Abstract
Transcutaneous administration of nonsteroidal anti-inflamma- tory drugs and essential fatty acids from fish oil, principally eicosapentaenoic acid (EPA) and docosahexaenoic acid (DHA), may simultaneously lead to increased cyclooxygenase inhibition and the production of less potent inflammatory mediators within joints. The objective of our study was to determine the permeation of ketoprofen, EPA, and DHA (from fish oil) across pig ear skin in vitro in the presence of the enhancer 1,8-cineole. Formulations containing 2.5% ketoprofen in fish oil with varying concentrations of 1,8-cineole were prepared and applied to full-thickness pig ear skin mounted in all glass Franz-type diffusion cells. Simultaneous permeation of ketoprofen and EPA and DHA from these formulations was determined by reverse phase HPLC over a 48-hr period (n = 6). We found that fish oil alone enhanced the permeation of ketoprofen across pig ear by a factor of 1.72 relative to a water vehicle. There was a dose-dependent increase in the rate of permeation of ketoprofen relative to the concentration of 1,8-cineole. The highest Q24 and Q48 was obtained with a 20% 1,8-cineole formulation with values of 355.78 ± 50.73 μg cm−2 and 963.29 ± 136.69 μg cm−2, respectively. Surprisingly, no clear effect upon the permeation of EPA and DHA by 1,8-cineole was observed, with the highest Q24 and Q48 values seen in a formulation containing no 1,8-cineole. This may have been due to differential solvation effects prior to or during the permeation process or modulation of the skin during the permeation process.
Rheumatoid arthritis (RA) and osteoarthritis (OA) together affect up to 2.5% of the world's population. Typically, nonsteroidal anti-inflammatory drugs (NSAIDs), for example ibuprofen and ketoprofen, are the first line treatment and act by inhibiting cyclooxygenase (COX) (Buchanan Citation1997). A significant limitation to the use of NSAIDs is their side effect profile when dosed orally (Hersh, Moore, and Ross 2000) and topical formulations are of limited value. Currently, there is an increasing trend among those affected for alternative, natural product supplementation.
The low incidence of a number of disease states prevalent in western society, including arthritis, among the Inuit population of North America and Greenland has been linked to their high consumption of oily fish. Fish oil is a complex mixture of triacylglycerols containing a high percentage of polyunsaturated fatty acids (PUFA), in particular eicospentaenoic acid (EPA) and docosahexaenoic acid (DHA) (Belch 1990). The relationship between PUFA and inflammation lies with a group of chemical messengers that act within the immune system termed eicosanoids and includes prostaglandins (PG) and leukotrienes (LT). The membranes of most immune cells contain high amounts of arachidonic acid (ARA), compared with EPA and DHA; thus ARA is usually the principal precursor for eicosanoid synthesis.
Metabolism of ARA by the COX and 5-lipoxygenase (5-LOX) pathways gives rise to the 2-series PG and the 4-series LT respectively—both potent inflammatory mediators (Tempel et al. Citation1990). However, increased bioavailability of EPA and DHA leads to the biosynthesis of 3-series PG and 5-series LT, which are substantially less potent inflammatory mediators. Further elucidation of the biochemical basis for the antiarthritis properties of fish oil has been published (Curtis et al. Citation2000).
Cartilage is served by relatively limited local blood flow, and existing oral strategies for targeting NSAID and PUFAs to this tissue could be improved upon by delivering them locally to the focus of aggravation. Thus, an effective transcutaneous system containing NSAID and fish oil as a source of EPA and DHA would allow direct application to affected areas, producing relatively high and localized concentrations under the skin (Heard et al. Citation2003). However, due to the barrier function of the skin, enhancement measures are often required to improve and control rates of permeation across skin. Furthermore, it is evident that the successful development of any topical system containing fish oil needs to consider the unpleasant odors arising from the use of this natural product, in particular oxygenated PUFAs.
This article describes our efforts to enhance the simultaneous transcutaneous delivery (i.e., across entire skin to underlying joint) of EPA, DHA, and ketoprofen via chemical enhancement. We considered it important that the enhancer address the issue of odor, and we decided to incorporate into the formulation 1,8-cineole (eucalyptol), the major terpenoid of eucalyptus oil. This has demonstrated previously value as a highly effective permeation enhancer (Yamane, Williams, and Barry Citation1995) and also possesses a pleasant “medicinal” odor that had a long-acting odor-masking effect on the fish oil when applied to skin.
MATERIALS AND METHODS
Fish oil capsules 1000 mg (Boots Super Strength, batch number 100673) were purchased from a local store. Ketoprofen, butylated hydroxyanisole (BHA), and cetrimide British Pharmacopeia were obtained from Sigma–Aldrich Company (Poole, U.K.) High vacuum grease was from Dow Corning (Barry, U.K.), methanol (HPLC grade) and petroleum ether 60–80°C were obtained from Fisher Chemicals (Loughborough, U.K.). All other reagents were of analytical grade or equivalent. Freshly excised pig ears were obtained from a local abattoir prior to steam cleaning, and 4 were cleaned under running water and the hair removed using electric clippers. Full thickness skin was then removed from the dorsal side of the ear, cut into approximately 2 × 2 cm sections and stored at −20°C until required.
Preparation of Formulations
A fixed substurated dose of ketoprofen (2.5% w/w) was used in each formulation to represent commercial viability. The appropriate masses of ketoprofen and fish oil were combined, in addition to BHA (0.05% w/w) to inhibit PUFA degradation, and the formulation vortex mixed. Then, 1,8-cineole was incorporated as outlined in , and the formulations again vortex mixed. Three control formulations were employed: 2.5% ketoprofen in fish oil, 2.5% ketoprofen in deionized water, and fish oil alone. The formulations were stored at 2-4°C until required (≤24 hr).
Table 1 Formulations used in this study
Receptor Phase
Degassed 30 mg ml−1 Cetrimide solution, containing 0.05% BHA as a standard reagent to inhibit decomposition of the PUFAs, was used to provide an effective sink for ketoprofen and fish oil. In this solution, ketoprofen was freely soluble and the solubility of fish oil was estimated at 5.1 ± 0.3 μl ml−1 (5.2 ± 0.3 mg ml−1), approximating to 1.73 ± 0.1 mg (5.72 ± 0.33 μmol) EPA ml−1, by adding sequential aliquots of the oil to receptor phase et 37°C until a clear solution was no longer attainable. Furthermore, a preliminary study indicated that this receptor phase performed in a similar manner to the more ubiquitous alcohol-in-water system, giving rise to no perceptible deleterious effects on the skin (Heard et al. Citation2002).
In Vitro Skin Permeation Experiments
Skin permeation experiments were carried out using glass Franz-type diffusion cells incorporating pig ear skin as a model for human skin (Simon and Maibach Citation2000). The skin sections were equally distributed between the treatments to eliminate donor variability and placed, stratum corneum uppermost, onto the pre-greased cell flanges. The donor chambers were placed on top, the whole cells clamped, and the receptor chambers filled with receptor phase, ensuring no air was trapped beneath the skin. Microstirrer bars were added and the complete cells placed on a submersible magnetic stirrer plate (Variomag, Daytona Beach, FL, USA) in a water bath maintained at 37°C. After an equilibration time of 10 min, the cells were dosed with 1 ml (∼0.8 g) of formulation. Six replicates were carried out for each formulation. The entire receptor phases were removed at preset times over a 48-hr period. Aliquots of 500 μl were retained for the analysis of ketoprofen, with the remainder retained for the analysis of the fish oil. Samples were stored at −20°C prior to analysis. The diffusion cells were replenished with fresh, temperature-equilibrated receptor phase after each time-point.
ANALYTICAL METHODS
HPLC Analysis of Ketoprofen
Ketoprofen was determined on a Hewlett Packard 1100 HPLC system, fitted with a Phenomenex Kingsorb C18 column (150 × 4.6 mm, 5 μm) and a Phenomenex Securiguard guard column. The mobile phase was acetonitrile, potassium phosphate buffer (pH 1.5) (55:45; v/v), run isocratically for 10 min at a flow rate of 1.0 ml min−1. Detection of ketoprofen was by ultraviolet using a wavelength of 258 nm. Under these conditions, the retention time of ketoprofen was 6.8 min and the limit of detection (LOD) was 0.03 μg ml−1. A standard calibration curve was constructed over the range 1–120 μg ml−1, prepared in receptor phase, which provided an r2 of 0.99.
Preparation of Fatty Acid Methyl Esters (FAMEs)
PUFAs were transmethylated by heating under reflux in a sealed methylating tube with anhydrous methanol (10 ml) in the presence of an acid catalyst (2.5% v/v H2SO4) for 2 hr at 70°C. After this time the solution was allowed to cool to room temperature and the reaction quenched by the addition of 2.5 ml aqueous NaCl (5% w/v). The FAMEs were then extracted with 3 × 30 mls petroleum ether (60–80°C b.p.), the ether phases pooled and evaporated to dryness under vacuum [5]. The remaining product was dissolved in methanol (2 ml), bubbled with nitrogen, sealed, and stored under light exclusion prior to HPLC analysis. The technique was validated by the processing and HPLC analysis (section 2.4.3) of pure EPA and DHA. High conversion was confirmed by mass spectroscopy, demonstrating the presence of base peak molecular ions for EPA methyl ester (m/z = 317) and DHA methyl ester (m/z = 343), in addition to the absence of peaks for EPA and DHA free fatty acids (Thermoquest LCQ-Deca, Hemel Hempstead, UK).
HPLC Analysis of FAMEs
FAMEs were analyzed on a Hewlett Packard 1100 chromatograph with a Phenomenex Kingsorb C18 column (150 × 4.6 mm, 5 μm) and a Phenomenex Securiguard guard column. The mobile phase was methanol:water (95:5 v/v), run isocratically for 20 min at a flow rate of 1.0 ml min−1, and detection was by ultraviolet at 210 nm. EPA and DHA esters eluted at 10.8 min and 12.8 min, respectively. Standard calibration curves, prepared in receptor phase, were constructed over the range 1–250 μg ml−1 and provided r2 values of > 0.99. The LOD was 0.40 microgram per ml and 0.50 μg ml−1 for EPA methyl ester and DHA methyl ester, respectively.
RESULTS AND DISCUSSION
Transcutaneous Delivery of Ketoprofen
shows that steady-state flux of ketoprofen was attained for each formulation between ∼12 and 48 hr. Permeation data is summarized in . The lowest flux was from the water vehicle control (V) and it is apparent that a fish oil vehicle alone (I) enhanced the permeation of ketoprofen relative to water, by a factor of 1.72 (p = 0.079, where null hypothesis is equal to 0). This probably was due to greater absorption of the oil vehicle, incorporating the ketoprofen solute, into the lipoidal domains of the stratum corneum, followed by diffusion across the viable epidermis and dermis. The presence of these more hydrophilic layers appears not to have presented a major obstacle.
Figure 1 Permeation profile of ketoprofen from formulations with varying concentrations of 1,8-cineole (n = 6, ±SEM). I-ketoprofen in fish oil; II-ketoprofen in fish oil + 5% 1,8-cineole; III-ketoprofen in fish oil + 10% 1,8-cineole; IV-ketoprofen in fish oil + 20% 1,8-cineole; V-ketoprofen in water.
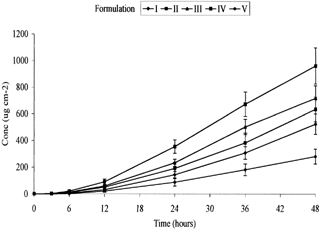
Table 2 Summary of permeation data for the in vitro transcutaneous delivery of ketoprofen across full-thickness pig ear skin (n = 6, ±SEM)
Several studies have shown that fatty acids can significantly improve the permeation of certain drugs. For example, Green, Guy, and Hadgraft (Citation1988) hypothesized that ion pair formation between naphazoline and fatty acids resulted in increased skin/ vehicle partitioning of the drug. The enhancement potential of linoleic acid previously has been studied in the transdermal delivery of melatonin across rat and porcin skin (Kandimalla et al. Citation1999) and for luteinizing hormone release hormone across human epidermis (Bhatia and Singh Citation1999). As is typical in such reports the fate of the fatty acids and vehicle was not reported, although the effects of occlusion on the percutaneous penetration of linoleic acid was reported (Taylor et al. Citation2002). A further major difference is that in the current work fish oil was dosed rather than free fatty acids—the latter being more amphiphilic and less liable to being absorbed by the stratum corneum lipids than the more lipophilic fish oil.
The mechanism of enhancement by fatty acids is reduction of skin resistance as a permeability barrier by disruption of tightly packed lipid regions of the stratum corneum. Perturbation increases the partitioning of a permeant molecule into the intercellular lipid matrix (Barry Citation1987; Wang, Yang, and Heng Citation2003). However, within the fish oil the fatty acids were largely present as triacylglycerols, rather than free fatty acids. Therefore, uptake of oil and ketoprofen would be more likely to be a consequence of absorption due to the miscibility between the skin lipids and the oil. and also show a dose-dependent increase in the permeation of ketoprofen relative to the proportion of 1,8-cineole. The greatest flux of ketoprofen, 24.31 ± 3.18 μg cm−2 h−1, was obtained with formulation IV containing 20% w/w 1,8-cineole, which was significantly different (p = < .001) from formulation V. Terpenoids are believed to act by disruption of lipid bilayers and increasing diffusivity (Yamane et al. Citation1995). Thus, a dual action mode of enhancement appeared to operate in formulations containing both fish oil and cineole. The Q24 and Q48 data also express the same dose-dependent relationship between ketoprofen and 1,8-cineole with the highest Q24 and Q48 values, 355.78 ± 50.73 μg cm−2 and 963.29 ± 136.69 μg cm−2, respectively, being observed in formulation IV containing 20% 1,8-cineole.
Our earlier paper showed the flux of ketoprofen from a saturated fish oil vehicle to be 6.12 ± 2.39 μg cm−2 h−1 through full thickness pig skin (Heard et al. Citation2003). The current study has shown the flux of ketoprofen from a 2.5% w/w fish oil vehicle (formulation I) to be 11.54 ± 1.84 μg cm−2 h−1. The flux of ketoprofen from the 2.5% w/w aqueous solution (formulation V) was 6.72 ± 1.78 μg cm−2 h−1. The differences in these values may be attributable to the greater amount of ketoprofen within the applied dose or the inherent variability associated with experiments involving excised skin.
Transcutaneous Delivery of EPA and DHA
Transcutaneous delivery of the fish oil, simultaneously with ketoprofen, was confirmed by the cumulative permeation EPA and DHA ( and ). The data are summarized in . The most apparent and surprising observation was that, the general shape of the permeation profiles was dissimilar to those obtained for ketoprofen. Yet unlike ketoprofen, the presence of 1,8-cineole had no discernible enhancing effect on EPA and DHA (which were both in much greater excess). This suggests that the enhancer was acting only with the ketoprofen, possibly as the 1,8-cineole may have solvated the ketoprofen in preference to the oil triacylglycerols. Furthermore, it is apparent that fluxes were not at steady-state throughout the 48-hr period, instead there initially was more rapid permeation followed by an approximately linear secondary permeation phase. Reasons for this behavior are not clear, although as ketoprofen provided textbook permeation profiles, breakdown of the barrier is unlikely. Saturation of the receptor phase also can be discounted as the sampling methods involved using the entire receptor phase and the levels of EPA in the receptor samples were some three orders of magnitude below solubility level. It is possible the morphology of the skin was altering in response to the permeating oil in a manner that restricted the permeation process. Seemingly, this response did not affect permeation of the (smaller) ketoprofen.
Figure 2 Permeation profile of EPA from formulations with varying concentrations of 1,8-cineole (n = 6, ±SEM). I-ketoprofen in fish oil; II-ketoprofen in fish oil + 5% 1,8-cineole; III-ketoprofen in fish oil + 10% + 1,8-cineole; IV-ketoprofen in fish oil + 20% 1,8-cineole.
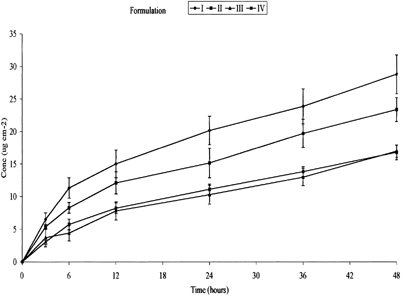
Figure 3 Permeation profile of DHA from formulations with varying concentrations of 1,8-cineole (n = 6, ± SEM). I-ketoprofen in fish oil; II-ketoprofen in fish oil + 5% 1,8-cineole; III-ketoprofen in fish oil + 10% 1,8-cineole; IV-ketoprofen in fish oil + 20% 1,8-cineole.
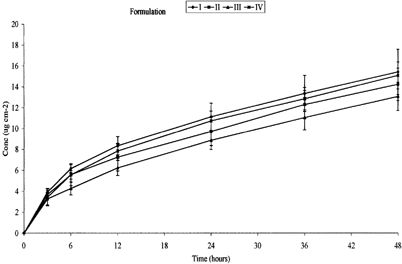
Table 3 Summary of the flux data for the in vitro transcutaneous delivery of EPA and DHA from fish oil across full-thickness pig ear skin (n = 6, ±SEM)
summarizes the Q24 and Q48 data for both EPA and DHA. These results reflect the pattern shown in and , no clear relationship can be determined between the permeation of either EPA or DHA and an increase in concentration of 1,8-cineole. There appeared to be an inverse dose-dependent relationship until formulation IV where the permeation increases slightly. Both the permeation profile and Q24 and Q48 values reflect the flux data shown in . The greatest 1st phase flux of EPA, 1.89 ± 0.26 μg cm−2 h−1 (67.20 × 10−5% of dose), was obtained from formulation I with no 1,8-cineole present. The 2nd phase flux also was the greatest of the formulations, 0.36 ± 0.06 μg cm−2 h−1 (8.44 × 10−5% of dose). Surprisingly the lowest 1st phase flux, 0.74 ± 0.20 μg cm−2 h−1 (28.41 × 10−5% of dose), was obtained from formulation III with 10% w/w 1,8-cineole. The lowest 2nd state flux was seen with formulation IV containing 20% w/w 1,8-cineole with a value of 0.24 ± 0.03 μg cm−2 h−1 (7.16 × 10−5% of dose). The very low percentages permeated reflect a combination of the high levels EPA and DHA in the infinite doses used and the high lipophilicity of the oil (Tayor et al. Citation2002).
Table 5 Amount permeated (Q24 and Q48) values for EPA and DHA delivered from fish oil across excised full-thickness pig ear skin
Statistical analysis shows no significant difference in results between formulation II, III, IV, and I (p = < 0.001 in each case, where null hypothesis is equal to 1) in the first phase flux. Lag times were too short to be measured. Similarly no significant effect of 1,8-cineole can be seen on the permeation of DHA. The greatest 1st phase flux, 1.03 ± 0.06 μg cm−2 h−1 (57.50 × 10−5% of dose), was once again seen in formulation I containing no 1,8-cineole. The lowest flux, 0.21 × 0.01 μg cm−2 h−1 (12.74 × 10−5% of dose), was seen in the second steady-state formulation IV containing 20% w/w 1,8-cineole. Once again statistical analysis showed no significant difference in the results of formulations II, III, and IV relative to I (p = < .001 in each case). No EPA or DHA were detected for formulations V and VI.
The 1st phase flux data for both EPA and DHA show no clear pattern of the affect of 1,8-cineole. The 2nd phase of steady state, however, shows an inverse dose-dependent relationship between the concentration of 1,8-cineole and the flux of both EPA and DHA: as the concentration of 1,8-cineole increases, the flux of both EPA and DHA decreased. From the permeation profiles of both EPA and DHA it would appear that there was an initial rapid flux, followed by a second phase apparently at steady-state. This is unusual as the receptor phase saturation is usually a well-defined plateau. If only one permeant was present, the initial rapid flux could not be explained by saturation of the receptor as it would be expected to saturate to the same level, although the plateau would be expected. However, the trend is the reverse of the results for ketoprofen, and it is possible that the lower the rate of permeation of ketoprofen, the greater the rate of EPA/DHA/fish oil as there was greater capacity in the receptor phase.
The criticality of vehicle formulation is well established. For example, Wang et al. (Citation2003) recently demonstrated that fatty acids with short chains in a lipophilic mineral oil formulation were able to perturb stratum corneum lipids but not in those based on propylene glycol. It is a basic physicochemical concept that solutes exist in discrete solvation cages, although a less well-probed phenomenon is that of differential solvation (Duffy and Jorgense Citation2000; Diaz and Berger Citation2001) within a vehicle and its consequences in topical drug delivery (Heard et al. Citation2003; Karia et al. 2004). The nature of solvated permeant molecules depends on the mutual interactions between all species present within the formulation, and it is feasible that the differences seen in enhancement between the PUFA and ketoprofen were due to selective solvation by 1,8-cineole. If the enhancer preferentially solvated ketoprofen molecules, then permeation may be expected to be relatively greater. Solvation of the smaller, more functionalized ketoprofen molecule would be preferential to the solvation of the large sterically hindered fish oil units.
Table 4 Percentage of the applied dose of EPA and DHA from fish oil permeating full thickness pig ear skin
As EPA and DHA were both administered as fish oil, the main barrier to transcutaneous permeation would have been the aqueous domains of the viable epidermis and the dermis, which are substantially more polar than the lipoidal domains of the stratum corneum. This may explain the very low levels of EPA and DHA in the receptor solutions. The unclear pattern of results when considering the concentration of 1,8-cineole may be explained by the method of enhancement of 1,8-cineole. Terpenoids act as enhancers by disrupting the lipid bilayers within the stratum corneum, although the dose dependency of such effects is less well documented. At levels up to 10% 1,8-cineole the integrity of the skin may be intact and the hypothesis that the greater the permeation of ketoprofen, the lower the permeation of the oil due to receptor phase capacity holds true. However, at 20% 1,8-cineole, far greater disruption to the integrity of the skin may have occurred allowing greater permeation of ketoprofen and fish oil. This could explain the increase in the 1st phase flux of EPA and DHA in formulation IV. Negligible amounts of EPA or DHA permeated from formulation VI that consisted of 100% fish oil. The main barrier to the transcutaneous permeation of a highly lipophilic substance would have been the dermis, which is substantially more polar than the lipoidal domains of the stratum corneum. The surprising inference from this observation was that ketoprofen enhanced the permeation of EPA and DHA in the other formulations.
Differences in the permeation of EPA and DHA were expected due to the different concentrations of the two within the fish oil, although no distinct pattern in the permeation of the two fatty acids was observed. This may be explained by differential metabolism within the (freshly excised) skin, a phenomenon that is currently under investigation.
CONCLUSIONS
The permeation of ketoprofen from a fish oil vehicle was ∼1.72 compared with an aqueous solution of the drug. A dose-dependent relationship was found between the flux of ketoprofen and the concentration of 1,8-cineole, although no clear effects were seen on the permeation of EPA and DHA as fish oil. This may have been due to differential solvation effects in the vehicle, in the permeation process, or modulation of the barrier that affected the permeation of the oil but not the ketoprofen.
We are very grateful to the Wellcome Trust for funding the mass spectrometer through an Equipment award.
REFERENCES
- Barry B. W. Mode of action of penetration enhancers in human skin. J. Contr. Rel. 1987; 6: 85–97
- Belch J. Fish oil and rheumatoid arthritis: dose a herring a day keep the rheumatologists away?. Ann. Rheum. Dis. 1996; 49: 71–72
- Bhatia K. S., Singh J. Effect of linolenic acid ethanol or limonene ethanol and iontophoresis on the in vitro percutaneous absorption of LHRH and ultrastructure of human epidermis. Int. J. Pharmac. 1999; 180: 235–250, [CROSSREF]
- Buchanan N. M. M. Is there a place for topical NSAIDs?. Pharmaceut. J. 1997; 259: 294–295
- Curtis C., Hughes C. E., Flannery C. R., Little C. B., Harwood J. L., Caterson B. n-3 fatty acids—specifically modulate catabolic factors involved in articular cartilage degradation. J. Biol. Chem. 2000; 275: 721–724, [PUBMED], [INFOTRIEVE], [CROSSREF]
- Diaz D. M., Berger S. Preferential solvation of a tetrapeptide by trifluoroethanol as studied by intermolecular NOE. Mag. Reson. Chem. 2001; 39: 369–373, [CROSSREF]
- Duffy E. M., Jorgensen W. L. Prediction of properties from simulations: free energies of solvation in hexadecane, octanol, and water. J. Am. Chem. Soc. 2000; 122: 2878–2888, [CROSSREF]
- Green P. G., Guy R. H., Hadgraft J. In vitro and in vivo enhancement of skin permeation with oleic and lauric acids. Int. J. Pharmaceut. 1988; 48: 103–111, [CROSSREF]
- Heard C. M., Gallagher S. J., Harwood J. L., Maguire P. B. The in vitro delivery of NSAIDs across skin was in proportion to the delivery of essential fatty acids in the vehicle—evidence that solutes permeate skin associated with their solvation cages?. Int. J. Pharmaceut. 2003; 261: 165–169, [CROSSREF]
- Hersh E. V., Moore P. A., Ross G. L. Over-the-counter analgesics and antipyretics: a critical assessment. Clin. Therapeut. 2002; 22(5)500–548, [CROSSREF]
- Kandimalla K., Kanikkannan N., Andega S., Singh M. Effect of fatty acids on the permeation of melatonin across rat and pig skin in-vitro and on the transepidermal water loss in rats in-vivo. J. Pharm. Pharmacol. 1999; 51: 783–790, [PUBMED], [INFOTRIEVE], [CROSSREF]
- Karia C., Harwood J. L., Heard C. M., Morris A. P. Simultaneous permeation of tamoxifen and γ linolenic acid across excised human skin. Further evidence of the permeation of solvated complexes. Int. J. Pharmaceut. 2004; 271(1–2)305–309, [CROSSREF]
- Heard C. M., Harwood J., Maguire P., McNaughton G., Pugh W. J. Simultaneous penetration of NSAID and essential fatty acid esters as a dual-action anti-arthritis therapy. Perspectives in Percutaneous Penetration, K. R. Brain, K. A. Walters. Vol 8a, STS Publishing, Cardiff 2002; 93
- Simon G. A., Maibach H. I. The Pig as an experimental animal model of percutaneous permeation in man: qualitative and quantitative observations—an overview. Skin Pharmacol. Appl. Skin Physiol. 2000; 13: 229–234, [PUBMED], [INFOTRIEVE], [CROSSREF]
- Taylor L. J., Lee R. S., Long M., Rawlings A. V., Tubek J., Whitehead L., Moss G. P. Effect of occlusion on the percutaneous penetration of linoleic acid and glycerol. Int. J. Pharm. 2002; 249: 157–164, [PUBMED], [INFOTRIEVE], [CROSSREF]
- Tempel H., Tulleken J. E., Limburg P. C., Muskiet F. A. J., Rijswijk M. H. Effects of fish oil supplementation in rheumatoid arthritis. Ann. Rheum. Dis. 1990; 49: 76–80, [PUBMED], [INFOTRIEVE]
- Wang M. Y., Yang Y. Y., Heng P. W. S. Role of solvent in interactions between fatty acids-based formulations and lipids in porcine stratum corneum. J. Contr. Rel. 2003; 94: 207–216, [CROSSREF]
- Yamane M. A., Williams A. C., Barry B. W. Effects of terpenes and oleic-acid as skin penetration enhancers towards 5-fluorouracil as assessed with time-permeation, partitioning and differential scanning calorimetry. Int. J. Pharmaceut. 1995; 116: 225–237, [CROSSREF]