicles, before and after encapsulation, was assessed under varying conditions of time and temperature and was found to be stable for 1 year and over a temperature range of 4-40°C. The ultraviolet-visible spectrum of diospyrin after encapsulation in the compartmentalized medium remained almost identical to that dissolved in chloroform. The single-dose acute toxicity of V1 and DV1 was assessed in vivo by carrying out survival study and enzyme assay in Swiss Albino mice.The vehicle was safe at a volume of 0.05 ml when injected intraperitoneally into the mice.
Development of microemulsions (Lawrence Citation1996; Paul and Moulik Citation1997; Tenjarala Citation1999; Trotta Citation1999; Moreno et al. Citation2000), liposomes (Gregoriadis Citation1993), niosomes (Uchegbu and Florence Citation1995; Sinha et al. Citation2002), organogels (Kantaria, Rees and Lawrence Citation1999), and nanocapsules (Watnasirichaikul et al. Citation2002) as drug delivery systems has been a major challenge for the pharmaceutical industry during the past decade. These organized systems used as carriers could achieve increased solubilization of lipophilic drugs with concomitant modification of their pharmacokinetic profiles, often leading to improvement in therapeutic efficacy (Trotta, Ugazio, and Gasco Citation1995; Corswant, Von Thorem, and Engstrom Citation1998; Cortesi and Nastruzzi Citation1999; Park and Kim Citation1999). Moreover, these systems are known to protect the encapsulated drugs against enzymatic degradation (Sariciaux, Alan, and Sado Citation1995) and to enhance membrane permeability induced by the surfactant and cosurfactant constituents (Swenson and Curatolo Citation1992).
It is desirable that delivery systems be biodegradable and nontoxic. In addition, tolerance toward additives, stability over a wide range of temperatures, low viscosity, small size, and ease of elimination from the body also are required for therapeutic application. Moreover, the size of the encapsulated particles needs to be controlled to avoid blockage of capillaries; hence, submicron-sized delivery systems are preferable for parenteral use (Kreuter Citation1994). Since microemulsions satisfy most of these criteria, and also because of their thermodynamic stability, optical clarity, and ease of preparation, considerable interest has been generated over the years to develop them as drug delivery systems. Thus, nontoxic and biocompatible amphiphiles are used to prepare “biological microemulsions,” in which vegetable oils (Acharya, Sanyal, and Moulik Citation2001a), fatty acid esters (Acharya, Sanyal, and Moulik Citation2001b), and triglycerides (Corswant et al. Citation1998) are used instead of aliphatic oils. Encapsulation of water insoluble drugs in the nanometer-sized oil droplets of the oil-in-water microemulsion might lead to its dissolution into aqueous phase in vivo resulting in better absorption.
The present work describes, for the first time, the preparation and physicochemical characterization of a stable o/w microemulsion composed of clove oil, Tween-20, and water. Clove oil, obtained from the spice plant Eugenia caryophyllata, and its main constituent, eugenol, both are used in pharmaceutical preparations, particularly to treat dental problems (Selvi and Niranjali Citation1998; Jadhav et al. Citation2004) Further, the application of this microemulsion also was explored to encapsulate a model drug, diospyrin, which is a highly lipophilic bioactive compound derived from an indigenous plant (Hazra et al. Citation1984). Presently, diospyrin, a bisnaphthoquinonoid, has emerged as a potential “lead molecule” for development of new drugs against cancer (Chakraborty et al. Citation2002), tuberculosis (Lall et al. Citation2003), and several parasitic diseases like visceral and cutaneous leishmaniasis (Ray et al. Citation1998; Hazra et al. Citation2002), which are causing major public health problems worldwide. Incidentally, the preparation of o/w microemulsion for delivery of another lipophilic drug, amphotericin B also used in the treatment of several types of leishmaniasis, recently has been reported (Moreno et al. Citation2001). In our present study, application of the clove oil-in-water microemulsion was examined as a delivery system in terms of its single-dose toxicity in vivo and compared with the dimethylsulfoxide formulation generally used for the delivery of lipophilic compounds in biological experiments.
MATERIALS AND METHODS
Clove oil was purchased from SD Fine Chemicals, India, with the following specifications (Gupta, Mukhopadhya, and Moulik Citation1995); acid value = 5.12 mg KOH/g; saponification value = 5.23 mg KOH/g; iodine value = 88.34% I2; eugenol content 80%. The surfactant polyoxyethylene sorbitan monolaurate (Tween-20), cholesterol, dipalmitoyl phosphatidyl choline (DPPC), and dimethylsulfoxide (DMSO) were from Sigma Chemicals (USA).
Diospyrin was isolated from the stem-bark of Diospyros montana Roxb., and purified as described earlier (Hazra et al. Citation1984). Its chemical structure is presented in ). Spectroscopically pure chloroform, sodium pyruvate, 2, 4-dinitrophenyl hydrazine, α -ketoglutaric acid, and DL-alanine were purchased from SISCO Research Laboratories (India). p-Nitrophenylphosphate (PNPP) was a product of Loba Chemicals India, and diethanolamine was purchased from Merck, India. Doubly distilled water was used in all aqueous preparations.
Methods
Construction of Ternary Phase Diagram
To construct the phase diagram of the pseudoternary system of clove oil, Tween-20, and water, the amphiphile was dissolved in clove oil in different ratios in several stoppered test tubes and they were placed in a constant temperature water bath. To each of these samples, water was added from a micropipette under constant stirring condition until the solution became just turbid due to phase separation. The measurements were checked for reproducibility, and the compositions were expressed in weight percent to construct the phase diagram on triangular coordinates ().
Selection of Vehicle Composition
For the encapsulation of diospyrin, a weight percent composition of clove oil/Tween-20/ water as 5/30/65 (V1) was selected from the oil-in-water region of the pseudoternary phase diagram marked as S in . Two other modifications of the delivery system were prepared: a DPPC (V2) and a mixture of DPPC and cholesterol (V3), respectively ().
TABLE 1. Composition of drug delivery system before and after encapsulation of diospyrin
Encapsulation of Diospyrin in Microemulsion
First, 1.5 mg and 1.0 mg of diospyrin were dissolved separately in two portions of clove oil (0.25 ml each) and allowed to stand overnight to ensure complete dissolution. To each of the preparations, Tween-20 (1.5 ml) was added followed by water (3.25 ml). The compositions were thoroughly mixed in a vortex mixer to obtain the encapsulated samples (DV1A and DV1B, respectively). Similarly, diospyrin (1.5 mg each) also was incorporated in V2 and V3 to produce DV2 and DV3, respectively. The compositions of the delivery systems before and after incorporation of diospyrin are given in . The final concentration of diospyrin in DV1A and DV1B amounts to 0.3 and 0.2 mg/ml, respectively.
Diospyrin Dispersion in Dimethyl Sulphoxide
A dispersion (Dp) of diospyrin in DMSO was prepared by maceration of the compound with a droplet of Tween-20. A calculated volume of DMSO was used to prepare Dp to deliver the requisite dose of diospyrin in vivo to compare its effect with the same amount of the drug delivered through its microemulsion formulation (DV1). The vehicle (DMSO + Tween-20) also was used as a “control” in the biological studies presented in and .
TABLE 2. Single dose acute toxicity, in Swiss mice, of a microemulsion vehicle (V1), diospyrin encapsulated in the same vehicle (DV1A and DV1B), and diospyrin dissolved in DMSO with the help of Tween−20™(Dp)
TABLE 3. Estimation of the activity of alkaline phosphatase (AP) and glutamate pyruvate transaminase (GPT) in blood serum of Swiss A mice collected 24 hr after treatment
Characterization of the Delivery System
The empty as well as the drug-encapsulated microemulsions—V1, V2, V3, DV1A, DV1B, DV2, and DV3—were characterized in terms of macroscopic appearance, particle size, surface tension, and pH. Their color, isotropy, and homogeneity were examined visually, as well as by cross-polarized light at room temperature. The droplet size distribution of the oil phase and the polydispersity of empty and drug-loaded vehicles, as well as their modifications, were determined by dynamic light scattering (DLS). Ultraviolet-visible spectra of diospyrin dissolved in a chloroform solution, as well as preparations DV1A, DV2, and DV3, were recorded.
The pH of the delivery system with and without diospyrin was determined in triplicate at room temperature using a global digital pH meter. The pH was maintained at 7.0 by dilute NaOH. The surface tension of the drug delivery vehicle as well as the drug-loaded vehicle was determined with a calibrated du Nouy Tensiometer (Kruss, Germany) by the platinum ring detachment technique. The results were accurate within ± 0.1 mN m−1. The surface tension of V1 and DV1A was 29.5 mNm−1.
DLS Measurements
The dimensions of the nano-dispersions were measured in a DLS spectrophotometer (Model DLS 700, Otsuka Electronics, Japan), using a He-Ne laser of wavelength 632.8 nm (Moulik et al. Citation1999). The sample tube was placed in the thermostated chamber of the goniometer, and the scattered intensity was monitored at 90°. The autocorrelation function of the scattered intensity was obtained from a 1024-channel photon correlator. The experimental solutions were filtered (three to four times) through Millipore filters (0.45 μ m) to remove the extraneous particles. The results of measurements for each sample were processed using the instrument's software to obtain the hydrodynamic diameter (dh), the polydispersity index (PDI), and the diffusion coefficient (D) of the dispersed droplets. The measurements were repeated five times (, ).
FIG 3. Particle size distribution of the drug delivery system (V1A) of weight percent composition clove oil/Tween-20/water (5/30/65).
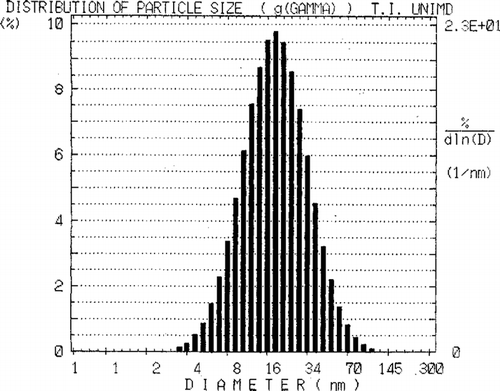
TABLE 1b. Dynamic light scattering data for empty and drug-loaded microemulsion of clove oil/Tween-20™/water (5/30/65)
Spectroscopic Measurement
The individual spectra of clove oil, 0.25M Tween-20™ (concentration same as that in the drug delivery vehicle), and the compositions DV1A, DV2, and DV3 (concentration of diospyrin 0.16 mM) were recorded in a Shimadzu (model 1601A) ultraviolet-visible spectrophotometer ( and ). The measurements were repeated three times to check reproducibility. The biochemical studies were undertaken using Ultrospec 2000 ultraviolet-visible spectrophotometer (Pharmacia Biotech, Sweden).
FIG 5. Visible spectra of clove oil, Tween-20, and diospyrin encapsulated in different delivery systems. (Insets; a = clove oil; b = 0.25 mM Tween-20, c = DV1A, d = DV2, e = DV3.) Concentration of diospyrin for all the spectral studies were 0.16 mM. The error in measurement is ± 1.5% that falls within size of the symbols used.
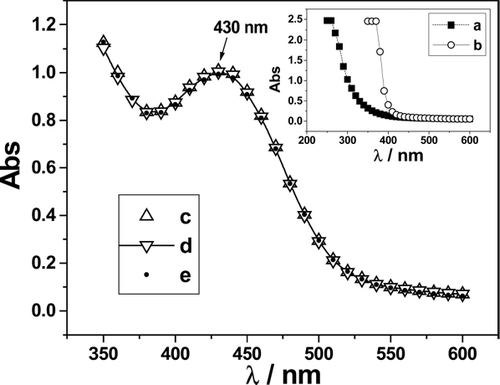
Stability Studies
The drug delivery vehicles, with and without diospyrin, were found to be physically very stable; no phase separation, appearance of haziness or turbidity, and change of color were observed even after 1 year. The stability was examined in the temperature range of 4–40°C.
Water Tolerance
All the empty and drug-loaded delivery systems were tested for dilution effect. The formulations were found to remain stable on dilution up to 60% with water.
Biological Studies
Single-Dose Acute Toxicity Studies
The study was started with two groups of Swiss A mice (body weight 20 g; 10 per group) that were administered intraperitoneally (i.p.) by a single bolus injection of 0.05 ml and 0.1 ml of V1, respectively. As the lower dose was found to be well tolerated, the injection volume was fixed at 0.05 ml for all the treatments carried out with Dp, DV1A, and DV1B. Control experiments with vehicles, DMSO and V1, also were performed similarly. The mortality occurring within 24 hr was recorded as a measure of acute toxicity of the treatment protocols used, and the survival was followed up to 30 days. All the experiments were repeated three times for confirmation, and the results (mean of three observations ± standard deviation) are presented in as percentages of the mortality and survival of experimental mice.
Biochemical Study
Another set of experiments was set up as above with 5 mice in each group and treated in the same way. The mice were sacrificed after 24 hr to collect blood serum from each mouse for estimation of the following liver function enzymes (Bergmeyer and Gawehn Citation1974).
Serum Alkaline Phosphatase (AP)
A buffered substrate solution of PNPP (1.25 mM; pH 9.8) was prepared by mixing with diethanolamine (0.1M). 0.4 ml of this solution and incubated with 10 μl of serum sample at 25°C for 30 min. Sodium hydroxide solution (0.05 N; 2 ml) was added and the absorbance was measured at 420 nm. The activity was determined from the standard curve of p-nitrophenol in terms of PNPP hydrolyzed per minute.
Serum Glutamate Pyruvate Transaminase (GPT)
A buffered substrate solution (pH 7.4) was prepared by dissolving 2 mM α -ketoglutaric acid and 0.2 M DL-alanine in 0.1 M phosphate buffer. A chromogen solution was prepared with 2,4-dinitrophenyl hydrazine (1 mM dissolved in 1N HCl) and sodium pyruvate (2 mM). The serum sample was added to buffered substrate solution (1 ml) and incubated at 37°C for 30 min. The chromogen solution (1 ml) was added to this mixture and allowed to stand at room temperature for 20 min, followed by addition of sodium hydroxide (0.4 N; 10 ml). The absorbance at 520 nm was noted after 5 min, and the activity was calculated from the standard curve of pyruvate solution and expressed in terms of pyruvate formed per minute.
RESULTS AND DISCUSSION
Phase Behavior of the Ternary System
In the present work, clove oil was used as the internal phase because diospyrin was soluble in clove oil. A nearly symmetrical phase boundary has been observed with 43% amphiphile at its maximum (). The clear “pseudomonophasic” microemulsion zone covered 48% of the total area of the triangle. The compositions falling under the curve were found to be macroscopically biphasic. To obtain a good oil-in-water zone for microemulsion, amphiphiles with high hydrophilic–lipohilic balance (HLB) values were chosen (the HLB value of Tween-20 is 16.7). A large clear zone was obtained without the addition of a cosurfactant, making the composition simple. Presence of a cosurfactant is undesirable for pharmaceutical application (Baroli et al. Citation2000). Enhanced solubility of steroids in three component microemulsion systems of soyabean oil, polyethylene ether surfactants, and water also is reported (Malcomson and Lawrence Citation1993, Citation1995). The majority of such pharmaceutical microemulsions use a large number of constituents and hence suffer from the complexity of their compositions (Trotta et al. Citation1995, Citation1999).
Droplet Size
In addition to composition, bioacceptability of the delivery system also is influenced by the particle size. The size of the dispersed clove oil droplets ranges between 9–20 nm (). The nano-droplets with drug were nearly 2-fold larger than those without drug. A representative size distribution observed by the DLS method for the sample V1A is illustrated in . The broadness of the distribution speaks in favor of polydispersity (PDI = 0.369). Their diffusion coefficients were inversely proportional to the droplet size. Because the diameter of the dispersed clove oil droplets of the present microemulsion system is much smaller than the smallest blood capillary (400 nm), the chances of capillary blockage during transport of the vehicle should be minimum (Kreuter Citation1994). A small size distribution of the vehicle is favorable for higher circulation time (T1/2) after in vivo application. Park and Kim (Citation1999) reported micro-emulsion system of ethyl oleate, Tween-20, and water with droplet diameter of ∼100 nm. The mean droplet diameter of betamethasone encapsulated microemulsion composed of isopropyl myristate, aerosol OT, butanol, and water ranged between 20–30 nm (Trotta et al. Citation1990).
Diospyrin Encapsulation and Stability
Microemulsions prepared with nonionic surfactants are characteristically sensitive to temperature variations (Oh et al. Citation1995), whereas the present system was quite stable in the temperature range of 4–40°C. An added benefit of microemulsions with nonionic surfactants is their low susceptibility to phase separation with variations in ionic strength and pH (Constantindes Citation1995) which are likely to be encountered in the biological environment. Therefore, the chances of precipitation of the drug due to phase separation would be less during transport through the blood vessels.
The present systems of V1, DV1A, and DV1B have been observed to tolerate 60% of water uptake. The droplet structure of o/w microemulsion is often retained on dilution (Constantinides Citation1995). However, the process of dilution results in gradual desorption of surfactant located at the droplet interface. The process is thermodynamically driven by the requirement of surfactant to maintain an aqueous phase concentration equivalent to its critical micelle concentration (cmc) under the prevailing condition of temperature, pH, and ionic strength. Because nonionic surfactants typically have lower cmcs, the present o/w microemulsion formulation is likely to offer superior in vivo stability. As phosphatidylcholine and cholesterol exist in the body in major amounts, a good acceptability may be expected when these substances are used as excipients for the delivery system (as in liposomes).
Phospholipids are used in a number of microemulsions meant for pharmaceutical applications, but in all such preparations, addition of cosurfactant is needed (Trotta et al. Citation1990, Citation1995). Cortesi et al. (Citation1997) observed enhanced solubility of camptothecin in microemulsion (comprising Labrasol®, plurol isostearate, isostearyl isostearate and water) over that in water. The drug encapsulated formulation was stable for 30 days whereas diospyrin encapsulated microemulsion was stable over one year. A pseudoternary system of ethyl oleate, Tween-20, and isotonic saline was reported as a parenteral drug carrier for the poorly soluble drug flurbiprofen (Park and Kim Citation1999).
Spectral Features
The ultraviolet-visible spectra of diospyrin in CHCl3 produced a sharp peak at 250 nm and a broad peak at 425 nm (). The spectra of both clove oil and Tween-20 in water (inset ) produced intense and sharply increasing absorbance at wavelength <400 nm. The sharp peak of diospyrin at 250 nm was thus masked in the o/w microemulsion by the contributions from the oil and the amphiphile. The absorption spectrum of the drug in DV1, DV2, and DV3 (depicted in ) has the same electronic transition with the broad maximum at 430 nm as has been observed for diospyrin in CHCl3. In the compartmentalized condition, the spectral patterns of the drug with and without the additives (cholesterol and DPPC) were the same indicating that the additives did not alter the spectral feature of the encapsulated drug.
Single Dose Acute Toxicity Studies
The survival data of mice treated i.p. with a single dose of diospyrin delivered either through DMSO (Dp) or the microemulsion (DV1A and DV1B), along with the appropriate “vehicle control” groups, are presented in . The vehicle V1 was found to be safe for i.p. injection at a volume of 0.05 ml. The doses of diospyrin in Dp and DV1 were chosen to be in the same range (0.5–0.75 mg/kg) as done previously for treatment of tumor-bearing mice (Hazra et al. Citation1984). V1A and V1B were found to be reasonably well tolerated in i.p. treatment at the dose used in the present study. Performing enzyme assays on the aforesaid groups of mice also supported this. As shown in , the treatments did not produce any deleterious effect on the liver function enzymes.
CONCLUSION
Clove oil/Tween-20/water could form stable o/w microemulsions with droplet dimensions of 9–20 nm that could favorably encapsulate the oil-soluble quinonoid compound, diospyrin. From the study of the single dose acute toxicity of the microemulsion vehicle, we found that it was reasonably tolerated by Swiss mice when delivered i.p. at a dose of 0.05 ml, without and with incorporation of diospyrin. The vehicle did not impart any hepatotoxicity at this dose as we found from the assay of liver function enzymes carried out on the blood sera collected from the experimental mice. Eugenol, which constitutes ∼ 80% of clove oil, is regarded as a safe food–flavoring agent, and the acceptable limit for human intake given by the Food and Drug Administration is 2.5 mg/kg (IARC Citation1985, FAO/WHO Citation1982). In the microemulsion composition presented here, the clove oil delivered per dose in vivo was 0.125 mg/kg. The biological acceptability of nonionic surfactants has been reported (Attwood Citation1994). The amount of Tween-20 used in the treatment regimen (0.8 mg/kg) also was considerably less than the limit of 25 mg/kg approved by FAO and WHO (FAO/WHO Citation1974). Hence, the feasibility of the above microemulsion formulations as a drug delivery system for compounds that are insoluble in water but soluble in clove oil appears to be promising. Further, the prospect of its application through routes other than i.p. remains to be investigated.
S. Gupta, S. K. Sanyal, and S. Datta thank the Department of Science and Technology, Government of India, for financial support (research grant no. SP/S1/H-31/99) and S. P. Moulik thanks the University Grants Commission for an Emeritus Fellowship. B. Hazra is grateful for a Research Grant [No.58/4/2000/BMS] from the Indian Council of Medical Research, New Delhi.
REFERENCES
- Acharya A., Sanyal S. K., Moulik S. P. Formation and characterization of an useful biological microemulsion system using mixed oil (ricebran oil and isopropyl myristate), polyoxyethylene(2) oleyl (Brij–92), isopropyl alcohol and water. J. Disp. Sci. Technol. 2001a; 22: 551–561, [CSA]
- Acharya A., Sanyal S. K., Moulik S. P. Formation and characterization of a pharmaceutically useful microemulsion derived from isopropyl myristate, polyoxyethylene (4) lauryl ether (Brij-30), isopropyl alcohlo and water. Current Sci. 2001b; 81: 362–370, [CSA]
- Attwood D. Microemulsion. Colloidal Drug Delivery Systems, J. Kreuter. Marcel Dekker, New York 1994
- Bergmeyer H. U., Gawehn K. Methods of Enzymatic Analysis. Academic Press, New York 1974
- Baroli B., Lopez-Quintela M. A., Delgado-Charro M. B., Fadda A. M., Blanc Mendez J. Microemulsions for topical delivery of 8-methoxsalen. J. Control Release 2000; 69: 209–218, [PUBMED], [INFOTRIEVE], [CSA], [CROSSREF]
- Chakrabarty S., Roy M., Hazra B., Bhattacharya R. K. Induction of apoptosis in human cancer cell lines by diospyrin, a plant-derived bisnaphthoquinonoid, and its synthetic derivatives'. Cancer Lett. 2002; 188: 85–93, [PUBMED], [INFOTRIEVE], [CSA], [CROSSREF]
- Constantinides P. P. Lipid microemulsions for improving drug dissolution and oral absorption: physical and biopharmaceutical aspects. Pharm. Res 1995; 12: 1561–1572, [PUBMED], [INFOTRIEVE], [CSA], [CROSSREF]
- Cortesi R., Esposito E., Maietti A., Menegatti E., Nastruzzi C. Formulation study of the antitumor drug camptothecin: liposomes, micellar solution and microemulsion. Int. J. Pharm 1997; 159: 95–103, [CSA], [CROSSREF]
- Cortesi R., Nastruzzi C. Liposomes, micelles, and microemulsions as new delivery systems for cytotoxic alkaloids. Pharmaceut. Sci. Technol. Today 1999; 2: 288–298, [CSA], [CROSSREF]
- Corswant Von C., Thorem P., Engstrom S. Triglyceride base microemulsion for intravaneous administration of sparingly soluble substance. J. Pharm. Sci. 1998; 87: 200–208, [CSA], [CROSSREF]
- FAO. Evaluation of food additives and contaminants. 1982, Technical Report Series No.20.FAO/WHO Expert Committee on Food Additives
- FAO/WHO. Toxicological evaluation of general principles and of specifications. 1974; 539, 17th Report of the joint FAO/WHO Expert Committee on Food additives. Technical Report Series World Health Organization.No
- Gregoriadis G. Liposome. Technology, 2nd ed. CRC, Boca Raton, FL 1993; vol 1
- Gupta S., Mukhopadhya L., Moulik S. P. Activity of alkaline phosphatase in water-in-oil microemulsion containing vegetable oil. Ind. J. Biochem. Biophys. 1995; 32: 261–265, [CSA]
- Hazra B., Golenser J., Nechemiya O., et al. Inhibitory activity of diospyrin derivatives on Leishmania major parasites in vitro. Ind. J. Pharmacol. 2002; 34: 422–427, [CSA]
- Hazra B., Sur P., Roy D. K., et al. Biological activity of diospyrin owards Ehrlich carcinoma in Swiss A mice. Planta Med. 1984; 51: 295–297, [CSA]
- IARC Monographs. LyonFrance 1985; 75–78
- Jadhav B. K., Khandelwal K. R., Ketkar A. R., Pisal S. S. Formulation and evaluation of mucoadhesive tablets containing eugenol for the treatment of periodontal diseases. Drug Dev. Ind. Pharm. 2004; 30: 195–203, [PUBMED], [INFOTRIEVE], [CSA], [CROSSREF]
- Kantaria S., Rees G. D., Lawrence M. J. Geletin based organogels: rheology and application in ionophoretic transdermal drug delivery. J. Control Rel. 1999; 60: 355–365, [CSA], [CROSSREF]
- Kreuter J. Nanoparticles. Colloidal Drug Delivery Systems, J. Kreuter. Marcel Dekker, New York 1994
- Lall N., Hazra B., Das Sarma M., Meyer J. J. M. In vitro activity of derivatives of diospyrin against drug—resistant and drug—sensitive strains of Mycobacterium tuberculosis. J. Antimicrob. Chemother. 2003; 51: 435–438, [PUBMED], [INFOTRIEVE], [CSA], [CROSSREF]
- Lawrence M. J. Microemulsions as drug delivery vehicles. Curr. Opin. Coll. Interf. Sci. 1996; 1: 826–832, [CSA]
- Malcomson C., Lawrence M. J. A comparison of the incorporation of the model steroids into non-ionic micellar and microemulsion systems. J. Pharm. Pharmacol. 1993; 45: 141–143, [CSA]
- Malcomson C., Lawrence M. J. Three component non-ionic oil-in-water microemulsions using polyethylene ether surfactants. Coll Surf B: Biointerf. 1995; 4: 97–109, [CSA], [CROSSREF]
- Moreno M., Frutos Ballesteros M., et al. Release of nortriptyline hydrochloride from oil-in-water microemulsion. Chem. Pharm. Bull. 2000; 48: 1623–1627, [PUBMED], [INFOTRIEVE], [CSA]
- Moreno M. A., Frutos P., Ballesteros M. P. Lyophilized lecithin–based oil-in-water microemulsions as a new and low toxic delivery system for amphotericin B. Pharm. Res. 2001; 18: 344–351, [PUBMED], [INFOTRIEVE], [CSA], [CROSSREF]
- Moulik S. P., Dey G. C., Bhowmik B. B., Panda A. K. Physicochemical studies in microemulsion VI: dynamics and thermodynamics of percolation of water/AOT/n-heptane in presence of sodium cholate and salicylate. J. Phys. Chem. B. 1999; 103: 7122–7129, [CSA], [CROSSREF]
- Oh K. H., Baran J. R., Wade W. H., Weerrasooriy A. V. Temperature insensitive microemulsion phase behavior with nonionic surfactants. J. Disp. Sci. Technol. 1995; 16: 165–188, [CSA]
- Park K. M., Kim C. K. Preparation and evaluation of flurbiprofen loaded microemulsion for parenteral delivery. Int. J. Pharm 1999; 181: 173–179, [PUBMED], [INFOTRIEVE], [CSA], [CROSSREF]
- Paul B. K., Moulik S. P. Microemulsions: an overview. J. Disp. Sci. 1997; 18: 301–318, [CSA]
- Ray S., Hazra B., Mitra B., et al. Diospyrin, a bisnaphthoquinone: a novel inhibitor of type I DNA topoisomerase of Leishmania donovani. Mol. Pharmacol. 1998; 54: 994–999, [PUBMED], [INFOTRIEVE], [CSA]
- Sarciaux M. J., Alan L., Sado P. A. Using microemulsion for drug delivery of therapeutic peptides. Int. J. Pharm. 1995; 12: 127–136, [CSA], [CROSSREF]
- Selvi R. T., Niranjali S. Inhibition by eugenol of diethylnitrosamine-induced microsomal degranulation. Fitoterapia 1998; 69: 115–117, [CSA]
- Sinha J., Ray B., Medda S., et al. Bacosasaponin C: critical evaluation of antileishmanial properties of various delivery modes. Drug Del. 2002; 9: 55–62, [CSA], [CROSSREF]
- Swenson E. C., Curatolo W. J. Intestinal permeability enhancement for proteins, peptides and other polar drugs:mechanism and potential toxicity. Adv. Drug Del. Rev. 1992; 8: 39–42, [CSA], [CROSSREF]
- Tenjarala S. Microemulsion: an overview and pharmaceutical application. Crit. Rev. Therap. Drug Carrier Sys. 1999; 16: 461–521, [CSA]
- Trotta M. Influence of phase transformation on indomethicin release from microemulsion. J. Control Rel. 1999; 60: 399–405, [CSA], [CROSSREF]
- Trotta M., Gallarate M., Pattarino F., Carlotti M. E. Investigation of phase behavior of systems containing lecithin and 2-acyl lysolecithin derivatives. Int. J. Pharm. 1999; 190: 83–89, [PUBMED], [INFOTRIEVE], [CSA], [CROSSREF]
- Trotta M., Gasco M R., Pattarini F. Diffusion of steroid hormones from o/w microemulsions:influence of the cosurfactant. Acta Pharm. Technol. 1990; 36: 226–234, [CSA]
- Trotta M., Ugazio E., Gasco M. R. Psedoternary phase diagram of lecithin- based microemulsions: influence of monoalkylphosphates. J. Pharm. Pharmacol. 1995; 47: 451–454, [PUBMED], [INFOTRIEVE], [CSA]
- Uchegbu I. F., Florence A. T. Non-ionic surfactant vesicles (niosomes): physical and pharmaceutical chemistry. Adv. Coll. Interf. Sci. 1995; 58: 1–55, [CSA], [CROSSREF]
- Watnasirichaikul S. S., Davies N. M., Rades T., Tucker I. G. Preparation of biodegradable insulin nanocapsules from biocompatible microemulsions. Pharm. Res. 2002; 17: 684–689, [CSA], [CROSSREF]