Abstract
Chitosan, a natural-based polymer obtained by alkaline deacetylation of chitin, is nontoxic, biocompatible, and biodegradable. These properties make chitosan a good candidate for conventional and novel drug delivery systems. This article reviews the approaches aimed to associate bioactive molecules to chitosan in the form of colloidal structures and analyzes the evidence of their efficacy in improving the transport of the associated molecule through mucosae and epithelia. Chitosan forms colloidal particles and entraps bioactive molecules through a number of mechanisms, including chemical crosslinking, ionic crosslinking, and ionic complexation. A possible alternative of chitosan by the chemical modification also has been useful for the association of bioactive molecules to polymer and controlling the drug release profile. Because of the high affinity of chitosan for cell membranes, it has been used as a coating agent for liposome formulations. This review also examines the advances in the application of chitosan and its derivatives to nonviral gene delivery and gives an overview of transfection studies that use chitosan as a transfection agent. From the studies reviewed, we concluded that chitosan and its derivatives are promising materials for controlled drug and nonviral gene delivery.
Controlled drug delivery technology represents one of the frontier areas of science, which involves multidisciplinary scientific approach, contributing to human health care. The development of appropriate carriers for drug delivery is a challenge for biomedical scientists. Peptides, proteins, oligonucleotides, and genes are unstable compounds that need to be protected from degradation in the biological environment. Moreover, their efficacy is highly limited by their ability to cross biological barriers and reach the target site. As such, the future of these molecules as therapeutic agents clearly depends on the design of an appropriate carrier for their delivery to the body. Several studies have been reported so far in the development of these carriers, among which the design of biodegradable nanoparticles has drawn considerable interest. In particular, nanoparticles made of hydrophobic or amphiphilic polymers such as poly (lactide-co-glycolide) (PLGA) and poly(lactic) acid (PLA)–poly(ethylene)glycol (PEG) offer great promise (Blanco and Alonso Citation1997; Tobio et al. Citation1998; Tobio et al. Citation2000). But these nanoparticles have a limitation in their preparation procedure, which requires the use of organic solvents and surfactants as well as sonication.
A challenging alternative to these hydrophobic particles are colloidal carriers made of hydrophilic polymers. For this application, chitosan has come to be a particularly interesting polymer for the association and delivery of labile macromolecular compounds. Chitosan, α (1–4) 2-amino 2-deoxy β -D glucan, has structural characteristics similar to glycosaminoglycans. This polycationic biopolymer is generally obtained by alkaline deacetylation from chitin, which is the main component of the exoskeleton of crustaceans, such as shrimps (Muzzarelli Citation1973). The physicochemical and biological properties of chitosan led to the recognition of this polymer as a promising material for drug delivery. It is extremely important that chitosan be hydrosoluble and positively charged. These properties enable it to interact with negatively charged polymers, macromolecules, and polyanions on contact in an aqueous environment. It has the special feature of adhering to mucosal surfaces, a fact that makes it a useful polymer for mucosal drug delivery (Lehr et al. Citation1992; Artursson et al. Citation1994; Lueben et al. Citation1996; Illum, Farraj, and Davis Citation1994). Chitosan has interesting biopharmaceutical characteristics such as pH sensitivity, biocompatibility, and low toxicity (Hirano et al. Citation1990, Citation1989; Bersch, Nies, and Liebendorfer Citation1995). Moreover, chitosan is metabolized by certain human enzymes, especially lysozyme, and is considered as biodegradable (Muzzarelli Citation1997; Koga Citation1998). In addition, chitosan acts as a penetration enhancer by opening epithelial tight-junctions (Schipper et al. Citation1997; Illum Citation1998). Due to these favorable properties, the interest in chitosan and its derivatives as excipients in drug delivery has increased in recent years.
A few review articles on the potential of chitosan for pharmaceutical applications have been published (Sinha et al. Citation2004; Hejazi and Amiji Citation2003; Borchard Citation2001; Lubben et al. Citation2001). Therefore, our review focuses on the specific features and applications of the chitosan-based colloidal systems designed for the association and delivery of drugs. At present, a variety of chitosan-based colloidal delivery vehicles have been described for the association and delivery of drugs. In this review, the major classes of colloidal delivery vehicles (microparticles/microspheres, nanoparticles, beads, hydrogels, and selfassemblies) and their applications to the controlled drug delivery are discussed. Special attention is given to chemical modification of chitosan to improve the absorption of hydrophilic or hydrophophic macromolecules and mucoadhesive properties. In addition, the formation and controlled drug delivery properties of chitosan complexes with macromolecules and chitosan-coated colloidal systems is reported. Our final section is focused to the application of chitosan and its derivatives as transfection agents to nonviral gene delivery.
CROSSLINKED CHITOSAN PARTICLES
Covalently Crosslinked Particles
Crosslinking of biodegradable polymers potentially is important to control swelling and degradation rates. The earliest work in chitosan nanostructures predominantly involved chemical crosslinking reactions to the polymer chain. Watzke and Dieschbourg (Citation1994) formed chitosan-silica nanocomposites by reacting tetramethoxysilane to the hydroxyl groups on the chitosan monomer. However, attempts were not made to associate active agents to the polymer network. Ohya et al. (Citation1994) was the first to present data involving chitosan nanospheres for drug, 5-fluorouracil (5-FU) delivery applications using water-in-oil emulsion method followed by glutaraldehyde crosslinking of the chitosan amino groups. Yao et al. (Citation1995) prepared an inverse emulsion of 5-FU or its derivative solution of hydrochloric acid of chitosan in toluene containing span 80. Chitosan was crosslinked with Schiff's salt formation by adding glutaraldehyde into toluene solution. At the same time, the amino derivatives of 5-FU were immobilized, obviously resulting in an increase in the amount of drug within the microspheres. The microspheres were coated with anionic polysaccharides (e.g., carboxymethylchitin, etc.) through a polyion complex formation reaction.
In the case of lipid-coated microsphere, the microspheres, along with dipalmitoyl phosphalidyl choline (DPPC), were dispersed in chloroform. After evaporation of the solvent, microspheres were obtained coated with a DPPC lipid multilayer that exhibited a transition temperature of a liquid crystal phase at 41.4°C. A comparative study on the release of 5-FU and its derivatives from a polysaccharide-coated microspheres was carried out in physiological saline at 37°C. Data indicated that the 5-FU release rate decreased in the order: free-5-FU > carboxymethyl type 5-FU > ester type 5-FU. The results revealed that the coating layers on the microspheres were effective barriers to 5-FU release. The lipid multilayer with a homogeneous composition generally shows a transition of gel-liquid crystal. When the temperature is raised to 42°C, which is higher than the phase transition of 41.4°C, the release amount of 5-FU increased, the amount of drug delivered decreased at 37°C, which is lower than the transition temperature. Due to the improved recognition function of polysaccharide chains for animal cell membranes, it is reasonable to develop targeting delivery systems from polysaccharide-coated microspheres.
Different preparation, crosslinking methods and types of chitosan can vary the size and degradation rate of chitosan particles. Akbuga and Durmaz (Citation1994) studied the effect of chitosan type and concentration, drug concentration, crosslinking process, the viscosity of oil, and stirring rate during the preparation on microsphere properties. In this study, chitosan microspheres containing furosemide were prepared from water/oil emulsion system using liquid paraffin as the external phase and a solution of chitosan in acetic acid as the disperse phase. Discrete spherical furosemide microsperes having a 350–690 μ m diameter range were produced. Results showed that microshere properties are affected by preparation variables such as the type and concentration of chitosan, glutaraldehyde concentration, drug concentration, the viscosity of oil, and stirring rate.
Genta et al. (Citation2000) reported the influence of glutaraldehyde on drug release and mucoadhesive properties of chitosan microsperes. Chitosan microspheres were prepared by a modified emulsification/separation method and loaded with a hydrophilic model drug, theophylline. Glutaraldehyde was used at different concentrations to prepare chitosan microspheres with various crosslinking densities modulating the rate of theophylline release. Glutaraldehyde reacts with chitosan and it crosslinks in inter- and intramolecular fashion through the formation of covalent bonds mainly with the amino groups of the polysaccharide. Since chitosan mucoadhesive properties seem to be mediated by ionic interactions between positively charged amino groups in polymer and negatively charged sialic acid residues in mucus, in this work the effect of glutaraldehyde on the chitosan/mucus layer interactions was verified by a new in vitro technique based on electron microscopy. The suitable drug release rate was obtained with the lowest glutaraldehyde concentration (7 or 15% w/v) to limit the toxicological effect of the crosslinking agent. Scanning electron and transmission electron microscope investigations showed that the chitosan/mucin chain interaction is related to the presence of glutaraldehyde. The results also showed that uncrosslinked chitosan microspheres have high affinity for mucin whereas crosslinked microspheres lose this property.
Chitosan microparticles for colonic delivery of prednisolone were prepared by a precipitation/coacervation technique using chitosan and sodium sulphate as precipitant agent (Berthold et al. Citation1996, Citation1996a). These microparticles also were crosslinked to increase their acid stability in the acidic environment of the stomach. In spite of the increased acid stability, crosslinking with glutaraldehyde may result in a drastic decrease of bioadhesiveness and increased toxicity (Genta et al. Citation2000; Carreno and Duncan Citation1997). Another chitosan-based system for colonic drug delivery was described by Lorenzo et al. (Citation1998). The chitosan microparticles were loaded with sodium diclofenac and incorporated into eudragit polymers. This approach resulted in controlled drug release by using pH-dependent and bacterial degradation of chitosan in the colonic environment.
In vivo evaluation in rabbits has shown that chitosan nanoparticles (300–400 nm) increase nasal insulin absorption to a greater extent than chitosan solutions, probably due to the intensified contact of the nanoparticles at the nasal mucosa as compared with chitosan solutions (Fernandez et al. Citation1999, Citation1999a; Williams et al. Citation1998). Chitosan microspheres and solutions also have been tested for their nasal clearance characteristics in human volunteers using γ -scintigraphy. The microspheres and solutions resulted in four and two times longer clearance half-lives (compared with controls), respectively. These observations support the hypothesis that chitosan delivery systems can reduce the clearance rate from the nasal cavity, thereby increasing the contact time of the delivery system with the nasal mucosa and providing the potential for raising the bioavailability of hydrophilic drugs incorporated into these systems.
Gupta and Ravikumar (Citation2000) have discussed the preparation techniques and characteristics of novel pH-sensitive beads for controlled release formulations, based on crosslinked chitosan with glutaraldehyde interpenetrating glycine polymer network. The release experiments were performed using thiamine hydrochloride as a model drug. The amount of drug release was found to be higher in acidic than in basic solution. It was explained by considering the rate of diffusion from the swollen beads in acidic and basic media. In basic medium (pH 7.4), a limited swelling of the beads inhibits the diffusion of drugs at a faster rate as it occurs in acidic medium (pH 2). The pH dependent swelling behavior of chitosan beads makes them appropriate for delivery of drugs at a controlled rate. Several works (Nishimura et al. Citation1986; Ida et al. Citation1998) have reported simple coacervation of chitosan in the production of chitosan beads.
The porosity and strength of the beads correspond to the concentration of the chitosan–acid solution, the degree of N-deacetylation of chitosan, and the type and concentration of coacervation agents used. It was confirmed that the porous surface of chitosan beads make good cell culture carrier. Hayashi and Ikada (Citation1991) have immobilized protease onto the porous chitosan beads that carry active groups with a spacer and found the immobilized protease had higher pH and thermal storage stability and gave rather higher activity toward the ester substrate, N–benzyl-L-arginine ethyl ester. In addition, Nishimura et al. (Citation1986) investigated the possibilities of using chitosan beads as a cancer chemotherapeutic carrier for adriamycin. Chandy et al. (Citation1987, Citation1992, Citation1993) prepared chitosan microbeads for oral sustained delivery of nefedipine, ampicillin, and various steroids by adding to chitosan and then going through a simple coacervation process. These coacervate beads can be hardened by crosslinking with glutaraldehyde or epoxychloropropane to produce microcapsules containing rotundine (Yao et al. Citation1995). The release profiles of the drugs from all these chitosan delivery systems were monitored and in general showed the higher release rates at pH 1–2 than that at pH 7.2–7.4. The effect of the amount of drug loaded, the molecular weight of chitosan, and the crosslinking agent on the drug delivery profiles have been discussed.
A novel technique for the preparation of pH-sensitive beads of chitosan was reported. Declofenac sodium, thyamine hydrochloride, chlorphenramine maleate, and isoniazid were used as model drugs (Gupta et al. Citation2000, Citation2000a, Citation2000b). In these studies, glycine and PEG were employed as spacer groups to enhance the flexibility of the polymer networks and influence the swelling behavior through macromolecular interactions (Yao et al. Citation1995). The swelling behaviour, solubility, hydrolytic degradation, and drug loading capacity of the beads were investigated (Gupta et al. Citation1999, Citation2000, Citation2000a; Citation2000b). The swelling ratio of the beads at pH 2.0 was obviously higher than that at pH 7.4. This pH-sensitive swelling was due to the transition of bead network between the collapsed and the expanded rates, which was related to ionization degree of amino group on chitosan in different pH solutions. The amounts and percent release in chitosan-PEG system were a bit higher, when compared with the chitoan-glycine system, due to the water diffusivity and pore forming properties of PEG. The effects of the amount of drug loaded and the crosslinking agent on the delivery profiles were reported as well. These studies clearly demonstrated the feasibility of synthesizing stable, reproducible different kinds of chitosan particles or beads that could entrap and deliver drugs.
However, the later discovery of the negative effects of chemical crosslinking with glutaraldehyde on cell viability and the integrity of macromolecular drugs shifted general interest to a less harsh procedure for synthesis of these colloidal systems. Mi et al. (Citation2000, Citation2002) prepared an injectable chitosan-based delivery system with low cytotoxicity. The chitosan microsphere with small particle size, low crystallinity, and good sphericity was prepared by a spray-drying methods followed by crosslinking with a naturally occuring crosslinking agent, genipin. The results of the study demontrated that the genipin-crosslinked chitosan microspheres had a superior biocompatibility and a slower degradation rate than the glutaraldehyde-crosslinked chitosan microspheres. They concluded that the genipin-crosslinked chitosan microspheres may be a suitable polymeric carrier for long-acting injectable drug delivery.
Ionically Crosslinked Particles
The cationic nature of chitosan has been conveniently exploited for the development of particulate drug delivery systems. Aside from its complexation with negatively charged polymers, an interesting property of chitosan is its ability to gel on contact with specific polyanions. This gelation process is due to the formation of inter- and intramolecular crosslinkages mediated by these polyanions. Bodmeier, Chen, and Paeratakul (Citation1989) first reported the inotropic gelation of chitosan with tripolyphosphate (TPP) for drug encapsulation. Alonso et al. (Citation1998) developed chitosan nanoparticles involving the addition of an alkaline phase (pH 7–9) containing TPP into an acidic phase (pH 4–6) containing chitosan. In this method, nanoparticles are formed immediately upon mixing of the two phases through inter- and intramolecular linkages created between TPP phosphates and chitosan amino groups. The result showed that to produce a high yield of nanoparticles, the chitosan:TPP weight ratio should be within the range of 3:1–6:1.
The introduction of hydrophilic polymers in the chitosan nanoparticle increases their versatility in terms of the association and delivery of proteins and their susceptibility to interact with biological surfaces. Interesting properties have been observed with nanoparticles made of chitosan and a diblock copolymer of ethylene oxide and propylene oxide (PEO-PPO) (Calvo et al. Citation1997). The size and zeta potential of these nanoparticles were conveniently modulated by varying the ratio of chitosan:PEO-PPO. The increased particle size and reduced zeta potential observed with nanoparticles containing PEO-PPO suggested that this copolymer becomes integrated into the nanoparticles structure. This result forms a chitosan/PEO semi-interpenetrating network via intermolecular hydrogen bonds between the electronegative oxygen of the polyether and the electropositive amino hydrogen of chitosan (Yao et al. Citation1994). The coating of a PEG on the surface of preformed nanoparticles was reported to modify the surface properties of chitosan nanoparticles. This firmly attached PEG coating was found to decrease significantly the positive surface charge of the particles, noticeably improving their biocompatibility (Calvo et al. Citation1998).
Chitosan nanoparticles appeared to be a promising system for the association and delivery of sensitive macromolecules such as bovine serum albumin (BSA), tetanus toxoid, diphtheria toxoid, and the peptide insulin due to mild condition requirements for their formation (Calvo et al. Citation1997; Urrusuno et al. Citation1999). Protein association studies carried out at different pHs indicated that, for a particular protein, the greatest loading efficiency is obtained when the protein is dissolved at a pH above its isoelectric point, so that the macromolecule is predominantly negatively charged (Calvo et al. Citation1997). This observation suggests that the mechanism of association of proteins to chitosan is, at least, partially mediated by an ionic interaction between the two macromolecules. Other macromolecular compounds, such as oligo-nucleotides, also can be associated to chitosan nanopartilces efficiently. These macromolecules are able to form nanoparticulated complexes with chitosan without requiring the addition of TPP. However, the association of oligonucleotides was found to be more efficient when the nanoparticles were formed by ionic gelation with TPP (Calvo et al. Citation1998). Similar results were reported in the study of the association of plasmids to chitosan particles (Janes, Calvo, and Alonso Citation2001).
Janes et al. (Citation2001a) evaluated the potential of chitosan nanoparticles as carriers for the anthracycline drug, doxorubicin. Here, a cationic, hydrophilic molecule was entrapped into nanoparticles by ionic gelation of the positively charged chitosan. To achieve this objective, an attempt has been made to mask the positive charge of doxorubicin by complexing it with the polyanion, dextran sulphate. This modification doubled doxorubicin encapsulation efficiency relative to controls and enabled real loading up to 4% wt doxorubicin. Fluorimetric analysis of the drug released in vitro showed an initial release phase, the intensity of which depended on the association mode, followed by a very slow release. The evaluation of the activity of doxorubicin-loaded nanoparticles in cell cultures indicated that those containing dextran sulphate were able to maintain cytostatic activity relative to free doxorubicin, while doxorubicin complexed to chitosan before nanoparticle formation showed slightly decreased activity. Additionally, confocal microscopy studies showed that doxorubicin was not released in the cell culture medium but entered the cells while remaining associated to the nanoparticles. In conclusion, these studies showed the feasibility of chitosan nanoparticles to entrap the basic drug doxorubicin and to deliver it into the cells in its active form.
Shu and Zhu (Citation2002) investigated the electrostatic interactions between multivalent phosphates (phosphate, pyrophosphate, and tripolyphosphate) and chitosan, as well as the influence of electrostatic interactions on the properties of chitosan films ionically crosslinked with phosphates. The molecular structure of multivalent phosphate strongly influenced the interactions with chitosan by salt bonds. The greater number of negative charges of tripolyphosphates and pyrophosphate resulted in their greater ability to ionically crosslink chitosan, whereas a weakly electrostatic interaction between phosphate and chitosan was observed. Solution pH played an important role in the charge numbers carried by tripolyphosphate, pyrophosphate, and chitosan and hence affected their electrostatic interactions, especially in the case of pyrophosphate/chitoan. Compared with tripolyphosphate/chitosan, pyrophosphate/chitosan films exhibited better pH-sensitive swelling and drug controlled release properties due to their relatively weak interactions.
Chitosan nanoparticles obtained by ionic crosslinking with TPP display attractive features such as formation under extremely mild conditions; a homogeneous and adjustable size (from 200 nm up to 1 μ m) and a positive surface charge that can be conveniently modulated; a great capacity for the association of peptides, proteins, vaccines, oligonucleotides, and plasmids; release of the associated molecules at different rates depending on the composition of the particles; and retention of their integrity and the activity of the associated compounds following freeze-drying and reconstitution.
CHEMICALLY MODIFIED CHITOSAN PARTICLES
The chemical modification of chitosan is a powerful tool to control the interaction of the polymer with drugs, to enhance the load capability and to tailor the release profile of the particles. Chemically modified chitosan improves its bulk properties for the preparation of sustained drug release systems. Many works in this context have been reported in the literature.
N-(Aminoalkyl) Chitosan
The most promising encapsulation system yet developed appears to be the encapsulation of calcium alginate beads with poly-L-lysine (PLL). However, the use of this system on a large scale, such as for oral vaccination of animals, is not feasible due to the high cost of PLL. Therefore it would be desirable to develop an economic and reliable microencapsulation system based on chitosan and alginate. The better membrane-forming properties of PLL over chitosan included. PLL containing a number of long chain alkylamino groups that extend from the polyamide backbone. These chains may extend in a number of directions and interact with various alginate molecules, through electrostatic interactions, resulting in a highly crosslinked membrane. Chitosan, on the other hand, has amino groups that are very close to the polysaccharide backbone. Interaction between the charged amino groups of chitosan and carboxylate groups of alginate may be lessened due to steric repulsion between the two molecules. Dunn and co-workers (Citation1993) attempted to mimic the properties of PLL by extending the length of the cationic spacer arm on the chitosan main chain. In the chemical modification, chitosan was reacted first with α -bromoacylbromide followed by reaction with an amine. The major problem in this procedure was the competing hydrolysis reaction of the bromoacylbromide. Furthermore, the lack of characterization of the modified chitosan caused ambiguity in the effectiveness of the chitosan modification. No significant difference was found in membrane properties between modified and unmodified chitosan. A two-step synthetic method for attaching long alkylamine side chains to chitosan is represented in .
The approach outlined in is designed to attach flexible alkylamine side chains to the chitosan backbone, possibly simulating the behavior of PLL. The presence of two amino groups in this side chain may even enhance membrane-forming properties. Chemical modification of poly(vinyl alcohol) by a similar procedure also may produce α -polyamine with membrane forming properties similar to that of PLL. All alkylation products were characterized by solution 1H- and 13C-NMR and by solid-state CP-MAS 13C-NMR. The above synthetic polymer derivatives, as well as chitosan, polyallylamine, and polyethyleneimine, were used to form membrane coatings around the calcium alginate beads in which blue dextran of molecular weight 7.08 × 104 or 26.6 × 104 was entrapped. These microcapsules were prepared by extrusion of a solution of blue dextran in sodium alginate into a solution containing calcium chloride and the membrane polymer (Dunn et al. Citation1993). Measuring the elution of the blue dextran from the capsules, spectrophotometrically, assessed membrane integrity and permeability.
Succinyl, Quateraminated, and Octanoyl Chitosan
Porous chitosan microspheres suitable for the delivery of antigen were prepared using a wet phase-inversion method by Mi et al. (Citation1999). The results showed that the pore structure of the chitosan microsphere could be modified by the change of pH value of the coagulation medium, which is the aqueous solution of TPP. High porosity of chitosan microsphere with an open porous structure on its surface was prepared by coagulation in TPP aqueous solution of pH 8.9. The porous chitosan microspheres were modified chemically with reagents to introduce carboxyl, hydrophobic acyl, and quaternary ammonium groups (). Antigen of Newcastle disease (ND) vaccine was immobilized into the pores of porous chitosan microspheres and the adsorbed antigen was assayed by the hemoglobin aggregation analytical method. Sustained release of ND vaccine's antigen was studied by an adsorption-desorption release test. The chemical modification of the porous chitosan microspheres had a strong influence on the adsorption efficiency or release rates of the antigen investigated. The porous microspheres had higher adsorption efficiency and the slower release rate of antigen when modified chemically with 3-chloro-2-hydroxypropyltrimethylamonium chloride.
Mitomycin C Conjucated N-Succinyl-Chitosan
N-succinyl-chitosan has favorable properties such as biocompatibility, low toxicity, and long-term retention in the body as a drug carrier. Succinyl-chitosan has unique characteristics in vitro and in vivo due to many carboxyl groups. For example, ordinary chitosan can be dissolved in acidic water but not in alkaline, whereas highly succinylated succinyl-chitosan (degree of succinylation > 0.65) exhibits the opposite behaviour (Yamaguchi et al. Citation1981). Succinyl-chitosan can react easily with many kinds of agents due to having –NH2 and –COOH groups in its structure. It is valuable for the drug carrier to readily prepare its conjugates with various drugs to avoid vexatious complications. Mitomycin C (MMC) is a widely used anticancer agent. Anticancer activity of MMC has the tendency to reduce by chemical modification of the aziridine ring, one of the active groups, and its effect is manifested as the substituent becomes larger (Shirahata Citation1984). Song, Onishi, and Machida (Citation1992) have attempted the preparation of the water insoluble conjugates of MMC with succinyl-chitosan. MMC, succinyl-chitosan (MW: 3.0 × 105; succinylation degree: 0.72 mol/sugar unit) and water-soluble carbodiimide (EDC) (Sheehan and Hlavka Citation1956) were mixed in water for 45 min after adjusting to pH 5.0, because the MMC content of the conjugates was lowered with the increase in the pH in the reaction medium. After that, the precipitate was isolated by filtration, washed with water, and dried in vacuum. The resulting solid was used as succinyl-chitosan-MMC (MMC content: 12%).
In addition, the preparation of microparticles was attempted (Song et al. Citation1995).
Onishi et al. (Citation2001) prepared the crosslinked conjugate microparticles of succinyl-chitosan with MMC having an adequate size for liver targeting (0.2–3 μ m). The release characteristics of succinyl-chitosan-MMC exhibited monoexponential liberation of MMC at pH 7.4 (Onishi et al. Citation1994; Onishi, Nagai, and Machida Citation1997). Prolongation of the drug release was achieved at physiological pH. Crosslinked conjugate microparticles of succinyl-chitosan with MMC exhibited a faster release as compared with succinyl-chitosan-MMC. These differences may be attributed to the stiffness of the particles (tight or loose) rather than the binding between succinyl-chitosan and MMC, because the liberation of MMC from the conjugates of MMC with succinyl-chitosan depends on the pH in the medium (Song et al. Citation1996; Sato et al. Citation1996; Kato, Onishi, and Machida Citation2000).
Sato et al.(Citation1996a) attempted to prepare water soluble succinyl-chitosan drug conjugates by mixing glutaric mitomycin C (glu-MMC) in N-N′-dimethylformamide with a solution of succinyl-chitosan in purified water. This solution was stirred at 4°C overnight after adjusting to pH 6. The MMC content of succinyl-chitosan-glu-MMC conjugates was ∼1.3% (w/w). It was difficult to obtain high MMC-loading conjugates although the reaction between succinyl-chitosan and glu-MMC produced water-soluble conjugates. That is why water–soluble conjugates of MMC with succinyl-chitosan were further examined. The procedure, based on a method described by Song et al. (Citation1992), produced water-insoluble conjugates because of crosslinking within or among the polymer supports. Since the crosslinking was considered to be formed between amino groups and carboxyl groups of succinyl-chitosan, increased N-succinylation of succinyl-chitosan was expected to reduce the crosslinking. Moreover, a small amount of EDC was employed to suppress of the condensation reaction between amino and carboxyl groups of succinyl-chitosan. In addition, a reduction of the reaction time also was applied to suppress the crosslinking. The resultant water-soluble conjugates were termed here as succinyl (II)-chitosan-MMC. Succinyl (II)-chitosan-MMC afforded high MMC content (12%) that is comparable to succinyl-chitosan-MMC.
In vitro release of MMC from water soluble conjugates depended on the pH in the medium, i.e., the release from the conjugates increased with the rise of pH in the medium (Song et al. Citation1996; Sato et al. Citation1996; Kato et al. Citation2000). Succinyl-chitosan-glu-MMC and succinyl (II)-chitosan-MMC showed a similar release rate at each pH. Both water-soluble conjugates exhibited a faster release than water-insoluble succinyl-chitosan-MMC at pH 7.4. We propose that the drug molecules are not only bound to succinyl-chitosan but also encapsulated in the precipitates due to tight crosslinking in the case of water-insoluble conjugates. The crosslinking conjugate microparticles prepared by Onishi et al. (Citation2001) improved this hyperslow release at physiological pH.
N-Alkyl and Acylated Chitosan
The introduction of an alkyl or acyl chain offers several possibilities in molecular design of chitosan. The utility of modifying chitosan with hydrophobic branches for controlling solubility properties also has been demonstrated (Holme and Hall Citation1991; Nishimura et al. Citation1993). The introduction of an alkyl chain onto a water-soluble modified chitosan (N-methylene phosphonic chitosan) offers the presence of both hydrophobic and hydrophilic branches in its structure. A methodology for the preparation of N-lauryl-N-methylene phosphonic chitosan has been developed by Ramos et al. (Citation2003) on the water soluble N-methylene phosphonic chitosan. The presence of alkyl groups in N-lauryl-N-methylene phosphonic chitosan seems to weaken the hydrogen bond and provides good solubility in organic solvents. Its chemical identity was determined by FTIR and confirmed by 1H and 13C NMR. The degree of lauryl substitution was estimated to be 0.33. As a result of the amphiphilic properties, such as surface activity typical for surfactants, this derivative opens new perspectives in pharmaceutical and cosmetic field.
N-acylation of chitosan with various fatty acid (C6-C16) chlorides increased its hydrophobic character and made important changes in its structural features. Tien et al. (Citation2003) described the N-acylation of chitosan with fatty acyl chlorides to introduce hydrophobicity for use as matrix for drug delivery (). They expected that such derivatization would reduce hydration of the matrix and play a role in network stabilization by hydrophobic interactions. In this study, unmodified chitosan exhibited a low degree of order and a weak tablet crushing strength. Chitosan acylated with a short chain length (C6) possessed similar properties but exhibited significant swelling. Acylation with longer side chains (C8–C16) resulted in a higher degree of order and crushing strength but lower swelling.
The best mechanical characteristics and drug release properties were found for palmitoyl chitosan (substitution degree 40–50%) tablets with 20% acetaminophen as a tracer. The high stability of these nanolithic tablets appears to be due to hydrophobic interactions between side chains. Drug dissolution kinetics showed longer release times for higher degrees of functionalization, i.e., 30 hr (for 47% substitution) and 90-hr (for 69% substitution), suggesting palmitoyl chitosan excipients as interesting candidates for oral and subdermal pharmaceutical applications.
It also was reported that the chitosan derivatives with both hydrophobic groups (long acyl groups) and hydrophilic groups (sulphate groups) could form micelles and solubilize hydrophobic compounds (Yoshioka et al. Citation1995). Moreover, polymermicelle is better than other carriers for use as passive targeting carrier of anticancer drugs. N-lauryl carboxymethyl chitosan with both hydrophobic and hydrophilic groups was studied by Miwa et al. (Citation1998) in connection with delivery of taxol to cancerous tissues.
Chitosan Hydrochloride
The effects of chitosan hydrochloride (Ch-HCl) on in vitro release of ofloxacin from mucoadhesive erodible ocular inserts and on the relevant ocular pharmacokinetics have been studied by Colo et al. (Citation2002).In this study, chitosan was converted into Ch-HCl by making an aqueous chitosan suspension to pH 4.7 with 1N HCl. Following filtration to remove traces of undissolved material, a small aliquot of solution was evaporated to dryness, the residue was weighed, and then the solution was diluted to a concentration of 0.5%. Medicated Ch-HCl microspheres were prepared by spray-drying 0.5% Ch-HCl solutions containing different concentrations of ofloxacin. The results showed that the addition of Ch-HCl microparticles to polyethylene oxide-based inserts produced microstructural changes that accelerated both insert erosion and ofloxacin release. The latter was accelerated more than the former, meaning that Ch-HCl increased the diffusive contribution to the release mechanism with respect to the inserts based on plain PEO.
Thiolated Chitosans
The modification of chitosan with 2-iminothiolane was studied by Schnurch, Hornof, and Zoidl (Citation2003) to improve the properties of chitosan as excipient in drug delivery systems. In this study, chitosan-2 –iminothiolane was prepared by dissolving 1 g of chitosan in 700 ml of 1% acetic acid for 5 hr and then to this solution, different amounts of 2-iminothiolane HCl were added and the pH adjusted with 5M NaOH. avoid an oxidation process during the coupling reaction optionally 2-mercaptoethanol was added in a final concentration of 3% (v/v). After an incubation period of 24 hr at room temperature under continuous stirring, the resulting polymer conjugates were dialyzed against 5 mM HCl, two times against 5 mM HCl containing 1% NaCl, against 5mM HCl, and finally against 1mM HCl. Thereafter samples were lyophilized by drying at −30°C. The reaction scheme is shown in .
Figure 4 Synthetic pathway of (a) chitosan-4-thio butylamidine conjugate, and (b) chitosan-thioglycolic acid conjugate.
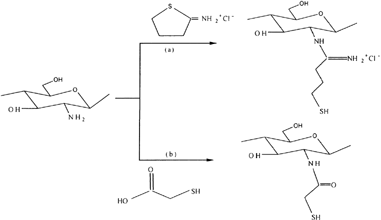
The results showed that the modification of chitosan with 2-iminothiolane exhibiting excellent in situ gelling properties due to the formation of disulfide bonds based on an oxidation process of the immobilized thiol groups under physiological conditions. In addition, the mucoadhesive and controlled drug releasing properties also were strongly improved by the covalent attachment of thiol groups on chitosan. Roldo et al. (Citation2004) studied the influence of the molecular mass and the amount of immobilized thiol groups on the mucoadhesive properties of chitosan-4-thiobutylamidine (Ch-TBA) conjugates. This study reported that the mucoadhesive properties are increased when increasing the level of 4-thiobutylamidine substructures immobilized on the polymer. In addition, thiolated chitosan of medium molecular weight was identified as comparatively the most mucoadhesive polymer. Recently, Schnurch, Guggi, and Pinter (Citation2004) reported the permeation-enhancing effect of Ch-TBA conjugate in combination with the permeation mediator glutathione. Release studies of this conjugate were performed with tablets comprising 10% cefadroxil used as model drug, 10% glutathione, and 80% Ch-TBA conjugate at pH 6.8 and 37°C. Results showed a 3-fold higher permeation enhancing effect of the Ch-TBA conjugate/glutathione system in comparison to unmodified chitosan. Mucoadhesion studies revealed that the lower the pH of the thiolated chitosan is, the higher are its mucoadhesive properties. Release studies showed a sustained release of both cefadroxil and glutathione over several hours.
Similarly, mucoadhesive properties of chitosan covalently attached with thioglycolic acid were studied by Kast and Schnorch (Citation2001). The results showed that chitosan-thioglycolic acid (Ch-TGA) conjugates exhibiting an up to 10 times improved mucoadhesion. The viscoelastic properties of Ch-TGA conjugates with different amounts of thiol groups were also studied (Hornof, Kast, and Schnurch Citation2003). The elastic properties of the conjugates were found to increase with increasing thiol group content at pH 5.5. Accordingly, proof that chitosan modified by the introduction of thiol groups has in situ gelling properties due to the formation of inter- and intramolecular disulfide bonds at physiological pH values is provided.
Poly(N-Vinyl-2-Pyrrolidone-Polyethylene Glycol Diacrylate)-Chitosan
Recent years have witnessed significant advances made in the controlled drug delivery technology using polymeric hydrogels. Stimuli responsive polymeric hydrogels, which swell or shrink in response to changes in the environmental conditions, have been extensively studied and used as smart materials for various biomedical applications (Peppas Citation1997; Kurisawa, Matsuo, and Yui Citation1998). These polymeric hydrogels are being prepared from a limited number of synthetic polymers and their derivatives such as copolymers of methacrylic acid, acrylamide and N-isopropylacrylamide (Kopecek, Vacik, and Lim Citation1971; Suzuki and Tanaka Citation1990; Kokufuta, Zhang, and Tanaka Citation1991). Also, pH-responsive hydrogels based on N-vinyl pyrrolidone, polyethylene glycol diacrylate, and chitosan were prepared by Shantha and Harding (Citation2000) for controlled drug delivery. These interpolymeric hydrogels were synthesized by a free radical polymerization using azobisisobutyronitrile as initiator and N,N′-methylenebisacrylamide as a crosslinker (). Swelling studies indicated that these hydrogels were swollen more in simulated gastric fluid when compared with intestinal fluids. The in vitro release profiles of theophylline and 5-flluorouracil were established in simulated gastric fluid. The results also showed that more than 50% of the entrapped drugs were released in the first 2 hr at gastric pH and the rest of the drug release was slower.
Conjugated with Lipid and Other Biomolecules
The conjugation of lipid groups into chitosan molecule can create an amphiphilic self-aggregate molecule, which can be used as a drug delivery system. Uchegbu et al. (Citation2001) have synthesized palmitoyl glycol chitosan (GCP) by reacting glycol chitosan and sodium bicarbonate with palmitic acid N-hydroxysuccinimide in ethanol solution (). This conjugated polymer was converted into quaternized form by dispersing palmitoyl glycol in pyrolidine overnight and further reaction with methyl iodide and sodium iodide in alkali solution. The amphiphilic polymer was shown to be nonhemolytic when present as the liquid solution and relatively noncytotoxic, presenting a potential for solubilizing and entrapping of hydrophobic drugs by micelle forming ability. GCP hydrogel has been evaluated as an erodible controlled release system for the delivery of hydrophilic macromolecules (Martin et al. Citation2002). As the degree of amphiphilic palmitic acid increased hydrophobicity, and as a result slower release of model drug was reported, probably due to decreased water entrance and unfavorable diffusion of drug from the gel. They also have investigated the buccal absorption of denbufylline from this mucoadhesive GCP hydrogel, using the rabbit animal model and Carbopol (CP), denbufylline, used as control mucoadhesive polymers. Sustained release of drug was observed for at least 5 hr after dosing from GCP hydrogels compared with 1 hr release from control, showing retarding effect of palmitoylation.
Lee et al. (Citation1998) synthesized hydrophobically modified chitosan containing 5.1 deoxycholic acid groups per 100 anhydroglucose units by an EDC-mediated coupling reaction (). Chitosan was hydrophobically modified by deoxychlolic acid to yield self-aggregates has a small size with an unimodal size distribution in aqueous media. The feasibility of chitosan self-aggregates as a delivery carrier for the transfection of genetic material in mammalian cells also was investigated. Self-aggregates can form charge complexes when mixed with plasmid DNA. These self-aggregate/DNA complexes are considered to be useful for transfer of genes into mammalian cells in vitro and served as a good delivery system composed of biodegradable polymeric materials.
A novel injectable in situ gelling thermosensitive chitosan-b-glycerophosphate (C–GP) formulation has been proposed for tissue repair and drug delivery (Gariepy et al. Citation2002). The system can sustain the release of macromolecules over a period of several hours to a few days. However, with low-molecular-weight hydrophilic compounds, the release is generally completed within 24 hr. In this study, liposomes were added to the C-GP solution and their effect on the viscoelastic properties of the system and release kinetics of encapsulated carboxyfluorescein was investigated. The gelation rate and gel strength were slightly increased by the presence of the liposomes. The in vitro release profiles demonstrated controlled delivery over at least 2 weeks. The release rate strongly depended on the liposome size and composition (i.e., addition of cholesterol), and on the presence of phospholipase in the release medium. The kinetics was not substantially modified when using liposomes prepared with a negatively charged lipid or a lipid having a high phase transition temperature. These results indicate that the liposome-C-GP system rapidly gels at body temperature and can sustain the delivery of low-molecular-weight hydrophilic compounds.
CHITOSAN COMPLEXES
Polyelectrolyte complexes (PECs), are formed by the reaction of a polyelectrolyte with an oppositely charged polyelectrolyte in an aqueous solution. Polysaccharides, which have bulky pyranose rings and highly stereoregular configuration in their rigid, linear backbone chains, have been frequently studied (Ravikumar and Kumar Citation2001). PECs have numerous applications such as membranes, antistatic coatings, environmental sensors, chemical detectors and medical prosthetic materials. Their wide use as membranes for dialysis, ultrafiltration, and other solute separation processes are of special interest and also made it possible for their use in microcapsule membranes. Microcapsules can be used for mammalian cell culture and the controlled release of drugs, vaccines, antibiotics, and hormones (Yoshioka et al. Citation1995; Miwa et al. Citation1998; Colo et al. Citation2002). Few results have been reported about the formation of the PECs of alginate with chitosan under acidic condition. Although alginate/chitosan microcapsules have been studied extensively the studies have been limited in a narrow pH region due to the solubility of chitosan.
Lee, Park, and Ha (Citation1997) described a new procedure to overcome the solubility of chitosan. In this procedure, chitosan was heterogeneously deacetylated with a 47% sodium hydroxide solution and followed by a homogeneous reacetylation with acetic anhydrides to control the N-acetyl content of the chitosan having a similar molecular weight. The chitosan having different degrees of n-deacetylation were complexed with sodium alginate, and the formation behavior of the PECs was examined by the viscometry in various pH ranges. The maximum mixing ratio (Rmax) increased with a decrease in the degree of N-acetylation of the chitosan at the same pH, and with a decrease in pH at the same degree of N-acetylation. Similarly, N-acylated chitosan also was prepared. The N-acyl chitosans scarcely affected the formation behavior of PEC with sodium alginates. For the application of PECs produced, the microencapsulation of a drug was performed and the release property of drug was tested. The microcapsules were prepared in one step by the extrusion of a solution of a guaifenesin and sodium alginate into a solution containing calcium chloride and chitosan through interpolymeric ionic interactions.
The drug release during the drug-loaded microcapsules storage in saline was pH dependent, where the microcapsules were formed and the kind of N-acyl groups introduced to the chitosan. The microcapsules prepared at pH 4.8 showed a minimum release rate and the release rate varied with the pH due to the loop formation of backbone chains of polyelectrolytes. The N-acyl groups introduced to the chitosan enhanced the release rate remarkably.
Chitosan-poly(acrylic) acid (Ch-PAA) complex nanoparticles, which are well dispersed and stable in aqueous solution, were prepared under mild conditions without any organic solvents and surfactants by template polymerization of acrylic acid in chitosan solution (Hu et al. Citation2002). The physicochemical properties of nanoparticles were investigated by size exclusion chromatography, FT-IR, dynamic light scattering, transmission electron microscope, and zeta potential. The molecular weight of PAA in nanoparticles increased with the increase of molecular weight of chitosan, indicating that the polymerization of acrylic acid in the chitosan solution was a template polymerization. Also the prepared nanoparticles carried a positive charge and showed the size from 50 to 400 nm. These nanoparticles are stable under acidic and neutral conditions ranging from 4 to 8 and aggregate at pH > 9. The results of model drug, silk peptide, loading, and release experiments indicated that this system seems to be a promising vehicle for the administration of hydrophilic drugs, proteins, and peptides. Furthermore, due to their pH-sensitive behavior, these Ch-PAA nanoparticles are appropriate carriers for the delivery of drugs in the gastric cavity. Torrado et al. (Citation2004) have developed a Ch-PAA based controlled drug release system for gastric antibiotic delivery. Different mixtures of amoxicillin, chitosan, and PAA were used to obtain these polyionic complexes.
The swelling behavior and drug release from these complexes was studied. Swelling studies indicated that the extent of swelling was greater in the polyionic complexes than in the single chitosan formulations. The amoxicillin diffusion from the hydrogels was controlled by the polymer/drug interaction. The ability of these complexes to control the solute diffusion depends on the network mesh size, which has a significant effect in the overall behavior of the hydrogels. The results of this study suggested that these polyionic complexes are good systems for specific gastric drug delivery.
Yin and co-workers (Citation1996) reported chitosan/gelatine complex microspheres for controlled release of cimetidine. The drug-loaded microspheres were prepared by dissolving chitosan, gelatine (1:1 by weight), and cimetidine in 5% acetic acid. A certain amount of Tween 80 and liquid paraffin at water-to-oil ratio of 1:10 was added to the chitosan/gelatine mixture under agitation at 650 rpm at 30°C. A suitable amount of 25% aqueous glutaraldehyde solution was added to the inverse emulsion and maintained for 2 hr. Finally, the liquid paraffin was vaporized under vacuum to obtain microspheres. The drug release studies were performed in hydrochloric acid solution (pH 1.0) and potassium dihydrogen phosphate (pH 7.8) buffer at ionic strength 0.1 mol/L. In this study, a pH-dependent pulsed-release behavior of the chitosan/gelatine matrix was observed. Moreover, the drug release rate was controlled by the composition of the chitosan/gelatine and the degree of deacetylation of chitosan.
The PEC of oxychitin-chitosan microcapsules were prepared and assayed for the purpose of delivering miconazole (Genta et al. Citation2003). The drug-loaded micrcapsules formed upon contacting 4% oxychitin with a mixture of 2% chitosan solution, 1–2% calcium chloride, and 1% miconazole. The microcapsules were characterized by an external layer of polyelectrolyte complex and a core of oxychitin with miconazole crystals. The drug-loaded microcapsules were spherical, translucent, and stable over the observation period of 7 days in the wet state. Their swelling in various media amounted to 20–45% and made the microcapsules translucent. The microcapsules were disintegrated in the presence of sodium dodecyl sulphate at pH 7.1 and the delivery of miconazole was delayed compared with control. The microcapsules were also degraded by lysozyme that was partially inhibited by the oxychitin-rich fragments released by the external layer as well as by the oxychitin entrapped in the core.
Surini et al. (Citation2003) have designed an implant-controlled release system for protein drug delivery based on a polyion complex device composed of chitosan and hyaluronate (Ch-HA). The conditions that generated the greatest amount of the polyion solid complex were studied to ascertain the formation of polyion complex between chitosan and hyaluronate. In this study, the greatest amount of the polyion complex was formed at the weight ratio of 3 to 7 (Ch:HA) at pH 3.5. Furthermore, the Ch-HA pellets were prepared and then drug release from Ch-HA pellets was evaluated using insulin as a model drug. The results demonstrated that the insulin release from Ch-HA pellets was markedly influenced by both the change in the polymer mixing ratio and the total pellet weight, whereas compression pressure did not affect the release significantly. An artificial neural network and biharmonic spline interpolation were used to predict the actual relation between causal factors and the release rate constant of insulin.
Recently, PEC gel matrices have been investigated extensively for design of drug delivery systems to release a pharmacologically active agent in a predetermined, predictable, and reproducible fashion. Phosphorylated chitosan is one of the polyelectrolyte complex matrices that has attracted considerable interest because of its various advantages such as anti-inflammatory property, formation of metal complexes, and formation of anionic polyelectrolyte hydrogels (Lee and Shin Citation1991). Win et al. (Citation2003) have developed polyelectrolyte complex gel beads based on phosphorylated chitosan for controlled release of ibuprofen in oral administration. These beads were prepared from soluble phosphorylated chitosan by using an ionotropic gelation with counter polyanion, tripolyphophate at pH 4. The release percents of ibuprofen from phosphorylated chitosan gel beads were increased as the pH of the dissolution medium increased. The release rate of ibuprofen at pH 7.4 was higher than the release rate at pH 1.4 due to the ionization of phosphate groups and high solubility of ibuprofen at pH 7.4. These results suggest that the phosphorylated chitosan gel beads may be useful for controlled drug delivery system through oral administration by avoiding the drug release in the highly acidic gastric fluid region of the stomach.
CHITOSAN-COATED MATERIALS
To prevent the loss of encapsulated material, the microcapsules should be coated with another polymer that forms a membrane at the bead surface. The most well known and promising system is encapsulation of alginate beads with PLL. Because this system has a high cost, other systems such as alginate beads coated with chitosan or its derivatives have been developed. Because of the high affinity of chitosan for cell membranes, several investigators (Hughes Citation1975; Olson et al. Citation1988) have used chitosan derivatives as coating agents for liposome formulation with particle sizes below 1 μ m create hybrid systems frequently termed chitosomes. Negatively charged liposomes strongly adsorb soluble chitosan upon incubation, significantly changing surface properties and suspension stability. Although chitosan does not play a direct role in the initial nanoencapsulation of the therapeutic agent, the effects of the chitosan coating are critical to the ultimate success of this drug delivery system. Henriksen, Smistad, and Karlsen (Citation1994) provided the first thorough examination of chitosan-liposome interactions. Using an L-α -phosphatidylcholine/L-α -phosphatidyl-DL-glycerol liposome system, the study employed two molecular weights of chitosan to systematically investigate the effects of polymer concentration and mixing strategy on particle size, zeta potential, and stability. The result showed that the most stable coating is created when liposomes are added to an excess solution of high molecular weight chitoan.
The earliest work involving the effects of chitosan adsorption on negatively charged colloidal surfaces, however, took place in the field of lipid emulsions. Faldt, Bergenstahl, and Claesson (Citation1993) provided the first experimental investigations of this nature, using soybean oil emulsions (∼450 nm in size) coated with different combinations of cholesterol, phosphatidylcholine, and glycocholic acid. The interaction between these surfaces and chitosan was predominantly electrostatic and strongly dependent upon pH.
Alonso et al. (Citation1997) studied the formation of chitosan-coated nanoemulsions, chitosan-coated poly-(ε -caprolactone) (Pε CL) nanocapsules, and chitosan-coated PLGA nanoparticles. The formation of these colloidal carriers was based on the solvent displacement and solvent evaporation techniques. For PLGA nanoparticles, chitosan molecules anchor to the surface due to entanglements between polymer chains, thus resulting in a core-coated structure. In nanoemulsions and nanocapsules, chitosan adsorption was found to be highly mediated by its ionic interaction with phospholipids molecules located at the surface of these colloidal systems. Similarly, the encapsulation of various molecules (hemoglobin, bovine serum albumin, and dextrans with various molecular weights) in calcium alginate beads coated with chitosan has been reported (Huguet et al. Citation1994, Citation1996, Citation1996a). Their release has been compared and the influence of the conformation, the chemical composition, and the molecular weight of the encapsulated materials has been analysed.
Major research issues in protein delivery include the stabilization of proteins in delivery devices and the design of appropriate protein carriers to overcome mucosal barriers. Vila et al. (Citation2002) have attempted to combine both issues through new biodegradable polymer nanoparticles, such as PEG-coated PLA nanoparticles, chitosan coated PLGA nanoparticles, and chitosan nanoparticles. In this study, these nanoparticles were tested for their ability to load proteins, to deliver them in an active form, and to transport them across the nasal and intestinal mucosae. Additionally, the stability of some of these nanoparticles in simulated physiological fluids was studied. Results showed that the PEG coating improves the stability of PLA nanoparticles in the gastrointestinal fluids and helps the transport of the encapsulated protein, tetanus toxoid, across the intestinal and nasal mucosae. Furthermore, intranasal administration of these nanoparticles provided high and long-lasting immune responses.
On the other hand, the coating of PLGA nanoparticles with chitosan improved the stability of the particles in the presence of lysozyme and enhanced the nasal transport of the encapsulated tetanus toxoid. Finally, nanoparticles made solely of chitosan also were stable upon incubation with lysozyme. Moreover, these particles were very efficient in improving the nasal absorption of insulin as well as the local and systemic immune responses to tetanus toxoid, following intranasal administration. In summary, these results showed that a rational modification in the composition and structure of the nanoparticles, using safe materials, increases the prospects of their usefulness for mucosal protein delivery and transport.
GENE DELIVERY SYSTEM
An emerging application of chitosan-based colloidal systems is in gene delivery. In an effort to avoid the problems commonly associated with viral-mediated transfection, a number of groups have investigated cationic polymers as condensing agents for DNA. While such systems have shown some capabilities of facilitating transgene expression, polymer toxicity remains a major hurdle. Given the numerous studies reporting the low toxicity and biocompatibility of chitosan (Knapczyk et al. Citation1989; Hirano et al. Citation1989, Citation1990; Bersch et al. Citation1995; Weiner Citation1992), recent interest has focused on this natural polycation as a gene delivery system. Mumper et al. (Citation1995) were the first to propose chitosan as a gene delivery system. Gentle mixing, followed by incubation, of chitosan and DNA solution generated “broad” distribution of chitosan-DNA particulate complexes with mean sizes between 100 and 600 nm. Since particle formation was elicited solely by the tropism of the two oppositely charged macromolecules for one another, these particles were termed as complexes. Sato et al. (Citation1996b) further studied the nature of this complex and its uptake efficiency. Although chitosan complexes dramatically promoted DNA uptake in Hela cells (44% uptake versus 3% for naked DNA), they were unable to increase the DNA uptake in human blood monocytes (10 versus 9%). This group later showed that, despite the lower uptake efficiency, chitosan-DNA mixtures were far superior to polygalactosamine-DNA complexes in transfecting A549 cells.
Florea et al. (Citation2000) have prepared trimethyl chitosan oligomers (TMO) of 40% and 50% degrees of quaternization, respectively. These oligomers were examined for their potency as DNA carrier systems in two cell lines, COS-1 and Caco-2. The result showed that TMO proved to be superior to oligomeric chitosan in transfecting COS-1 cells; however, none of the used chitosan/DNA and lipofectin/DNA complexes was able to increase transfection efficiency in differentiated Caco-2 cells. Similarly, trimethylated chitosan (80% degree of quaternization) polymers, bearing antennary galactose residues through a 6-O-linked carboxymethyl group, also were examined as DNA carrier (Murata, Ohya, and Ouchi Citation1997). The complexes were tested for Hep-G2 cells, which are expressing the galactose receptor, and expression of β -galactosidase activity. The complexes efficiently transfected the Hep-G2 cells and the transfection efficiency was inhibited significantly in the presence of an inhibitor indicating that the conjugates were specifically internalized via the galactose receptor present on the cellular surface of Hep-G2 cells.
Galactosylated chitosan-graft-dextran DNA complexes were prepared (Park et al. Citation2000). Galactose groups were chemically bound to chitosan for liver specificity and dextran was grafted to increase the complex stability in water. This system could efficiently transfect Chang liver cells expressing the asialoglycoprotein receptor in vitro, indicating a specific interaction of the galactose ligands bound to chitosan with receptor. Roy, Nao, and Leong (Citation1997) showed that chitosan-DNA nanospheres could transfect HEK 293, IB3, and HTE cell lines, though at lower levels than lipofectamine controls. From this basic construct, several modifications were investigated. Although the coencapsulation of chloroquine significantly increased the transfection efficiency of another natural, cationic polymer (gelatine), chitosan did not substantially benefit from the same coincorporation (Mao et al. Citation1996; Leong et al. Citation1998). In the same studies, direct surface coupling of the transferring ligand, shown to augment transfection with other polycation-based vectors (Zenke et al. Citation1990), likewise caused a negligible increase in transgene expression with chitosan nanospheres, as did the coupling of mannose-6-phosphate (Mao et al. Citation1997).
Chitosan nanoparticles may be suitable carriers for DNA vaccines in mucosal immunization. Nanoparticles made from high molecular weight chitosan and pCMVArah2 plasmid DNA encoding for a major peanut allergen elicited secretory IgA and serum IgG2a production in mice after oral administration (Roy et al. Citation1999). Challenge tests showed less severe and delayed anaphylactic responses in sensitized mice immunized with chitosan/pCMVArah2, than in the control group (nontreated animals) or in mice treated with chitosan/DNA lacking the Arah2 gene. In vitro studies in a cell culture model showed features of intestinal Peyer's patches transcellular transport of chitosan/ DNA nanoparticles, that depended on the molecular weight of the chitosan used, pH of the cell culture medium, and conjugation of transferring to the nanoparticles (Kerneis et al. Citation1997; Kadiyala et al. Citation2000). In another study, oral application of chitosan nanoparticles bearing a plasmid encoding for blood coagulation factor-IX (FIX) resulted in detectable FIX plasma levels, which gradually declined over 14 days (Okoli, Hortelano, and Leong Citation2000).
CONCLUSIONS
Chitosan is an abundant natural based polymer, obtained by alkaline N-deacetylation of chitin. The physical and chemical properties of chitosan, such as inter- and intramolecular hydrogen bonding and the cationic charge in acidic medium, makes this polymer attractive for conventional and novel pharmaceutical products. Thus far, a number of chitosan-based colloidal systems are promising carriers for bioactive molecules. As a bioadhesive polymer and having antibacterial activity, chitosan is a good candidate for oral cavity drug delivery. Also, because of its favorable biological properties such as nontoxicity, biocompatibility, and biodegradability, chitosan is a promising candidate for the enhancement of absorption of drugs using buccal delivery system. Chitosan microspheres can reside longer in the stomach and allow for stomach-specific drug delivery. Chitosan acts as an absorption enhancer in the intestine by increasing the residence time of dosage forms at mucosal sites, inhibiting proteolytic enzymes, and increasing the permeability of protein and peptide drugs across mucosal membranes. Researchers have reported that chitosan is degraded by microorganisms, that are present in the colon (Tozaki et al. Citation1997, Citation1999; Yamamoto et al. Citation2000). Thus, this polymer could have promising application in colon-specific drug delivery.
A number of in vitro and in vivo studies showed that chitosan is a suitable material for efficient nonviral gene and DNA vaccine delivery. As a result of the physical, chemical, and biological properties, chitosan has been used in many different formulations for drug and gene delivery in the gastrointestinal tract. A better understanding of the mechanisms of action of these novel vehicles will provide a basis for their further optimization, thus opening more exciting opportunities for improving the administration of macromolecules. We anticipate that more uses of chitosan will be forthcoming as additional derivatives are synthesized and newer formulations are developed.
M. Prabaharan thanks the Portugese Foundation for Science and Technology, Government of Portugal, for awarding a Post-Doctoral Research Associateship.
REFERENCES
- Akbuga J., Durmaz G. Preparation and evaluation of cross-linked chitosan microspheres containing furosemide. Intl. J. Pharm. 1994; 111: 217–222
- Alonso M. J., Calvo P., Remunan C., Jato J. L. V. Stabilization of colloidal systems by the formation of ionic lipid-polysaccharide complexes. EP 0 771 566 A1, 1997
- Alonso M. J., Calvo P., Remunan C., Jato J. L. V. Application of nanoparticles based on hydrophilic polymers as pharmaceutical forms. EP 0 860 166 A1, 1998
- Artursson A., Lindmark T., Davis S. S., Illurn L. Effect of chitosan on the permeability of mono layers of intestinal epithelial cells (Caco-2). Pharm. Res. 1994; 11: 1358–1361, [PUBMED], [INFOTRIEVE], [CROSSREF]
- Bersch P. C., Nies B., Liebendorfer A. Evaluation of the biological properties of different wound dressing materials. J. Mater. Sci. Med. 1995; 6: 231–240
- Berthold A., Cremer K., Kreuter J. Influence of cross-linking on the acid stability and physicochemical properties of chitosan microspheres. STP Pharm. Sci. 1996; 6: 358–364
- Berthold A., Cremer K., Kreuter J. Preparation and characterization of chitosan microspheres as drug carrier for prednisolone sodium phosphate as model for anti-inflammatory drugs. J. Control. Rel. 1996a; 39: 17–25, [CROSSREF]
- Blanco D., Alonso M. J. Development and characterization of protein-loaded poly (lactide-co-glycolide) nanospheres. Euro. J. Pharm. Biopharm. 1997; 43: 287–294, [CROSSREF]
- Bodmeier R., Chen H., Paeratakul O. A novel approach to the delivery of microparticles or nanoparticles. Pharm. Res. 1989; 6: 413–417, [PUBMED], [INFOTRIEVE], [CROSSREF]
- Borchard G. Chitosan for gene delivery. Adv. Drug Del. Rev. 2001; 52: 145–150, [CROSSREF]
- Calvo P., Remunan C., Jato J. L. V., Alonso M. J. Novel hydrophilic chitosan-polyethylene oxide nanoparticles as protein carriers. J. Appl. Polym. Sci. 1997; 63: 125–132, [CROSSREF]
- Calvo P., Bougaba A., Appel M., Fattel E., Alonso M. J., Couvreur P. Oligonucleotide-chitosan nanoparticles as new gene therapy vector. Proceedings of 2nd world Meeting APGI/APV. International Association for Pharmaceutical Technology, Paris 1998
- Carreno B. G., Duncan R. Evaluation of biological properties of soluble chitosan and chitosan microspheres. Intl. J. Pharm. 1997; 148: 231–240, [CROSSREF]
- Chandy T., Sharma C. P. Chitosan beads and granules for oral sustained delivery of nifedipine: in vitro studies. Biomaterials 1992; 13: 949–952, [PUBMED], [INFOTRIEVE], [CROSSREF]
- Chandy T., Sharma C. P. Chitosan matrix for oral sustained delivery of ampicillin. Biomaterials 1993; 14: 939–944, [PUBMED], [INFOTRIEVE], [CROSSREF]
- Chandy T., Sharma C. P., Sunny M. C. Inhibition of platelet adhesion to glow discharge modified surfaces. J. Biomat. Appln. 1987; 1: 533–552
- Colo G. D., Zambito Y., Burgalassi S., Serafini A., Saettone M. F. Effect of chitosan on in vitro release and ocular delivery of ofloxacin from erodible inserts based on poly(ethylene oxide). Intl. J. Pharm. 2002; 248: 115–122, [CROSSREF]
- Dunn E. J., Zang X., Sun D., Goosen M. F. A. Synthesis of N-(aminoalkyl) chitosan for microcapsules. J. Appl. Polym. Sci. 1993; 50: 353–365, [CROSSREF]
- Faldt P., Bergenstahl B., Claesson P. M. Stabilization by chitosan of soybean oil emultion coated with phospholipids and glycocholic acid. Coll. Surf. 1993; 71: 187–195, [CROSSREF]
- Fernandez R. U., Calvo P., Jato J. R. V., Alonso M. J. Enhancement of nasal absorption of insulin using chitosan nanopartilces. Pharm. Res. 1999; 16: 1576–1581, [CROSSREF]
- Fernandez R. U., Romani D., Calvo P., Jato J. R. V., Alonso M. J. Development of a freeze-dried formulation of insulin loaded chitosan nanoparticles intended for nasal administration. STP Pharm. Sci. 1999a; 9: 429–436
- Florea B. I., Thanou M., Geldof M., Meaney C., Junginger H. E., Borchard G. Modified chitosan oligosaccharides as transfection agents for gene therapy of cystic fibrosis. Proceedings of 27th International Symposium on Controlled Release of Bioactive Materials. Controlled Release Society, Paris 2000
- Gariepy E. R., Leclair G., Hildgen P., Gupta A., Loroux J. C. Thermosensitive chitosan based hydrogel containing liposomes for the delivery of hydrophilic molecules. J. Control. Rel. 2002; 82: 373–383, [CROSSREF]
- Genta I., Costantini M., Asti A., Conti B., Montanari L. Influence of glutaraldehyde on drug release and mucoadhesive properties of chitosan microspheres. Carbohydrate Polym. 2000; 36: 81–88, [CROSSREF]
- Genta I., Perugini P., Modena T., Pavanetto F., Castelli F., Muzzarelli R. A. A., Muzzarelli C., Conti B. Miconazole-loaded 6-oxychitin-chitosan microcapsules. Carbohydrate Polym. 2003; 52: 11–18, [CROSSREF]
- Gupta K. C., Ravikumar M. N. V. Structural changes and release characteristics of cross-linked chitosan beads in response to solution pH. J. M.S. Pure and Appl.Chem. 1999; A36: 827–841, [CROSSREF]
- Gupta K. C., Ravikumar M. N. V. Drug release behavior of beads and microgranules of chitosan. Biomaterials 2000; 21: 1115–1119, [PUBMED], [INFOTRIEVE], [CROSSREF]
- Gupta K. C., Ravikumar M. N. V. Preparation, characterization and release profiles of pH-sensitive chitosan beads. Polym. Intl. 2000a; 49: 141–146, [CROSSREF]
- Gupta K. C., Ravikumar M. N. V. Semi-interpenetrating polymer network beads of cross-linked chitosan-glycine for controlled release of chlorphenramine maleate. J. Appl. Polym. Sci. 2000b; 76: 672–683
- Hayashi T., Ikada Y. Protease immobilization onto porous chitosan beads. J. Appl. Polym. Sci. 1991; 42: 85–92, [CROSSREF]
- Hejazi R., Amiji M. Chitosan-based gastrointestinal delivery systems. J. Control. Rel. 2003; 89: 151–165, [CROSSREF]
- Henriksen I., Smistad G., Karlsen J. Interactions between liposomes and chitosan. Intl. J. Pharm. 1994; 101: 227–236, [CROSSREF]
- Hirano H., Seino H., Akiyama I., Nonaka I. A biocompatible material for oral and intravenous administration. Chitosan, C. G. Gebelein, R. L. Dunn. Plenum Press, New York 1990; 283–289
- Hirano S., Seino H., Akiyama I., Nonaka I. Biocompatibility of chitosan by oral and intravenous administration. Polym. Eng. Sci. 1989; 59: 897–901
- Holme K., Hall L. Chitosan derivatives bearing C10 alkyl glycosides branches: A temperature induced gelling polysaccharide. Macromolecules 1991; 24: 3828–3833
- Hornof M. D., Kast C. E., Schnurch A. B. In vitro evaluation of the viscoelastic properties of chitosan-thioglycolic acid conjugates. Euro. J. Pharm. and Biopharm. 2003; 55: 185–190, [CROSSREF]
- Hu Y., Jiang X., Ding Y., Ge H., Yuan Y., Yang C. Synthesis and characterization of chitosan-poly (acrylic acid) nanoparticles. Biomaterials 2002; 23: 3193–3201, [PUBMED], [INFOTRIEVE], [CROSSREF]
- Hughes R. C. The complex carbohydrates of mammalian cell surfaces and their biological roles. Essays Biochem. 1975; 11: 1–36, [PUBMED], [INFOTRIEVE]
- Huguet M. L., Dellacherie E. Calcium alginate beads coated with chitosan, effect of structure encapsulated materials on their release. Process Biochem. 1996; 31: 745–751, [CROSSREF]
- Huguet M. L., Groboillot A., Neufeld R. J., Poncelet D., Dellacherie E. Hemoglobin encapsulation in chitosan/calcium alginate beads. J. Appl. Polym. Sci. 1994; 51: 1427–1432, [CROSSREF]
- Huguet M. L., Neufeld R. J., Dellacherie E. Calcium alginate beads coated with polycationic polymers: comparison of chitosan and DEAE-dextran. Process Biochem. 1996a; 31: 347–353, [CROSSREF]
- Ida G., Monica C., Annalia A., Bice C., Luisa M. Influence of glutaraldehyde on drug release and mucoadhesive properties of chitosan microspheres. Carbohydrate Polym. 1998; 36: 81–88, [CROSSREF]
- Illum L. Chitosan and its use as a pharmaceutical excipient. Pharm. Res. 1998; 15: 1326–1331, [PUBMED], [INFOTRIEVE], [CROSSREF]
- Illum L., Farraj N. F., Davis S. S. Chitosan as a novel nasal delivery system for peptide drugs. Pharm. Res. 1994; 11: 1186–1189, [PUBMED], [INFOTRIEVE], [CROSSREF]
- Janes K. A., Calvo P., Alonso M. J. Polysaccharide colloidal partilce as delivery systems for macromolecules. Adv. Drug Deliv. Rev. 2001; 47: 83–97, [PUBMED], [INFOTRIEVE], [CROSSREF]
- Janes K. A., Fresneau M. P., Marazuela A., Fabra A., Alonso M. J. Chitosan nanoparticles as delivery systems for doxorubicin. J. Control. Rel. 2001a; 73: 255–267, [CROSSREF]
- Kadiyala I., Roy K., Rice J., Leong K. W. Transport kinetics of DNA nanopartilces in a Caco-2-peyer's patch model of intestinal epithelium. Proceedings of 27th International Symposium on Controlled Release of Bioactive Materials. Controlled Release Society, Paris 2000
- Kast C. E., Schnurch A. B. Thiolated polymers- thiomers, development and in vitro evaluation of chitosan-thioglycolic acid conjugates. Biomaterials 2001; 22: 2345–2352, [PUBMED], [INFOTRIEVE], [CROSSREF]
- Kato Y., Onishi H., Machida M. A novel water-soluble N-succinyl chitosan-mitomycin C conjugate prepared by direct carbodiimide coupling: physicochemical properties, antitumor characteristics and systemic retention. STP Pharm. Sci. 2000; 10: 133–142
- Kerneis S., Bogdanova A., Kraehenbuhl J. P., Pringault E. Conversion by Peyer's patch lymphocytes of human enterocytes into M cells that transport bacteria. Science 1997; 277: 949–952, [PUBMED], [INFOTRIEVE], [CROSSREF]
- Knapczyk J., Kroweczynski L., Krzck J., Brzeski M., Nirnberg E., Schenk D., Struszcyk H. Chitin and chitosan: source, chemistry, biochemistry, physical properties and applications. Requirements of Chitosan for Pharmaceutical and Biomedical Applications, G. Shak, T. Anthonsen, P. Sandford. Elsevier, London 1989; 657–663
- Koga D. Chitin enzymology-chitinase. Adv. Chitin Sci. 1998; 3: 16–23
- Kokufuta E., Zhang Y. Q., Tanaka T. Saccharide sensitive phase transition of a lectin-loaded gel. Nature 1991; 351: 302–304, [CROSSREF]
- Kopecek J., Vacik J., Lim D. Permeability of membranes containing ionogenic group. J. Polym. Sci. 1971; 9: 2801–2815
- Kurisawa M., Matsuo Y., Yui N. Modulated degradation of hydrogels with thermo-responsive network in relation to their swelling behavior. Macromol. Chem. Phys. 1998; 199: 705–709
- Lee K. Y., Kwon I. C., Kim Y. H., Jo W. H., Jeong S. Y. Preparation of chitosan self-aggregates as a gene delivery system. J. Control. Rel. 1998; 51: 213–220, [CROSSREF]
- Lee K. Y., Park W. H., Ha W. S. Polyelectrolyte complexes of sodium alginate with chitosan or its derivatives. J. Appl. Polym. Sci. 1997; 63: 425–432, [CROSSREF]
- Lee Y. M., Shin E. M. Pervaporation separation of water-ethanol through modified chitosan membranes, IV. Phosphorylated chitosan membranes. J. Membrane Sci. 1991; 64: 145–152
- Lehr C. M., Bowstra J. A., Schacht E. H., Jungiger H. E. In vitro evaluation of mucoadhesive properties of chitosan and some other natural polymers. Intl. J. Pharm. 1992; 78: 43–48
- Leong K. W., Mao H. Q., Truong V. L., Roy K., Walsh S. M., August J. T. DNA-polycation nanospheres as non-viral gene delivery vehicles. J. Control. Rel. 1998; 53: 183–193, [CROSSREF]
- Lorenzo M. L., Remunan C. L., Jato J. L. V., Alonso M. J. Design of microencapsulated chitosan microspheres for colonic drug delivery. J. Control. Rel. 1998; 52: 109–118, [CROSSREF]
- Lubben I. M. V. D., Verhoef J. C., Borchard G., Junginger H. E. Chitosan and its derivatives in mucosal drug and vaccine delivery. Euro. J. Pharm. Sci. 2001; 14: 201–207, [CROSSREF]
- Lueben H. L., Leeuw B. J. D., Langemeyer B. W., Boer A. G. D., Verhoef J. C., Junginger H. E. Mucoadhesive polymers in peroral peptide drug delivery. VI. Carbomer and chitosan improve the intestinal absorption of the peptide buserelin in vivo. Pharm. Res. 1996; 13: 1668–1672, [CROSSREF]
- Mao H. Q., Roy K., Truong V. L., August J. T., Leong K. W. DNA-chitosan nanospheres, derivitization and storage stability. Proceedings of 24th International Symposium on Controlled Release of Bioactive Materials. Controlled Release Society, Stockholm 1997
- Mao H. Q., Roy K., Walsh S. M., August J. T., Leong K. W. DNA-chitosan nanoparticles for gene delivery. Proceedings of 23rd International Symposium on Controlled Release of Bioactive Materials. Controlled Release Society, Kyoto 1996
- Martin L., Wilson C. G., Koosha F., Tetley L., Gray A. I., Senel S., Uchegbu I. F. The release of model macromolecules controlled by the hydrophobicity of palmitoyl glycol chitosan hydrogels. J. Control. Rel. 2002; 80: 87–91, [CROSSREF]
- Mi F. L., Shyu S. S., Chen C. T., Schoung J. Y. Porous chitosan microsphere for controlling the antigen release of Newcastle disease vaccine: preparation of antigen-adsorbed microsphere and in vitro release. Biomaterials 1999; 20: 1603–1612, [PUBMED], [INFOTRIEVE], [CROSSREF]
- Mi F. L., Sung H. W., Shyu S. S. Synthesis and charecterization of a novel chitosan.based network prepared using naturally occuring crosslinker. J. Polym. Sci. Part A: Polym. Chem. 2000; 38: 2804–2814
- Mi F. L., Tan Y. C., Liang H. F., Sung H. W. In vivo biocompatibility and degradability of a novel injectable-chitosan-based implant. Biomaterials 2002; 23: 181–191, [PUBMED], [INFOTRIEVE], [CROSSREF]
- Miwa A., Ishibe A., Nakano M., Yamahira T., Itai S., Jinno S., Kawahara H. Development of novel chitosan derivatives as micellar carriers of taxol. Pharm. Res. 1998; 15: 1844–1850, [PUBMED], [INFOTRIEVE], [CROSSREF]
- Mumper R. J., Wang J., Claspell J. M., Rolland A. P. Novel polymeric condensing carriers for gene delivery. Proceedings of 22nd International Symposium on Controlled Release of Bioactive Materials. Controlled Release Society, Washington 1995
- Murata J., Ohya Y., Ouchi T. Design of quaternary chitosan conjugate having antennary galactose residues as a gene delivery tool. Carbohydrate Polymers 1997; 32: 105–109, [CROSSREF]
- Muzzarelli R. Natural chelating polymers. Chitosan, R. Muzzarelli. Pergamon Press, Oxford 1973; 144–176
- Muzzarelli R. A. A. Human enzymatic activities related to the therapeutic administration of chitin derivatives. Cell Mol. Life Sci. 1997; 53: 131–140, [PUBMED], [INFOTRIEVE]
- Nishimura K., Nishimura S., Seo H., Nishi N., Tokura S., Azuma I. Macrophage activation with multiporous beads prepared from partially deacetylated chitin. J. Biomed. Mater. Res. 1986; 20: 1359–1372, [PUBMED], [INFOTRIEVE]
- Nishimura S., Miyura Y., Ren L., Sato M., Yamagishi A., Nishi N., Tokura S., Kurita K., Ishii S. An efficient method for the synthesis of novel amphiphilic polysaccharide by region and thermo selective modifications of chitosan. Chemistry letters 1993; 1623–1626
- Ohya Y., Shiratani M., Kobayashi H., Ouchi T. Release behavior of 5-fluorouracil from chitosan-gel nanospheres immobilizing 5-fluorouracil coated with polysaccharides and their cell specific cytotoxicity. Pure Appl. Chem. 1994; A31: 629–642
- Okoli G., Hortelano G., Leong K. W. Oral delivery of plasmid DNA encoding the factor IX gene. Proceedings of 27th International Symposium on Controlled Release of Bioactive Materials. Controlled Release Society, Paris 2000
- Olson R., Schwartzmiller D., Weppner W., Winandy R. Chitin and chitosan sources, chemistry, biochemistry, physical properties and applications. Biomedical Application of Chitin and its Derivatives, G. S. Braek, T. Antonsen, P. Sandford. Elsevier, London 1988; 813–828
- Onishi H., Nagai T., Machida Y. Applications of chitin and chitosan. Application of Chitin, Chitosan, and their Derivatives to Drug Carriers for Microparticulated or Conjugated Drug Delivery System, M. F. A. Goosen. Technomic Publishing Co., Lancaster 1997; 205–231
- Onishi H., Takahashi H., Yoshiyasu M., Machida Y., Nagai T. Macromolecular prodrugs of cytarabine and mitomycin C with chitosan, N-succinyl chitosan and 6-O-carboxymethyl-chitin as drug carriers. Chitin World, L. S. Karnicki, M. M. Brzeski, P. J. Bykowski, A. P. Wojtasz. Bremerhaven, Wirtchaftsverlag, NW 1994; 301–310
- Onishi H., Takahashi T., Yoshiyasu M., Machida Y. Preparation and in vitro properties of N-succinyl chitosan or carboxyl methyl chitin- mitomycin C conjugates microparticles with specified size. Drug. Dev. Ind. Pharm. 2001; 27: 659–667, [PUBMED], [INFOTRIEVE], [CROSSREF]
- Park Y. K., Park Y. H., Shin B. A., Choi E. S., Park Y. R., Akaike T., Cho C. S. Galactosylated chitosan-graft-dextran as hepatocyte targeting DNA carrier. J. Control. Rel. 2000; 69: 97–108, [CROSSREF]
- Peppas N. A. Hydrogels and drug delivery. Curr. Opin. Coll. Inter. Sci. 1997; 2: 531–537
- Ramos V. M., Rodriguez N. M., Rodriguez M. S., Heras A., Agullo E. Modified chitosan carrying phosphonic and alkyl groups. Carbohydrate Polymers. 2003; 51: 425–429, [CROSSREF]
- Ravikumar M. N. V., Kumar N. Polymeric controlled drug delivery systems: perspective issues and opportunities. Drug Devel. Ind. Pharm. 2001; 27: 1–18, [CROSSREF]
- Roldo M., Hornof M., Caliceti P., Schnurch A. B. Mucoadhesive thiolated chitosans as platforms for oral controlled drug delivery: synthesis and in vitro evaluation. Euro. J. Pharm. Biopharm. 2004; 57: 115–121, [CROSSREF]
- Roy K., Mao H. Q., Huang S. K., Leong K. W. Oral gene delivery with chitosan-DNA nanopartilces generates immunologic protection in a murine model of peanut allergy. Nature Med. 1999; 5: 387–391, [PUBMED], [INFOTRIEVE], [CROSSREF]
- Roy K., Mao H. Q., Leong K. W. DNA-chitosan nanospheres, transfection efficiency and cellular uptake. Proceedings of 24th International Symposium on Controlled Rellease of Bioactive Materials. Controlled Release Society, Stockholm 1997
- Sato M., Onishi H., Machida M., Nagai T. Preparation and drug release characteristics of the conjugates of mitomycin C with glycol-chitosan and n-succinyl chitosan. Biol. Pharm. Bull. 1996; 19: 241–245, [PUBMED], [INFOTRIEVE]
- Sato M., Onishi H., Takahara J., Machida Y., Nagai T. In vivo drug release and antitumor characteristics of water-soluble conjugates of mitomycin C with glycol-chitosan and N-succinyl-chitosan. Biol. Pharm. Bull. 1996a; 19: 1170–1177, [PUBMED], [INFOTRIEVE]
- Sato T., Shirakawa N., Nishi H., Okahata Y. Formation of a DNA/polygalactosamine complex and its interaction with cells. Chem. Lett. 1996b; 9: 725–726
- Schipper N. G. M., Hoogstraate J. A., Boer A. G. D., Varum K. M., Artursson P. Chitosan as absorption enhancers for poorly absorbable drugs. 2. Mechanism of absorption enhancement. Pharm. Res. 1997; 14: 923–929, [PUBMED], [INFOTRIEVE], [CROSSREF]
- Schnurch A. B., Guggi D., Pinter Y. Thiolated chitosans: development and in vitro evaluation of a mucoadhesive, permeation enhancing oral drug delivery system. J. Control. Rel. 2004; 94: 177–186, [CROSSREF]
- Schnurch A. B., Hornof M., Zoidl T. Thiolated polymers-thiomers: synthesis and in vitro evaluation of chitosan-2-iminothiolane conjugates. Intl. J. Pharm. 2003; 260: 229–237, [CROSSREF]
- Shantha K. L., Harding D. R. K. Preparation and in vitro evaluation of poly(N-vinyl-2-pyrrolidone-polyethylene glycol diacrylate)-chitosan interpolymeric pH-responsive hydrogels for oral drug delivery. Intl. J. Pharm. 2000; 207: 65–70, [CROSSREF]
- Sheehan J. C., Hlavka J. J. The use of water-soluble and basic carbodiimides in peptide synthesis. J. Org. Chem. 1956; 21: 439–441
- Shirahata K. Mitomycin basic studies. Derivatives and Activities, T. Taguchi. Kyowa Kikaku Tsushin, Tokyo 1984; 25–43
- Shu X. Z., Zhu K. J. The influence of multivalent phosphate structure on the properties of ionically cross-linked chitosan films for controlled drug release. Euro. J. Pharm. Biopharm. 2002; 54: 235–243, [CROSSREF]
- Sinha V. R., Singla A. K., Wadhawan S., Kaushik R., Kumria R., Bansal K., Dhawan S. Chitosan microspheres as a potential carrier for drugs. Intl. J. Pharm. 2004; 274: 1–33, [CROSSREF]
- Song Y., Onishi H., Machida Y. Synthesis and drug release characteristics of the conjugates of mitomycin C with N-succinyl-chitosan and carboxymethy-chitin. Chem. Pharm. Bull. 1992; 40: 2822–2825, [PUBMED], [INFOTRIEVE]
- Song Y., Onishi H., Machida Y., Nagai T. Particle characteristics of carboxymethyl chitin–mitomycin C conjugate and their distribution and histological effect on some tissues after intravenous administration. STP Pharm. Sci. 1995; 5: 162–170
- Song Y., Onishi H., Machida Y., Nagai T. Drug release and antitumor characteristics of N-succinyl chitosan-mitomycin C as an implant. J. Control. Rel. 1996; 42: 93–100, [CROSSREF]
- Surini S., Akiyama H., Morishita M., Nagai T., Takayama K. Release phenomena of insulin from an implantable device composed of a polyion complex of chitosan and sodium hyaluronate. J. Control. Rel. 2003; 90: 291–301, [CROSSREF]
- Suzuki A., Tanaka T. Phase transition in polymer gels induced by visible light. Nature 1990; 346: 345–347, [CROSSREF]
- Tien C. L., Lacroix M., Szabo P. I., Mateescu M. A. N-acylated chitosan: hydrophobic matrices for controlled drug release. J.Control. Rel. 2003; 93: 1–13, [CROSSREF]
- Tobio M., Gref R., Sanchez A., Langer R., Alonsa M. J. Stealth PLA-PEG nanoparticles as protein carriers for nasal administration. Pharm. Res. 1998; 15: 270–275, [PUBMED], [INFOTRIEVE], [CROSSREF]
- Tobio M., Sanchez A., Vila A., Soriano I., Evora C., Jato J. L. V. The role of PEG on the stability in digestive fluids and in vivo fate of PEG-PLA nanoparticles. Coll. Surf. Biointerf. 2000; 18: 315–323, [CROSSREF]
- Torrado S., Prada P., Torre P. M., Torrado S. Chitosan-poly(acrylic)acid polyionic complex: in vivo study to demonstrate prolonged gastric retention. Biomaterials 2004; 25: 917–923, [PUBMED], [INFOTRIEVE], [CROSSREF]
- Tozaki H., Fujita T., Odoriba T., Terabe A., Suzuki T., Tanaka C., Okabe S., Muranishi S. Colon-specific delivery of R68070, a new thromboxane synthase inhibitor, using using chitosan capsules, therapeutic effects against 2,4,6-trinitrobenzenesulfonic acid-induced ulcerative colitis in rats. Life Sci. 1999; 64: 1155–1162, [PUBMED], [INFOTRIEVE], [CROSSREF]
- Tozaki H., Komoike J., Tada C., Maruyama T., Terabe A., Suzuki T., Yamamoto A., Muranishi S. Chitosan capsules for colon-specific drug delivery, improvement of insulin absorption from the rat colon. J. Pharm. Sci. 1997; 86: 1016–1021, [PUBMED], [INFOTRIEVE], [CROSSREF]
- Uchegbu I. F., Sadiq L., Arastoo M., Gray A. I., Wang W., Waigh R. D., Schatzleina A. G. Quaternary ammonium palmitoyl glycol chitosan—a new polysoap for drug delivery. Intl. J. Pharm. 2001; 224: 185–189, [CROSSREF]
- Urrusuno R. F., Calvo P., Lopez C. R., Jato J. L. V., Alonso M. J. Enhancement of nasal absorption of insulin using chitosan nanoparticles. Pharm. Res. 1999; 16: 1576–1581, [CROSSREF]
- Vila A., Sanchez A., Tobio M., Calvo P., Alonso M. J. Design of biodegradable particles for protein delivery. J. Control. Rel. 2002; 78: 15–24, [CROSSREF]
- Watzke H. J., Dieschbourg C. Novel silica-biopolymer nanocomposites: the silica sol-gel process in biopolymer organogels. Adv. Coll. Interfac. Sci. 1994; 50: 1–14, [CROSSREF]
- Weiner M. L. An overview of the regulatory status and of the safety of chitin and chitosan as food and pharmaceutical ingredients. Advances in Chitin and Chitosan, C. J. Brine, P. A. Sanford, J. P. Zikakis. Elsevier, London 1992; 670–678
- Williams R. O., Barron M. K., Alonso M. J., Remunan C. L. Investigation of a Pmdi system containing chitosan microspheres and p134a. Intl. Pharm. 1998; 174: 209–222, [CROSSREF]
- Win P. P., Ya Y. S., Hong K. J., Kajiuchi T. Formulation and charecterization of pH sensitive drug carrier based on phosphorylated chitosan. Carbohydrate Polym. 2003; 53: 305–310, [CROSSREF]
- Yamaguchi R., Arai Y., Itoh T., Hirano S. Preparation of partially N-succinylated chitosans and their cross-linked gels. Carbo. Res. 1981; 15: 172–175, [CROSSREF]
- Yamamoto A., Tozaki H., Okada N., Fujita T. Colon-specific delivery of peptide drugs and anti-inflammatory drugs using chitosan capsules. STP Pharm. Sci. 2000; 10: 23–34
- Yao K., Peng T., Xu M., Yuan C. pH-dependent hydrolysis and drug release of chitosan/polyether interpenetrating polymer network hydrogel. Polym. Intl. 1994; 34: 213–219
- Yao K. D., Peng T., Yu J. J., Xu M. X., Goosen M. F. A. Microcapsules/Microspheres related to chitosan. Rev. Macromol. Chem. Phys. 1995; C35: 155–180
- Yin Y., Xu M., Chen X., Yao K. D. Drug release behavior of chitosan/gelatine network polymer microspheres. Chinese Sci. Bull. 1996; 41: 1266–1270
- Yoshioka H., Nonaka K., Fukada K., Kazama S. Chitosan derived polymer surfactants and their micellar properties. Biosci. Biotech. Biochem. 1995; 59: 1901–1904
- Zenke M., Steinlein P., Wagner E., Cotton M., Beug H., Birnstiel M. L. Receptor-mediated endocytosis of transferring-poly cation conjugates, an efficient to introduce DNA into hematopoietic cells. Proceedings of the 87th National Academy of Sciences. National Academy of Sciences, Washington 1990