Abstract
Polyethylene glycol (PEG)ylated (stealth) immunoliposomes directed against human gliofibrillary acidic protein (GFAP) were prepared by coupling the thiolated monoclonal anti-GFAP antibodies with a maleimide derivative of phosphatidyl ethanolamine of the liposomal membrane. Experiments with cell cultures demonstrated specific and competitive binding of these immunoliposomes to embryonic rat brain astrocytes. Administered intravenously into rats, the immunoliposomes displayed typical kinetics with elimination half-lives of 8–15 hr. Being incapable of penetrating the unimpaired blood-brain barrier (BBB), these immunoliposomes, nevertheless, may be useful in delivering drugs to glial brain tumors (which continue to express GFAP) or to other pathological loci in the brain with a partially disintegrated BBB.
Creation of systems capable of delivering drugs from systemic circulation to cells of the central nervous system (CNS) continues to be one still-not-attained aims of neuropharmacology. As is well known, methodologically, there are three main problems: preparation of stable container systems that could release the agents transported specifically at their target cells, tissues, or organs; finding specific vector molecules that could guarantee a highly selective interaction between the transporting system and its target cells; and penetration through the brain-blood barrier (BBB). The third problem is now considered to be the most complicated one, and its future solution certainly will require some distinctly sophisticated approaches (like those of Cerletti et al. Citation2000; Gutman, Peacock, and Lu Citation2000; Huwyler, Wu, and Pardridge Citation1996; Shi, Boado, and Pardridge Citation2000; Shi and Pardridge Citation2000; Wu and Pardridge Citation1999).
In certain cases, however, this problem becomes less important: in pathological conditions with an impaired integrity of the BBB caused by brain tumors, injuries, or other disorders. In these cases, penetration through the BBB is not essential, and both the specificity of the vector components and the reliability of the container construction, as well as its systemic longevity, become the most important factors. With the advent of stealth (e.g., polyethylene glycol (PEG)ylated) liposomes, the latter problem may be considered as solved more or less successfully, because the PEGylated liposomes are relatively long-living in the blood, which essentially improves the pharmacokinetic efficiency of the water-soluble or lipophilic drugs carried by them.
Substantial progress also was made in the development of efficient and mild procedures for coupling these liposomes with immunochemical vectors (most frequently, with appropriate antibodies). In this context, of no small importance is the fact that numerous chemically modified lipids, able both to be integral parts of liposomal membranes and to chemically conjugate with vector proteins, recently have become commercially more easily available.
In our previous experiments (Chekhonin et al. Citation1991; Chekhonin, Ryabukhin, and Zhirkov Citation1995), the monospecific polyclonal antibodies (and their F(ab)-fragments, as well) to certain brain-specific proteins were used as vectors for efficient drug targeting to brain cells. Now, we have succeeded in producing monoclonal antibodies to the same proteins (Chekhonin, Dmitriyeva, and Zhirkov Citation2000; Chekhonin et al. Citation2000a; Chekhonin et al. Citation2001). In this work, the D4 monoclonal antibodies, raised against human gliofibrillary acidic protein (GFAP), were tested as potential vectors for stealth immunoliposomes directed against embryonic rat brain astrocytes.
MATERIALS AND METHODS
Liposome Preparation
Liposomes were prepared according to the procedure described by the Dutch group (Kamps et al. Citation2000) with certain modifications. A heptapeptide Lys-Tyr-Lys-Pro-Lys-Tyr-Asp 125I-labeled at its phenyl rings, synthesized in the Institute of Protein Research of the Russian Academy of Sciences (Pushchino, Moscow oblast, Russia), was used as a model compound transported by the liposomes.
The following lipid components were used: egg yolk lecithin (palmitoyl-oleyl-phosphatidylcholine, PC), cholesterol (Chol), maleimido - 4 - (p - phenylbutyryl) - phosphatidylethanolamine (MPB-PE), and poly-(ethylene glycol)-distearoyl-phosphatidyl ethanolamine (PEG-DSPE, with a PEG chain of an average molecular weight of 2000). All these compounds were purchased from Avanti Polar Lipids (Alabaster, AL, USA).
Other chemicals were obtained from the following sources: 2-iminothiolane, N-ethylmaleimide, HEPES, water-free sodium sulfate, sodium chloride, sodium hydroxide, Sephadex G-25 (superfine), ammonium thiocyanate, and ferric chloride hexahydrate from ICN Biomedicals (Aurora, OH, USA); fluorescent indicator Dil (1,1′-octadecyl-3,3,3′,3′,-tetramethylindocarbocyanin perchlorate) and Sepharose CL-4B from Fluka Chemie AG (Buchs, Switzerland); sodium and potassium phosphates from Serva Feinbiochemica (Heidelberg, Germany); chloroform and methanol from Merk (Whitehouse Station, NJ, USA); toluene and cyclohexane from Aldrich Chemical (Milwaukee, WI, USA); and the protein determination kit from Pierce Biotechnology (Rockford, IL, USA). All chemicals were of the best grade available.
All manipulations (except those of short duration) were performed under the argon atmosphere; high-purity argon was purchased from the Oxygen-Works (Balashikha, Moscow oblast, Russia).
PC, Chol, MPB-PE, PEG-DSPE, and Dil were mixed in chloroform: methanol (9:1) at a concentration of ∼10 mg total lipids in a molar ratio of 23:16:1:1.6:0.4. This lipid mixture was dried under reduced argon pressure in a Laborata 4000 rotary evaporator (with a Rotavac Control membrane pump; Heildolph Instruments, Schwabach, Germany). The dry lipid film was dissolved in absolute cyclohexane (to the total lipid concentration of 15 mg/ml), lyophilized (LP35 freeze-drier, Jouan, France), and hydrated in a solution of the 125I-labeled heptapeptide in the working buffer (0.01 M HEPES with 0.135 M NaCl or the phosphate-buffered saline, pH ∼ 7.5) at a total lipid concentration of 50 mg/ml. The emulsion was rotated overnight on the rotary evaporator under argon at atmospheric pressure.
For the hydration step, a conceivable degree of the peptide entrapment by the liposomes should be taken into account, because, in the absence of any selective peptide accumulation within the liposomes, this degree can be equal only to that part of the whole emulsion that corresponds to the internal volume of all the liposomes present in it. At the emulsion concentration used in this work (50 mg total lipids in 1 ml), this part could not exceed 20%. One also should consider that the unavoidable loss of liposomes, at the following concentrating and gel-filtering steps, can amount up to an additional 15–30%.
After the overnight maturation, the emulsion was sonicated in a G112SPl Special Ultrasonic Cleaner (Laboratory Supplies, Hicksvill, NY, USA; four times for 15 min, with 20-min breaks). The liposomes were then sized by repeated extrusion (13 times) through polycarbonate filters (Costar, Cambridge, MA, USA) with a pore size of 50 nm using an Avanti miniextruder (Avanti Polar Lipids) or with a high-pressure extruder (Lipex, Vancouver, Canada), depending of the emulsion batch size. According to Kamps et al. (Citation2000), the liposomes produced in this way (before and after their conjugation with protein) should have an average diameter of 70 and 100 nm, respectively.
The free peptide was removed by gel-filtration through a 1 × 50 cm column of Sephadex G-25 (superfine) equilibrated with the working buffer. The process could be easily followed visually due to the intense crimson coloration of the liposomes caused by Dil. The separation efficiency was controlled through the 125I-radioactivity of the fractions collected: the liposomes were eluted with the front (colored by Dil); they were followed by a broad band of the free peptide accompanied with a trace amount of the inorganic iodide (). The liposomal emulsion, rather diluted at this step, had to be concentrated to its initial volume (0.5–1 ml). This was performed by a centrifugal ultrafiltration (at 1000 g) through a CF50 CentriFlo conical membrane filters with a 50-kD exclusion threshold (Amicon, The Netherlands).
Figure 1 Gel filtration of 0.15 ml emulsion of PEGylated liposomes (7.5 mg total lipids) after their hydration with a solution of the 125I-labeled heptapeptide, sonication, and extrusion (but before coupling with antibodies) through a 1 × 47-cm column of Sephadex G-25(superfine) equilibrated with 10 mM sodium phosphate buffer, pH 7.4, at a flow rate of 16 ml/hr.
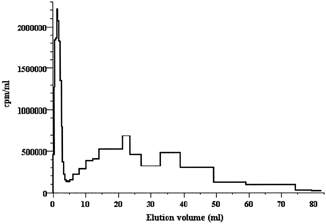
The liposomes were conjugated with protein according to the procedure described by Benzinger et al. (Citation2000), with some modifications. Murine monoclonal antibodies D4, used for conjugation with the liposomes, were raised against human GFAP; they were produced in our laboratory as described elsewhere (Chekhonin et al. Citation2000; Chekhonin et al. Citation2000a; Chekhonin et al. Citation2001). An irrelevant murine immunoglobulin and the monoclonal antibodies raised against neuron-specific enolase (NSE, also produced in our laboratory) were used as negative controls.
To achieve maximum coupling, the proteins were additionally thiolated with the Traut's reagent (2-iminothiolane): 1 mg of antibody dissolved in the working buffer (1–5 mg/ml) was mixed with 1 mg of 2-iminothiolane dissolved in the same buffer (1–10 mg/ml) and incubated for 1 hr in the dark at room temperature under argon; the excess of the thiolating agent was then removed by gel filtration through a 1×17-cm column of Sephadex G-25 (superfine). The thiolated proteins were incubated overnight with the fresh prepared liposomes (at 4°C in the dark under argon). The coupling reaction was stopped by addition of a 100-fold molar excess of N-ethylmaleimide that blocked free sulfhydryls; the mixture was then concentrated by centrifugal ultrafiltration, and the immunoliposomes were isolated by gel filtration through a 1 × 50-cm column of Sepharose CL-4B.
Immunoliposomes prepared were analyzed for their protein and phospholipid contents. Protein, after precipitation according to Wessel and Flügge (Citation1984), was determined with bicinchoninic acid (the BCA reagent from Pierce) and with an irrelevant murine IgG as a calibration standard; total phospholipids, after extraction from the water phase, were determined with ammonium ferrothiocyanate (Stewart Citation1980) with egg yolk phosphatidylcholine as a standard. The amount of the heptapeptide entrapped by the liposomes was determined by its radioactivity with a 1260 MultiGamma gamma counter (LKB, Sweden).
Systemic Elimination of the Liposomes
The experiments consisted in the comparison of the systemic elimination of the 125I-labeled heptapeptide after its bolus intravenous injections into rats as a free substance and as the liposome-entrapped forms. They were performed with the ketamine-anesthetized (80 mg/kg body weight, intraperitoneally) male Wistar rats (300–350 g) divided into three groups (8–12 rats in each group). Each rat of the first group was intravenously (into the femoral vein) injected with 0.54 μCi of the free radiolabeled peptide; the rats of the second and the third groups were injected with 0.38 μCi of the liposomal forms of the peptide (2.3 μmol total lipids for each rat). The PEGylated liposomes injected into the rats of the second group were not coupled with protein, whereas those injected into the rats of the third group were conjugated with the D4 antibodies. At the time points indicated, blood samples (0.2–1 ml) were taken from the same veins.
The blood time profiles of the radiolabeled peptide were analyzed by the nonlinear regression using a Prophet 5.0 statistical software (BBN Systems and Technologies, NIH, USA); the best fit was found with biexponential functions of the general form:
where C is the current peptide concentration in the blood (cpm/ ml); Cd0 and Ce0 are the parameters of this equation, apparently equal to initial concentrations at the distribution and elimination stages, respectively (cpm/ml); kd and ke are the corresponding apparent rate constants (h−1); and t is the time (h). The data from different kinetic experiments were normalized and expressed as percentage of apparent initial concentration (C0 = Cd0+ Ce0) calculated for each kinetic profile recorded. In all cases, the calculated parameters were statistically highly significant: probabilities of corresponding null hypotheses never exceeded 0.0001, and the coefficients of determination, R2, were over 0.99.
Embryonic Rat Brain Astrocytes
The embryonic rat brain astrocytes were isolated from 16-day rat embryos (Wistar) in our laboratory as described by Nakanishi, Nakanishi, and Kukita (Citation1999; Froes et al. Citation1999; Barnea and Roberts Citation1999), with slight modifications. The cells were grown during 16–18 days on the poly-L-lysine-coated glass plates in DMEM/F12 supplemented with 10% fetal calf serum, 2 mM glutamine, 0.45% glucose, 0.2 U/ml insulin, and 40 μg/ml gentamicin in a humidified 5% CO2-95% air atmosphere. The identity of the culture was proved by its characteristic light microscopic pattern recorded with a Leica DM LB HC microscope (Wetzlar, Germany) combined with a MPS60 Leica digital video camera (with an IM50 Image Manager software), as well as by immunofluorescence analysis with the D4 monoclonal anti-GFAP-antibodies. For this analysis, the cells were fixed with formaldehyde/acetic acid/ethanol, rinsed with the phosphate buffered saline with Tween 20, incubated with 0.5% bovine serum albumin, washed once more, and incubated with the D4 antibodies. The cell-bound D4 were visualized with the goat antimouse antibodies labeled with fluorescein isothiocyanate (). Primary antibodies (i.e., D4) were used at a concentration of 1–2 μg/ml; the secondary (FITC-labeled) antibodies (Sigma, cat. No. F8264) were used at a dilution of 1:64 recommended by the manufacturer.
Figure 2 Fluorescence micrographs of the cultured embryonal rat brain astrocytes (original magnification 200 ×). (A) Cells treated with D4 monoclonal anti-GFAP antibodies followed by goat antimouse antibodies labeled with fluorescein isothiocyanate. (B) Red fluorescence of the astrocytes incubated with Dil-labeled PEGylated immunoliposomes coupled with the D4 antibodies.
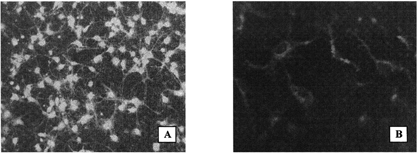
The cell-bound immunoliposomes were detected by their red fluorescence caused by Dil integrated in their lipid bilayers. To the embryonic astrocytes cultured on glass plates in Petri dishes, rinsed three times with the washing buffer (5 mM HEPES-TRIS-HCl, pH 7.4, with 145 mM NaCl, 54 mM KCl, 1.2 mM CaCl2, 1 mM MgCl2, and 1 mM Na2HPO4), 1 ml of the immunoliposome emulsion, appropriately diluted, was added. The culture was incubated at 36.6°C under the humidified CO2-O2 (5:95) atmosphere for 3, 6, or 9 hr. Then the culture was carefully washed with the same buffer and inspected under a Leica DM LB HC fluorescence microscope with 515–560-nm excitation filters (to detect the Dil-associated fluorescence). The specificity of this interaction was proved by its inhibition caused by a preincubation of the cells with uncoupled D4 antibodies. The liposomes coupled with an unspecific murine IgG or with the monoclonal antibodies to NSE (which display no affinity to astrocytes) were used as negative controls (as well as the similar liposomes bearing no protein at all).
RESULTS AND DISCUSSION
Our results have proved that the procedure described by Kamps et al. (Citation2000) for preparing the immunoliposomes is completely adequate and reproducible. According to this procedure, we prepared the immunoliposomes that, as regards their composition and kinetic behavior, were similar to those described by Kamps et al. (Citation2000). The only dissimilarities are that our liposomes were coupled with another immunochemical vector (i.e., with the D4 monoclonal antibodies against human brain GFAP), as well as in the compound they transported (a 125I-labeled model heptapeptide Lys-Tyr-Lys-Pro-Lys-Tyr-Asp).
shows the changes of the total blood radioactivity observed after intravenous administration of the radiolabeled peptide into rats (as a free substance or as liposome-entrapped formulations). These curves demonstrate those well-known differences in the elimination rates that are distinctive features of both the extremely rapidly disappearing oligopeptides administered intravenously and the comparatively long-circulating stealth liposomes. Thus, the encapsulation into liposomes (not coupled with any protein) prolonged essentially both stages of the apparently biphasic kinetics of the peptide: the half-lives for the first and second kinetic phases were increased from 7.3 up to 33 sec and from 3.6 up to 14.7 hr, respectively. The corresponding areas under the pharmacokinetic curves (AUCs), which reflect the systemic bioavailability of the preparations administered, increased by a factor of ∼90 (11 versus 978 arb. units, respectively). Just as in the work of Kamps et al. (Citation2000), after 24 hr, 20–30% of the liposomes (as a percentage of the normalized C0) were still present in the blood, whereas for the free peptide this value was only as low as 0.130 ± 0.006%. Similar behavior also was observed for the PEGylated liposomes coupled with the D4 antibodies (). However, the presence of the protein attached to these liposomes accelerated both stages of their pharmacokinetics by a factor of 2–3.
Figure 3 Systemic elimination of the free or liposome-entrapped 125I-labeled heptapeptide Lys-Tyr-Lys-Pro-Lys-Tyr-Asp after its intravenous administration to rats. ▪ = radioactivity of the free peptide, ▴ = radioactivity of the peptide within PEGylated liposomes not coupled with vector antibodies, • = radioactivity of the peptide within PEGylated immunoliposomes coupled with the D4 monoclonal anti-GFAP antibodies.
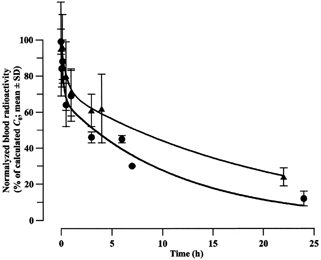
Unfortunately, we were not able to measure directly the size of the liposomes prepared. Nevertheless, our data allowed us to estimate it indirectly. Thus, after integrating the chromatographic profile shown in , we concluded that the liposomes had entrapped approximately 20% of all the label present in the entire emulsion volume. For the concentration of the liposomes used in this experiment, this percentage is absolutely equal to the percentage of the internal volume of the unilamellar liposomal vesicles with an average diameter of ∼70 nm formed by extrusion through membrane filters with a pore size of 50 nm. Hence, this result implies, although indirectly, that the liposomes prepared had an average diameter of 70 nm.
Analysis of lipid and protein contents of the immunoliposomes showed that, depending on the initial protein-to-lipid ratios, they contained 150–600 μg protein per 1 μmol of total phospholipids, which agrees well with the reference data for the coupling procedure applied (Allen Citation1995). A theoretical calculation, considering that the liposomes prepared were unilamellar vesicles with a diameter of ∼70 nm, as well as the fact that they consisted of about 100,000 lipid molecules, allowed the conclusion to that, at the protein-to-lipid ratios indicated, each immunoliposome was coupled with 60–240 antibody molecules, which also is typical of the immunoliposomes of this kind.
The vector efficacy of the immunoliposomes was proved in the binding experiments with the cultures of embryonic rat brain astrocytes (). In these experiments, we used the Dil-labeled anti-GFAP immunoliposomes that contained ∼240 μg protein per 1 μmol total phospholipids (i.e., each liposome was coupled with about 100 molecules of the D4 antibodies). The concentration range was from 0.2 to 1 μmol total phospholipids per 1 ml, and the cells were incubated with these immunoliposomal emulsions for 3, 6, or 9 hr. The Dil-labeled PEGylated liposomes coupled with an unspecific murine IgG or with monoclonal antibodies to NSE, as well as the liposomes that were not conjugated with any protein at all, were used as negative controls (at the same concentrations and with the same incubation times).
demonstrates an intense red fluorescence of Dil occurred after incubation of the cultured embryonal rat brain astrocytes with PEGylated liposomes coupled with the D4 monoclonal antibodies. This fluorescence was observed already at the lowest concentrations of the immunoliposomes and at the shortest incubation times; neither increase in concentration nor prolongation of the incubation caused any further increase in the fluorescence. A decrease in the incubation temperature to 4°C did not change its intensity, which suggests a rather receptor-independent interaction.
None of the control preparation (PEGylated liposomes without any protein conjugated, as well as the liposomes bearing either a nonspecific murine IgG or the anti-NSE monoclonal antibodies) was able to induce such a fluorescence of the astrocytes. Moreover, a preincubation of the culture with the saturating amount of free D4 antibodies inhibited completely the occurrence of this fluorescence. The absence of unspecific binding also was verified by incubating the D4-bearing liposomes with the cultured fibroblasts. (As the corresponding fluorescence microphotographs displayed only the background “black squares,” they are not shown here.)
These results suggest that the immunoliposomes prepared preserved the immunochemical properties of the antibodies coupled and were able to interact specifically with GFAP, a specific marker protein of the astrocytes. Hence, with their systemic longevity, as well as with their immunochemical specificity, these immunoliposomes, although incapable of penetrating the unimpaired BBB, may be useful in delivering the pharmacological agents to the glial brain tumors (which still continue to express GFAP) or to other pathological loci in the brain with a partially disintegrated BBB.
REFERENCES
- Allen T. M., Hansen C. B., Zalipsky S. Antibody-targeted stealth liposomes. Stealth Liposomes, D. Lasic, F. Marin. CRS Press, Boca Raton, FL 1995; 233–244
- Barnea A., Roberts J. A method for dissociation and aggregate culture of human fetal brain cells in serum-free medium. Brain Res. Protocols 1999; 4: 156–164
- Benzinger P., Martiny-Baron G., Reusch P., Siemeister G., Kley J. T., Marme D., Unger C., Massing U. Targeting of endothelial KDR receptors with 3G2 immunoliposomes in vitro. Biochim. Biophys. Acta 2000; 1466: 71–78, [PUBMED], [INFOTRIEVE]
- Cerletti A., Drewe J., Fricker G., Eberle A. N., Huwyler J. Endocytosis and transcytosis of an immunoliposome-based brain drug delivery system. J. Drug Target. 2000; 8: 435–446, [PUBMED], [INFOTRIEVE]
- Chekhonin V. P., Dmitriyeva T. B., Zhirkov Yu. A. Immunochemical Analysis of Neurospecific Antigens. Meditsina, Moscow 2000, (in Russian; submitted for publication in English, Pergamon Press, 2002)
- Chekhonin V. P., Gurina O. I., Dmitriyeva T. B., Shepeleva I. I., Ryabukhin I. A., Babich G. N. Monoclonal antibodies against GFAP: preparation, characterization, and immunoenzyme assay. Byul. Eksp. Biol. Med. Russian Bull. Exp. Biol. Med. 2001; 132: 188–191, (in Russian)
- Chekhonin V. P., Gurina O. I., Ryabukhin I. A., Shepeleva I. I., Savchenko E A., Semenova A. V., Khaustova M. Yu., Biktimirov R. G., Kekelidze Z. I., Dmitriyeva T. B. An immunoenzyme assay for GFAP with monoclonal antibodies in the assessment of the blood brain barrier permeability in various neural and/or mental diseases. Ross. Psikhiatr. Zh. Russ. Psych. J. 2000a; 1: 14–18, (in Russian)
- Chekhonin V. P., Kabanov A. V., Zhirkov Yu. A., Morozov G. V. Fatty acid acylated F(ab)-fragments of antibodies to neurospecific proteins as carriers for neuroleptic targeted delivery in brain. FEBS Lett. 1991; 287: 149–152, [PUBMED], [INFOTRIEVE], [CROSSREF]
- Chekhonin V. P., Ryabukhin I. A., Zhirkov Yu. A. Transport of hydrophobized fragments of anitbodies through the blood-brain barrier. Neuroreport 1995; 7: 129–132, [PUBMED], [INFOTRIEVE]
- Froes M. M., Correia A. H. P., Garcia-Abreu J., Spray D. C., Campos de Carvalho A., Neto V. M. Gap-junctional coupling between neurons and astrocytes in primary central nervous system cultures. Proc. Natl. Acad. Sci. USA 1999; 96: 7541–7546, [PUBMED], [INFOTRIEVE], [CROSSREF]
- Gutman R. L., Peacock G., Lu D. R. Targeted drug delivery for brain cancer treatment. J. Control. Rel. 2000; 65: 31–41, [CROSSREF]
- Huwyler J., Wu D., Pardridge W. M. Brain drug delivery of small molecules using immunoliposomes. Proc. Natl. Acad Sci. USA 1996; 93: 14164–14169, [PUBMED], [INFOTRIEVE], [CROSSREF]
- Kamps J. A. A. M., Konig G. A., Velinova M. J., Morselt H. W. M., Wilkens M., Gorter A., Donga J., Scherphof G. L. Uptake of long-circulating immunoliposomes, directed against colon adenocarcinoma cells, by liver metastases of colon cancer. J. Drug Target. 2000; 8: 235–245, [PUBMED], [INFOTRIEVE]
- Nakanishi K., Nakanishi M., Kukita F. Dual intracellular recording of neocortical neurons in a neuron-glia coculture system. Brain Res. Protocols 1999; 4: 105–114, [CROSSREF]
- Shi N., Pardridge W. M. Noninvasive gene targeting to the brain. Proc. Natl. Acad. Sci. USA 2000; 97: 7567–7572, [PUBMED], [INFOTRIEVE], [CROSSREF]
- Shi N., Boado R. J., Pardridge W. M. Antisense imaging of gene expression in the brain in vivo. Proc. Natl. Acad. Sci. USA 2000; 97: 14709–14714, [PUBMED], [INFOTRIEVE], [CROSSREF]
- Stewart J. C. M. Colorimetric determination of phospholipids with ammonium ferrothiocyanate. Anal. Biochem. 1980; 104: 10–14, [PUBMED], [INFOTRIEVE], [CROSSREF]
- Wessel D., Flügge U. I. A method for the quantitative recovery of protein in dilute solution in the presence of detergents and lipids. Anal. Biochem. 1984; 138: 141–143, [PUBMED], [INFOTRIEVE], [CROSSREF]
- Wu D., Pardridge W. M. Neuroprotection with noninvasive neurotrophin delivery to the brain. Proc. Natl. Acad. Sci. USA 1999; 96: 254–259, [PUBMED], [INFOTRIEVE], [CROSSREF]