Abstract
Recent studies have implicated the cytokine tumor necrosis factor-alpha (TNF-α) in the inflammation associated with Crohn's disease (CD). Thalidomide has been shown to decrease this inflammation by the suppression of TNF-α secretion. However, side effects associated with thalidomide have precluded its widespread usage. In the present study we investigated the efficacy of a “targeted delivery approach” for thalidomide at the site of inflammation. We observed that alginate-poly-l-lysine-alginate (APA) polymer-based microcapsule formulations that encapsulate thalidomide could be designed. These capsules could be delivered at target sites where they almost entirely suppress TNF-α secretion in lipopolysaccharide activated RAW 264.7 macrophage cells in vitro. These findings indicate that targeted delivery of thalidomide using APA capsules could facilitate its usage in reducing the inflammation associated with chronic conditions such as Crohn's disease and ulcerative colitis.
Inflammatory bowel diseases (IBD) such as Crohn's disease (CD) are common chronic diseases especially affecting “more affluent” populations (Karlinger et al. Citation2000; Tragnone et al. Citation1996). Although substantial progress has been made in determining therapeutic strategies, presently no single treatment is effective. Heterogeneity in disease presentation, variability in disease course, and potential steroid resistance has prompted research into other treatment modalities. Tumor necrosis factor-alpha (TNF-α), a proinflammatory cytokine secreted from cells such as macrophages in the immune system, has been implicated in CD-related inflammation (Sandborn Citation2003; van Deventer Citation1997; Wichan, Chojnacki, and Wojtun Citation2004). Several studies, both in vivo and vitro, have demonstrated the anti-TNF therapeutic potential of thalidomide (Ehrenpreis et al. Citation1999; Gardner-Medwin et al. Citation1994; Keifer et al. Citation2001; Mazzon et al. Citation2005; Ochonisky et al. Citation1994; Sabate et al. Citation2002; Sampaio et al. Citation1991; Vasiliauskas et al. Citation1999). Notably, recent clinical trials and/or clinical studies have shown that thalidomide is effective in reducing the inflammation associated with CD (Caprilli et al. Citation2005; Mazzon et al. Citation2005; Mutlu, Farhadi, and Keshavarzian Citation2002; Ehrenpreis et al. Citation1999; Odeka and Miller Citation1997; Vasiliauskas et al. Citation1999; Wettstein and Meagher Citation1997). The anti-inflammatory effects of the drug are thought to be due to its capacity to regulate TNF-α mRNA degradation through inhibition of specific transcription factor activities (Moreira et al. Citation1993). In spite of its potential efficacy in the treatment of CD-associated inflammation, the risks for systemic toxicity and adverse effects (such as teratogenecity and neuropathy) associated with its use preclude its long term usage.
In the present study, we investigated the use of artificial cell alginate-poly-lysine-alginate (APA) polymeric microcapsules for targeted oral delivery of thalidomide for possible therapeutic applications in CD and other diseases. Artificial cell APA microcapsules previously have been successfully used for encapsulation and targeted delivery purposes based on their suitability and biocompatibility (Chang Citation1999, Citation2005; Ma, Vacek, and Sun Citation1994). The specific objectives of our study were to design APA membrane thalidomide formulations, and to test the capsule's targeted drug delivery efficiency and performance in vitro in simulated gastrointestinal (GI) conditions.
MATERIALS AND METHODS
Alginic acid (low viscosity, MW 240,000), dimethyl sulfoxide, DMSO, MW 78.13), thalidomide (MW 258.2), Escherichia coli 055:B5 lipopolysaccharides were purchased from Sigma-Aldrich, (Canada). Polyoxyethylene (Tween 20) and sorbitan monolaurate was purchased from VWR, (Canada). The RAW 264.7 mouse macrophage cells, Dulbecco's Modified Eagle's medium, and fetal bovine serum used were purchased from American Type Culture Collection (USA).
Preparation of Microcapsule Thalidomide Formulation
To prepare APA microcapsule thalidomide formulation, alginic acid was added to deionized water to make a 1.5% alginate solution. Drug thalidomide ((±)-2-(2,6-dioxo-3-piperidinyl)-1H-isoindole-1,3(2H)-dione) (Sigma) was dissolved in dimethyl sulfoxide and diluted with deionized water. Then 1 ml of solution containing 0.7 mg of thalidomide was added to the alginate solution. Alginate solution was added to maintain a 1.5% concentration of thalidomide. APA microcapsules were then formed by running the solution through an Inotech (Inotech Inc. Rockville, MD, USA) encapsulator pump using a 300 μ m nozzle. The encapsulator frequency was set to 528 Hz, the flow rate to 20.8 ml/min, and the voltage to 0.348 kV. Formed beads were collected in a prepared 0.1 M calcium chloride solution to avoid cell aggregation. The beads were then washed with deionized water and soaked in a 0.1% poly-l-lysine (Sigma) bath for 10 min. Beads were washed again and soaked in 0.15% alginate solution for 15 min. Final washing was done with water and the beads stored.
Macrophage Cell Culturing
Mouse RAW 264.7 macrophage cells were cultured according to standard culture procedures using Dulbecco's Modified Eagle's Medium (purchased from ATCC, supplemented with 10% fetal bovine serum and 0.1% penicillin streptomycin antibiotic (Caputo Citation1988; Center for Disease Control Citation1993; Fleming et al. Citation1995; Hay, Caputo, and Macy Citation1992; Ralph and Nakoinz Citation1977)). The cells were incubated in a 37°C and 5% CO2 environment in a Sanyo MCO-18M oxygen/carbon dioxide incubator.
TNF-α Secretion by Macrophage Cells
Lipopolysaccharide (LPS) from E. coli 055:B5 dilutions of 0.1, 1, 10, and 100 μ g/mL in autoclaved PBS solution were prepared to stimulate 264.7 macrophage cells. Standard TNF-α secretion experiments were performed by adding 0.23 mL media solution containing 300,000 264.7 macrophage cells to wells within a Falcon Brand 24-well flat bottom tissue culture plate. Then 0.23 mL of each of the four LPS dilutions were added and allowed to incubate in the individual wells containing the media and cells. Six samples of each of the four dilutions were prepared for sampling at six separate time intervals (0.5, 1, 3, 12, 24, and 48 hr). Control samples containing only cells in media also were prepared. All samples were incubated in a standard 5% CO2 environment. After withdrawing the supernatant from the appropriate wells, these solutions were frozen for storage in a Sanyo MDF-U50V -86°C freezer prior to ELISA analysis.
Testing Delivery of APA Microcapsule Encapsulated Thalidomide
APA microcapsules were initially submerged in 1.5 pH buffer solutions to mimic acidic stomach conditions. These capsules were then added to culture of 264.7 macrophage cells in media of pH 7.4. The cells were stimulated using LPS using suitable quantities of cells and LPS solution as described above. Similar amounts of APA capsules (∼ 0.116 g) were added to the culture plate wells by weighing the samples. Samples of LPS, cells in culture media, and APA beads were incubated in a 5% CO2 environment and withdrawn at 0.5, 1, 3, 12, 24, and 48 hr. Supernatant from the appropriate wells were withdrawn and analyzed using ELISA. Samples, when required, were stored in a Sanyo MDF-U50V -86°C freezer.
TNF-α ELISA Analysis
To detect TNF-α secretion by mouse 264.7 macrophage cells, an ELISA test was performed using an EBioscience Mouse TNF-alpha ELISA Ready-SET-Go¡ kit. For the latter Nunc Maxisorb 96 well plates were coated with capture antibody and incubated at 4°C overnight. Wells were then manually washed three times using PBS containing 0.05% Tween-20, and then aspirated. Assay diluents were then added to each well and allowed to incubate for 1 hr at room temperature. Manual washing was repeated thrice, plates were aspirated, and then 8 concentrations of 2-fold serial dilutions of the included top standard of 1000 pg/ml were added to the appropriate wells. Samples of supernatant were spun in a NAPCO 2028R centrifuge for 5 min at 1000 rpm to separate the remaining cells. These samples were then added to the appropriate wells. The plates were sealed and incubated at room temperature for 2 hr Plates were washed manually five times with PBS before aspiration and detection antibodies were added to the plates. The plates were again sealed and incubated for 1 hr at room temperature.
Subsequently, wells were washed five times using PBS, aspirated, and Avidin-HRP enzyme was added. The plates were then sealed and incubated at room temperature for 30 min. Wells were washed seven times using PBS that was allowed to soak in each well for 1–2 min before plate aspiration. Substrate solution was added to the wells and allowed for reaction for 15 min at room temperature. Finally 2N sulfuric acid was added to wells, as a stop solution for color development and the optical density of the plates measured at 450 nm and 570 nm using a bio-Tek μ Quant universal microplate spectrophotometer.
RESULTS
Experiments were designed to prepare APA microcapsules encapsulating thalidomide for targeted thalidomide delivery. Results show that APA microcapsules containing thalidomide could be prepared. shows the photomicrograph of a 300 μm ± 50 μm size APA microcapsule containing thalidomide. These microcapsule formulations when analyzed by light microscopy for membrane integrity, stability, and uniformity. Results indicate no changes in APA capsule morphology because of encapsulation of thalidomide ().
FIG. 1 Photomicrographs of freshly prepared APAmicrocapsule thalidomide formulation (size 300 μm ± 50 μm, ×250).
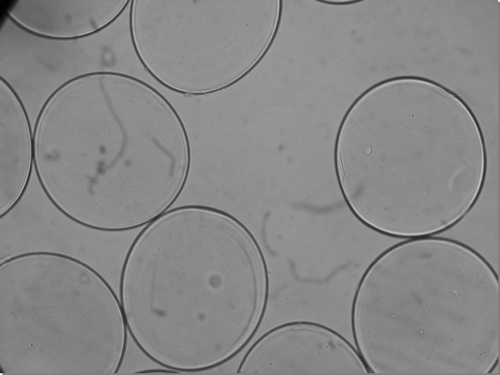
Experiments were designed to test APA thalidomide delivery on the suppression of TNF-α secretion. summarizes TNF-α ELISA standard curve; a linear graph with an R2 value of 0.9916 was generated. To test APA thalidomide delivery, 0.116 g of APA microcapsules containing 1.5 μ g of thalidomide were exposed to 10 μ g/ml LPS-stimulated RAW 264.7 cells and supernatant samples were analyzed for TNF-α content using ELISA and compared with 10 μ g/ml LPS-stimulated RAW 264.7 control macrophage cells. There were no significant changes in TNF-α secretion by control and APA thalidomide exposed cells for the first.05 and 1 hr. Between 12 and 24 hr, TNF levels rose to 80% in both control and APA thalidomide exposed cells. However, after 24 hr, TNF secretion was suppressed significantly to concentrations of 0.056 ng/ml or 5% of control concentrations ().
FIG. 3 The concentration of TNF-α secretion from RAW 264.7 macrophage cells stimulated with 10 μ g/ml of LPS. These values are compared with TNF-α secretion from stimulated RAW 264.7 macrophage cells in the presence of APA encapsulated thalidomide. Comparisons were made after incubation times of 0.5, 1, 3, 12, 24, and 48 hr.
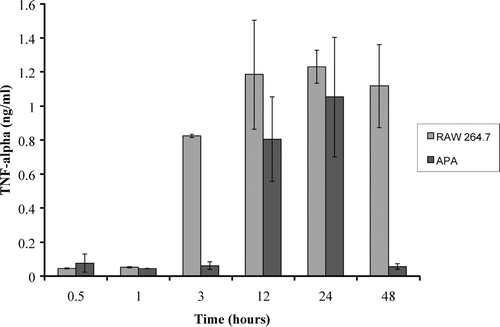
Furthermore, initially TNF-α level remained quite low at concentrations of 0.086 ng/ml or 7% of control levels for up to 24 hr. We also designed experiments with 10 times more LPS simulation of RAW 264.7 macrophage cells. To achieve this, APA capsules containing thalidomide were exposed to RAW 264.7 cells stimulated with 100 μ g/ml of LPS (). We observed that RAW 264.7 macrophage cells TNF-α secretion was delayed in the presence of the microcapsule thalidomide formulation. Cytokine TNF-α concentrations of 0.23 ng/ml, or 24% of control concentrations, were detected after 3 hr incubation and TNF levels rose in a lag-response fashion to 96% of control concentrations ().
FIG. 4 The concentration of TNF-α secretion from RAW 264.7 macrophage cells stimulated with 100 μ g/ml of LPS. These values are compared with TNF-α secretion from stimulated 264.7 macrophage cells in the presence of APA encapsulated thalidomide. Comparisons were made after incubation times of 0.5, 1, 3, 12, 24, and 48 hr.
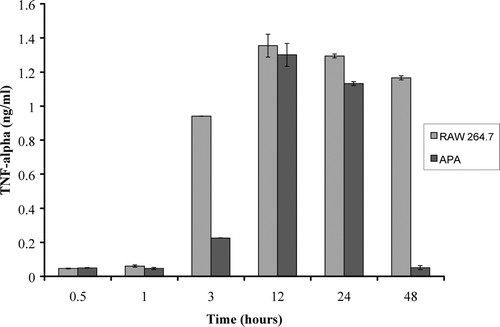
After 24 hr, concentrations of TNF-α decreased to 87% in experimental macrophage cells (APA thalidomide exposed macrophage cells) compared with TNF-α levels of RAW 264.7 control macrophage cells. After 48 hr, secretion of TNF-α was as low as 0.051 ng/ml compared with control macrophage cells (control concentrations of 1.17 ng/ml). Thus, a 96% reduction in TNF-α secretion due to APA thalidomide formulation exposures was observed. Similar trends in TNF-α secretion were observed () in both 10 and 100 μ g/ml LPS-stimulated RAW 264.7 macrophage cells.
FIG. 5 TNF-α secretion from RAW 264.7 macrophage cells in comparison to RAW 264.7 macrophage cells incubated with APA encapsulated thalidomide at time intervals from 0.5, 1, 3, 12, 24, and 48 hr. Turquoise and blue lines in the graph represent cells stimulated with 100 μ g/ml of LPS versus the green and yellow lines that represent cells stimulated with 10 μ g/ml of LPS.
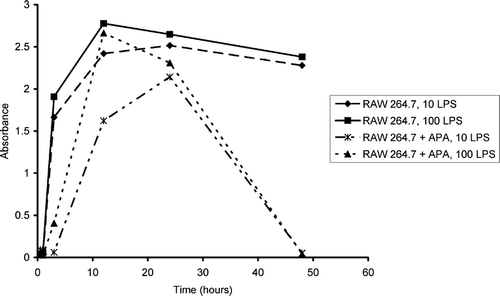
The effects of microcapsule thalidomide exposure to the RAW 264.7 macrophage cells also were studied using microscopic evaluation of control and APA thalidomide exposed macrophage cells. Macrophage cells were observed for growth and morphological differences between standard cultured cells and postincubated macrophage cells with APA encapsulated thalidomide formulations (). Microcapsules remained intact and after exposure, the macrophage cells were identical in shape and had ovular projections like the cells prior to thalidomide exposure but with reduced overall cell size (). When tested for growth, the macrophage cells (after 48 hr exposure with APA capsules containing thalidomide) maintained their ability to grow in Dulbecco's modified Eagle's medium.
FIG. 6 Photomicrographs of RAW 264.7 control macrophage cells and experimental RAW 264.7 macrophage cells after exposure to APA encapsulated thalidomide formulations. (A) Photomicrograph of control macrophages (×200) (B) Experimental: photomicrograph of macrophages after 48 hr exposure to APA microcapsules thalidomide exposers (×200). APA microcapsule thalidomide formulations also are seen in the photomicrograph.
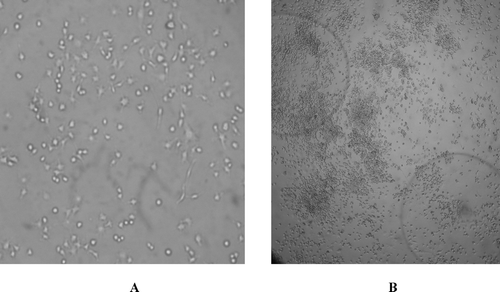
DISCUSSION
Cytokines act as signal transmitters between cells in the immune system creating a response pathway to various external stimuli. From among these TNF-α has been implicated in processes such as inflammation, apoptosis or cell death, and thrombosis. Specifically, this molecule has been implicated in mediating the inflammatory mechanisms associated with inflammatory bowel diseases (IBD), such as CD, by initiating an increase in inflammatory cells to areas of the gut (van Deventer Citation1997). Therapies for treatment of CD thus have concentrated on anti-TNF-α mechanisms. Treatments with monoclonal antibodies, eicosanoids, PPARγ, phosphodiesterase inhibitors, signal transduction inhibitors, TNF-α converting enzyme inhibitors, and thalidomide have been evaluated (van Deventer Citation2002). Thalidomide has shown notable efficacy in clinical trials, but treatment has been associated with side effects leading to patient dropout (Ehrenpreis et al. Citation1999; van Deventer Citation2002). These treatment failures hamper the complete exploration of the potential benefits related to thalidomide use.
In the present study we evaluated a potential local targeted therapy method by encapsulating the drug in artificial cell APA microcapsules. Delivery of the drug was successful and provided a means for decreasing TNF secretion in stimulated RAW 264.7 macrophage cells at two different LPS concentrations: 10 and 100 μ g/ml. Thus, artificial cell microencapsulation of thalidomide could avoid side effects related to the systemic delivery of thalidomide and enhance its usage for the treatment of CD and other diseases. This formulation could allow use of small targeted dosage of thalidomide at the site of inflammation. Further research, specifically on mechanisms whereby thalidomide is being delivered from APA microcapsules and the in vivo preclinical evaluations in experimental CD models, would be required before the full potential of this formulation can be realized.
CONCLUSION
Adequate treatment modalities for CD has been hampered by the inability to determine specific factors that perpetuate and maintain the inflammation. Nevertheless, considerable progress has been made. Treatments focusing on combating the proinflammatory effects of TNF-α have achieved some measure of success in inducing and maintaining remission of the inflammatory process (Aeberli et al. Citation2002; Ehrenpreis et al. Citation1999; Rutgeerts et al. Citation1999). Among the latter, the anti–TNF effects of thalidomide have increased interest in its potential for usage in CD treatment (Gordon and Goggin Citation2003; Podolsky Citation2002). Evidence from open-label clinical trials and other case series suggest that systemic administration of the drug could prove effective in providing pain relief, reducing ulcer formation, and stopping intestinal bleeding (Ehrenpreis et al. Citation1999; Gardner-Medwin et al. Citation1994a; Keifer et al. Citation2001a; Ochonisky et al. Citation1994; Odeka and Miller Citation1997; Sabate et al. Citation2002; Vasiliauskas et al. Citation1999; Wettstein and Meagher Citation1997). However, well-known teratogenecitic and neuropathic effects of the drug could limit usage of the drug for long periods, a pre-requisite for maintaining remission and preventing relapse (Aeberli et al. Citation2002a; Bariol et al. Citation2002; Brocvielle et al. Citation2003; Valero and Gonzalez Citation2002). The initial promise shown by thalidomide could be enhanced if methods to limit systemic administration and simultaneous targeting to inflamed areas in the gut could be designed. The latter could substantially augment the clinical efficacy of the drug and reduce associated side effects.
We have proposed that a therapy may be designed to orally deliver thalidomide using APA microcapsule thalidomide formulation studied in our research. This APA formulation, in in vitro studies, shows up to 96% reduction in TNF-α secretion by mouse macrophage. Our data suggest that microcapsule thalidomide formulation may be an excellent alternative for targeted remission of inflammation in IBD and CD. However, further study is required.
This work was supported by research grants (to S. Prakash) from the Canadian Institute of Health Research. Its New Investigator Award to S. Prakash is acknowledged. H. Chen and T. Haque acknowledge postgraduate scholarship supports from the Natural Sciences and Engineering Research Council of Canada.
REFERENCES
- Aeberli D., Oertle S., Mauron H., et al. Inhibition of the TNF pathway: use of infliximab and etanercept as remission–inducing agents in cases of therapy-resistant chronic inflammatory disorders. Swiss Med. Wkly. 2002; 132(29–30)414–422, [INFOTRIEVE], [CSA]
- Bariol C., Meagher A. P., Vickers C. R., et al. Early studies on the safety and efficacy of thalidomide for symptomatic inflammatory bowel disease. J. Gastroenterol. Hepatol. 2002; 17(2)135–139, [INFOTRIEVE], [CROSSREF], [CSA]
- Brocvielle H., Muret P., Plenat E., et al. Management of a patient receiving thalidomide. Presse Medicale 2003; 32(40)1894–1898, [CSA]
- Caprilli R., Angelucci E., Cocco A., et al. Appropriateness of immunosuppressive drugs in inflammatory bowel diseases assessed by RAND method: Italian Group for IBD (IG-IBD) position statement. Dig. Liver Dis. 2005; 37(6)407–417, [INFOTRIEVE], [CROSSREF], [CSA]
- Caputo J. L. Biosafety procedures in cell culture. J. Tiss. Culture Meth. 1988; 11: 223–227, [CROSSREF], [CSA]
- Center for Disease Control. Biosafety in Microbiological and Biomedical Laboratories, , et al. Human Health Service Publication, Washington, DC 1993; 3, 1
- Chang T. M. Artificial cells, encapsulation, and immobilization. Ann. N. Y. Acad. Sci. 1999; 875: 71–83, [INFOTRIEVE], [CROSSREF], [CSA]
- Chang T. M. Therapeutic applications of polymeric artificial cells. Nat. Rev. Drug Discov. 2005; 4(3)221–235, [INFOTRIEVE], [CROSSREF], [CSA]
- Ehrenpreis E. D., Kane S. V., Cohen L. B., et al. Thalidomide therapy for patients with refractory Crohn's disease: an open-label trial. Gastroenterology 1999; 117(6)1271–1277, [INFOTRIEVE], [CROSSREF], [CSA]
- Fleming D. O., Richardson J. H., Tulis J. J., Vesley D. Laboratory Safety: Principles and Practice. ASM Press, Washington, DC 1995; 2, 1
- Gardner-Medwin J. M., Smith N. J., Powell R. J. Clinical experience with thalidomide in the management of severe oral and genital ulceration in conditions such as Behcet's disease: use of neurophysiological studies to detect thalidomide neuropathy. Ann. Rheum. Dis. 1994; 53(12)828–832, [INFOTRIEVE], [CSA]
- Gordon J. N., Goggin P. M. Thalidomide and its derivatives: emerging from the wilderness. Postgrad. Med. J. 2003; 79(929)127–132, [INFOTRIEVE], [CROSSREF], [CSA]
- Hay R. J., Caputo J. L., Macy M. L. ATCC quality control methods for cell lines. ATCC Qual. Control 1992; 2(1), [CSA]
- Karlinger K., Gyorke T., Mako E., et al. The epidemiology and the pathogenesis of inflammatory bowel disease. Eur. J. Radiol. 2000; 35(3)154–167, [INFOTRIEVE], [CROSSREF], [CSA]
- Keifer J. A., Guttridge D. C., Ashburner B. P., Baldwin A. S., Jr. Inhibition of NF-kappa B activity by thalidomide through suppression of IkappaB kinase activity. J. Biol. Chem. 2001; 276(25)22382–22387, [INFOTRIEVE], [CROSSREF], [CSA]
- Ma X., Vacek I., Sun A. Generation of alginate-poly-l-lysine-alginate (APA) biomicrocapsules: the relationship between the membrane strength and the reaction conditions. Artif. Cells Blood Substit. Immobil. Biotechnol. 1994; 22(1)43–69, [INFOTRIEVE], [CSA]
- Mazzon E., Muia C., Paola R. D., et al. Thalidomide treatment reduces colon injury induced by experimental colitis. Shock 2005; 23(6)556–564, [INFOTRIEVE], [CSA]
- Moreira A. L., Sampaio E. P., Zmuidzinas A., et al. Thalidomide exerts its inhibitory action on tumor necrosis factor alpha by enhancing mRNA degradation. J. Exp. Med. 1993; 177(6)1675–1680, [INFOTRIEVE], [CROSSREF], [CSA]
- Mutlu E. A., Farhadi A., Keshavarzian A. New developments in the treatment of inflammatory bowel disease. Expert Opin. Invest. Drugs 2002; 11(3)365–385, [CROSSREF], [CSA]
- Ochonisky S., Verroust J., Bastuji–Garin S., et al. Thalidomide neuropathy incidence and clinico-electrophysiologic findings in 42 patients. Arch. Dermatol. 1994; 130(1)66–69, [INFOTRIEVE], [CROSSREF], [CSA]
- Odeka E. B., Miller V. Thalidomide in oral Crohn's disease refractory to conventional medical treatment. J. Pediatr. Gastroenterol. Nutr. 1997; 25(2)250–251, [INFOTRIEVE], [CROSSREF], [CSA]
- Podolsky D. K. Inflammatory bowel disease. N. Engl. J. Med. 2002; 347(6)417–429, [INFOTRIEVE], [CROSSREF], [CSA]
- Ralph P., Nakoinz I. Antibody-dependant killing of erythrocyte and tumor targets by macrophage-related cell lines: enhancement by PPD and LPS. J. Immunol. 1977; 119: 950–954, [INFOTRIEVE], [CSA]
- Rutgeerts P., D'Haens G., Targan S., et al. Efficacy and safety of retreatment with anti-tumor necrosis factor antibody (infliximab) to maintain remission in Crohn's disease. Gastroenterology 1999; 117(4)761–769, [INFOTRIEVE], [CROSSREF], [CSA]
- Sabate J. M., Villarejo J., Lemann M., et al. An open–label study of thalidomide for maintenance therapy in responders to infliximab in chronically active and fistulizing refractory Crohn's disease. Aliment. Pharmacol. Ther. 2002; 16(6)1117–1124, [INFOTRIEVE], [CROSSREF], [CSA]
- Sampaio E. P., Sarno E. N., Galilly R., et al. Thalidomide selectively inhibits tumor necrosis factor alpha production by stimulated human monocytes. J. Exp. Med. 1991; 173(3)699–703, [INFOTRIEVE], [CROSSREF], [CSA]
- Sandborn W. J. Strategies for targeting tumour necrosis factor in IBD. Best Pract. Res. Clin. Gastroenterol. 2003; 17(1)105–117, [INFOTRIEVE], [CROSSREF], [CSA]
- Tragnone A., Corrao G., Miglio F., et al. Incidence of inflammatory bowel disease in Italy: a nationwide population-based study. Int. J. Epidemiol. 1996; 25(5)1044–1052, [INFOTRIEVE], [CSA]
- Valero F. C., Gonzalez V. B. Lung toxicity due to thalidomide. Arch. Bronconeumolog. 2002; 38(10)492–494, [CSA]
- van Deventer S. J. Small therapeutic molecules for the treatment of inflammatory bowel disease. Gut 2002; 50(suppl 3)III47–III53, [INFOTRIEVE], [CSA]
- van Deventer S. J. Tumour necrosis factor and Crohn's disease. Gut 1997; 40(4)443–448, [INFOTRIEVE], [CSA]
- Vasiliauskas E. A., Kam L. Y., Abreu-Martin M. T., et al. An open–label pilot study of low-dose thalidomide in chronically active, steroid–dependent Crohn's disease. Gastroenterology 1999; 117(6)1278–1287, [INFOTRIEVE], [CROSSREF], [CSA]
- Wettstein A. R., Meagher A. P. Thalidomide in Crohn's disease. Lancet 1997; 350(9089)1445–1446, [INFOTRIEVE], [CROSSREF], [CSA]
- Wichan P., Chojnacki J., Wojtun S. Immunotherapy of inflammatory bowel diseases. Pol. Merkuriusz. Lek. 2004; 17(suppl 1)40–43, [INFOTRIEVE], [CSA]