Abstract
The aim of the study was to determine penetration properties of Famotidine fro the formulations through colon adenocarcinoma (Caco)-2 cell monolayers and to compare in vitro with in vivo test results. It also aimed to determine the effect of particle size on the penetration properties of Famotidine when microsphere formulations were used. Famotidine was chosen as a model drug and Caco-2 cell culture model was used. Biodegradable Famotidine microspheres of poly(lactide-co-glycolide)(PLGA) polymer (50:50) were prepared by using multiple emulsion technique. Microspheres were coded according to their particle size and polymer[LHIV:60 μm Famotidine-PLGA(high viscosity), SHIV:6 μm Famotidine PLGA(high viscosity), LLIV:60 μm Famotidine-PLGA (low viscosity), SLIV:6 μm Famotidine-PLGA (low viscosity)]. Famotidine solution(5 mg/ml) and microsphere formulations were administered orally to mice and blood drug levels were determined and compared with the Caco-2 cell experiments. Permeability values of Famotidine through Caco-2 cells from various formulations were determined (log ksolution = 7, 274 ± 0, 010, log kSHIV = −3, 884 ± 0, 033, log kLHIV = −2, 300 ± 0, 009, log kSLIV = −4, 076 ± 0, 208, log kLLIV = 3, 525 ± 0, 045). Our results showed that H2 receptor antagonists alter the barrier properties of the Caco-2 cell monolayer by causing an increment in the tightness of the tight junctions. Therefore, amount of the H2 receptor antagonist-like drug at the site of action was found to be important as well as polymer type and particle size of microspheres for drug permeation. Permeation of the drug was lower when higher amounts of Famotidine were present at the diffusion site. A controlled release dosage form of H2 receptor antagonist-like drugs may be beneficial for long-term treatments.
Oral administration generally is a preferred route, considering advantages such as patient compliance and convenience. Mucin layer in the intestine regulates the diffusion of various substances from the intestinal lumen to the epithelial surface, protecting the epithelial cells from environmental stimulus. In pharmaceutics, the mucin layer works as a diffusion barrier and profoundly affects the absorption of drugs after oral administration (Desai et al. Citation1996; Greenwood and Al-Achi Citation1997; Desai et al. Citation1997). Many absorptive cell lines including colon adenocarcinoma (Caco)-2 cells produce mucin molecules. Thus, most of the adenocarcinoma cell lines can be morphologically characterized as absorptive cells (Artursson Citation1991). There are two possible pathways for permeation; the trancellular and paracellular route refers to the tight-junctional pathway between the cells and they can be considered as pores filled with water (Tanaka et al. Citation1995). Hydrophilic and charged drugs passively absorbed through the tight junctions use the paracellular route. Lipophilic drugs are rapidly absorbed transcellulary (Tanaka et al. Citation1995; Camenisch, Folkers, and Waterbeemd Citation1997).
The Caco-2 monolayer model has been widely used in many studies concerning both active and passive transport of drug molecules. In mechanistic studies of various drug additives and absorption enhancers (Hovgaard, Brondsted, and Nielsen Citation1995; Caro et al., Citation1995; Lentz et al. Citation2000), the Caco-2 monolayer model has been a good model due to its simplistic nature (Artursson Citation1991; Tanaka et al. Citation1995; Camenisch et al. Citation1997; O'leary et al. Citation1996; Foster, Yazdenian, and Audus Citation2001). The literature has reported that the H2–antagonists cause a increase in the tightness of the tight junctions specific. The H2 antagonists caused a decrease in permeability of their own transport rate across the Caco-cell monolayer (Gan et al. Citation1998).
Poly(lactide-co-glycolic acid) (PLGA) has been studied extensively as a biodegradable polymer for controlled release dosage form of many thearapeutic agents (Kim and Burgess Citation2001; Castewllanos et al. 2001; Vanrell and Refojo Citation2001). The physical properties and FDA approval of PLGA make them the most extensively studied and commercially available biodegradable, biocompatible, and bioresorbable polymers (Birnbaum et al. Citation2000).
Famotidine was chosen as a model drug and Caco-2 cell culture model was used to investigate drug permeability through the gastrointestinal cells. Biodegradable Famotidine microspheres of PLGA polymer (50:50) were prepared by the multiple emulsion technique.
Our other objective in this study was to investigate and determine the efficacy of uptake of various size biodegradable Famotidine PLGA microparticles.
MATERIALS AND METHODS
Famotidine was supplied by Ltd. Turkey. Transwell (24 mm diameter, 0.4 mm pore size polycarbonate membrane filter) was purchased from Costar-US. Caco-2 cells were obtained from American Type Culture Collection (US) DMEM, FBS, penicilline streptomycin solution were purchased from Gibco Lab. (U.K.) Methanol and potassium of hydrogen phosphate (HPLC grade) were purchased from Sigma. All other chemicals and solvents were of analytical grade except those used in the high performance liquid chromatography (HPLC) procedure, which were of HPLC grade.
In Vitro Preparation of PLGA Microparticles
Emulsification/solvent evaporation process has been used to prepare microspheres (Birnbaum et al. Citation2000; Değim, ünal, and Abbasoğlu Citation2001). Briefly, 400 mg PLGA and 10 mg Famotidine were added to 5 ml of solvent (dichloromethane and methanol (90:10). The dispersion was poured into 60 ml of aqueous PVA solution (0.4%) solution and the medium was stirred using ultraturrax at various speed to obtain microparticles with various dimensions. After 2 min, the emulsion was quickly transferred into 800 ml of distilled water and stirred at 400 rpm until the organic solvent was completely evaporated (3 hr). The microspheres were collected by centrifugation, washed with distilled water, and dried at room temperature. Microspheres were coded according to their particle size and polymer used. LHIV:Famotidine-PLGA (high viscosity) microspheres with the diameter of 60 μm SHIV:Famotidine-PLGA (high viscosity) microspheres with the diameter of 6 μm LLIV:Famotidine-PLGA (low viscosity) microspheres with the diameter of 60 μm SLIV:Famotidine-PLGA (low viscosity) microspheres with the diameter of 6 μm.
Microspheres Size and Shape
The microspheres were redispersed in water and the mean particle size was measure using laser diffraction particle size analyzer (Sympatec, Germany).
Drug Content in the Microspheres
The PLGA microspheres were dissolved in 5 mL methylene chloride and polymer was then precipitated with methanol. The amount of entrapped drug was then determined by HPLC (Değim et al. Citation2002). The HPLC work was performed with an HP pump model 1050; Lichrosorb RP 18 column (10 μm, 250 × 4.1 mm) and an HP series 1050 ultraviolet-detector (26 nm). The mobile phase consisted of 15% methanol, 16% phosphate buffer, and 69% water. The flow rate was 2 ml·min−1. All experiments were done in triplicate.
In Vitro Release Studies
First, 20 mg of microspheres were placed in 25 mL of DMEM in a glass vial. The microspheres in the medium were shaken 50 cpm in a thermostated bath at 37°C. The samples were removed at appropriate time intervals and analyzed using HPLC.
Diffusion/Permeation Studies
Caco-2 cell monolayers were prepared by cultivating Caco-2 cells of polycarbonate membrane filter for 3 weeks. The pore size of the membrane was 0.4 μm and the diameter of the membrane was 24 mm. Radial diffusion cells were used for the determination of drug absorption. Caco-2 cell monolayer on the membrane filter was fixed between the donor and receptor compartment. Famotidine solution (5 mg/ml) or Famotidine microspheres (20 mg) in DMEM applied to the donor chamber (4 ml), receptor compartment (4 ml) also was filled with DMEM solution. Transport studies across Caco-2 monolayers were performed in a carbogen atmosphere at 37°C. The samples were collected from the donor compartment during 30 hr and analyzed by HPLC. Permeability values were calculated by dividing the flux values (μg/h) with the concentrations of donor phase (μg/ml).
In Vivo Animal Experiments
Swiss-Albino female mice (n = 24), weighing 30.0±1.0 g were used for in vivo studies. Famotidine microspheres were administered orally to the mice with dose of 0.2 g/kg. Plasma samples were collected directly from the heart during 24 hr. The plasma was separated from the collected blood samples by centrifugation at 3000 × g for 15 min and stored at −70°C until used. The plasma samples were analyzed, and the plasma profiles of Famotidine will obtained and the cumulative Famotidine released (in vitro from the Caco-2 cell monolayers experiments).
RESULTS and DISCUSSION
Inverted Microscopy
The cells were trypsinized 0.5 ml of the cell suspension containing approximately 10 cells/ml and the formation of the cell monolayer was checked using the electrical resistance of the system. It also was checked by microscopic analysis (the inverted microscope Olympus CK2, Japan).
Encapsulation Efficiency Percent
The amount of entrapped drug was determined by HPLC (Değim and Ağabeyoğlu Citation2002) as mentioned. Encapsulation efficiencies were found to be 87% and 81% when high viscosity polymer was used. The particle sizes were determined as 60 and 6 μm. The encapsulation efficiencies were determined as 88% and 80% when the low viscosity polymers were used. Their particle sizes were 60 and 6 μm respectively.
Evaluation of In Vitro Release Studies
There was an initial burst release of Famotidine from the microspheres. This burst effect especially was observed in SHIV microspheres. This burst effect was probably due to release from the surface of the microspheres ().
Transport Studies
The Caco-2 cell monolayer model was used as a transport membrane in this study. The Caco-2 cells have similar properties with absorptive intestinal cells containing similar microvilli, the carrier-mediated transport systems, and paracellular pathways for the transport through tight junctions. Therefore, the Caco-2 cell monolayers have been widely preferred and used for in vitro drug absorption studies as a good model (Desai et al. Citation1996, Citation1997). The use of biodegradable polymers such as PLGA is widely used as a matrix material for the production of microparticulate systems (Birnbaum et al. Citation2000; Kim and Burgess Citation2001; Castewllanos et al. 2001). In this study, Famotidine microspheres were prepared and determined the penetration properties of Famotidine through Caco-2 cell monolayer. The effect of particle size on the penetration properties also was examined when various Famotidine microspheres were used. The drug release from PLGA microspheres depended on various factor including the polymer composition, polymer molecular weight, and the particle size of microspheres (Tunçay et al. Citation2000). There was a significantly large burst of release for the microparticles encapsulated PLGA (Birnbaum et al. Citation2000; Tunçay et al. Citation2000). Therefore, Caco-2 cell monolayer was chosen and used for Famotine penetration experiments from prepared microspheres with PLGA, in this study.
The electrical resistance of the Caco-2 cell lines were measured as 830–1050 Ωcm−2 at room temperature in DMEM medium. The drug permeations through Caco-2 cell monolayer were determined and compared for LHIV, SHIV, LLIV, and SLIV ( and ).
Figure 2 Penetrated amount of Famotidine through cm2 of Caco-2 cell monolayer from Famotidine solution and microspheres (LHIV = 60 μm, SHIV = 6 μm) prepared with PLGA (high viscosity).
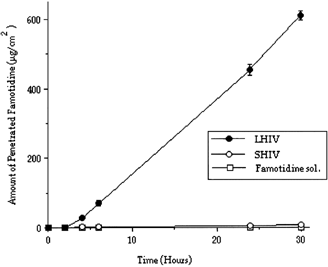
Figure 3 Penetrated amount of Famotidine through cm2 of Caco-2 cell monolayer from Famotidine solution and microspheres (LLIV = 60 μm, SLIV = 6 μm) prepared with PLGA (low viscosity).
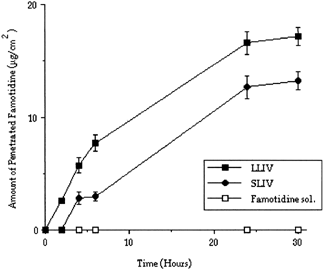
Permeability values also were calculated and are given in . Permability values indicate that the Famotidine molecule penetrates faster from LHIV microspheres than others Famotidine molecules from solution and SHIV microspheres were observed not to be penetrated as high as LHIV microspheres. Exactly similar properties were observed with SLIV and LLIV microspheres and Famotidine solution. A lower amount of Famotidine penetrated through Caco-2 cell monolayer when SLIV and LLIV microspheres were used comparing with SHIV and LHIV microspheres. In our study, the release of Famotidine from PLGA microspheres showed a release depended polymer type. Faster releases from microspheres to the medium were observed when low viscosity polymer was used.
Table 1 Calculated permeability values of Famotidine through Caco-2 cell monolayer from various formulations
When penetration of high viscosity PLGA microspheres were tested in vitro, using Caco-2 cell monolayer, the amount of the released Famotidine were determined as 2. (LHIV) and 0.053% (SHIV), respectively, during 30 hr. When PLGA microspheres prepared with low viscosity PLGA will considered, it was only 0.064% (LLIV) and 0.03% (SLIV) for the same time period ( and ).
As mentioned, the H2 receptor antagonist drug molecules penetrates through intestinal mucosa and causes an increase in the tightness of the tight junctions (Gan et al. Citation1998). Famotidine, also an H2 receptor antagonist, may act similarly, resulting in low penetration. We have examined the effect of Famotidine on the function of the tight junctions in in vitro experiments. We thought that to provide a lower amount of Famotidine to be penetrated through intestinal mucosa may be a good idea because a higher amount of Famotidine penetration can be obtained using controlled release of Famotidine from the microspheres by providing a lesser amount of H2 receptor antagonists to the tight junctions.
Our in vitro experiments showed that, Famotidine-PLGA (high viscosity) microspheres with the diameter of 6 μm (SHIV) penetrated the Caco-2 cell monolayer, but the amount of Famotidine in the receptor phase were found to be very low. When Famotidine-PLGA (high viscosity) microspheres with the 60 μm of diameter (LHIV) were used, higher Famotidine penetrations were observed. In other words, higher penetrations were observed with Famotidine microspheres that release the drug with lower rate. Because a high amount of H2 receptor antagonists (like Famotidine) at the site of action (at the area of diffusion) augments the tightness of the tight junctions, barrier properties were increased when Famotine solutions or fast drug releasing microspheres were used.
Famotidine plasma levels after oral administration of Famotidine solution and microspheres to the mice are shown in .
Figure 4 Famotidine plasma levels after oral administration of Famotidine solution and microspheres.
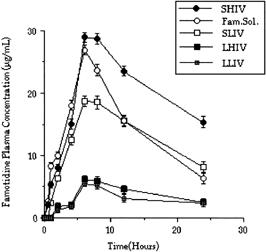
Interestingly, in vivo test results showed that higher blood Famotidine levels can be obtained when Famotidine-PLGA (high viscosity) microspheres with the diameter of 6 μm (SHIV) were used in comparison with LHIV. SHIV microspheres were determined as fast drug releasing microspheres earlier. In fact, a faster release rate was observed with the formulation prepared using high viscosity polymer (SHIV, 6 μm), because of a bigger surface are of the microspheres. Therefore, higher blood Famotidine levels were obtained with SHIV than with LHIV microspheres. Exactly similar in vivo results were obtained with SLIV and LLIV microspheres. Famotidine solutions gave even higher blood levels. The surface area of the intestine (in vivo) is significantly large compared with the surface area of the Caco-2 cell monolayer used in in vivo experiments. When the conditions were considered in in vitro experiments, we noticed that the surface area of the Caco-2 cell monolayer was quite small and the drug localized to the diffusional area. Therefore, the negative effects of H2 receptor antagonist Famotidine were easily observed, and it reduced the penetration by augmenting the tightness of the cell junctions.
The electrical resistances of the Caco-2 cell lines also were measured when Famotidine solution was present in the donor phase and we found it to be 1.766 times higher than the electrical resistance determined in DMEM medium after 24 hr. An increased permeability value was obtained when a higher amount of Famotidine was provided at the receptor phase as it reported in the literature (Gan et al. Citation1998). But in in vivo experiments, the drug molecules and also the microspheres were not localized in the intestine (as in in vitro experiments) because prepared microspheres have no adhesive properties at all.
Therefore, Famotidine microspheres behaved differently in augmenting the barrier properties of the membranes during in vivo experiments Microspheres dispersed all over the intestine and the drug cannot reach enough concentration at the penetration site of the intestinal cell membranes. Therefore, no big augmenting effect on the tight junctions was observed. This issue was found to be very important and necessary to address for Caco-2 monolayer experiments.
When the in vitro/in vivo correlations were considered, good correlations were obtained between penetrations of Famotidine through Caco-2 cell monolayer and percent amount of Famotidine found in mice plasma (for especially SHIV, 6 μm) microspheres ().
CONCLUSION
The particle size of the microsphere was important for the Famotidine penetration through the cell membranes when PLGA polymers were used. We emphasize that the Famotidine is a H2 receptor antagonist and penetrates through intestinal mucosa and causes an increment in the tightness of the tight junctions. Therefore, when H2 receptor antagonists are planned to be used for Caco-2 cell monolayer penetration experiments, extra attention and concern are needed. By preparing a controlled release dosage from of Famotidine, the penetration of the molecule can be increased/controlled which may be useful for long-term treatments with Famotidine.
Using the Caco-2 cell monolayer model, in vivo/in vitro correlations can be obtained and useful a precise predictions can be made.
REFERENCES
- Artursson P. Cell cultures as models for drug absorption across the intestinal mucosa. Therap. Drug Carrier Sys. 1991; 8: 305–330
- Birnbaum D. T., Kosmala J. D., Henthom D. B., Peppas L. B. Controlled release of β estradiol from PLAGA microparticles: the effect of organic phase solvent on encapsulation and release. J. Cont. Rel. 2000; 65: 375–387, [CROSSREF]
- Camenisch G., Folkers G., Waterbeemd H. Comparison of passive drug transport through Caco-2 cells and artificial membranes. Int. J. Pharm. 1997; 147: 61–70, [CROSSREF]
- Caro I., Boulenc X., Rousset M., Meunier V., Bourrié M., Julian B., Joyeux H., Roques C., Berger Y., Zweibaum A., Fabre G. Characterisation of a newly isolated caco 2 clone(TC-7), as a model of transport processes and biotransformation of drugs. Int. J. Pharmaceuts. 1995; 116: 147–158, [CROSSREF]
- Castewllanos I. J., Carrasquillo K. G., Lopez J. J., Alvarez M., Griebenow K. Encapsulation of bovine serum albumin in poly(lactide-coglycolide) microspheres by the solid in on in water technique. J. Pharm. Pharmacol. 2000; 53: 167–178, [CROSSREF]
- Değim Z., Ünal N., Abbasoğlu U. Caco-2 cell culture as a model for famotidine and insuline absorption across the intestinal mucosa. World Congress of Pharmacy and Pharmaceutical Sciences. 61st International Congress of FIP, Singapore 2001, Abstracts, 53
- Değim Z., Ağabeyoğlu İ. Nonisothermal stability tests of famotidine and nizatidine. Il Farmaco 2002; 57: 729–735, [CROSSREF]
- Desai M. P., Labhasetwar V., Walter E., Levy R. J., Amidon G. L. The mechanism of uptake of biodegradable microparticles in Caco-2 cells is size dependent. Pharm. Res. 1997; 14: 1568–1573, [PUBMED], [INFOTRIEVE], [CROSSREF]
- Desai M. P., Labhasetwar V., Amidon G. L., Levy R. J. Gastrointestinal uptake of biodegradable microparticles: Effect of particle size. Pharm. Res. 1996; 13(12)1838–1845, [PUBMED], [INFOTRIEVE], [CROSSREF]
- Foster K. A., Yazdenian M., Audus K. L. Microparticulate uptake mechanisms of in vitro cell culture models of the respiratory epithelium. J. Pharm. Pharmacol. 2001; 53: 57–66, [PUBMED], [INFOTRIEVE], [CROSSREF]
- Gan L. L., Yanni S., Thakker D. R. Modulation of the tight junctions of the Caco-2 cell monolayers by H2-antagonists. Pharm. Res. 1998; 15: 53–57, [PUBMED], [INFOTRIEVE], [CROSSREF]
- Greenwood R., Al-Achi A. Human insulin diffusion profile through a layer of caco 2 cells. Drug Dev. Ind. Pharm. 1997; 23: 221–224
- Hovgaard L., Brondsted H., Nielsen H. M. Drug delivery studies in caco-2 monolayers. II. absorption enhancer effects of lysophosphatdylcholines. Int. J. Pharm. 1995; 114: 141–149, [CROSSREF]
- Kim T. K., Burgess D. J. Formulation and release characteristics of poly(lactic-cc glycolic acid) microspheres containing chemically modified protein. J. Pharm. Pharmacol. 2001; 53: 23–31, [PUBMED], [INFOTRIEVE], [CROSSREF]
- Lentz K. A., Hayashi J., Lucisano L. J., Polli J. E. Development of a more rapid, reduced serum culture system for caco-2 monolayers and application to the biopharmaceutics classication system. Int. J. Pharmaceut. 2000; 200: 41–51, [CROSSREF]
- O'leary G., Değim Z., Gumbleton M., Mcguigan C. Permeability of caco-2 novel d4t-monophosphate prodrugs. Eur. J. Pharmaceuti. Sci. 1996, 4: S194
- Tanaka Y., Taki Y., Sakane T., Tanekazu N., Sezaki H., Yamashita S. Characterization of drug transport through tight-juntional pathway in caco-2 monolayer: comparison with isolated rat jejenum and colon. Pharmaceut. Res. 1995; 12: 523–528, [CROSSREF]
- Tunçay M., Çaliş S., Kaş H. S., Ercan M. T., Peksoy İ., Hincal A. Diclofena sodium incorporated PLGA (50:50) microspheres: formulation considerations and in vitro/in vivo evaluation. Int. J. Pharm. 2000; 195: 179–188, [CROSSREF]
- Vanrell R. H., Refojo M. F. Biodegradable microspheres for vitreoretinal drudelivery. Adv Drug Del. Rev. 2001; 52: 5–16, [CROSSREF]